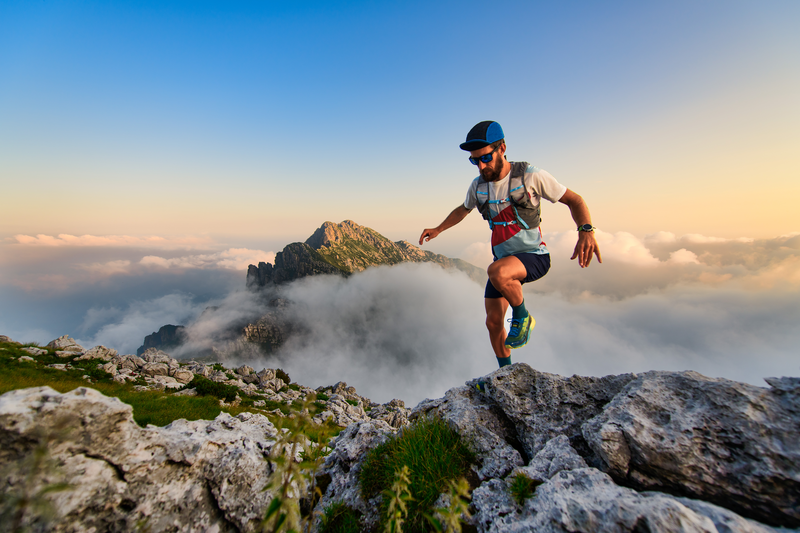
95% of researchers rate our articles as excellent or good
Learn more about the work of our research integrity team to safeguard the quality of each article we publish.
Find out more
ORIGINAL RESEARCH article
Front. Hum. Neurosci. , 13 June 2024
Sec. Cognitive Neuroscience
Volume 18 - 2024 | https://doi.org/10.3389/fnhum.2024.1394706
This article is part of the Research Topic The Cognitive Neuroscience of Aging: Where we are and where we are going View all 7 articles
Introduction: Although memory challenges in autistic individuals have been characterized recently, the functional connectivity of the hippocampus and ventral temporal lobe, two structures important for episodic and semantic memory functions, are poorly understood in autistic individuals. Moreover, age-related differences in the functional connectivity associated with these two memory networks are unrevealed.
Methods: The current study investigated age-related differences in intrinsic connectivity of the hippocampal and ventral temporal lobe (vTL) memory networks in well-matched ASD (n = 73; age range: 10.23–55.40 years old) and Non-ASD groups (n = 74; age range: 10.46–56.20 years old) from the open dataset ABIDE-I. Both theory-driven ROI-to-ROI approach and exploratory seed-based whole-brain approach were used.
Results and discussion: Our findings revealed reduced connectivity in ASD compared to Non-ASD peers, as well as an age-related reduction in the connectivity of hippocampal and vTL networks with triple networks, namely, the default mode network (DMN), the central executive network (CEN), and the salience network (SN), potentially underpinning their challenges in memory, language, and social functions. However, we did not observe reliable differences in age-related effects between the ASD and Non-ASD groups. Our study underscores the importance of understanding memory network dysfunctions in ASD across the lifespan to inform educational and clinical practices.
Individuals diagnosed with autism spectrum disorder (ASD) often experience cognitive and social difficulties that significantly impact their daily functioning (Di Martino et al., 2009; Zablotsky et al., 2015; Danker et al., 2016; Cai et al., 2020). In addition to commonly recognized symptoms such as repetitive behaviors, restricted interests, and challenges in socio-communicative functions, autistic individuals frequently exhibit atypical memory functions (Lynn et al., 2018; Desaunay et al., 2020; Liu et al., 2023; Chen et al., 2024). Although their behavioral profiles of memory difficulties are now well characterized in the literature (Desaunay et al., 2020), there remains a notable gap in our understanding of the neural correlates underlying their memory challenges (Bowler et al., 2011; Cooper et al., 2017; Banker et al., 2021; Desaunay et al., 2023). Furthermore, little attention has been given to investigating how these neurobiological underpinnings change over the lifespan, particularly regarding memory functions in autistic individuals. Thus, this study aims to elucidate the age-related alterations in intrinsic brain connectivity associated with memory functions in autistic individuals, compared to their non-autistic counterparts.
Among the various types of memory functions, challenges in episodic memory have received considerable attention in the research on autistic individuals (Lind and Bowler, 2010; Griffin et al., 2022). Studies have consistently indicated that autistic individuals often exhibit challenges in episodic memory compared to their Non-ASD peers (Boucher et al., 2012; Boucher and Anns, 2018). For instance, one study revealed that a group of autistic adults, with an average age of 36, showed diminished relational encoding abilities, consequently contributing to their decreased recollection (Gaigg et al., 2015). Similarly, investigations have suggested that episodic memory difficulties may manifest in autistic children as early as the age of 6 (Naito et al., 2020). Given the established role of the hippocampus in episodic memory (Scoville and Milner, 1957; Bird and Burgess, 2008; Voss et al., 2017), previous literature also explored the role of the hippocampus in ASD. Studies have observed increased activation patterns in the hippocampus during episodic memory tasks in autistic individuals (Hogeveen et al., 2020) and reported reduced functional connectivity of the hippocampus compared to Non-ASD (Cooper et al., 2017; Hogeveen et al., 2020). Some studies even revealed increased hippocampal activations in ASD when they were exposed to sensory stimuli (Green et al., 2013). Collectively, these findings suggest that hippocampal dysfunction likely contributes to the challenges in episodic memory of autistic individuals.
Considering its crucial role in episodic memory functions, numerous studies have investigated the structure and function of the hippocampus in ASD across different developmental stages (Richards et al., 2020; Xu et al., 2020). In early childhood (24–72 months), research suggests that male individuals with ASD have a larger right hippocampus compared to non-autistic peers (Reinhardt et al., 2020). Similar findings have been reported for autistic individuals in late childhood (7.5–18.5 years old; Schumann et al., 2004) and young adulthood (6.5–27 years old; Xu et al., 2020). However, studies focusing on older adult groups (mean age ≥ 50 years old) have indicated smaller hippocampal volumes in ASD and a faster rate of reduction compared to the matched Non-ASD (Braden et al., 2017; Van Rooij et al., 2018; Pagni et al., 2022). Despite extensive research on resting-state functional connectivity in ASD (Hull et al., 2017), there is limited understanding regarding the resting-state functional connectivity of the hippocampus in ASD and relevant age-related effects. Some studies have reported greater connectivity of subcortical regions, including the hippocampus, only in young autistic children (Wiggins et al., 2011; Nomi and Uddin, 2015; Liu et al., 2023). Meanwhile, one study noted reduced functional connectivity of the hippocampus in young autistic adults compared to non-autistic individuals (Pereira et al., 2018). Therefore, a more comprehensive analysis is needed to examine the age-related differences in resting-state hippocampal connectivity across a broader age range beyond children and young adults.
In addition to episodic memory, aging in typically developing (TD) populations is often linked with semantic memory decline (Nilsson, 2003). While the neurobiological underpinnings of episodic memory are predominantly rooted in the medial temporal lobe (Voss et al., 2017), semantic memory relies on a distributed brain network with a critical hub in the ventral part of the temporal lobe (vTL), especially its anterior segment (Rogers et al., 2004; Binney et al., 2010; Visser et al., 2012; Lambon Ralph et al., 2016; Chen et al., 2017). For instance, studies have consistently demonstrated that profound semantic memory deficits in semantic dementia coincide with atrophy in the bilateral ventral temporal lobes (Hodges et al., 1992; Patterson et al., 2006; Chen and Rogers, 2014; Lambon Ralph et al., 2016). Additionally, research has highlighted a significant association between reduced lateral temporal surface area and aging, further suggesting the important role of the vTL in memory over aging (Hogstrom et al., 2013).
In contrast to episodic memory, previous studies indicated that semantic memory was preserved in autistic individuals (Coderre et al., 2017; Gladfelter and Goffman, 2018), leading to a lack of research on semantic memory or the vTL in ASD. However, recent behavioral studies have shown that ASD participants generally exhibit lower semantic fluency compared to TD, suggesting challenges in utilizing semantic knowledge for language functions to some extent (Ehlen et al., 2020; Foldager et al., 2023). Neuroimaging studies on vTL changes over aging in ASD are quite limited. Only a couple of studies suggested that the temporal cortex tends to be thinner in autistic individuals over aging (Wallace et al., 2010), and a greater local functional connectivity in the right temporal pole was observed in adults but not in children with ASD (Dajani and Uddin, 2016). Consequently, there is a pressing need for a comprehensive characterization of vTL-based connectivity for semantic memory across different age groups in ASD, particularly in comparison to the hippocampal network underlying episodic memory.
In sum, the present study aimed to address significant gaps in the literature by investigating age-related differences in intrinsic connectivity within the hippocampal memory network (related to episodic memory) and the ventral temporal lobe memory network (related to semantic memory) in ASD compared to Non-ASD populations. First, we employed a theory-driven network approach to examine the intrinsic connectivity of hippocampal and vTL nodes with brain regions that were shown to be significant in ASD. Specifically, our theory-driven network analysis would focus on brain regions in the Default Mode Network (DMN), Central Executive Network (CEN), and Salience Network (SN), known as the triple networks associated with neural dysfunctions in ASD (Uddin et al., 2011; Menon, 2018). Given the findings in the literature, we hypothesized that ASD would show aberrant functional connectivity between hippocampal and vTL memory nodes with brain regions in triple networks compared to Non-ASD. More importantly, these connectivity patterns would be associated with their age, and an Age-by-Group interaction could be observed in some connectivity. Next, we utilized a seed-to-whole-brain approach to explore the atypical functional connectivity of hippocampal and vTL nodes with brain regions outside the triple networks in ASD compared to Non-ASD. Then, we explored whether these atypical patterns of connectivity between ASD and Non-ASD would show age-related differences. By addressing these literature gaps, we aim to contribute to a more comprehensive understanding of the neurobiological basis of memory functions in ASD across the lifespan.
All the participants were selected from the open-access dataset, The Autism Brain Imaging Data Exchange (ABIDE I) (Di Martino et al., 2014) from multiple sites (USM, NYU, CALTECH, CMU, YALE, UM_1, UM_2, and SDSU) since these sites used the same parameters for repetition time (TR = 2s) and scanning sequence (interleaved). The initial sample was a total of 587 individuals with 280 in the ASD group and 307 in the Non-ASD group. To ensure the quality of the fMRI data, we only included participants: (a) with an age > 10 years; (b) with a full-scale IQ (FIQ) score above 70, and (c) with the framewise displacement of scans below 0.5 mm. After screening, there were 493 participants with 223 in the ASD and 270 in the Non-ASD groups. Using an in-house matching script, we then selected 75 participants with an ASD diagnosis and 75 Non-ASD individuals. We matched the gender, age, full-scale IQ, distribution across multiple sites, and their movement parameters (i.e., framewise displacement; FD) in the resting-state fMRI scan between the diagnosis groups (ASD vs. Non-ASD) and also across different age ranges (participants in the 10∼19, 20∼30, and 30+ age groups). The individuals in the Non-ASD group were not reported with other neurological or psychological disorders, whereas two autistic individuals were reported with ADHD, two with dysthymia, and five with anxiety, mood disorders, or phobia. After visual inspection of the imaging data, 2 participants from the ASD and 1 participant from the Non-ASD groups were excluded due to the low quality of the fMRI data. The detailed demographic information and the movement parameters of the final sample are presented in Table 1.
Table 1. Demographic, cognitive profiles, and movement parameters of matched age groups for ASD and Non-ASD groups.
The resting-state fMRI data from ABIDE I were downloaded and analyzed using SPM12 (Ashburner et al., 2014). The first 8 volumes were not analyzed to allow for T1 equilibration. The data then were preprocessed with the standard pipeline, including correction for slice timing, motion realignment, and band filtering (0.008∼0.1 Hz). The individual data were then normalized into MNI152 space and then smoothed with a Gaussian kernel with a full width at half maximum (FWHM) of 6 mm.
We chose bilateral hippocampus (−/+24, −14, −20) for the hippocampal network of episodic memory (Kahn et al., 2008; Liu et al., 2023), and bilateral vTL (L: −45, −57, −15; R: 45, −36, −24) for the semantic memory based on previous literature (Visser and Lambon Ralph, 2011). In addition, we chose the following coordinates for the triple network nodes based on the literature (Uddin et al., 2011): for DMN, ventromedial prefrontal cortex (vmPFC), −2, 38, −12; posterior cingulate cortex (PCC), −6, −44, 34; for CEN, bilateral dorsolateral prefrontal cortex (dlPFC) −/+46, 20, 44 and bilateral posterior parietal cortex (PPC), −40, −56, 44, and 52, −52, 50; for SN, bilateral fronto-insular cortex (FIC) −34, 20, −8, and 39, 23, −4, and anterior cingulate cortex (ACC) 6, 24, 32. Note that these specific regions were chosen because previous literature suggested that they are critical to the dysfunctions in ASD (Nomi and Uddin, 2015), but these networks could encompass other brain regions that were not selected in this study, e.g., ventral parietal regions of DMN.
The ROI-to-ROI network analysis was theory-driven to examine the relationship between memory networks and triple networks. At the individual level, the mean time series data of all ROI seeds (i.e., hippocampus, vTL, DMN, CEN, and SN seeds) were extracted, and Pearson’s correlation coefficients were calculated between all pairs of ROIs for each individual. We then examined the main effect of Group, Age, and the interaction between Age and Group on these coefficients across individuals with gender, FIQ, and FD entered as covariates. This analysis allowed us to reveal atypical functional connectivity between memory regions and brain regions that are important for ASD compared to Non-ASD based on previous literature (Uddin, 2015; Padmanabhan et al., 2017). Furthermore, we could also distinguish the different age effects between the groups.
In addition to the theory-driven approach to examine the connectivity between memory seeds and regions in the triple networks, we also explored age-related effects beyond the triple networks. To be more conservative, we used a constrained approach to examine the age effects on functional connectivity in ASD. We examined the functional connectivity of hippocampal and vTL seeds that differed between the ASD and non-ASD groups first and then tested whether these patterns of connectivity showed age-related differences. At the individual level, the mean time series data of each hippocampal and vTL seed were de-meaned and then regressed on all other voxels in the brain for each participant while the effects of movement parameters on 6 directions (x, y, z, pitch, yaw, and roll) were controlled for. The beta coefficients were then converted to t maps for each ROI. At the group level, we compared the t -maps of autistic individuals to those of Non-ASD, to reveal the atypical functional connectivity in ASD. Following the recent literature (Abrams et al., 2019; Chen et al., 2022; Hoeppli et al., 2022; Tarchi et al., 2022), we first used a more relaxed threshold to examine the effects but also reported results at a more stringent threshold. At the relaxed level, the significant clusters were identified at a height threshold of p < 0.01 with family wise error (FWE) corrections for multiple comparisons at the cluster level of p < 0.01 (128 voxels based on Monte Carlo simulations). A more stringent height thresholding was carried out with p < 0.001 with a corrected cluster level of p < 0.01 (41 voxels). We then examined the main effect of Age and Age*Group interaction on all the revealed connectivity while controlling for the effects of gender, FIQ, and FD.
For hippocampal and vTL seeds, ASD overall showed reduced functional connectivity within the memory networks, between bilateral vTL and bilateral hippocampus seeds, as well as between left vTL and ACC, and right vTL and bilateral FIC (all ps < .05; see Table 2; Figure 1A) when the effects of gender, FIQ, and FD were controlled for. For age-related differences, we observed a general pattern of reduced connectivity over age in both ASD and Non-ASD groups (see Table 2; Figure 1B), and these connectivity patterns were found between hippocampal and vTL seeds, as well as the hippocampal and vTL seeds with DMN, CEN, and SN nodes. Interestingly, the functional connectivity showing the age-related effect and those showing the group-related effect had little overlap. However, we did not observe a reliable Age-by-Group interaction. At the uncorrected level, only the connectivity between the right vTL and the right hippocampus showed a significant interaction between Age and Group, β = −0.336, t = 2.235*, p = 0.027, but it did not survive the FDR correction, adjusted p = 0.108. In addition, when the two oldest participants were excluded, there was a significant age effect, β = −0.248, t = −2.373, p = 0.019, but with an attenuated Age-by-Group interaction, β = −0.230, t = −1.518, p = 0.1312.
Table 2. Significant results of main effects for Age and Group as well as the interaction between Age and Group in the ROI-ROI analysis.
Figure 1. Significant effects of Group and Age on the functional connectivity between theory-driven ROIs chosen based on the literature. (A) Seed ROIs of five networks (hippocampal memory, ventral temporal memory, salience, central executive, and default-mode networks) and the group-related connectivity between them (all ASD < Non-ASD). The thickness of the lines represented the t values in the regression model. For more details, see Methods and Table 2. (B) The age-related connectivity between the same sets of theory-driven ROIs (reduced across ages). L/R dlPFC, left and right dorsolateral prefrontal cortex; PPC, posterior parietal cortex; ACC, anterior cingulate cortex; FIC, fronto-insular cortex; vmPFC, ventromedial prefrontal cortex; PCC, posterior cingulate cortex; hippo, hippocampus; vTL, ventral temporal lobe.
We further explored the age-related effects in functional connectivity of hippocampal and vTL memory seeds beyond the triple networks. We observed that overall, the ASD group showed reduced functional connectivity of both hippocampus and vTL, but a greater connectivity of the right hippocampus with the left dorsolateral prefrontal cortex compared to Non-ASD (see Table 3; Figure 2). We did not find any significant relationship between the Age and the functional connectivity of hippocampal or vTL seeds or a significant interaction between Age and Group on atypical connectivity of ASD compared to Non-ASD.
Table 3. Brain regions showing significantly different functional connectivity of hippocampal and vTL seeds in ASD compared to Non-ASD.
Figure 2. Seed to the whole brain analysis showed the atypical connectivity (ASD < Non-ASD in Red and ASD > Non-ASD in Blue) of bilateral hippocampal and vTL seeds. Hippo, hippocampus; pMTG, posterior middle temporal gyrus; vITG, ventral inferior temporal gyrus; FG, fusiform gyrus; dlPFC, dorsolateral prefrontal cortex; SMG, supramarginal gyrus; PostCG, postcentral gyrus; PreCG, precentral gyrus.
Our study used a well-matched sample of individuals with ASD and Non-ASD across a broad age spectrum and unveiled significant associations between resting-state functional connectivity within the hippocampal and ventral temporal lobe (vTL) memory networks. Specifically, both memory networks showed a notable reduction in connectivity with nodes in the triple networks over aging. Surprisingly, no reliable difference in age-related effects was observed in ASD compared to Non-ASD individuals. These observations urged further research to focus on the neurobiological basis of the memory and cognitive challenges experienced by autistic individuals.
Overall, both our ROI-to-ROI and seed-based analyses demonstrated reduced resting-state functional connectivity of the hippocampal and vTL networks in ASD compared to Non-ASD individuals. The ROI-to-ROI analysis mostly revealed the reduced connectivity within the hippocampal and vTL memory networks as well as the bilateral vTL with the SN nodes, namely ACC and bilateral FIC. Surprisingly, the seed-based analysis revealed little overlaps but identified a few more regions beyond the triple networks. These results aligned with findings from some previous studies (Lynch et al., 2013; von dem Hagen et al., 2013; Verly et al., 2014; Eilam-Stock et al., 2016; Arnold Anteraper et al., 2019), but not others (Mennes et al., 2011; Liu et al., 2023). Hence, our results further underscored that dysfunctions in ASD relate to a complex pattern of both greater and reduced intrinsic signals between different brain regions (Yizhar et al., 2011; Uddin et al., 2013; Kleinhans et al., 2016; Foss-Feig et al., 2017; Hull et al., 2017). Several prior investigations have specifically explored the resting-state functional connectivity of the hippocampus (Nomi and Uddin, 2015; Traynor et al., 2018; Liu et al., 2023) and found greater hippocampal connectivity in ASD compared to their control groups. One plausible explanation could be that the autistic participants in these studies were predominantly in the younger age range (<20 years old), consistent with our findings indicating a reduction in the functional connectivity of the hippocampal memory network with age in autistic individuals. Given the limited understanding of vTL-based functional connectivity in ASD, our study suggests that challenges in semantic processing (Ehlen et al., 2020; Foldager et al., 2023) may be associated with reduced connections of the vTL with other brain regions such as the dorsolateral prefrontal cortex, fronto-insular cortex, and hippocampus, which play crucial roles in information encoding and retention (Rosenbaum et al., 2001; O’Reilly and Norman, 2002; Postle, 2009; Dickerson and Eichenbaum, 2010), as well as with motor-sensory regions such as the fusiform gyrus and pre/postcentral gyri, involved in processing and representing multi-modal information (Rogers et al., 2005; Pulvermuller et al., 2009; Binkofski and Buxbaum, 2013; Chen and Rogers, 2015; Lambon Ralph et al., 2016; Bi, 2021).
Our study also revealed reduced functional connectivity of bilateral hippocampal and left vTL seeds over aging in ASD, compared to Non-ASD individuals. This finding may help reconcile some inconsistencies in the literature regarding both greater and reduced functional connectivity in ASD (Abrams et al., 2013; Verly et al., 2014; Hull et al., 2017; Lynn et al., 2018; Traynor et al., 2018; Liu et al., 2023), suggesting that participants’ age may be a contributing factor. Additionally, our findings may imply a nonlinear relationship between age and functional connectivity of memory networks in ASD, as indicated by structural and functional differences reported in previous research (Reinhardt et al., 2020; Pagni et al., 2022). However, one limitation is that the sample size of older adult participants was relatively small (15 ASD and 15 non-ASD), possibly affecting the accuracy of our estimations regarding age-related effects. Therefore, future studies should aim to recruit larger cohorts of elderly adults to further explore potential differences between ASD and Non-ASD groups.
Notably, the age-related effects were observed in the ROI-to-ROI approach, but not in the seed-based whole-brain analysis. One possible explanation could be that the age-related differences in functional connectivity are more likely to be observed between the memory networks, especially the hippocampal regions, and brain regions in the triple networks. Another reason is pertinent to the method we used in the seed-based whole-brain analysis. To be more conservative with our approach, we only examined the age-related effects in the brain regions that showed significant differences between the ASD and Non-ASD groups. However, from the ROI-to-ROI results, we observed that the age-related and group-related effects hardly overlapped. If the age-related effects on functional connectivity are relatively independent of differences between ASD and Non-ASD groups in brain regions beyond the triple networks as well, our seed-based whole brain analysis was less likely to reveal significant age-related effects. Therefore, future studies should examine the age-related effects independently.
One of the most important goals of the current study is to examine the connectivity difference in age-related effects between ASD and Non-ASD groups. To our surprise, we did not reveal any reliable Age-by-Group interaction. We only observed a potential trend of less pronounced reduction in functional connectivity between the right hippocampus and vTL in ASD. However, this finding was not significant after multiple comparison corrections and the exclusion of possible outliers. The right hippocampus has been shown to exhibit atypical developmental trajectories in ASD (Schumann, 2004; Reinhardt et al., 2020), with its abnormalities linked to social and cognitive challenges in ASD (Hogeveen et al., 2020; Liu et al., 2023). Meanwhile, the right ventral temporal lobe has been associated with challenges in face recognition (Schultz et al., 2000; Scherf et al., 2015), symptom severities (Kim et al., 2021), and language processing (Graves et al., 2022) in ASD. Therefore, given the significance of these two regions in ASD, although our study did not reveal reliable effects for these two regions, it is then critical for the future study to examine the atypical connectivity between the hippocampal and vTL memory networks, and their roles in in memory, language, and social challenges in autistic individuals.
Our study has clear limitations. First, we did not examine the relationship between atypical functional connectivity and memory abilities in ASD. Thus, it remains unclear how reduced connectivity in ASD may be associated with their social and cognitive abilities over aging. Furthermore, our study was based on a publicly available dataset and we tried to carefully match the ASD and Non-ASD groups, and therefore, the number of participants we included was still small, and the individuals in the older age range were limited (only 15 in each group with an age larger than 30). Consequently, the effect of the Age-by-Group interaction was relatively weak, which was only observed in a theory-driven and planned ROI-to-ROI analysis. Also, it did not survive with a correction for multiple corrections, or when the older participants were excluded from the analysis. Future studies should utilize a much larger sample with more participants in the older age range. This also highlighted the inadequacy of the current research to examine aging in ASD.
In conclusion, our study provides evidence of reduced functional connectivity in the hippocampal and vTL memory networks in ASD across the lifespan, emphasizing the importance of investigating memory network dysfunctions in ASD to better understand cognitive challenges in memory, language, and social skills. Furthermore, our results offer insights for educational and clinical practices to address both episodic and semantic memories when supporting autistic individuals in achieving independence in various aspects of life. Future research should delve deeper into how the atypical connectivity of memory networks may be linked to various types of memory challenges across different age groups in ASD.
Publicly available datasets were analyzed in this study. This data can be found at: ABIDE I (https://fcon_1000.projects.nitrc.org/indi/abide/).
Ethical approval was not required for the study involving humans in accordance with the local legislation and institutional requirements. Written informed consent to participate in this study was not required from the participants or the participants’ legal guardians/next of kin in accordance with the national legislation and the institutional requirements.
LC: Conceptualization, Formal analysis, Investigation, Methodology, Project administration, Supervision, Visualization, Writing – original draft, Writing – review & editing. MA: Visualization, Writing – original draft, Writing – review & editing. MF: Visualization, Writing – original draft, Writing – review & editing. YG: Visualization, Writing – original draft, Writing – review & editing. ZT: Visualization, Writing – original draft, Writing – review & editing. XZ: Writing – original draft, Writing – review & editing.
The author(s) declare that financial support was received for the research, authorship, and/or publication of this article. This research was supported by the WAVE Visualization Award and Summer Stipend Award from Santa Clara University to LC.
The authors declare that the research was conducted in the absence of any commercial or financial relationships that could be construed as a potential conflict of interest.
The reviewer YZ declared a past co-authorship with the author LC to the handling editor.
All claims expressed in this article are solely those of the authors and do not necessarily represent those of their affiliated organizations, or those of the publisher, the editors and the reviewers. Any product that may be evaluated in this article, or claim that may be made by its manufacturer, is not guaranteed or endorsed by the publisher.
Abrams, D. A., Lynch, C. J., Cheng, K. M., Phillips, J., Supekar, K., Ryali, S., et al. (2013). Underconnectivity between voice-selective cortex and reward circuitry in children with autism. Proc. Natl. Acad. Sci. 110, 12060–12065. doi: 10.1073/pnas.1302982110
Abrams, D. A., Padmanabhan, A., Chen, T., Odriozola, P., Baker, A. E., Kochalka, J., et al. (2019). Impaired voice processing in reward and salience circuits predicts social communication in children with autism. ELife 8:e39906. doi: 10.7554/eLife.39906
Arnold Anteraper, S., Guell, X., D’Mello, A., Joshi, N., Whitfield-Gabrieli, S., and Joshi, G. (2019). Disrupted cerebrocerebellar intrinsic functional connectivity in young adults with high-functioning autism spectrum disorder: a data-driven, whole-brain, high-temporal resolution functional magnetic resonance imaging study. Brain Connectivity 9, 48–59. doi: 10.1089/brain.2018.0581
Ashburner, J., Barnes, G., Chen, C. C., Daunizeau, J., Flandin, G., Friston, K., et al., (2014). SPM12 manual. Wellcome Trust Centre for Neuroimaging: London, 2464.
Banker, S. M., Pagliaccio, D., Ramphal, B., Thomas, L., Dranovsky, A., and Margolis, A. E. (2021). Altered structure and functional connectivity of the hippocampus are associated with social and mathematical difficulties in nonverbal learning disability. Hippocampus 31, 79–88. doi: 10.1002/HIPO.23264
Bi, Y. (2021). Dual coding of knowledge in the human brain. Trends Cogn. Sci. 25, 883–895. doi: 10.1016/J.TICS.2021.07.006
Binkofski, F., and Buxbaum, L. J. (2013). Two action systems in the human brain. Brain and Language 127, 222–229.
Binney, R. J., Embleton, K. V., Jefferies, E., Parker, G. J. M., and Lambon Ralph, M. A. (2010). The ventral and inferolateral aspects of the anterior temporal lobe are crucial in semantic memory: evidence from a novel direct comparison of distortion-corrected fMRI, rTMS, and semantic dementia. Cereb. Cortex 20, 2728–2738. doi: 10.1093/cercor/bhq019
Bird, C. M., and Burgess, N. (2008). The hippocampus and memory: insights from spatial processing. Nat. Rev. Neurosci. 9, 182–194. doi: 10.1038/nrn2335
Boucher, J., and Anns, S. (2018). Memory, learning and language in autism spectrum disorder. Autism Dev. Lang. Impairments 3, 1–13. doi: 10.1177/2396941517742078
Boucher, J., Mayes, A., and Bigham, S. (2012). Memory in autistic spectrum disorder. Psychol. Bull. 138, 458–496. doi: 10.1037/a0026869
Bowler, D., Gaigg, S., and Lind, S. (2011). “Memory in autism: binding, self and brain,” in Researching the Autism Spectrum: Contemporary Perspectives, eds I. Roth and P. Rezaie (Cambridge: Cambridge University Press).
Braden, B. B., Smith, C. J., Thompson, A., Glaspy, T. K., Wood, E., Vatsa, D., et al. (2017). Executive function and functional and structural brain differences in middle-age adults with autism spectrum disorder. Autism Res. 10, 1945–1959. doi: 10.1002/aur.1842
Cai, R. Y., Uljarević, M., and Leekam, S. R. (2020). Predicting mental health and psychological wellbeing in mothers of children with autism spectrum disorder: roles of intolerance of uncertainty and coping. Autism Res. 13, 1797–1801. doi: 10.1002/aur.2341
Chen, L., and Rogers, T. T. (2014). Revisiting domain-general accounts of category specificity in mind and brain. Wiley Interdisciplinary Rev. Cogn. Sci. 5, 327–344. doi: 10.1002/wcs.1283
Chen, L., and Rogers, T. T. (2015). A model of emergent category-specific activation in the posterior fusiform gyrus of sighted and congenitally blind populations. J. Cogn. Neurosci. 27, 1981–1999. doi: 10.1162/jocn_a_00834
Chen, L., Chang, H., Rudoler, J., Arnardottir, E., Zhang, Y., de los Angeles, C., et al. (2022). Cognitive training enhances growth mindset in children through plasticity of cortico-striatal circuits. Npj Sci. Learn. 7:30. doi: 10.1038/s41539-022-00146-7
Chen, L., Lambon Ralph, M. A., and Rogers, T. T. (2017). A unified model of human semantic knowledge and its disorders. Nat. Hum. Behav. 1:39. doi: 10.1038/s41562-016-0039
Chen, L., Liu, J., Kang, J. B., Rosenberg-Lee, M., Abrams, D. A., and Menon, V. (2024). Atypical pattern separation memory and its association with restricted interests and repetitive behaviors in autistic children. Autism doi: 10.1177/13623613231223354 Online ahead of print.
Coderre, E. L., Chernenok, M., Gordon, B., and Ledoux, K. (2017). Linguistic and non-linguistic semantic processing in individuals with autism spectrum disorders: an ERP study. J. Autism Dev. Disord. 47, 795–812. doi: 10.1007/s10803-016-2985-0
Cooper, R. A., Richter, F. R., Bays, P. M., Plaisted-Grant, K. C., Baron-Cohen, S., and Simons, J. S. (2017). Reduced hippocampal functional connectivity during episodic memory retrieval in autism. Cereb. Cortex 27, 888–902. doi: 10.1093/cercor/bhw417
Dajani, D. R., and Uddin, L. Q. (2016). Local brain connectivity across development in autism spectrum disorder: a cross-sectional investigation. Autism Res. 9, 43–54. doi: 10.1002/aur.1494
Danker, J., Strnadová, I., and Cumming, T. M. (2016). School experiences of students with autism spectrum disorder within the context of student wellbeing: a review and analysis of the literature. Australasian J. Special Educ. 40, 59–78. doi: 10.1017/jse.2016.1
Desaunay, P., Briant, A. R., Bowler, D. M., Ring, M., Gérardin, P., Baleyte, J.-M., et al. (2020). Memory in autism spectrum disorder: a meta-analysis of experimental studies. Psychol. Bull. 146, 377–410.
Desaunay, P., Guillery, B., Moussaoui, E., Eustache, F., Bowler, D. M., and Guénolé, F. (2023). Brain correlates of declarative memory atypicalities in autism: a systematic review of functional neuroimaging findings. Mol. Autism 14:2. doi: 10.1186/s13229-022-00525-2
Di Martino, A., Ross, K., Uddin, L. Q., Sklar, A. B., Castellanos, F. X., and Milham, M. P. (2009). Functional brain correlates of social and non-social processes in autism spectrum disorders: an ALE meta-analysis. Biol. Psychiatry 65, 63–74. doi: 10.1016/j.biopsych.2008.09.022.Functional
Di Martino, A., Yan, C. G., Li, Q., Denio, E., Castellanos, F. X., and Alaerts, K. (2014). The autism brain imaging data exchange: towards a large-scale evaluation of the intrinsic brain architecture in autism. Mol. Psychiatry 19, 659–667. doi: 10.1038/mp.2013.78
Dickerson, B. C., and Eichenbaum, H. (2010). The episodic memory system: neurocircuitry and disorders. Neuropsychopharmacology 35, 86–104.
Ehlen, F., Roepke, S., Klostermann, F., Baskow, I., Geise, P., Belica, C., et al. (2020). Small semantic networks in individuals with autism spectrum disorder without intellectual impairment: a verbal fluency approach. J. Autism Dev. Disord. 50, 3967–3987.
Eilam-Stock, T., Wu, T., Spagna, A., Egan, L. J., and Fan, J. (2016). Neuroanatomical alterations in high-functioning adults with autism spectrum disorder. Front. Neurosci. 10:237. doi: 10.3389/fnins.2016.00237
Foldager, M., Vestergaard, M., Lassen, J., Petersen, L. S., Oranje, B., Aggernaes, B., et al. (2023). Atypical semantic fluency and recall in children and adolescents with autism spectrum disorders associated with autism symptoms and adaptive functioning. J. Autism Dev. Disord. 53, 4280–4292. doi: 10.1007/s10803-022-05677-x
Foss-Feig, J. H., Adkinson, B. D., Ji, J. L., Yang, G., Srihari, V. H., and McPartland, J. C. (2017). Searching for cross-diagnostic convergence: neural mechanisms governing excitation and inhibition balance in schizophrenia and autism spectrum disorders. Biol. Psychiatry 81, 848–861. doi: 10.1016/j.biopsych.2017.03.005
Gaigg, S. B., Bowler, D. M., Ecker, C., Calvo-Merino, B., and Murphy, D. G. (2015). Episodic recollection difficulties in ASD result from atypical relational encoding: behavioral and neural evidence. Autism Res. 8, 317–327. doi: 10.1002/aur.1448
Gladfelter, A., and Goffman, L. (2018). Semantic richness and word learning in children with autism spectrum disorder. Dev. Sci. 21:e12543. doi: 10.1111/desc.12543
Graves, W. W., Levinson, H., Coulanges, L., Cahalan, S., Cruz, D., Sancimino, C., et al. (2022). Neural differences in social and figurative language processing on the autism spectrum. Neuropsychologia 171:108240.
Green, S. A., Rudie, J. D., Colich, N. L., Wood, J. J., Shirinyan, D., Hernandez, L., et al. (2013). Overreactive brain responses to sensory stimuli in youth with autism spectrum disorders. J. Am. Acad. Child Adolesc. Psychiatry 52, 1158–1172. doi: 10.1016/j.jaac.2013.08.004
Griffin, J. W., Bauer, R., and Gavett, B. E. (2022). The episodic memory profile in autism spectrum disorder: a bayesian meta-analysis. Neuropsychol. Rev. 32, 316–351. doi: 10.1007/s11065-021-09493-5
Hodges, J. R., Patterson, K., Oxbury, S., and Funnell, E. (1992). Semantic dementia progressive fluent aphasia with temporal lobe atrophy. Brain 115, 1783–1806. doi: 10.1093/brain/115.6.1783
Hoeppli, M. E., Nahman-Averbuch, H., Hinkle, W. A., Leon, E., Peugh, J., Lopez-Sola, M., et al. (2022). Dissociation between individual differences in self-reported pain intensity and underlying fMRI brain activation. Nat. Commun. 13:3569. doi: 10.1038/s41467-022-31039-3
Hogeveen, J., Krug, M. K., Geddert, R. M., Ragland, J. D., and Solomon, M. (2020). Compensatory hippocampal recruitment supports preserved episodic memory in autism spectrum disorder. Biol. Psychiatry Cogn. Neurosci. Neuroimaging 5, 97–109. doi: 10.1016/j.bpsc.2019.08.009
Hogstrom, L. J., Westlye, L. T., Walhovd, K. B., and Fjell, A. M. (2013). The structure of the cerebral cortex across adult life: age-related patterns of surface area, thickness, and gyrification. Cereb. Cortex 23, 2521–2530.
Hull, J. V., Jacokes, Z. J., Torgerson, C. M., Irimia, A., Van Horn, J. D., Aylward, E., et al. (2017). Resting-state functional connectivity in autism spectrum disorders: a review. Front. Psychiatry 7:205. doi: 10.3389/fpsyt.2016.00205
Kahn, I., Andrews-Hanna, J. R., Vincent, J. L., Snyder, A. Z., and Buckner, R. L. (2008). Distinct cortical anatomy linked to subregions of the medial temporal lobe revealed by intrinsic functional connectivity. J. Neurophysiol. 100, 129–139. doi: 10.1152/jn.00077.2008
Kim, D., Lee, J. Y., Jeong, B. C., Ahn, J. H., Kim, J. I., Lee, E. S., et al. (2021). Overconnectivity of the right Heschl’s and inferior temporal gyrus correlates with symptom severity in preschoolers with autism spectrum disorder. Autism Res. 14, 2314–2329. doi: 10.1002/aur.2609
Kleinhans, N. M., Reiter, M. A., Neuhaus, E., Pauley, G., Martin, N., Dager, S., et al. (2016). Subregional differences in intrinsic amygdala hyperconnectivity and hypoconnectivity in autism spectrum disorder. Autism Res. 9, 760–772.
Lambon Ralph, M. A., Jefferies, E., Patterson, K., Rogers, T. T., Lambon Ralph, M. A., Jefferies, E., et al. (2016). The neural and computational bases of semantic cognition. Nat. Rev. Neurosci. 18, 42–55. doi: 10.1038/nrn.2016.150
Lind, S. E., and Bowler, D. M. (2010). Episodic memory and episodic future thinking in adults with autism. J. Abnormal Psychol. 119, 896–905. doi: 10.1037/a0020631
Liu, J., Chen, L., Chang, H., Rudoler, J., Al-Zughoul, A. B., Kang, J. B., et al. (2023). Replicable patterns of memory impairments in children with autism and their links to hyperconnected brain circuits. Biol. Psychiatry Cogn. Neurosci. Neuroimaging 8, 1113–1123. doi: 10.1016/j.bpsc.2023.05.002
Lynch, C. J., Uddin, L. Q., Supekar, K., Khouzam, A., Phillips, J., and Menon, V. (2013). Default mode network in childhood autism: posteromedial cortex heterogeneity and relationship with social deficits. Biol. Psychiatry 74, 212–219. doi: 10.1016/j.biopsych.2012.12.013
Lynn, A. C., Padmanabhan, A., Simmonds, D., Foran, W., Hallquist, M. N., Luna, B., et al. (2018). Functional connectivity differences in autism during face and car recognition: underconnectivity and atypical age-related changes. Dev. Sci. 21:e12508. doi: 10.1111/desc.12508
Mennes, M., Zuo, X. N., Kelly, C., Di Martino, A., Zang, Y. F., Biswal, B., et al. (2011). Linking inter-individual differences in neural activation and behavior to intrinsic brain dynamics. NeuroImage 54, 2950–2959. doi: 10.1016/j.neuroimage.2010.10.046
Menon, V. (2018). The triple network model, insight, and large-scale brain organization in autism. Biol. Psychiatry 84, 236–238. doi: 10.1016/j.biopsych.2018.06.012
Naito, M., Hotta, C., and Toichi, M. (2020). Development of episodic memory and foresight in high-functioning preschoolers with ASD. J. Autism Dev. Disord. 50, 529–539. doi: 10.1007/s10803-019-04274-9
Nilsson, L. G. (2003). Memory function in normal aging. Acta Neurol. Scand. Suppl. 107, 7–13. doi: 10.1034/j.1600-0404.107.s179.5.x
Nomi, J. S., and Uddin, L. Q. (2015). Face processing in autism spectrum disorders: From brain regions to brain networks. Neuropsychologia 71, 201–216. doi: 10.1016/j.neuropsychologia.2015.03.029
O’Reilly, R. C., and Norman, K. A. (2002). Hippocampal and neocortical contributions to memory: advances in the complementary learning systems framework. Trends Cogn. Sci. 6, 505–510. doi: 10.1016/S1364-6613(02)02005-3
Padmanabhan, A., Lynch, C. J., Schaer, M., and Menon, V. (2017). The default mode network in autism. Biol. Psychiatry Cogn. Neurosci. Neuroimaging 2, 476–486. doi: 10.1016/j.bpsc.2017.04.004
Pagni, B. A., Walsh, M. J. M., Ofori, E., Chen, K., Sullivan, G., Alvar, J., et al. (2022). Effects of age on the hippocampus and verbal memory in adults with autism spectrum disorder: longitudinal versus cross-sectional findings. Autism Res. 15, 1810–1823. doi: 10.1002/aur.2797
Patterson, K., Lambon Ralph, M. A., Jefferies, E., Woollams, A., Jones, R., Hodges, J., et al. (2006). Presemantic” cognition in semantic dementia: six deficits in search of an explanation. J. Cogn. Neurosci. 18, 169–183. doi: 10.1162/jocn.2006.18.2.169
Pereira, A. M., Campos, B. M., Coan, A. C., Pegoraro, L. F., de Rezende, T. J. R., Obeso, I., et al. (2018). Differences in cortical structure and functional MRI connectivity in high functioning autism. Front. Neurol. 9:539. doi: 10.3389/fneur.2018.00539
Postle, B. R. (2009). “The hippocampus, memory, and consciousness,” in The Neurology of Consciousness: Cognitive Neuroscience and Neuropathology, eds S. Laureys and G. Tononi (Cambridge: Academic Press), 326–338.
Pulvermuller, F., Kherif, F., Hauk, O., Mohr, B., and Nimmo-Smith, I. (2009). Distributed cell assemblies for general lexical and category-specific semantic processing as revealed by fMRI cluster analysis. Hum. Brain Mapp. 30, 3837–3850. doi: 10.1002/hbm.20811
Reinhardt, V. P., Iosif, A. M., Libero, L., Heath, B., Rogers, S. J., Ferrer, E., et al. (2020). Understanding hippocampal development in young children with autism spectrum disorder. J. Am. Acad. Child Adolesc. Psychiatry 59, 1069–1079. doi: 10.1016/j.jaac.2019.08.008
Richards, R., Greimel, E., Kliemann, D., Koerte, I. K., Schulte-Körne, G., Reuter, M., et al. (2020). Increased hippocampal shape asymmetry and volumetric ventricular asymmetry in autism spectrum disorder. NeuroImage Clin. 26:102207. doi: 10.1016/j.nicl.2020.102207
Rogers, T. T., Hocking, J., Mechelli, A., Patterson, K., and Price, C. (2005). Fusiform activation to animals is driven by the process, not the stimulus. J. Cogn. Neurosci. 17, 434–445. doi: 10.1162/0898929053279531
Rogers, T. T., Lambon Ralph, M. A., Garrard, P., Bozeat, S., McClelland, J. L., Hodges, J. R., et al. (2004). Structure and deterioration of semantic memory: a neuropsychological and computational investigation. Psychol. Rev. 111, 205–235. doi: 10.1037/0033-295x.111.1.205
Rosenbaum, R. S., Winocur, G., and Moscovitch, M. (2001). New views on old memories: re-evaluating the role of the hippocampal complex. Behav. Brain Res 127, 183–197.
Scherf, K. S., Elbich, D., Minshew, N., and Behrmann, M. (2015). Individual differences in symptom severity and behavior predict neural activation during face processing in adolescents with autism. NeuroImage Clin. 7, 53–67. doi: 10.1016/j.nicl.2014.11.003
Schultz, R. T., Gauthier, I., Klin, A., Fulbright, R. K., Anderson, A. W., Volkmar, F., et al. (2000). Abnormal ventral temporal cortical activity during face discrimination among individuals with autism and Asperger syndrome. Arch. General Psychiatry 57, 331–340. doi: 10.1001/archpsyc.57.4.331
Schumann, C. M. (2004). The amygdala is enlarged in children but not adolescents with autism; the hippocampus is enlarged at all ages. J. Neurosci. 24, 6392–6401. doi: 10.1523/jneurosci.1297-04.2004
Schumann, C. M., Hamstra, J., Goodlin-Jones, B. L., Lotspeich, L. J., Kwon, H., Buonocore, M. H., et al. (2004). The amygdala is enlarged in children but not adolescents with autism; the hippocampus is enlarged at all ages. J. Neurosci. 24, 6392–6401. doi: 10.1523/JNEUROSCI.1297-04.2004
Scoville, W. B., and Milner, B. (1957). Loss of recent memory after bilateral hippocampal lesions. J. Neuropsychiatry Clin. Neurosci. 12, 103–113. doi: 10.1176/jnp.12.1.103-a
Tarchi, L., Damiani, S., Fantoni, T., Pisano, T., Castellini, G., Politi, P., et al. (2022). Centrality and interhemispheric coordination are related to different clinical/behavioral factors in attention deficit/hyperactivity disorder: a resting-state fMRI study. Brain Imaging Behav. 16, 2526–2542. doi: 10.1007/s11682-022-00708-8
Traynor, J. M., Doyle-Thomas, K. A. R., Hanford, L. C., Foster, N. E., Tryfon, A., Hyde, K. L., et al. (2018). Indices of repetitive behaviour are correlated with patterns of intrinsic functional connectivity in youth with autism spectrum disorder. Brain Res. 1685, 79–90.
Uddin, L. Q. (2015). Idiosyncratic connectivity in autism: developmental and anatomical considerations. Trends Neurosci. 38, 261–263. doi: 10.1016/j.tins.2015.03.004
Uddin, L. Q., Supekar, K. S., Ryali, S., and Menon, V. (2011). Dynamic reconfiguration of structural and functional connectivity across core neurocognitive brain networks with development. J. Neurosci. 31, 18578–18589.
Uddin, L. Q., Supekar, K., and Menon, V. (2013). Reconceptualizing functional brain connectivity in autism from a developmental perspective. Front. Hum. Neurosci. 7:458. doi: 10.3389/fnhum.2013.00458
Van Rooij, D., Anagnostou, E., Arango, C., Auzias, G., Behrmann, M., and Busatto, G. F. (2018). Cortical and subcortical brain morphometry differences between patients with autism spectrum disorder and healthy individuals across the lifespan: results from the ENIGMA ASD working group. Am. J. Psychiatry 175, 359–369. doi: 10.1176/appi.ajp.2017.17010100
Verly, M., Verhoeven, J., Zink, I., Mantini, D., Peeters, R., Deprez, S., et al. (2014). Altered functional connectivity of the language network in ASD: role of classical language areas and cerebellum. NeuroImage Clin. 4, 374–382. doi: 10.1016/j.nicl.2014.01.008
Visser, M., and Lambon Ralph, M. A. (2011). Differential contributions of bilateral ventral anterior temporal lobe and left anterior superior temporal gyrus to semantic processes. J. Cogn. Neurosci. 23, 3121–3131. doi: 10.1162/jocn_a_00007
Visser, M., Jefferies, E., Embleton, K. V., and Ralph, M. A. L. (2012). Both the middle temporal gyrus and the ventral anterior temporal area are crucial for multimodal semantic processing: distortion-corrected fMRI evidence for a double gradient of information convergence in the temporal lobes. J. Cogn. Neurosci. 24, 1766–1778.
von dem Hagen, E. A. H., Stoyanova, R. S., Baron-Cohen, S., and Calder, A. J. (2013). Reduced functional connectivity within and between “social” resting state networks in autism spectrum conditions. Soc. Cogn. Affect. Neurosci. 8, 694–701. doi: 10.1093/scan/nss053
Voss, J. L., Bridge, D. J., Cohen, N. J., and Walker, J. A. (2017). A closer look at the hippocampus and memory. Trends Cogn. Sci. 21, 577–588. doi: 10.1016/j.tics.2017.05.008
Wallace, G. L., Dankner, N., Kenworthy, L., Giedd, J. N., and Martin, A. (2010). Age-related temporal and parietal cortical thinning in autism spectrum disorders. Brain 133, 3745–3754. doi: 10.1093/brain/awq279
Wiggins, J. L., Peltier, S. J., Ashinoff, S., Weng, S. J., Carrasco, M., Welsh, R. C., et al. (2011). Using a self-organizing map algorithm to detect age-related changes in functional connectivity during rest in autism spectrum disorders. Brain Res. 1380, 187–197. doi: 10.1016/j.brainres.2010.10.102
Xu, Q., Zuo, C., Liao, S., Long, Y., and Wang, Y. (2020). Abnormal development pattern of the amygdala and hippocampus from childhood to adulthood with autism. J. Clin. Neurosci. 78, 327–332. doi: 10.1016/j.jocn.2020.03.049
Yizhar, O., Fenno, L. E., Prigge, M., Schneider, F., Davidson, T. J., Ogshea, D. J., et al. (2011). Neocortical excitation/inhibition balance in information processing and social dysfunction. Nature 477, 171–178. doi: 10.1038/nature10360
Keywords: hippocampus, ventral temporal lobe, autism spectrum disorder, memory, functional connectivity
Citation: Chen L, Abate M, Fredericks M, Guo Y, Tao Z and Zhang X (2024) Age-related differences in the intrinsic connectivity of the hippocampus and ventral temporal lobe in autistic individuals. Front. Hum. Neurosci. 18:1394706. doi: 10.3389/fnhum.2024.1394706
Received: 02 March 2024; Accepted: 22 May 2024;
Published: 13 June 2024.
Edited by:
Ryan Stanley Falck, University of British Columbia, CanadaReviewed by:
B. Blair Braden, Arizona State University, United StatesCopyright © 2024 Chen, Abate, Fredericks, Guo, Tao and Zhang. This is an open-access article distributed under the terms of the Creative Commons Attribution License (CC BY). The use, distribution or reproduction in other forums is permitted, provided the original author(s) and the copyright owner(s) are credited and that the original publication in this journal is cited, in accordance with accepted academic practice. No use, distribution or reproduction is permitted which does not comply with these terms.
*Correspondence: Lang Chen, bGNoZW40QHNjdS5lZHU=
Disclaimer: All claims expressed in this article are solely those of the authors and do not necessarily represent those of their affiliated organizations, or those of the publisher, the editors and the reviewers. Any product that may be evaluated in this article or claim that may be made by its manufacturer is not guaranteed or endorsed by the publisher.
Research integrity at Frontiers
Learn more about the work of our research integrity team to safeguard the quality of each article we publish.