- 1Norman Fixel Institute for Neurological Diseases, Gainesville, FL, United States
- 2Department of Neurology, University of Florida, Gainesville, FL, United States
- 3Department of Neurosurgery, University of Florida, Gainesville, FL, United States
Deep brain stimulation (DBS) is an effective surgical therapy for carefully selected patients with medication refractory essential tremor (ET). The most popular anatomical targets for ET DBS are the ventral intermedius nucleus (VIM) of the thalamus, the caudal zona incerta (cZI) and the posterior subthalamic area (PSA). Despite extensive knowledge in DBS programming for tremor suppression, it is not uncommon to experience stimulation induced side effects related to DBS therapy. Dysarthria, dysphagia, ataxia, and gait impairment are common stimulation induced side effects from modulation of brain tissue that surround the target of interest. In this review, we explore current evidence about the etiology of stimulation induced side effects in ET DBS and provide several evidence-based strategies to troubleshoot, reprogram and retain tremor suppression.
Introduction
Essential tremor (ET) is among the most prevalent hyperkinetic movement disorders with a pooled prevalence estimate of approximately 1% across all ages. Its prevalence increases with advancing age affecting up to 20% of people over 95 years old (Louis and McCreary, 2021). ET is defined as a chronic, insidiously progressive, isolated tremor syndrome characterized by an action tremor of both upper extremities, lasting for a minimum of 3 years in the absence of any other neurological signs such as parkinsonism, ataxia, or dystonia, and may or may not be accompanied by tremor in the head, voice, or lower limbs (Bhatia et al., 2018).
Pharmacological therapy has long been the mainstay of treatment for ET (Deuschl et al., 2011). First-line medications can provide approximately 55–60% mean reduction in tremor amplitude when used as monotherapy (Deuschl et al., 2011). Combined pharmacotherapy can sometimes yield better clinical outcomes (Wagle Shukla, 2022). Up to 55% of patients, however, manifest medication-refractory tremor (Louis, 2005), and thus surgical intervention may be considered in cases with refractory and disabling symptoms (Wagle Shukla, 2022).
Since its FDA approval in 1997, deep brain stimulation (DBS) has been considered a safe and effective therapy for medication refractory ET when applied to carefully selected patients. The location of the implanted lead is a critical determinant in achieving tremor suppression while limiting the manifestation of stimulation-induced side effects. The ventral intermediate nucleus of the thalamus (VIM) has been referred to as a “relay station” in the tremor network, connecting the cerebellum and motor cortex (Schnitzler et al., 2009), and it is the primary target for ET DBS (Benabid et al., 1987, 1991; Koller et al., 1997). The posterior subthalamic area (PSA) has been consistently reported as a target which may also provide optimal tremor suppression (Blomstedt et al., 2009, 2010; Barbe et al., 2011; Fytagoridis et al., 2016), particularly for tremors that are difficult to control with conventional VIM DBS (Kim et al., 2021). The clinical effect of ET DBS has been attributed to the direct modulation of the dentato-rubro-thalamic tract (DRTT) inclusive of the pre-lemniscal radiation and the caudal zona incerta (cZI) (Holslag et al., 2018). In this review we will use the term “thalamic DBS” to include all three of these anatomical regions.
Multiple side effects occur secondary to unintended stimulation of neighboring fiber tracts, which may modulate local and distal regions and neural networks. The most commonly encountered side effects in clinical practice are dysarthria, stimulation-induced ataxia, gait abnormalities, and loss of tremor benefit (habituation). Dysphagia is less common but has been reported. In this review, we will focus on these potential complications and discuss the current available options to reduce both acute and chronic stimulation induced side effects. In this review we discuss programing strategies that have been reported and trialed in the literature. While there is no consensus, this serves as a repository for evidence-based programing in challenging real-world scenarios.
An important concept to keep in mind is that some of the symptoms that we see as stimulation-induced side effects are often part of ET itself. The 2018 consensus classification of tremor (Bhatia et al., 2018 #2) adds a category of “soft signs” or “ET plus” to account for the dysarthria (Biary and Koller, 1987; Barkmeier-Kraemer and Clark, 2017), ataxia (Bhatia et al., 2018), and gait impairment (Fasano et al., 2010) that can be seen in patients with ET as part of the disease. Dysphagia and dysarthria are also possible complications from botulinum toxin injections to treat vocal tremor (Newland et al., 2022). Therefore, careful preoperative clinical evaluation is important to establish each patient’s baseline symptoms and avoid later embarking on a long-winded odyssey to attempt troubleshooting symptoms that are a part of the underlying pathology.
Dysarthria
Dysarthria stands out as the most common stimulation-induced side effect of thalamic region DBS (Chiu et al., 2020; Lu et al., 2020), with a prevalence reported in the literature ranging from 9% up to 75% (Pahwa et al., 2006; Flora et al., 2010). Despite tremor improvement, thalamic DBS can lead to reduced vocalization and imprecise oral articulation (Mucke et al., 2018).
Speech production is mediated by a network that is centralized around the left laryngeal and orofacial regions of the primary motor cortex. These areas receive inputs from the surrounding premotor, somatosensory, and parietal cortices (Guenther and Vladusich, 2012; Fuertinger et al., 2015). Dysarthria may occur through the spread of current to the corticospinal/corticobulbar tracts and to the DRTT, reflecting either an aggravation of pre-existing cerebellar deficits and/or the involvement of the upper motor neuron (UMN) fibers of the internal capsule (Mucke et al., 2018). Those UMN fibers overlap with the networks associated with tremor benefit following stimulation (Petry-Schmelzer et al., 2021), rendering it challenging to increase stimulation parameters without negatively affecting speech.
Stimulation-induced dysarthria occurs more frequently in those undergoing bilateral DBS (Picillo et al., 2016; Kim et al., 2021). It is also more commonly associated with stimulation on the electrode contacts more dorsally located, usually above the intercommissural line (Barbe et al., 2014; Kim et al., 2021), and those electrodes located relatively lateral (Becker et al., 2017). Spread of current to the medial aspect of the VIM region and to the centromedian and parafascicular thalamic nuclei region may also account for some speech dysfunction following DBS (Crosson, 2013).
Strategies to address stimulation induced dysarthria include proactive pre-operative patient screening for dysarthria along with conscientious lead placement. Staging DBS procedure one lead at a time allows for revaluation of speech and helps in weighting risk vs. benefit of a second lead on speech function in a shared decision-making process. During surgery, microelectrode recordings for target mapping can be used to refine the lead trajectory by ensuring lead placement away from the leg somatotopic representation of the VIM, corresponding to the lateral part of the nucleus, which lies closer to the corticobulbar tract (Garonzik et al., 2002 #102). Macrostimulation from the electrode can further facilitate optimization of the target location by estimating the relative distance from the internal capsule through the stimulation threshold, assessment of clinical benefit, and determination of the presence of stimulation-induced side effects. Nonetheless, in about one-third of patients’ dysarthria will only appear with chronic stimulation (Bot et al., 2018). Therefore, it is important to note that even well-placed electrodes might elicit stimulation-induced adverse events with chronic stimulation. Equally important is to note that in the operating room setting the number of test parameters is limited and this may not translate to the outpatient setting.
When programming a patient with stimulation-induced dysarthria, the initial strategy is usually to decrease the stimulation amplitude (or current density). Although helpful in many cases, this may result in sub-optimal tremor control and should be balanced in a shared decision-making process with the patient (Kim et al., 2021), as most patients prefer the side effects over sub-optimal tremor control (Baizabal-Carvallo et al., 2014; Barbe et al., 2014). Another strategy is to decrease the amplitude one side at a time, starting with the lead that controls the least bothersome hemi-body.
The ability to provide the patient with different programming settings using a handheld patient interface facilitates adjustment of stimulation parameters to fit the context of the situation (e.g., eating vs. speaking). For example, if they were to engage in a public speaking event, they can choose a stimulation setting where they manifest suboptimal tremor control, however, minimal dysarthria is present. In a circumstance where they may be eating a meal, they can choose a stimulation setting where they have complete tremor suppression, but mild dysarthria.
Changing the stimulation site to more ventrally located contacts can reduce dysarthria, considering it is more common when stimulating through dorsal contacts. A bipolar contact configuration is also an option when trying to avoid spreading of current into adjacent structures and undesirable side effects (Kim et al., 2021). When using this strategy, the contact that provides the best tremor control during the monopolar review is chosen as the cathode and the adjacent contact (either dorsal or ventral) is set as the anode. The amplitude should be decreased (down to 1 mA is our practice) and then increase gradually until the side effect comes back to assess the stimulation threshold in this new configuration. Switching the polarity of the selected electrodes might improve effectiveness and provide better tremor control and fewer side effects (Marks, 2011). Another multi-contact technique is using a double monopolar configuration, which was previously described by Kim et al. (2021), in a study that simultaneously targeted the Vim and PSA regions. The double monopolar configuration is a flexible alternative to bipolar configurations through the utilization of current fractionation in some commercially available devices.
Newer generations of DBS hardware may offer options to avoid stimulation-induced dysarthria while maintaining clinical benefit. This can be attempted by applying directional current steering and current fractionation. Essentially these technologies modify the shape of the volume of tissues activated (VTA) around the activated DBS electrode. By shifting the electric field axially along the DBS lead, one can reduce unwanted current spread to adjacent fiber tracts and can decrease stimulation-induced side effects (Rebelo et al., 2018). Rebelo et al. (2018) previously reported on an experimental study significant gains in therapeutic window (91%) and reductions in therapeutic current strength (31%) with stimulation in the “best direction” compared to “omnidirectional stimulation,” without any loss of tremor suppression. Omnidirectional stimulation would be either a full ring or all three segments of a directional lead activated to simulate a complete ring mode. Blume et al. (2017) conducted a prospective, randomized, double-blind study of ten ET patients and observed that directional DBS provided a larger “therapeutic window,” mainly due to lower therapeutic thresholds but not a higher threshold for side effects; therapeutic window is typically defined as the range of stimulation parameters that provides improvement of tremor without causing stimulation-induced side effects. Directional DBS was equally effective as the standard omnidirectional DBS for tremor suppression, and this was not associated with higher energy consumption (Bruno et al., 2021). Though it may be tempting to assume directional DBS is superior to ring mode or omnidirectional DBS, there are few comparison studies and drawing firm conclusions can be tricky.
Interleaving stimulation (ILS) is another useful technique for troubleshooting stimulation-induced dysarthria. This programming method implements two spatially distinct stimulation configurations on the same DBS lead, and it applies the settings in a temporally alternating sequence (Wagle Shukla et al., 2017). Barbe et al. (2014) tested an individualized ILS setting, shifting current from the most effective contact to the immediately dorsal located contact, making this another option to reduce stimulation-induced dysarthria while maintaining tremor control if other strategies have not been successful. Though effective, ILS can also reduce IPG life span over time.
Lastly, turning off stimulation at night, or decreasing the amplitude on only one side (Patel et al., 2014; Contarino et al., 2017) may in some select cases reduce dysarthria that might arise as a result of chronic stimulation. A summary of these strategies is depicted in Figure 1. Given that these programing strategies have not been compared in head-to-head studies, there is no evidence to demonstrate that one strategy is more effective than another. Therefore, we recommend implementing them in order of the simplest strategy to the most complex. We present Figure 2 as a protocol in this order for the programmer to have a sequence to follow when cases become complex, and a systematic approach is warranted.
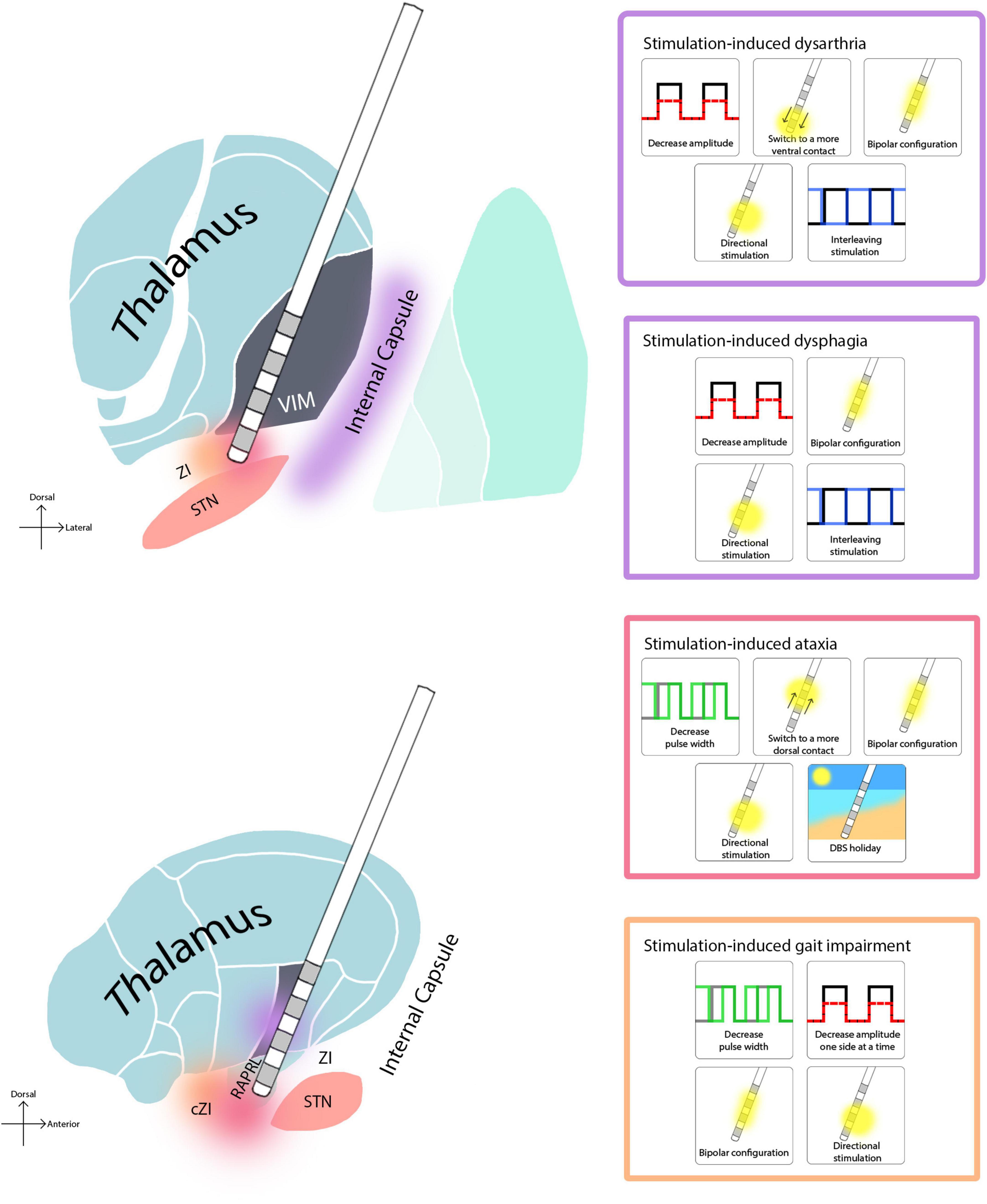
Figure 1. Regions associated with stimulation-induced side effects in thalamic DBS, and troubleshooting options. Since there is no side-to-side comparison of these strategies, we list them in ascending order of complexity.

Figure 2. Reprogramming strategies sorted in increasing order of complexity. Since there is no head-to-head comparison of how effective these interventions are, we recommend implementing them in order of simplicity.
Dysphagia
The prevalence of stimulation-induced dysphagia is not well documented in the literature. A study that used fiberoptic endoscopy demonstrated some degree of dysphagia in 12/12 thalamic DBS treated ET patients (Lapa et al., 2020). Dysphagia significantly improved in all patients after stimulation was turned off, with a reported mean improvement of 80% in the dysphagia score. The study was a small case series and given that dysphagia is not a common complaint post-thalamic DBS, we would advise caution in overinterpretation. As to the potential mechanism that leads to residual dysphagia, there are many possibilities including the implantation effect, spread of stimulation to corticobulbar fibers, suboptimal lead placement or a combination of a surgical effect plus disease progression. Authors have also postulated a “lingering neural network change” in dysphagia and stimulation-induced ataxia, however, this seems less likely. Finally, it is always important to investigate other potential underlying swallowing pathologies (Lapa et al., 2020).
Both cerebellar and corticobulbar fibers have been posited to play an important role in the process of swallowing. Corticobulbar fibers connect the motor cortex to the cranial nerve nuclei, which innervate the swallowing musculature (Lapa et al., 2020). There is a substantial neuroanatomical overlap of structures involved in the control and execution of speech and swallowing. It is this overlap that has led to speculation that both spread of current into the internal capsule or alternatively interference with the cerebellar network might impact swallowing physiology in a similar manner as in stimulation-induced dysarthria (Hamdy et al., 1996, 1997; Jayasekeran et al., 2011).
The options for troubleshooting stimulation-induced dysphagia are similar to those employed for stimulation-induced dysarthria. Brain imaging is critical to assess the anatomical relation of each contact with the surrounding structures, and “on and off DBS” testing during a barium swallow study can help to define the extent that stimulation is responsible for the acute issue. Reductions in pulse width and/or in amplitude may help swallowing, however, may also worsen tremor control. Bipolar stimulation settings, interleaved stimulation (Barbe et al., 2014), and/or current steering (Barbe et al., 2014; Bruno et al., 2021) may all be tried. Finally, brain imaging, on/off barium swallow testing and programming may in some cases not provide a path for conservative management. In these cases, a revision of the DBS lead should be considered and when retargeting the team should consider trajectory as well as lead location. For a summary of these strategies see Figure 1.
Ataxia
Stimulation-induced ataxia has been estimated to occur in 35% of patients with thalamic DBS (Chiu et al., 2020) and it has been shown to be acutely “inducible” in almost all patients in the operating room if enough current density is delivered to the thalamic target region (Groppa et al., 2014). Acute ataxia can similarly be reproduced in the clinic setting. Stimulation induced ataxia may impact the limbs, trunk, or features of gait.
Ataxia may also develop insidiously over many years following implantation. The average time has been reported to be approximately 5 years postoperatively, and in small series it is more common in older patients and in patients with a shorter disease duration at the time of DBS implantation (Chiu et al., 2020). The most common types of chronic ataxia presentations are appendicular ataxia (92%) and gait ataxia (44%) (Chiu et al., 2020).
Acute stimulation-induced ataxia most commonly arises following stimulation of the inferior aspect of the VIM and superior aspect of the zona incerta (Hidding et al., 2019). Proposed mechanisms for DBS-induced ataxia have been hypothesized to be related to antidromic stimulation of the cerebellar nodule via the uncinate tract from the subthalamic area (Reich et al., 2016), as well as secondary to plasticity changes in the cerebellum (Fasano and Helmich, 2019), possibly involving the stimulation of fibers to and from the red nucleus and inferior olive and/or fibers originating from interpositus nucleus, bundled within the dentatothalamic fibers (Elble, 2014; Groppa et al., 2014).
Acute stimulation-induced ataxia is now viewed as a circuit disorder by many experts and thus may be due to functional disruption of cerebello-thalamo-cortical networks (Garcia et al., 2003; Fasano et al., 2010; Groppa et al., 2014). Models which have calculated the VTA have shown that ventrocaudal stimulation in the subthalamic area corresponds with more significant gait ataxia and correlates with position emission tomography (PET) changes in which hypermetabolism in the cerebellar nodule increases as stimulation-induced gait ataxia worsens. These effects tend to normalize by approximately 72 h after stimulation is deactivated (Reich et al., 2016). This finding suggests that stimulation-induced ataxia may be reversible with programming or discontinuation of the electrical current.
Groppa et al. (2014) speculates that when we disrupt cerebello-thalamic input with therapeutic stimulation, a second pathway “compensates” for the information lost. Furthermore, he proposes that ataxia occurs when there is modulation of that “secondary pathway” and when the compensatory mechanism is inhibited (Groppa et al., 2014). The tract (if there is a specific tract) responsible for stimulation-induced ataxia has not been clearly identified or agreed upon. Some authors have proposed the ascending limb of the uncinate fasciculus present in the subthalamic area as the critical tract (Reich et al., 2016). This pathway connects efferent fibers from the deep cerebellar nuclei to the thalamus (Elsen et al., 2013; Fine et al., 2014). Finally, a few genetic subtypes of ataxia have been associated with axonal loss in the uncinate fasciculus providing further evidence to support this notion (Stezin et al., 2021).
Fasano and Helmich (2019) recently demonstrated the impact of acute thalamic DBS on gait ataxia in patients with ET, showing improvement with therapeutic stimulation and deterioration following supra-therapeutic stimulation (defined by increasing the amplitude and pulse width until decomposition of movement in the finger to nose test). This finding suggests that cerebellar dysfunction in these patients may be differentially modulated with optimal versus supra-therapeutic stimulation, possibly through recruitment of a different fiber system other than the DRTT based on chronaxie characteristics (Groppa et al., 2014). This observation has in general been translated into small studies using lower pulse widths of 30 μs (Choe et al., 2018) and 40 μs (Moldovan et al., 2018), which have demonstrated a reduction in acute stimulation-induced ataxia while retaining tremor benefit. An important caution is that chronaxie estimates using extracellular stimulation have been difficult to interpret versus regional neuroanatomy and thus must be interpreted with caution (Grill et al., 2005; Elble, 2014).
For troubleshooting, a common core principle is that strategies should include reprogramming trials that last at least a week or two to adequately evaluate delayed benefits and waning of benefits. The evaluation usually begins by repeating a monopolar review. A monopolar review is when the clinician programs for benefit and side effect at each contact and uses this information to guide potential strategies. Potential reprogramming strategies should in general aim to move the stimulation field, in a relative sense, away from cerebellar fibers. Moving active contact(s) dorsally is one such strategy. Another is using a bipolar configuration to narrow the VTA (Contarino et al., 2017). In select cases, current steering may lead to less ataxia compared to standard omni-directional stimulation (Bruno et al., 2021; Hidding et al., 2022; Roque et al., 2022). In newer generation hardware, a lower pulse width (less than 60 μs) (Choe et al., 2018; Moldovan et al., 2018) can also be attempted. Differences in axon diameters and chronaxies can be used by shortening pulse widths to achieve more selective activation of cerebellothalamic fibers, which may mediate tremor control, with less induction of ataxia (Kroneberg et al., 2019). If the monopolar review reveals low thresholds at active contacts and failure to maintain benefit, lead re-implantation may be considered.
In addition to reprogramming, another strategy is turning off the stimulation at night, as this may potentially impact the onset of stimulation induced ataxia (Rebelo et al., 2018). Finally, if ataxia or clumsiness emerges slowly and chronically, this effect is more likely disease progression and is less amenable to programming strategies. For a visual summary of these strategies see Figure 1, and for an example of their implementation see Figure 3.
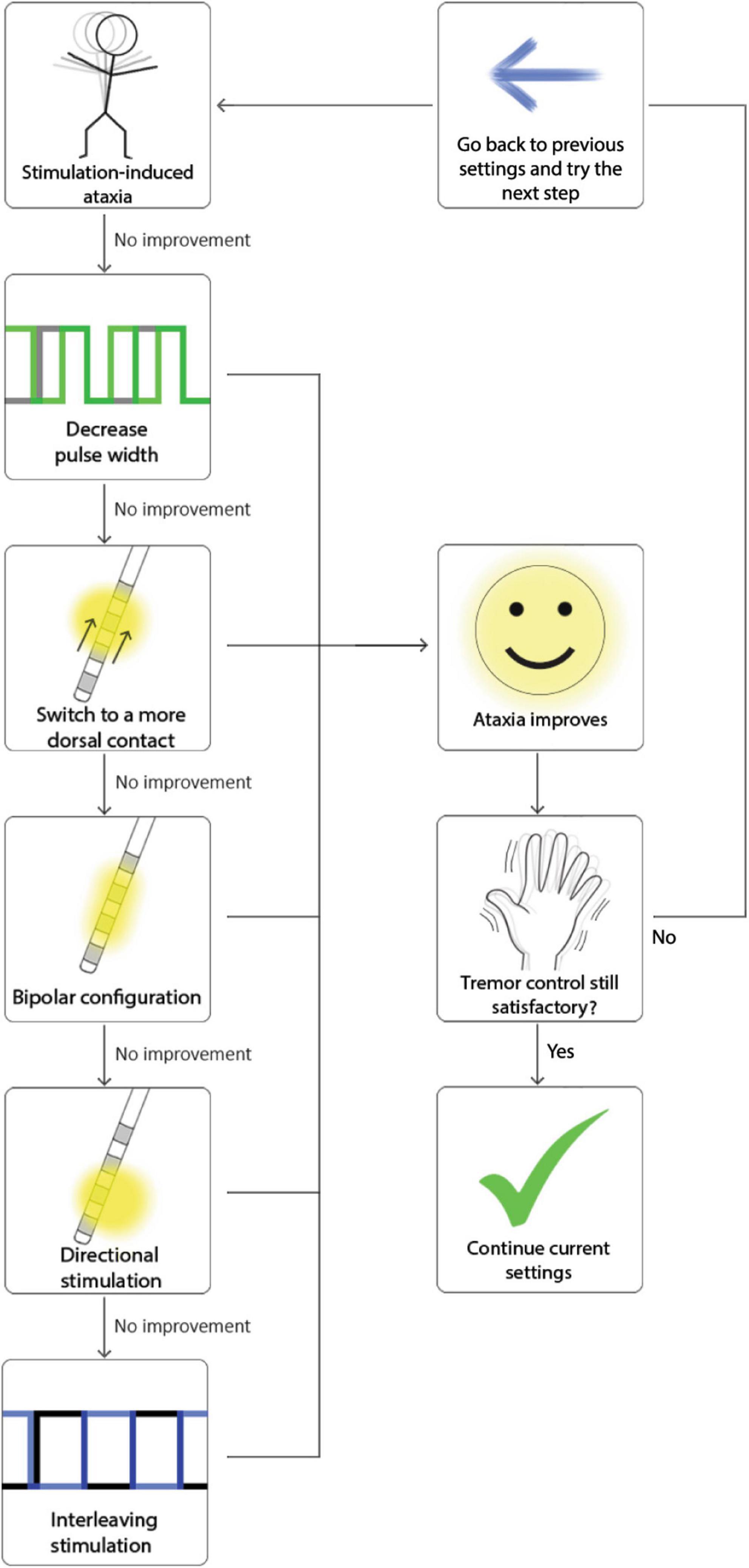
Figure 3. Example of how to troubleshoot ataxia implementing the strategies in Figure 1 and using the order proposed in Figure 2.
Gait and balance impairment
Impairments of balance and gait in patients with ET were reported by clinicians long before a formal association was explored or DBS was developed (Critchley, 1972; Singer et al., 1994). It has been shown in ET that there may be difficulties with tandem gait, balance confidence, and require significantly greater time to perform the Timed Up-and-Go relative to controls (Earhart et al., 2009). Worsening of pre-existing or new-onset gait and balance impairments following thalamic DBS affects between 5–50% of patients with ET (Benabid et al., 1998; Pahwa et al., 2006; Earhart et al., 2009; Kroneberg et al., 2019). However, others have presented contradictory evidence showing that DBS has no adverse effect on gait and balance in unilateral and bilateral stimulation (Earhart et al., 2009; Ramirez-Zamora et al., 2016). Age, disease severity and preoperative gait difficulties are considered risk factors for gait and balance impairment following DBS surgery (Newland et al., 2022). Despite adequate tremor control, patients may experience changes in gait either as an early acute or as a delayed side effect following DBS activation.
The acute phenomenon of gait and balance impairment with DBS is believed to be caused by stimulation-induced network dysfunction, specifically from antidromic cerebellar activation. However, the exact mechanism remains elusive (Earhart et al., 2009). More posterior and medial stimulation are believed to activate cerebellothalamic tracts, leading to gait disturbance, especially when stimulating below the ICL (Murata et al., 2003; Kim et al., 2021). The persistence of stimulation-induced gait impairment after turning off stimulation has led to the suggestion of a possible “microlesioned effect” as the cause (Roemmich et al., 2019). Temporal circuit plasticity is also a possible etiology if considering the 72-h delay observed in some cases for the gait to improve following discontinuation of DBS (Earhart et al., 2009).
Management of imbalance can be challenging, as reduction in stimulation parameters commonly leads to tremor recurrence or has no effect on balance (Ramirez-Zamora et al., 2016). Considering the probable etiological overlay between gait/balance impairments and the cerebellar ataxic features described in the “Stimulation-induced ataxia” section, the common core strategies to troubleshoot this side effect are the same as described above for ataxia.
Besides the previously suggested strategies, dual VIM + PSA stimulation have been reported as an efficacious strategy to mitigate gait disturbances by Kim et al. (2021), and reducing stimulation frequency from 170–185 Hz to 130 Hz after optimizing tremor control was also effective in improving balance difficulties while maintaining tremor control as demonstrated by Ramirez-Zamora et al. (2016).
Habituation versus disease progression
Habituation to stimulation, also referred to as “tolerance” (Benabid et al., 1996), is a hotly debated topic in the field of neuromodulation. Chronic high intensity stimulation has been hypothesized to induce detrimental plastic effects on tremor networks over time that may ultimately lead to decreased symptomatic control (Pilitsis et al., 2008). Alternatively, sparse post-mortem findings mildly support a biological adaptation to stimulation (Peters and Tisch, 2021). There is much debate about natural disease progression and habituation in the gradual loss of DBS efficacy over time. The characterization and quantification of the amount of overall worsening that is possibly due to loss of the stimulation effect, plastic effects or disease progression is challenging (Peters and Tisch, 2021).
In some studies, “habituation” has been found to occur in as many as 73% of patients with a mean follow-up of 56 months (Shih et al., 2013), and as early as 10 weeks post-implantation (Barbe et al., 2011). Another study showed that non-DBS treated ET controls had similar tremor worsening over time than those tracked with the DBS on and off. Favilla et al. (2012) made a strong argument that much of the chronic worsening in ET DBS may be related to disease progression. A retrospective analysis conducted by Tsuboi et al. (2020) assessed the long-term effects of VIM DBS in 97 patients with essential or dystonic tremor for as long as 13 years in some patients. In this study there were sustained benefits for both types of tremors.
Disease progression, lead misplacement, and suboptimal stimulation are the most commonly cited causes of gradual loss of efficacy in thalamic DBS (Blomstedt et al., 2007; Rodriguez Cruz et al., 2016; Fasano and Helmich, 2019), however, the placebo effect, loss of the microlesional effect (Benabid et al., 1987; Sydow et al., 2003), tissue impedance changes (Benabid et al., 1991; Boockvar et al., 2000; DiLorenzo et al., 2014), and stimulation-induced side effects may also be causes. Initial misdiagnosis has also been encountered in patients with tremor, including patients with multiple sclerosis, fragile X-associated tremor/ataxia syndrome (Artusi et al., 2018), and demyelinating neuropathy (Patel et al., 2014) rather that ET.
Whether the effect observed is called habituation or disease progression, overcoming it is challenging. Worsening typically manifests as a loss of initial DBS benefit in reducing tremor, and simply increasing the stimulation current may worsen tremor severity or induce stimulation-related side effects. Changing the active lead and stimulation parameters may lead to better tremor control, although these effects may not be sustained (Barbe et al., 2011). Studies have compared standard stimulation to weekly or daily rotating stimulation with mixed effects (Barbe et al., 2011; Seier et al., 2018; Petry-Schmelzer et al., 2019).
The initial evaluation should ideally include ruling out the presence of iatrogenic tremor caused by excessive stimulation (Fasano and Helmich, 2019). Fasano suggests reductions in pulse widths, followed by reduction in amplitude. Once stimulation-induced cerebellar tremor is ruled out, the next step should be increasing the frequency and then increasing the amplitude (Fasano and Helmich, 2019). Our experience is that once progression of ataxia and tremor have set in, there are limited management strategies to mitigate them.
Clinicians may attempt in challenging cases to widen the therapeutic window and allow for higher stimulation amplitudes. These strategies include using a bipolar lead configuration, switching the polarity of an existing bipolar setting, applying interleaved stimulation, utilizing directional leads, or shortening pulse widths. In some rare cases, adding an additional stimulation contact may also have a possible benefit, particularly if it is placed near the border of the thalamus (Picillo et al., 2016; Fasano and Helmich, 2019). Another suggested strategy is closed loop DBS (Kronenbuerger et al., 2006; Yamamoto et al., 2013).
There might be a potentially maladaptive response to long-term stimulation that may lead to some of the stimulation induced side-effects (Contarino et al., 2017). Therefore, having some time off the stimulation has been studied in the form of DBS holidays (Garcia Ruiz et al., 2001) or turning the stimulation off at night (Hariz et al., 1999). It should be noted that DBS holidays have been associated in some series with a prominent and debilitating rebound tremor despite symptomatic improvements. Paschen et al. (2019) recently observed that this rebound phenomenon tends to reach a plateau 30–60 min after DBS has been turned off, though the authors note that it is not always present. Furthermore, when utilized, the ideal duration of the DBS holiday is not known and, in our practice as in most expert practices, we do not recommend a DBS holiday.
The last option for a reduction in DBS benefit over time would be surgical revision. This can involve removal and repositioning of a lead or adding a new lead without removing the old one, often referred to as “rescue surgery” (Koller et al., 2001). Secondary leads have been added to many targets including the Vop, PSA or cZI (Yu et al., 2009; Oliveria et al., 2017). A key consideration for repeating surgery is the trajectory (more vertical may be useful for head tremor and helpful to avoid tracts leading to adverse events). Another consideration is whether the issue is ataxia or tremor. This therapy may be best when treating ataxia. Finally, distal tremor is easier to capture than proximal tremor, so a careful examination prior to making any surgical decisions should be pursued.
Conclusion
Stimulation-induced side effects are common in ET patients treated with thalamic DBS. Additionally most ET DBS patients experience some progression of disease or worsening of their tremor over time. As we learn more about the implicated brain networks in ET, we can potentially build several strategies to increase the therapeutic window for stimulation management without compromising tremor control. Understanding the pathophysiology of these ET DBS side effects will likely empower refined programming strategies and improved surgical planning.
Author contributions
AM-N: Visualization, Writing—original draft, Writing—review and editing. FS: Writing—original draft, Writing—review and editing. VC: Writing—review and editing. CH: Writing—review and editing. JH: Writing—review and editing. MO: Writing—review and editing. JW: Writing—review and editing.
Funding
The author(s) declare that no financial support was received for the research, authorship, and/or publication of this article.
Acknowledgments
We would like to acknowledge the Davis Rembert Fund for support of fellow research in essential tremor.
Conflict of interest
JW’s research was supported by NIH KL2TR001429. CH has received research grant funding from the University of Florida, Parkinson’s Foundation, NIH, the Michael J. Fox Foundation for Parkinson’s Research, and the Global Kinetics Corporation, and has received honoraria for speaking engagements, grant reviews, CME, and scientific reviews from The Parkinson’s Foundation and UptoDate Inc. MO serves as Medical Advisor the Parkinson’s Foundation, and has received research grants from NIH, Parkinson’s Foundation, the Michael J. Fox Foundation, the Parkinson Alliance, Smallwood Foundation, the Bachmann-Strauss Foundation, the Tourette Syndrome Association, and the UF Foundation. MO’s research was supported by: NIH R01 NR014852, R01NS096008, UH3NS119844, and U01NS119562. MO was PI of the NIH R25NS108939 Training Grant. MO has received royalties for publications with Demos, Manson, Amazon, Smashwords, Books4Patients, Perseus, Robert Rose, Oxford and Cambridge (movement disorders books). MO was an associate editor for New England Journal of Medicine Journal Watch Neurology and JAMA Neurology. MO has participated in CME and educational activities (past 12–24 months) on movement disorders sponsored by WebMD/Medscape, RMEI Medical Education, American Academy of Neurology, Movement Disorders Society, Mediflix and by Vanderbilt University. The institution and not MO receives grants from industry. MO has participated as a site PI and/or co-I for several NIH, foundation, and industry sponsored trials over the years but has not received honoraria. Research projects at the University of Florida receive device and drug donations.
The remaining authors declare that research was conducted in the absence of any commercial or financial relationships that could be construed as a potential conflict of interest.
The reviewer LR declared a past co-authorship with the author MO to the handling editor.
Publisher’s note
All claims expressed in this article are solely those of the authors and do not necessarily represent those of their affiliated organizations, or those of the publisher, the editors and the reviewers. Any product that may be evaluated in this article, or claim that may be made by its manufacturer, is not guaranteed or endorsed by the publisher.
References
Artusi, C. A., Farooqi, A., Romagnolo, A., Marsili, L., Balestrino, R., Sokol, L. L., et al. (2018). Deep brain stimulation in uncommon tremor disorders: indications, targets, and programming. J. Neurol. 265, 2473–2493. doi: 10.1007/s00415-018-8823-x
Baizabal-Carvallo, J. F., Kagnoff, M. N., Jimenez-Shahed, J., Fekete, R., and Jankovic, J. (2014). The safety and efficacy of thalamic deep brain stimulation in essential tremor: 10 years and beyond. J. Neurol. Neurosurg. Psychiatry 85, 567–572. doi: 10.1136/jnnp-2013-304943
Barbe, M. T., Dembek, T. A., Becker, J., Raethjen, J., Hartinger, M., Meister, I. G., et al. (2014). Individualized current-shaping reduces DBS-induced dysarthria in patients with essential tremor. Neurology 82, 614–619. doi: 10.1212/WNL.0000000000000127
Barbe, M. T., Liebhart, L., Runge, M., Deyng, J., Florin, E., Wojtecki, L., et al. (2011). Deep brain stimulation of the ventral intermediate nucleus in patients with essential tremor: stimulation below intercommissural line is more efficient but equally effective as stimulation above. Exp. Neurol. 230, 131–137. doi: 10.1016/j.expneurol.2011.04.005
Barkmeier-Kraemer, J. M., and Clark, H. M. (2017). Speech-language pathology evaluation and management of hyperkinetic disorders affecting speech and swallowing function. Tremor Other Hyperkinet. Mov. (N. Y.) 7:489. doi: 10.7916/D8Z32B30
Becker, J., Barbe, M. T., Hartinger, M., Dembek, T. A., Pochmann, J., Wirths, J., et al. (2017). The effect of uni- and bilateral thalamic deep brain stimulation on speech in patients with essential tremor: acoustics and intelligibility. Neuromodulation 20, 223–232. doi: 10.1111/ner.12546
Benabid, A. L., Benazzouz, A., Hoffmann, D., Limousin, P., Krack, P., and Pollak, P. (1998). Long-term electrical inhibition of deep brain targets in movement disorders. Mov. Disord. 13(Suppl. 3), 119–125.
Benabid, A. L., Pollak, P., Gao, D., Hoffmann, D., Limousin, P., Gay, E., et al. (1996). Chronic electrical stimulation of the ventralis intermedius nucleus of the thalamus as a treatment of movement disorders. J. Neurosurg. 84, 203–214.
Benabid, A. L., Pollak, P., Gervason, C., Hoffmann, D., Gao, D. M., Hommel, M., et al. (1991). Long-term suppression of tremor by chronic stimulation of the ventral intermediate thalamic nucleus. Lancet 337, 403–406.
Benabid, A. L., Pollak, P., Louveau, A., Henry, S., and De Rougemont, J. (1987). Combined (thalamotomy and stimulation) stereotactic surgery of the VIM thalamic nucleus for bilateral Parkinson disease. Appl. Neurophysiol. 50, 344–346. doi: 10.1159/000100803
Bhatia, K. P., Bain, P., Bajaj, N., Elble, R. J., Hallett, M., Louis, E. D., et al. (2018). Consensus Statement on the classification of tremors from the task force on tremor of the international parkinson and movement disorder society. Mov. Disord. 33, 75–87.
Blomstedt, P., Hariz, G. M., Hariz, M. I., and Koskinen, L. O. (2007). Thalamic deep brain stimulation in the treatment of essential tremor: a long-term follow-up. Br. J. Neurosurg. 21, 504–509.
Blomstedt, P., Sandvik, U., and Tisch, S. (2010). Deep brain stimulation in the posterior subthalamic area in the treatment of essential tremor. Mov. Disord. 25, 1350–1356.
Blomstedt, P., Sandvik, U., Fytagoridis, A., and Tisch, S. (2009). The posterior subthalamic area in the treatment of movement disorders: past, present, and future. Neurosurgery 64, 1029–1038; discussion 1038–1042. doi: 10.1227/01.NEU.0000345643.69486.BC
Blume, J., Schlaier, J., Rothenfusser, E., Anthofer, J., Zeman, F., Brawanski, A., et al. (2017). Intraoperative clinical testing overestimates the therapeutic window of the permanent DBS electrode in the subthalamic nucleus. Acta Neurochir. (Wien) 159, 1721–1726. doi: 10.1007/s00701-017-3255-4
Boockvar, J. A., Telfeian, A., Baltuch, G. H., Skolnick, B., Simuni, T., Stern, M., et al. (2000). Long-term deep brain stimulation in a patient with essential tremor: clinical response and postmortem correlation with stimulator termination sites in ventral thalamus. Case report. J. Neurosurg. 93, 140–144. doi: 10.3171/jns.2000.93.1.0140
Bot, M., Van Rootselaar, F., Contarino, M. F., Odekerken, V., Dijk, J., De Bie, R., et al. (2018). Deep brain stimulation for essential tremor: aligning thalamic and posterior subthalamic targets in 1 surgical trajectory. Oper. Neurosurg. (Hagerstown) 15, 144–152. doi: 10.1093/ons/opx232
Bruno, S., Nikolov, P., Hartmann, C. J., Trenado, C., Slotty, P. J., Vesper, J., et al. (2021). Directional deep brain stimulation of the thalamic ventral intermediate area for essential tremor increases therapeutic window. Neuromodulation 24, 343–352. doi: 10.1111/ner.13234
Chiu, S. Y., Nozile-Firth, K., Klassen, B. T., Adams, A., Lee, K., Van Gompel, J. J., et al. (2020). Ataxia and tolerance after thalamic deep brain stimulation for essential tremor. Parkinsonism Relat. Disord. 80, 47–53.
Choe, C. U., Hidding, U., Schaper, M., Gulberti, A., Koppen, J., Buhmann, C., et al. (2018). Thalamic short pulse stimulation diminishes adverse effects in essential tremor patients. Neurology 91, e704–e713. doi: 10.1212/WNL.0000000000006033
Contarino, M. F., Van Coller, R., Mosch, A., Van Der Gaag, N. A., and Hoffmann, C. F. (2017). Clinical approach to delayed-onset cerebellar impairment following deep brain stimulation for tremor. Brain 140:e27.
Critchley, E. (1972). Clinical manifestations of essential tremor. J. Neurol. Neurosurg. Psychiatry 35, 365–372.
Crosson, B. (2013). Thalamic mechanisms in language: a reconsideration based on recent findings and concepts. Brain Lang. 126, 73–88. doi: 10.1016/j.bandl.2012.06.011
Deuschl, G., Raethjen, J., Hellriegel, H., and Elble, R. (2011). Treatment of patients with essential tremor. Lancet Neurol. 10, 148–161.
DiLorenzo, D. J., Jankovic, J., Simpson, R. K., Takei, H., and Powell, S. Z. (2014). Neurohistopathological findings at the electrode-tissue interface in long-term deep brain stimulation: systematic literature review, case report, and assessment of stimulation threshold safety. Neuromodulation 17, 405–418; discussion 418. doi: 10.1111/ner.12192
Earhart, G. M., Clark, B. R., Tabbal, S. D., and Perlmutter, J. S. (2009). Gait and balance in essential tremor: variable effects of bilateral thalamic stimulation. Mov. Disord. 24, 386–391. doi: 10.1002/mds.22356
Elsen, G. E., Juric-Sekhar, G., Daza, R. A. M., and Hevner, R. F. (2013). “Development of cerebellar nuclei,” in Handbook of the cerebellum and cerebellar disorders, eds M. Manto, J. D. Schmahmann, F. Rossi, D. L. Gruol, and N. Koibuchi (Dordrecht: Springer), 179–205.
Fasano, A., and Helmich, R. C. (2019). Tremor habituation to deep brain stimulation: underlying mechanisms and solutions. Mov. Disord. 34, 1761–1773. doi: 10.1002/mds.27821
Fasano, A., Herzog, J., Raethjen, J., Rose, F. E., Muthuraman, M., Volkmann, J., et al. (2010). Gait ataxia in essential tremor is differentially modulated by thalamic stimulation. Brain 133, 3635–3648. doi: 10.1093/brain/awq267
Favilla, C. G., Ullman, D., Wagle Shukla, A., Foote, K. D., Jacobson, C. E. T., and Okun, M. S. (2012). Worsening essential tremor following deep brain stimulation: disease progression versus tolerance. Brain 135, 1455–1462. doi: 10.1093/brain/aws026
Fine, E. J., Salins, S., Shahdad, N., and Lohr, L. (2014). Neurognostic question. An English neurologist, neurophysiologist and neuro-anatomist who discovered a bundle in the brain stem. J. Hist. Neurosci. 23, 403–404. doi: 10.1080/0964704X.2014.934501
Flora, E. D., Perera, C. L., Cameron, A. L., and Maddern, G. J. (2010). Deep brain stimulation for essential tremor: a systematic review. Mov. Disord. 25, 1550–1559.
Fuertinger, S., Horwitz, B., and Simonyan, K. (2015). The functional connectome of speech control. PLoS Biol. 13:e1002209. doi: 10.1371/journal.pbio.1002209
Fytagoridis, A., Astrom, M., Samuelsson, J., and Blomstedt, P. (2016). Deep brain stimulation of the caudal zona incerta: tremor control in relation to the location of stimulation fields. Stereotact. Funct. Neurosurg. 94, 363–370. doi: 10.1159/000448926
Garcia Ruiz, P., Muniz De Igneson, J., Lopez Ferro, O., Martin, C., and Magarinos Ascone, C. (2001). Deep brain stimulation holidays in essential tremor. J. Neurol. 248, 725–726. doi: 10.1007/s004150170127
Garcia, L., Audin, J., D’alessandro, G., Bioulac, B., and Hammond, C. (2003). Dual effect of high-frequency stimulation on subthalamic neuron activity. J. Neurosci. 23, 8743–8751.
Garonzik, I. M., Hua, S. E., Ohara, S., and Lenz, F. A. (2002). Intraoperative microelectrode and semi-microelectrode recording during the physiological localization of the thalamic nucleus ventral intermediate. Mov. Disord. 17, S135–44. doi: 10.1002/mds.10155
Grill, W. M., Simmons, A. M., Cooper, S. E., Miocinovic, S., Montgomery, E. B., Baker, K. B., et al. (2005). Temporal excitation properties of paresthesias evoked by thalamic microstimulation. Clin. Neurophysiol. 116, 1227–1234. doi: 10.1016/j.clinph.2004.12.020
Groppa, S., Herzog, J., Falk, D., Riedel, C., Deuschl, G., and Volkmann, J. (2014). Physiological and anatomical decomposition of subthalamic neurostimulation effects in essential tremor. Brain 137, 109–121. doi: 10.1093/brain/awt304
Guenther, F. H., and Vladusich, T. (2012). A neural theory of speech acquisition and production. J. Neurolinguistics 25, 408–422.
Hamdy, S., Aziz, Q., Rothwell, J. C., Hobson, A., Barlow, J., and Thompson, D. G. (1997). Cranial nerve modulation of human cortical swallowing motor pathways. Am. J. Physiol. 272, G802–G808.
Hamdy, S., Aziz, Q., Rothwell, J. C., Singh, K. D., Barlow, J., Hughes, D. G., et al. (1996). The cortical topography of human swallowing musculature in health and disease. Nat. Med. 2, 1217–1224. doi: 10.1038/nm1196-1217
Hariz, M. I., Shamsgovara, P., Johansson, F., Hariz, G., and Fodstad, H. (1999). Tolerance and tremor rebound following long-term chronic thalamic stimulation for Parkinsonian and essential tremor. Stereotact. Funct. Neurosurg. 72, 208–218. doi: 10.1159/000029728
Hidding, U., Schaper, M., Gulberti, A., Buhmann, C., Gerloff, C., Moll, C. K. E., et al. (2022). Short pulse and directional thalamic deep brain stimulation have differential effects in parkinsonian and essential tremor. Sci. Rep. 12:7251. doi: 10.1038/s41598-022-11291-9
Hidding, U., Schaper, M., Moll, C. K. E., Gulberti, A., Koppen, J., Buhmann, C., et al. (2019). Mapping stimulation-induced beneficial and adverse effects in the subthalamic area of essential tremor patients. Parkinsonism Relat. Disord. 64, 150–155. doi: 10.1016/j.parkreldis.2019.03.028
Holslag, J. A. H., Neef, N., Beudel, M., Drost, G., Oterdoom, D. L. M., Kremer, N. I., et al. (2018). Deep brain stimulation for essential tremor: a comparison of targets. World Neurosurg. 110, e580–e584.
Jayasekeran, V., Rothwell, J., and Hamdy, S. (2011). Non-invasive magnetic stimulation of the human cerebellum facilitates cortico-bulbar projections in the swallowing motor system. Neurogastroenterol. Motil. 23, 831–e341. doi: 10.1111/j.1365-2982.2011.01747.x
Kim, M. J., Chang, K. W., Park, S. H., Chang, W. S., Jung, H. H., and Chang, J. W. (2021). Stimulation-induced side effects of deep brain stimulation in the ventralis intermedius and posterior subthalamic area for essential tremor. Front. Neurol. 12:678592. doi: 10.3389/fneur.2021.678592
Koller, W. C., Lyons, K. E., Wilkinson, S. B., Troster, A. I., and Pahwa, R. (2001). Long-term safety and efficacy of unilateral deep brain stimulation of the thalamus in essential tremor. Mov. Disord. 16, 464–468.
Koller, W., Pahwa, R., Busenbark, K., Hubble, J., Wilkinson, S., Lang, A., et al. (1997). High-frequency unilateral thalamic stimulation in the treatment of essential and parkinsonian tremor. Ann. Neurol. 42, 292–299. doi: 10.1002/ana.410420304
Kroneberg, D., Ewert, S., Meyer, A. C., and Kuhn, A. A. (2019). Shorter pulse width reduces gait disturbances following deep brain stimulation for essential tremor. J. Neurol. Neurosurg. Psychiatry 90, 1046–1050. doi: 10.1136/jnnp-2018-319427
Kronenbuerger, M., Fromm, C., Block, F., Coenen, V. A., Rohde, I., Rohde, V., et al. (2006). On-demand deep brain stimulation for essential tremor: a report on four cases. Mov. Disord. 21, 401–405. doi: 10.1002/mds.20714
Lapa, S., Claus, I., Reitz, S. C., Quick-Weller, J., Sauer, S., Colbow, S., et al. (2020). Effect of thalamic deep brain stimulation on swallowing in patients with essential tremor. Ann. Clin. Transl. Neurol. 7, 1174–1180.
Louis, E. D., and McCreary, M. (2021). How common is essential tremor? Update on the worldwide prevalence of essential tremor. Tremor Other Hyperkinet. Mov. (N. Y.) 11:28.
Lu, G., Luo, L., Liu, M., Zheng, Z., Zhang, B., Chen, X., et al. (2020). Outcomes and adverse effects of deep brain stimulation on the ventral intermediate nucleus in patients with essential tremor. Neural Plast. 2020:2486065.
Moldovan, A. S., Hartmann, C. J., Trenado, C., Meumertzheim, N., Slotty, P. J., Vesper, J., et al. (2018). Less is more - Pulse width dependent therapeutic window in deep brain stimulation for essential tremor. Brain Stimul. 11, 1132–1139. doi: 10.1016/j.brs.2018.04.019
Mucke, D., Hermes, A., Roettger, T. B., Becker, J., Niemann, H., Dembek, T. A., et al. (2018). The effects of thalamic deep brain stimulation on speech dynamics in patients with essential tremor: an articulographic study. PLoS One 13:e0191359. doi: 10.1371/journal.pone.0191359
Murata, J., Kitagawa, M., Uesugi, H., Saito, H., Iwasaki, Y., Kikuchi, S., et al. (2003). Electrical stimulation of the posterior subthalamic area for the treatment of intractable proximal tremor. J. Neurosurg. 99, 708–715. doi: 10.3171/jns.2003.99.4.0708
Newland, D. P., Novakovic, D., and Richards, A. L. (2022). Voice tremor and botulinum neurotoxin therapy: a contemporary review. Toxins (Basel) 14:773.
Oliveria, S. F., Rodriguez, R. L., Bowers, D., Kantor, D., Hilliard, J. D., Monari, E. H., et al. (2017). Safety and efficacy of dual-lead thalamic deep brain stimulation for patients with treatment-refractory multiple sclerosis tremor: a single-centre, randomised, single-blind, pilot trial. Lancet Neurol. 16, 691–700. doi: 10.1016/S1474-4422(17)30166-7
Pahwa, R., Lyons, K. E., Wilkinson, S. B., Simpson, R. K. Jr., Ondo, W. G., Tarsy, D., et al. (2006). Long-term evaluation of deep brain stimulation of the thalamus. J. Neurosurg. 104, 506–512.
Paschen, S., Forstenpointner, J., Becktepe, J., Heinzel, S., Hellriegel, H., Witt, K., et al. (2019). Long-term efficacy of deep brain stimulation for essential tremor: an observer-blinded study. Neurology 92, e1378–e1386. doi: 10.1212/WNL.0000000000007134
Patel, N., Ondo, W., and Jimenez-Shahed, J. (2014). Habituation and rebound to thalamic deep brain stimulation in long-term management of tremor associated with demyelinating neuropathy. Int. J. Neurosci. 124, 919–925. doi: 10.3109/00207454.2014.895345
Peters, J., and Tisch, S. (2021). Habituation after deep brain stimulation in tremor syndromes: prevalence, risk factors and long-term outcomes. Front. Neurol. 12:696950. doi: 10.3389/fneur.2021.696950
Petry-Schmelzer, J. N., Jergas, H., Thies, T., Steffen, J. K., Reker, P., Dafsari, H. S., et al. (2021). Network fingerprint of stimulation-induced speech impairment in essential tremor. Ann. Neurol. 89, 315–326. doi: 10.1002/ana.25958
Petry-Schmelzer, J. N., Reker, P., Pochmann, J., Visser-Vandewalle, V., Dembek, T. A., and Barbe, M. T. (2019). Daily alternation of DBS settings does not prevent habituation of tremor suppression in essential tremor patients. Mov. Disord. Clin. Pract. 6, 417–418.
Picillo, M., Lozano, A. M., Kou, N., Munhoz, R. P., and Fasano, A. (2016). Programming deep brain stimulation for tremor and dystonia: the toronto western hospital algorithms. Brain Stimul. 9, 438–452.
Pilitsis, J. G., Metman, L. V., Toleikis, J. R., Hughes, L. E., Sani, S. B., and Bakay, R. A. (2008). Factors involved in long-term efficacy of deep brain stimulation of the thalamus for essential tremor. J. Neurosurg. 109, 640–646. doi: 10.3171/JNS/2008/109/10/0640
Ramirez-Zamora, A., Boggs, H., and Pilitsis, J. G. (2016). Reduction in DBS frequency improves balance difficulties after thalamic DBS for essential tremor. J. Neurol. Sci. 367, 122–127. doi: 10.1016/j.jns.2016.06.001
Rebelo, P., Green, A. L., Aziz, T. Z., Kent, A., Schafer, D., Venkatesan, L., et al. (2018). Thalamic directional deep brain stimulation for tremor: spend less, get more. Brain Stimul. 11, 600–606. doi: 10.1016/j.brs.2017.12.015
Reich, M. M., Brumberg, J., Pozzi, N. G., Marotta, G., Roothans, J., Astrom, M., et al. (2016). Progressive gait ataxia following deep brain stimulation for essential tremor: adverse effect or lack of efficacy? Brain 139, 2948–2956.
Rodriguez Cruz, P. M., Vargas, A., Fernandez-Carballal, C., Garbizu, J., De La Casa-Fages, B., and Grandas, F. (2016). Long-term thalamic deep brain stimulation for essential tremor: clinical outcome and stimulation parameters. Mov. Disord. Clin. Pract. 3, 567–572.
Roemmich, R., Roper, J. A., Eisinger, R. S., Cagle, J. N., Maine, L., Deeb, W., et al. (2019). Gait worsening and the microlesion effect following deep brain stimulation for essential tremor. J. Neurol. Neurosurg. Psychiatry 90, 913–919. doi: 10.1136/jnnp-2018-319723
Roque, D. A., Hadar, E., Zhang, Y., Zou, F., and Murrow, R. (2022). Reducing ataxic side effects from ventral intermediate nucleus of the thalamus deep brain stimulation implantation in essential tremor: potential advantages of directional stimulation. Stereotact. Funct. Neurosurg. 100, 35–43. doi: 10.1159/000518238
Schnitzler, A., Munks, C., Butz, M., Timmermann, L., and Gross, J. (2009). Synchronized brain network associated with essential tremor as revealed by magnetoencephalography. Mov. Disord. 24, 1629–1635. doi: 10.1002/mds.22633
Seier, M., Hiller, A., Quinn, J., Murchison, C., Brodsky, M., and Anderson, S. (2018). Alternating thalamic deep brain stimulation for essential tremor: a trial to reduce habituation. Mov. Disord. Clin. Pract. 5, 620–626. doi: 10.1002/mdc3.12685
Shih, L. C., Lafaver, K., Lim, C., Papavassiliou, E., and Tarsy, D. (2013). Loss of benefit in VIM thalamic deep brain stimulation (DBS) for essential tremor (ET): how prevalent is it? Parkinsonism Relat. Disord. 19, 676–679. doi: 10.1016/j.parkreldis.2013.03.006
Singer, C., Sanchez-Ramos, J., and Weiner, W. J. (1994). Gait abnormality in essential tremor. Mov. Disord. 9, 193–196.
Stezin, A., Bhardwaj, S., Khokhar, S., Hegde, S., Jain, S., Bharath, R. D., et al. (2021). In vivo microstructural white matter changes in early spinocerebellar ataxia 2. Acta Neurol. Scand. 143, 326–332. doi: 10.1111/ane.13359
Sydow, O., Thobois, S., Alesch, F., and Speelman, J. D. (2003). Multicentre European study of thalamic stimulation in essential tremor: a six year follow up. J. Neurol. Neurosurg. Psychiatry 74, 1387–1391. doi: 10.1136/jnnp.74.10.1387
Tsuboi, T., Jabarkheel, Z., Zeilman, P. R., Barabas, M. J., Foote, K. D., Okun, M. S., et al. (2020). Longitudinal follow-up with VIM thalamic deep brain stimulation for dystonic or essential tremor. Neurology 94, e1073– e1084.
Wagle Shukla, A. (2022). Diagnosis and treatment of essential tremor. Continuum (Minneap. Minn.) 28, 1333–1349.
Wagle Shukla, A., Zeilman, P., Fernandez, H., Bajwa, J. A., and Mehanna, R. (2017). DBS programming: an evolving approach for patients with Parkinson’s disease. Parkinsons Dis. 2017:8492619.
Yamamoto, T., Katayama, Y., Ushiba, J., Yoshino, H., Obuchi, T., Kobayashi, K., et al. (2013). On-demand control system for deep brain stimulation for treatment of intention tremor. Neuromodulation 16, 230–235; discussion 235.
Keywords: deep brain stimulation, ataxia, dysarthria, dysphagia, gait impairment
Citation: Martinez-Nunez AE, Sarmento FP, Chandra V, Hess CW, Hilliard JD, Okun MS and Wong JK (2024) Management of essential tremor deep brain stimulation-induced side effects. Front. Hum. Neurosci. 18:1353150. doi: 10.3389/fnhum.2024.1353150
Received: 10 December 2023; Accepted: 01 February 2024;
Published: 22 February 2024.
Edited by:
Takashi Morishita, Fukuoka University, JapanReviewed by:
Luigi M. Romito, IRCCS Carlo Besta Neurological Institute Foundation, ItalyZuzana Kosutzka, Comenius University, Slovakia
Copyright © 2024 Martinez-Nunez, Sarmento, Chandra, Hess, Hilliard, Okun and Wong. This is an open-access article distributed under the terms of the Creative Commons Attribution License (CC BY). The use, distribution or reproduction in other forums is permitted, provided the original author(s) and the copyright owner(s) are credited and that the original publication in this journal is cited, in accordance with accepted academic practice. No use, distribution or reproduction is permitted which does not comply with these terms.
*Correspondence: Alfonso Enrique Martinez-Nunez, martineznuneza@ufl.edu