- 1Department of Radiological Technology, Niigata University of Health and Welfare, Niigata, Japan
- 2Department of Physical Therapy, Niigata University of Health and Welfare, Niigata, Japan
- 3Graduate School of Health and Welfare, Niigata University of Health and Welfare, Niigata, Japan
- 4Department of Healthcare Informatics, Takasaki University of Health and Welfare, Gunma, Japan
Background: Autonomous sensory meridian response (ASMR) is a sensory response such as tingling and pleasantness from audiovisual stimuli. ASMR videos come in a wide variety of types, and personal preferences are biased. There are many reports of the effects os ASMR on sleep onset, anxiety relief, and other relaxation effects. However, prior task-oriented studies have used ASMR videos provided by the experimenter. We hypothesized that ASMR movies of a personal preference would show significantly increased activity in the nucleus accumbens, frontal cortex, and insular cortex, which are brain areas associated with relaxation. Therefore, the purpose of this study was to elucidate the neuroscientific basis for the relaxation effects of ASMR videos that match someone’s personal preferences.
Methods: This study included 30 healthy individuals aged ≥18 years. ASMR enthusiasts were included as the target population due to the need to have a clear preference for ASMR videos. A control video (1 type) and ASMR videos (20 types) were used as the stimulus tasks. Among the ASMR videos, those with high and low evaluation scores were considered liked and dislikedASMR videos, respectively. Functional magnetic resonance imaging was performed while the participants viewed a block design with a resting task in between. The data were analyzed using Statistical Parametric Mapping 12 to identify the areas activated by control, disliked, and liked ASMR videos.
Results: Emotion-related areas (the amygdala, frontal cortex, and insular cortex) not activated by control and unliked ASMR videos were activated only by liked ASMR videos.
Conclusion: The amygdala, frontal cortex, and insular cortex may be involved in the limbic dopamine circuits of the amygdala and middle frontal gyrus and the autonomic balance of the left and right insular cortices. This suggests the potential of positive mood and its use as a treatment for patients with anxiety and depression. These results suggest that the use of ASMR videos to match individual preferences may induce relaxation and have beneficial effects on depression and other disorders, and also support the introduction of ASMR videos in mental health care.
1 Introduction
Autonomous sensory meridian response (ASMR) is a pleasant sensory response triggered by audiovisual stimuli. The reaction, called tingling, begins at the scalp, spreads to the neck and shoulders, and then to the back and limbs (Barratt and Davis, 2015). ASMR videos that cause tingling can be viewed on YouTube and other platforms. The triggers that cause the tingling are very personal. The videos include whispers, soft speech, tapping and scratching sounds, slow and expert hand movements, and meticulous attention (Barratt and Davis, 2015; Poerio et al., 2018). These videos have gained a large online community of young people in recent years.
The reported uses of ASMR include sleep induction, relaxation, and anxiety relief (Barratt and Davis, 2015; Barratt et al., 2017). Many studies have reported the effects of ASMR. In a physiological study, Poerio et al. (2018) reported contradictory results, with increased skin conductance and decreased heart rate. Engelbregt et al. (2022) reported that ASMR videos induced relaxation in individuals insensitive to ASMR, with evidence of a reduced heart rate. Valtakari et al. (2019) observed increased pupil diameter during tingling. Electroencephalography (EEG) studies have reported the sleep-inducing effects of ASMR and have proposed that this method can improve sleep quality (Lee et al., 2019; Vardhan et al., 2020). Fredborg et al. (2021) reported relaxation from increased α waves. Functional magnetic resonance imaging (fMRI) studies have also demonstrated brain function during tingling in areas involved in social behavior, reward systems, and empathy. The studies also suggested the contribution of oxytocin release to the relaxed state during tingling (Lochte et al., 2018). Lee et al. (2020) observed increased functional connectivity in the medial prefrontal cortex during the resting state and ASMR video viewing. This suggests the involvement of mentalization and self-referential processing in its induction and maintenance. We also compared the relaxation effects of ASMR and classical music by fMRI. In both cases, activation of the thalamus and other areas involved in sleep was observed, particularly that of the medial prefrontal cortex, which is involved in inducing relaxation in ASMR (Sakurai et al., 2021). In addition, we compared the effects of ASMR video and sound alone. Participants who watched the video showed activation of the dopamine pathway in the limbic system, suggesting that a positive mood can reduce stress and improve depression and that sound alone can balance the autonomic nervous system by activating the left and right insular cortices (Sakurai et al., 2023). Our study showed that relaxation was induced regardless of tingling.
ASMR does not occur in everyone. Roberts et al. (2020) stating that 28% consider themselves to be ASMR responders, while Swart et al. (2021) reported that 38% consider themselves to be ASMR responders. Because tingling is a subjective aspect of an individual, an ASMR video that induces tingling in one individual may not induce it in another (Barratt et al., 2017) ASMR videos have been shown to produce relaxation and positive mood even when tingling does not occur (Barratt and Davis, 2015). Barratt and Davis (2015) classified ASMR into four categories (whispering, personal attention, crisp sounds, and slow movements), whereas Fredborg et al. (2017) classified these sounds into five categories (watching, touching, repetitive, simulations, and mouth sounds). Thus, many categories and composites of these categories exist. Moreover, many other ASMR videos do not fit into these categories, making the content broad. This finding supports the hypothesis that individual preferences are highly biased.
In a study focusing on personal differences, Smith et al. (2019) investigated individual differences in sensitivity to five ASMR categories using resting-state fMRI. Functional connectivity was negatively correlated with ASMR sensitivity but positively correlated in the dorsal attention network, suggesting that ASMR is an attention-driven process. A more recent study developed a trigger checklist for ASMR responders consisting of 37 triggers, focusing on personal differences (Poerio et al., 2023).
Thus, it is clear that ASMR is highly influenced by personal differences, but studies tailored to personal differences are lacking because of the wide variety of types. In many previous studies of task-oriented ASMR research, the ASMR videos for the task were prepared by the experimenter and used by the subject (Lochte et al., 2018; Lee et al., 2019, 2020). This study is the first to use ASMR videos tailored to individual preferences and to prove their effectiveness based on brain function mechanisms. In many previous studies, the ASMR videos for the task were prepared by the experimenter and used by the participant. This study is the first to use ASMR videos tailored to individual preferences and to prove their effectiveness based on functional brain mechanisms.
Brain regions linked to the relaxation effects of ASMR, as inferred from our previous studies, suggest the involvement of the nucleus accumbens, prefrontal cortex, and insular cortex (Sakurai et al., 2021, 2023). As such, we hypothesized that the activity in these brain areas would be significantly increased in response to the preferred ASMR videos compared to that of ASMR videos of no particular interest. Therefore, the purpose of this study was to elucidate the neuroscientific basis for the relaxation effects of ASMR videos that match individual preferences because ASMR videos are personal and subjective.
2 Materials and methods
2.1 Participants
This study included 30 healthy participants (20 men and 10 women, mean age 20.1 ± 1.3 years) aged ≥18 years. The target population consisted of only ASMR enthusiasts because of the need for a clear preference for certain ASMR categories. However, the participants were required not to watch ASMR for 1 week before the experiment. This study was approved by the Research Ethics Committee of Niigata University of Health and Welfare (approval no. 18961-221208). Written informed consent was obtained from all participants. In addition, a medical interview was conducted to ensure the safety of the MRI.
2.2 Stimulus tasks
The stimulus tasks involved viewing a control video (1 type) and ASMR videos (20 types). For the control task, we prepared an “educational/demonstration video.” This video did not include gentle speech or slow, delicate hand movements, as employed in the ASMR videos (Poerio et al., 2018). ASMR videos were prepared to cover all five categories (Fredborg et al., 2017): watching, touching, repetitive sounds, simulations, and mouth sounds. There were five types of watching videos (turning pages, writing sounds, speaking words slowly, pouring soda, shaving soap), two kinds of touching videos (touching hand motions, ear-pulling), six kinds of repetitive sounds videos (holding slime, typing, rolling marbles, tapping nails, cutting vegetables, grilling meat), four types of simulation videos (shampoo, haircut, teeth brushing lecture, calm massage), and three kinds of mouth sounds videos (eating fried fish, eating salmon, eating noodles). Of these 20 ASMR videos, the videos that were liked and disliked were determined. The participants watched all 20 types of ASMR at least 1 week before the experiment and scored each for two mood types: relaxed mood and tingling mood (Sakurai et al., 2023). For each of the 20 ASMR videos, the degree to which they evoked more emotion than the control video was rated on a visual analog scale from 0 to 10. The two mood scores were recorded, and the videos with the highest and lowest total scores were defined as the favorite and least favorite ASMR videos, respectively. In the case of a tie, participants were asked to verbally indicate which video they preferred or did not like better. The resting task was presented with a fixed cross on a black screen while the subject listened to white noise. We used white noise as an emotionless and random signal with equal power at any frequency in a specified bandwidth (Cardona et al., 2020).
2.3 Block design
The block design consisted of alternating repetitions of 40 s for the rest period and 40 s for the stimulus task for a total of 4 min. The order in which the stimulus tasks were presented was random. White noise and fixed crosses interspersed between stimulation tasks returned the cerebral blood oxygen signal to baseline and separated the stimulation times. Video editing was performed using PowerDirector 18 (CyberLink, Taipei, Taiwan) to create compressed audio data in MP4 format with all volume settings set to 98 dB. The block design is illustrated in Figure 1.

Figure 1. Block design. The order of control, disliked ASMR, and liked ASMR videos in the task was random. ASMR, autonomous sensory meridian response.
2.4 Apparatus
Imaging was performed using a 3-T MRI system (Vantage Galan; Canon Medical Systems, Tochigi, Japan) with a 16-channel head coil. The participant lay in the MRI machine and watched the block design. The images were displayed on a magnetic resonance theater (Canon Medical Systems) and were viewed while the participant lay on his back. The sound source was an MRI headphone system (iMag; Star Products, Tokyo, Japan) with high sound insulation that blocks the scanning sounds.
2.5 MRI acquisition
High-resolution MRI scans were required to obtain detailed anatomical information before fMRI. For this purpose, a high-resolution T1-weighted magnetization-prepared rapid-gradient-echo (MP-RAGE) sequence was used to obtain structural images, with the following parameters: repetition time (TR) = 5.8 ms, echo time (TE) = 2.7 ms, inversion time (TI) = 900 ms, flip angle (FA) = 9, matrix = 256 × 256, field of view (FOV) = 230 × 230 mm, and slice thickness = 1.2 mm. Echoplanar imaging (EPI) was used to capture fMRI scans. The EPI scans were repeated, and images were captured to compare the two stimuli. The imaging conditions for fMRI to cover the entire brain were as follows: TR = 2,000 ms, TE = 25 ms, FA = 85°, matrix = 64 × 64, FOV = 240 × 240 mm, and slice thickness = 3 mm.
2.6 fMRI data analyses
The fMRI data were preprocessed and analyzed using Statistical Parametric Mapping 12 (Wellcome Trust Center for Neuroimaging, London, UK) in MATLAB (MathWorks, Natick, MA). After correcting the time difference using slice-timing correction, the displacement due to motion was corrected using realignment. Coagisters were applied to correct for misalignments between structural and functional images. The data were normalized by aligning each participant’s brain with the Montreal Neurological Institute standard brain coordinate template. The normalized images were smoothed using an 8-mm Gaussian kernel. After pretreatment, we used a general linear model (GLM) to identify changes in brain activity associated with liked and disliked ASMR videos and control videos by block design. Contrast images were created at the first level (single subject) using the following contrasts: (1) Liked ASMR = 1, Rest = 0; (2) Disliked ASMR = 1, Rest = 0; (3) Control = 1, Rest = 0; (4) Liked ASMR = 1, Disliked ASMR = −1. The contrast in (4) can be used to identify brain areas that show significantly increased activity in the non-preferred ASMR condition compared with that in the preferred ASMR condition. In the next group analysis (second level), a one-sample t-test was performed using the four aforementioned contrasts. The initial threshold for the voxel level was set at an uncorrected p < 0.001. Clusters were corrected for the false discovery rate (FDR), with those meeting p < 0.05 considered significant. Each of the three stimulus tasks was analyzed by subtracting disliked ASMR from liked ASMR. We also investigated the correlation between the relaxed and tingling mood scores for the liked and disliked ASMR videos.
3 Results
The participants in this study were ASMR enthusiasts, none of whom reported feeling somatosensory experiences such as creepiness during the experiment. fMRI showed significant activation in the left fusiform gyrus, right opercular part of the inferior frontal gyrus, left precentral gyrus, and left precuneus when the control videos were being viewed (FDR corrected, p < 0.05). The disliked ASMR videos showed significant activation of the right inferior occipital gyrus, right superior temporal gyrus, and left superior parietal lobule (FDR corrected, p < 0.05). The liked ASMR videos showed significant activation in the left inferior occipital gyrus, right amygdala, right and left insula, right postcentral gyrus, right superior parietal lobule, and left middle frontal gyrus (FDR corrected, p < 0.05). Table 1 shows the statistics and coordinates of the areas that were significantly activated during the stimulation. The axial images of the areas activated during the stimulation task are shown in Figures 2–4. Analysis of liked ASMR videos subtracted from disliked ASMR videos revealed no significant activation sites. No sites showed any correlation with scores for the two moods.
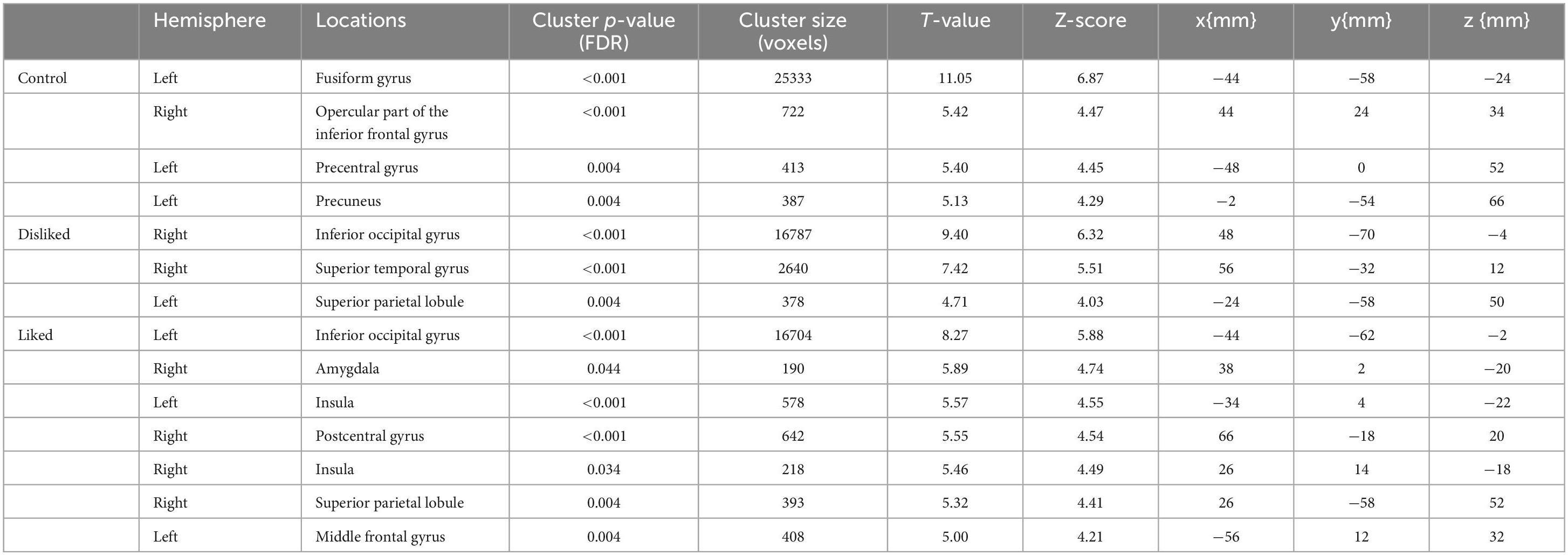
Table 1. P-values and coordinates of the areas significantly activated during video viewing (control, disliked ASMR, and liked ASMR videos).
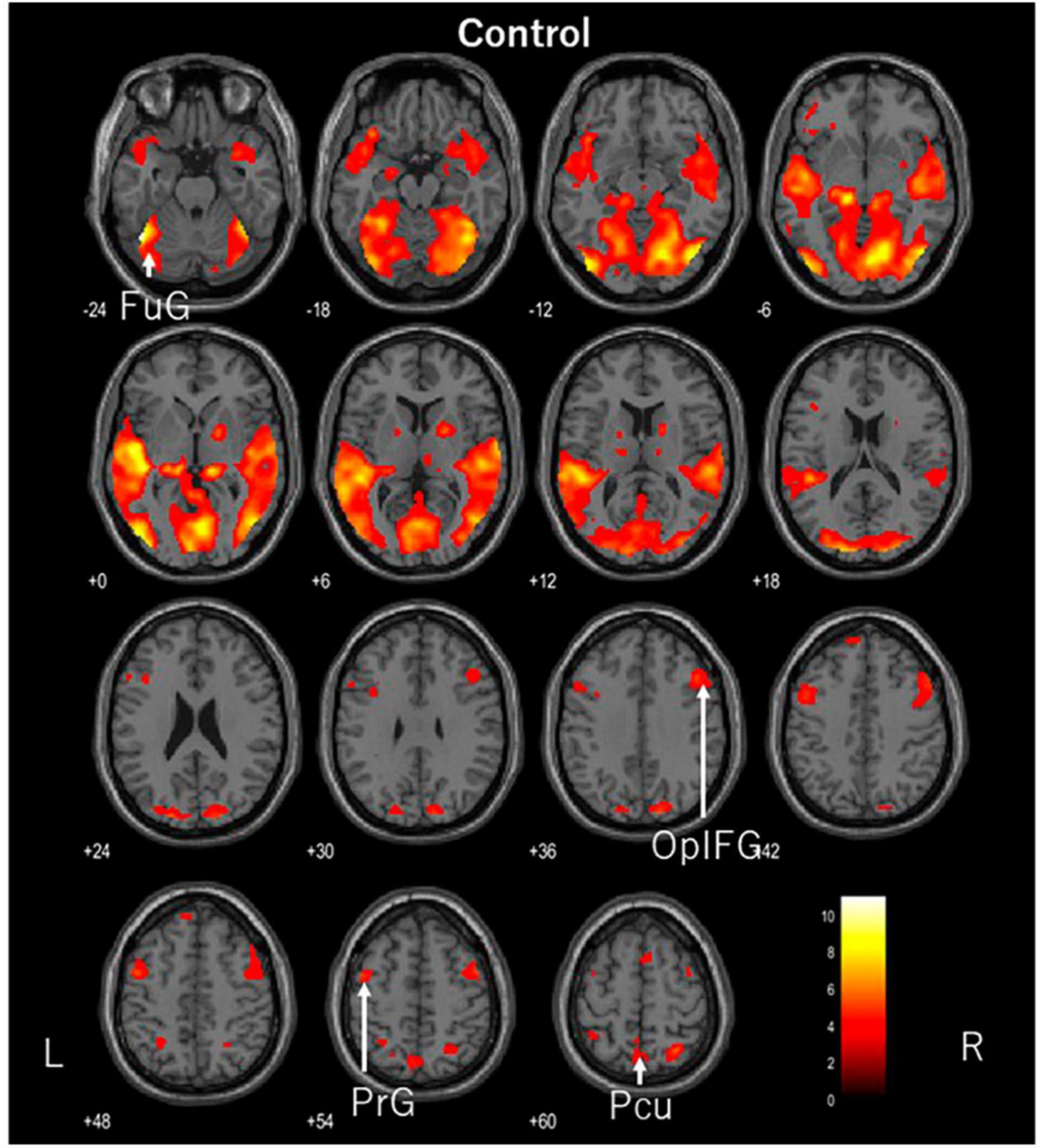
Figure 2. Axial images of the whole brain during viewing of the control video. The images show activation of the left fusiform gyrus (FuG), right opercular part of the inferior frontal gyrus (OplFG), left precentral gyrus (PrG), and left precuneus (Pcu).
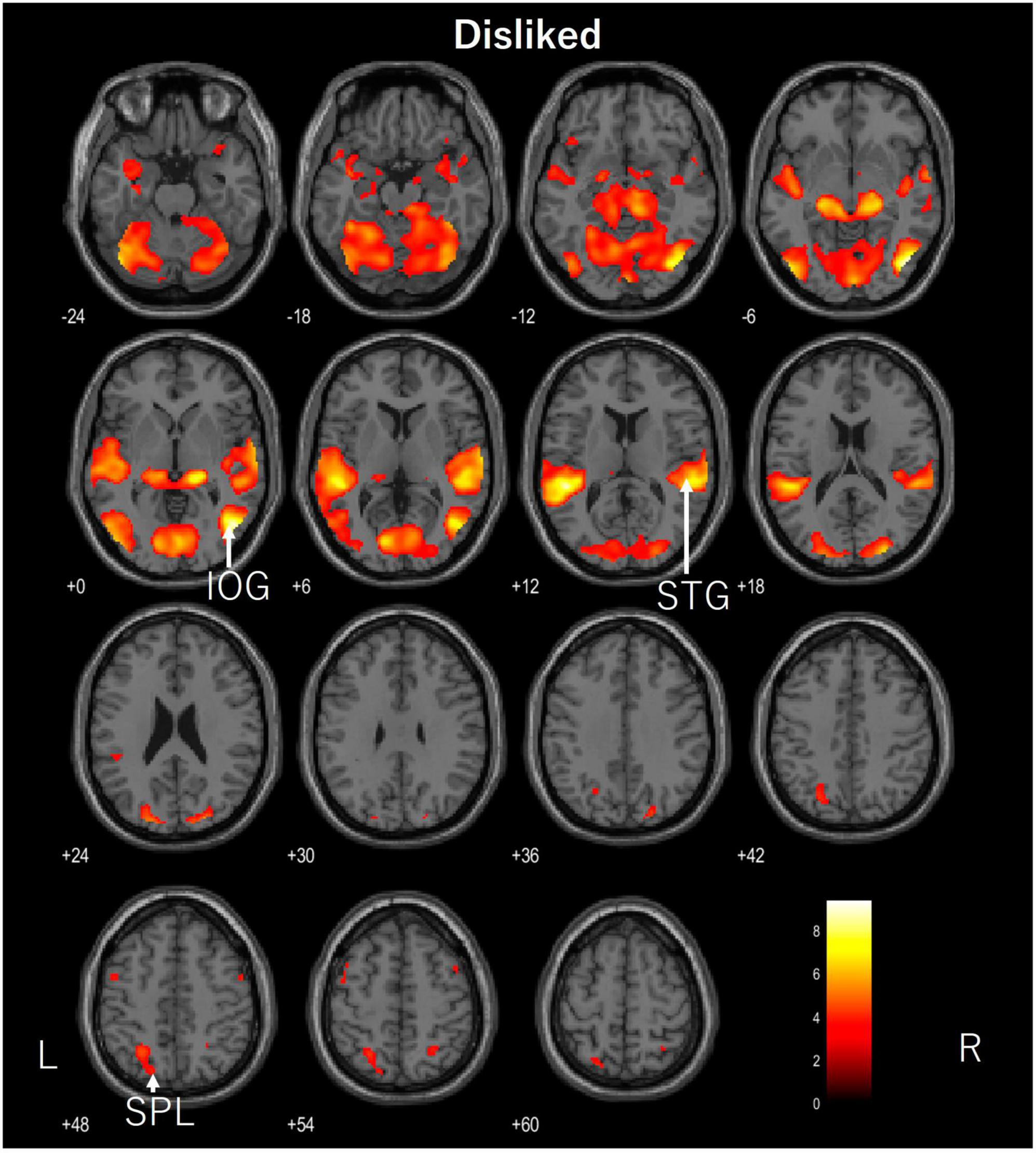
Figure 3. Axial images of the whole brain during viewing of the disliked ASMR video. The images show activation of the right inferior occipital gyrus (IOG), right superior temporal gyrus (STG), and left superior parietal lobule (SPL). ASMR, autonomous sensory meridian response.
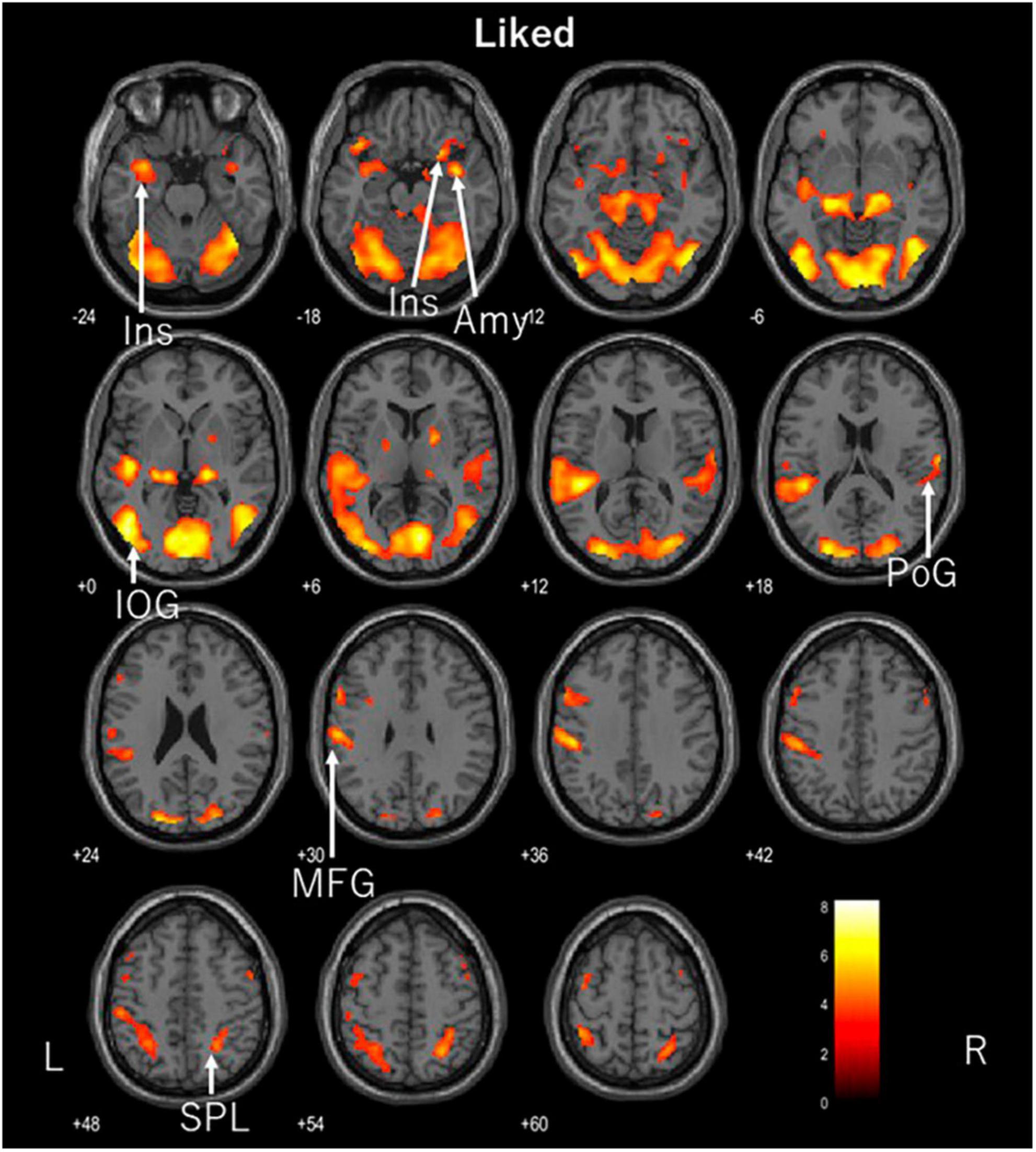
Figure 4. Axial images of the whole brain during viewing of liked ASMR videos. The images show activation of the left inferior occipital gyrus (IOG), right amygdala (Amy), right and left insula (Ins), right postcentral gyrus (PoG), right superior parietal lobule (SPL), and left middle frontal gyrus (MFG). ASMR, autonomous sensory meridian response.
4 Discussion
To our knowledge, this is the first study to identify the relaxation effects of ASMR videos based on personal preferences. We compared areas of the brain activated when viewing control, disliked, and liked ASMR videos. The temporal and occipital lobes were activated in the auditory and visual cortices, indicating their activation as large clusters because the task involved moving pictures. We also focused on other characteristic areas.
While the participants watched the control video, the left fusiform gyrus, right opercular part of the inferior frontal gyrus, left precentral gyrus, and left precuneus were activated. The fusiform gyrus is involved in the recognition of human facial expressions, while the opercular part of the inferior frontal gyrus serves as a functional link to various regions. The precentral gyrus is the primary motor cortex but is thought to be activated by eye movements. We believe that visual and somatosensory information is integrated within the precuneus. In this video, a man was lecturing in front of a whiteboard. Although he was expressionless, the person was facing the viewer and his entire face was visible. The lecturer’s facial expression and content may have been captured. Further study is needed on the selection of the control video.
The areas of activation in response to the unlinked ASMR videos were the inferior occipital gyrus, superior temporal gyrus, and superior parietal lobule. The inferior occipital gyrus is a part of the visual system that recognizes color and shape. The superior temporal gyrus is the primary auditory cortex, and the superior parietal lobule is involved in memory. Although the axial image appeared to show significant activation, it did not appear in any particular characteristic area and only in areas related to the visual and auditory cortices.
In contrast, the preferred ASMR videos showed activation in the visual and auditory areas of the inferior occipital gyrus, postcentral gyrus, and superior parietal lobule, as well as in the insular cortex, amygdala, and middle frontal gyrus. We previously compared the areas activated in the brain in response to ASMR videos and sound alone, in which the prefrontal cortex, including the nucleus accumbens, was activated by moving images, whereas the left and right insular cortices were activated by sound-only listening, suggesting that this led to relaxation (Sakurai et al., 2023). The activation of the frontal and insular cortices in this study was consistent with the hypothesis. No activation of the nucleus accumbens was observed; however, the amygdala was activated.
The amygdala plays a key role in emotional processing (Sergerie et al., 2008), is responsible for memory and learning emotional responses, and plays a major role in protecting against potential fear and danger (Adolphs, 1999). It is known primarily for its negative emotional response. We expected a correlation with the current tingling mood score, but there was no correlation. The amygdala, like the nucleus accumbens, is part of the network through which information circulates in the mesolimbic dopamine circuitry. Pleasurable emotions project dopamine to the nucleus accumbens via the frontal cortex, whereas the amygdala facilitates reward-seeking behavioral responses (Ambroggi et al., 2008). The amygdala is also an important part of the mesolimbic dopamine pathway, which links emotions to physiological responses. The amygdala was activated only on the right side. Regarding differences between the left and right brain hemispheres, the left hemisphere is significant for semantic material, such as language, while the right hemisphere is significant for non-semantic information, such as emotion (Markowitsch, 1998; Anderson and Phelps, 2001). In response to liked ASMR videos, the right amygdala, which may be involved in emotion processing, was activated. The mesolimbic dopamine circuit is also involved in stress, and is reported to be activated by social defeat stress and weakened by repeated social defeat stress. Furthermore, social defeat stress is a cause of psychiatric disorders such as depression (Tanaka et al., 2012).
Regarding the left-right difference in the insular cortices, the left and right insular cortices are involved in parasympathetic and sympathetic responses, respectively (Oppenheimer et al., 1992). In the present study, both the left and right insular cortices were activated. This state of autonomic balance is considered an interaction. This suggests that using liked ASMR videos may be more effective in inducing relaxation and autonomic balance than using control or disliked ASMR videos. The insular cortex also integrates the internal senses, which recognize the conscious perception of bodily responses, such as visceral sensation and autonomic control, with external senses, such as emotion and empathy (Uddin et al., 2017). This cross-sensory (decreased heart rate) and extrasensory (increased activity in somatosensory, motor, visual, and auditory cortices) activity is associated with the positive effects of ASMR on emotion via the autonomic nervous system (Poerio et al., 2022). We believe that the present study provides support that this cross-activity response occurs because of preferred ASMR videos, although not tingling.
An fMRI study by Datko et al. (2022) reported increased insular cortex activation after mindfulness training in patients with anxiety and depression (Datko et al., 2022), consistent with the insular cortical activation in the present study. Therefore, we believe that the effects of ASMR videos are similar to the inner receptive sensory awareness of mindfulness. Previously, Fredborg et al. (2018) suggested a similar effect to a mindfulness-based treatment program using ASMR. The increased relaxation and positive mood of ASMR also suggests that it may have a particularly beneficial effect on individuals with anxiety and depression (Barratt et al., 2017; Poerio et al., 2018; Smejka and Wiggs, 2022). This finding was further supported by functional brain mechanisms in the present study.
We hypothesized that ASMR videos would significantly increase activity in the nucleus accumbens, frontal cortex, and insular cortex, which are brain areas linked to the relaxation effect. The results showed activation in the amygdala, frontal cortex, and insular cortex in liked ASMR, suggesting involvement in mesolimbic dopamine circuits and autonomic balance. We propose that ASMR videos may be effective in counteracting depression and anxiety beyond relaxation by finding and selecting the ones you prefer. The results also support this from the viewpoint of brain function mechanisms, as viewing ASMR videos has already been incorporated into anxiety and depression prevention measures. We believe that this evidence provides further support for the effectiveness of ASMR.
However, this study has several limitations. First, comparisons with mindfulness were not performed. Thus, future comparative studies of the similarities and differences in mindfulness are needed. Second, the study did not evaluate participants with anxiety or depression; therefore, the effects are speculative. Finally, the connectivity in the activated areas should be tested using fMRI in real-life settings.
5 Conclusion
In the present study, emotion-related activation of the amygdala, frontal cortex, and insular cortex was observed only when participants watched their preferred ASMR videos. These areas may be involved in relaxation, autonomic balance, and mindfulness. The results support the introduction of ASMR videos into mental health care in the future, since ASMR videos are easy to access and can be used by anyone. It is desirable for more people to be aware of and use ASMR videos.
Data availability statement
The original contributions presented in this study are included in this article/supplementary material, further inquiries can be directed to the corresponding author.
Ethics statement
The studies involving humans were approved by the Research Ethics Committee of Niigata University of Health and Welfare. The studies were conducted in accordance with the local legislation and institutional requirements. The participants provided their written informed consent to participate in this study.
Author contributions
NS and NK conceived the study and designed experiments. NS, KN, KS, YY, ST, and NK collected the MR data and performed the statistical analyses. NS and KN interpreted the data. NS, SK, HO, and NK drafted the manuscript. All authors approved the final version of the manuscript.
Funding
This study was supported by KAKENHI (No. 20K11059) from the Japan Society for the Promotion of Science.
Acknowledgments
We would like to thank Editage (www.editage.com) for English-language editing.
Conflict of interest
The authors declare that the research was conducted in the absence of any commercial or financial relationships that could be construed as a potential conflict of interest.
Publisher’s note
All claims expressed in this article are solely those of the authors and do not necessarily represent those of their affiliated organizations, or those of the publisher, the editors and the reviewers. Any product that may be evaluated in this article, or claim that may be made by its manufacturer, is not guaranteed or endorsed by the publisher.
References
Adolphs, R. (1999). The human amygdala and emotion. Neuroscientist 5, 125–137. doi: 10.1177/107385849900500216
Ambroggi, F., Ishikawa, A., Fields, H. L., and Nicola, S. M. (2008). Basolateral amygdala neurons facilitate reward-seeking behavior by exciting nucleus accumbens neurons. Neuron 59, 648–661. doi: 10.1016/j.neuron.2008.07.004
Anderson, A. K., and Phelps, E. A. (2001). Lesions of the human amygdala impair enhanced perception of emotionally salient events. Nature 411, 305–309. doi: 10.1038/35077083
Barratt, E. L., and Davis, N. J. (2015). Autonomous sensory meridian response (ASMR): A flow-like mental state. PeerJ 3:e851. doi: 10.7717/peerj.851
Barratt, E. L., Spence, C., and Davis, N. J. (2017). Sensory determinants of the autonomous sensory meridian response (ASMR): Understanding the triggers. PeerJ 5:e3846. doi: 10.7717/peerj.3846
Cardona, G., Rodriguez-Fornells, A., Nye, H., Rif-Ros, X., and Ferreri, L. (2020). The impact of musical pleasure and musical hedonia on verbal episodic memory. Sci. Rep. 10:16113. doi: 10.1038/s41598-020-72772-3
Datko, M., Lutz, J., Gawande, R., Comeau, A., To, M. N., Desel, T., et al. (2022). Increased insula response to interoceptive attention following mindfulness training is associated with increased body trusting among patients with depression. Psychiatry Res. Neuroimaging 327:111559. doi: 10.1016/j.pscychresns.2022.111559
Engelbregt, H. J., Brinkman, K., van Geest, C. C. E., Irrmischer, M., and Deijen, J. B. (2022). The effects of autonomous sensory meridian response (ASMR) on mood, attention, heart rate, skin conductance and EEG in healthy young adults. Exp. Brain Res. 240, 1727–1742. doi: 10.1007/s00221-022-06377-9
Fredborg, B. K., Champagne-Jorgensen, K., Desroches, A. S., and Smith, S. D. (2021). An electroencephalographic examination of the autonomous sensory meridian response (ASMR). Conscious. Cogn. 87:103053. doi: 10.1016/j.concog.2020.103053
Fredborg, B. K., Clark, J. M., and Smith, S. D. (2018). Mindfulness and autonomous sensory meridian response (ASMR). PeerJ 6:e5414. doi: 10.7717/peerj.5414
Fredborg, B., Clark, J., and Smith, S. D. (2017). An examination of personality traits associated with autonomous sensory meridian response (ASMR). Front. Psychol. 8:247. doi: 10.3389/fpsyg.2017.00247
Lee, M., Song, C.-B., Shin, G.-H., and Lee, S.-W. (2019). Possible effect of binaural beat combined with autonomous sensory meridian response for inducing sleep. Front. Hum. Neurosci. 13:425. doi: 10.3389/fnhum.2019.00425
Lee, S., Kim, J., and Tak, S. (2020). Effects of autonomous sensory meridian response on the functional connectivity as measured by functional magnetic resonance imaging. Front. Behav. Neurosci. 14:154. doi: 10.3389/fnbeh.2020.00154
Lochte, B. C., Guillory, S. A., Richard, C. A. H., and Kelley, W. M. (2018). An fMRI investigation of the neural correlates underlying the autonomous sensory meridian response (ASMR). Bioimpacts 8, 295–304.
Markowitsch, H. J. (1998). Differential contribution of right and left amygdala to affective information processing. Behav. Neurol. 11, 233–244. doi: 10.1155/1999/180434
Oppenheimer, S. M., Gelb, A., Girvin, J. P., and Hachinski, V. C. (1992). Cardiovascular effects of human insular cortex stimulation. Neurology 42, 1727–1732. doi: 10.1212/wnl.42.9.1727
Poerio, G. L., Blakey, E., Hostler, T. J., and Veltri, T. (2018). More than a feeling: Autonomous sensory meridian response (ASMR) is characterized by reliable changes in affect and physiology. PLoS One 13:e0196645. doi: 10.1371/journal.pone.0196645
Poerio, G. L., Ueda, M., and Kondo, H. M. (2022). Similar but different: High prevalence of synesthesia in autonomous sensory meridian response (ASMR). Front. Psychol. 13:990565. doi: 10.3389/fpsyg.2022.990565
Poerio, G. L., Osman, F., Todd, J., Kaur, J., Jones, L., and Cardini, F. (2023). From the outside in: ASMR is characterised by reduced interoceptive accuracy but higher sensation seeking. Multisens. Res. doi: 10.1163/22134808-bja10108
Roberts, N., Beath, A., and Boag, S. (2020). A mixed-methods examination of autonomous sensory meridian response: Comparison to frisson. Conscious. Cogn. 86:103046. doi: 10.1016/j.concog.2020.103046
Sakurai, N., Nagasaka, K., Takahashi, S., Kasai, S., Onishi, H., and Kodama, N. (2023). Brain function effects of autonomous sensory meridian response (ASMR) video viewing. Front. Neurosci. 17:1025745. doi: 10.3389/fnins.2023.1025745
Sakurai, N., Ohno, K., Kasai, S., Nagasaka, K., Onishi, H., and Kodama, N. (2021). Induction of relaxation by autonomous sensory meridian response. Front. Behav. Neurosci. 15:761621. doi: 10.3389/fnbeh.2021.761621
Sergerie, K., Chochol, C., and Armony, J. L. (2008). The role of the amygdala in emotional processing: A quantitative meta-analysis of functional neuroimaging studies. Neurosci. Biobehav. Rev. 32, 811–830. doi: 10.1016/j.neubiorev.2007.12.002
Smejka, T., and Wiggs, L. (2022). The effects of autonomous sensory meridian response (ASMR) videos on arousal and mood in adults with and without depression and insomnia. J. Affect. Disord. 301, 60–67. doi: 10.1016/j.jad.2021.12.015
Smith, S. D., Fredborg, B. K., and Kornelsen, J. (2019). Atypical functional connectivity associated with autonomous sensory meridian response: An examination of five resting-state networks. Brain Connect. 9, 508–518. doi: 10.1089/brain.2018.0618
Swart, T. R., Bowling, N. C., and Banissy, M. J. (2021). ASMR-Experience Questionnaire (AEQ): A data-driven step towards accurately classifying ASMR responders. Br. J. Psychol. 113, 68–83. doi: 10.1111/bjop.12516
Tanaka, K., Furuyashiki, T., Kitaoka, S., Senzai, Y., Imoto, Y., Segi-Nishida, S. E., et al. (2012). Prostaglandin E2-mediated attenuation of mesocortical dopaminergic pathway is critical for susceptibilitly to repeated social defeat stress in mice. J. Neurosci. 32, 4319–4329. doi: 10.1523/JNEUROSCI.5952-11.2012
Uddin, L. Q., Nomi, J. S., Hébert-Seropian, B., Ghaziri, J., and Boucher, O. (2017). Structure and function of the human insula. J. Clin. Neurophysiol. 34, 300–306. doi: 10.1097/WNP.0000000000000377
Valtakari, N. V., Hooge, I. T. C., Benjamins, J. S., and Keizer, A. (2019). An eye-tracking approach to autonomous sensory meridian response (ASMR): The physiology and nature of tingles in relation to the pupil. PLoS One 14:e0226692. doi: 10.1371/journal.pone.0226692
Vardhan, V. V., Venkatesh, U., and Yadav, S. (2020). “Signal processing based autonomous sensory meridian response to treat insomnia,” in Proceedings of the International Conference on Electronics and Sustainable Communication Systems (ICESC). Coimbatore, India, 2-4 July 2020, Coimbatore. doi: 10.1109/ICESC48915.2020.9155950
Keywords: ASMR, insular cortices, amygdala, relaxation, fMRI
Citation: Sakurai N, Nagasaka K, Sasaki K, Yuguchi Y, Takahashi S, Kasai S, Onishi H and Kodama N (2023) The relaxation effect of autonomous sensory meridian response depends on personal preference. Front. Hum. Neurosci. 17:1249176. doi: 10.3389/fnhum.2023.1249176
Received: 29 June 2023; Accepted: 16 November 2023;
Published: 05 December 2023.
Edited by:
Moussa Antoine Chalah, GHU Paris Psychiatrie et Neurosciences, FranceReviewed by:
Giuliana Lucci, Università degli Studi Guglielmo Marconi, ItalyMarkus Zedler, Hannover Medical School, Germany
Copyright © 2023 Sakurai, Nagasaka, Sasaki, Yuguchi, Takahashi, Kasai, Onishi and Kodama. This is an open-access article distributed under the terms of the Creative Commons Attribution License (CC BY). The use, distribution or reproduction in other forums is permitted, provided the original author(s) and the copyright owner(s) are credited and that the original publication in this journal is cited, in accordance with accepted academic practice. No use, distribution or reproduction is permitted which does not comply with these terms.
*Correspondence: Naoki Kodama, kodama@nuhw.ac.jp