- 1Departments of Behavioral Neuroscience, Neurology, and Radiation Medicine, Division of Neuroscience, ONPRC, Oregon Health & Science University, Portland, OR, United States
- 2Department of Neurology, Oregon Alzheimer’s Disease Research Center, Oregon Health & Science University, Portland, OR, United States
- 3Department of Neurology, Veterans Affairs Portland Health Care System, Portland, OR, United States
Magnetic Resonance Imaging (MRI) T2-weighted white matter hyperintensity (WMH) is a marker of small vessel cerebrovascular pathology and is of ischemic origin. The prevalence and severity of WMH is associated with cardiovascular risk factors, aging, and cognitive injury in mild cognitive impairment (MCI), vascular dementia, and Alzheimer’s disease (AD). WMH especially affects executive function, with additional effects on memory and global cognition. Apolipoprotein E (apoE) plays a role in cholesterol metabolism and neuronal repair after injury. Human and animal studies support a role for apoE in maintaining white matter integrity. In humans, there are three major human apoE isoforms, E2, E3, and E4. Human apoE isoforms differ in risk to develop AD and in association with WMH. In this Mini Review, we propose an increased focus on the role of WMH in cognitive health and cognitive injury and the likely role of apoE and apoE isoform in modulating these effects. We hypothesize that apoE and apoE isoforms play a role in modulating WMH via apoE isoform-dependent effects on oxylipins and 7-ketocholesterol, as well as amyloid related vascular injury, as seen in cerebral amyloid angiopathy.
1. Introduction
In humans, Magnetic Resonance Imaging (MRI) T2-weighted white matter hyperintensity (WMH), a marker of small vessel cerebrovascular pathology (Pantoni, 2010), is of ischemic origin, and regions of white matter with reduced blood flow have been shown to develop new WMH on follow-up imaging (Bernbaum et al., 2015; Promjunyakul et al., 2015). The prevalence and severity of WMH is associated with cardiovascular risk factors like hypertension (Liao et al., 1996) and with aging and cognitive injury in mild cognitive impairment (MCI) (Vettore et al., 2021), vascular dementia (Alber et al., 2019), and Alzheimer’s disease (AD) (Prins and Scheltens, 2015; Boyle et al., 2016; Lee et al., 2016; Schoemaker et al., 2022). The predominant impact of WMH on cognition is in executive function, although relationships with memory and global cognition have also been observed (Hedden et al., 2012; Debette and Markus, 2010). The concept of reserve may explain variability in the impact of WMH on cognitive function, with results from a previous study demonstrating that, for a given level of performance on neuropsychological testing, WMH were higher in those with greater cognitive and brain reserve (Brickman et al., 2011). Additional mediating factors may include age (van Dijk et al., 2008; Garnier-Crussard et al., 2020), sex (de Leeuw et al., 2001; Brickman et al., 2011), and lesion location (Mortamais et al., 2013; Lampe et al., 2019). Confluent WMH seems more associated with cognitive injury than non-confluent WMH (Kumar et al., 2020) and genetic and environmental factors modulate this relationship (Prins and Scheltens, 2015).
Apolipoprotein E (apoE) plays a role in cholesterol metabolism and neuronal repair after injury (Mahley, 1988). ApoE, a component of lipoprotein particles, binds to surface receptors like low-density lipoprotein receptor (LDLR) family members as part of this role. In addition, apoE modulates synaptic function, glucose metabolism, and cerebrovascular function (Yamazaki et al., 2019). Some effects of apoE outside the brain may ultimately affect the brain. For example, apoE is expressed in the adrenal gland and modulates the adrenal secretion of corticosterone and regulation of the hypothalamic-pituitary-adrenal axis (Raber et al., 2000). ApoE is also expressed in the gut (Niemi et al., 2002) and modulated inflammation, gastrointestinal health, and the gut microbiome composition (Tran et al., 2019). Outside the brain, most apoE is expressed in the liver and apoE secreted from the liver can also affect the brain, as illustrated in humanized liver mice (Giannisis et al., 2022; Kessler et al., 2023). In humans, there are three major human apoE isoforms, E2, E3, and E4. Compared to E3, E4 is associated with increased risk to develop cardiovascular disease and AD (Farrer et al., 1997). These E4 effects are sex- and ethnicity-dependent (Farrer et al., 1997; Turney et al., 2020). In contrast, compared to E3, E2 is associated with reduced risk of developing AD (Farrer et al., 1997). However, E2 is associated with slightly reduced LDL-cholesterol levels and in the presence of other risk factors homozygous E2 carriers can develop type III hyperlipoproteinemia, an atherogenic disorder characterized by an accumulation of remnants of triglyceride-rich lipoproteins (Khalil et al., 2021). In those cases, E2 is also associated with increased risk of developing type II Diabetes Mellitus (Santos-Ferreira et al., 2019), which especially in E4 carriers is associated with cognitive injury and AD risk (Jangra and Tople, 2022). In patients with cerebral amyloid angiopathy (CAA), a degenerative vasculopathy associated with lobar intracerebral or sulcal hemorrhage, E2 is associated with a higher incidence of intracerebral hemorrhage under oral anticoagulation (Charidimou et al., 2015; Block and Dafotakis, 2017).
In this Mini Review, we hypothesize the role of WMH in effects of apoE and apoE isoform on cognitive injury.
1.1. ApoE isoform and WMH in humans
In cognitively healthy adults (45–75 years of age), pathological WMH is seen in E4 homozygotes but not E4 heterozygotes, suggesting a gene-dose effect (Rojas et al., 2017; Operto et al., 2018). While aging, hypertension and cardiovascular and dementia risk scales are also associated with pathological WMH, they do not modulate the effect of E4/E4 homozygosity (Rojas et al., 2017). However, in participants of the United Kingdom Biobank (mean age: 62 years of age), E3/E4 heterozygous carriers, in addition to E4/E4 homozygous carriers, had greater WMH burden than E3/E3 carriers. Of note, E2/E4 heterozygosity was not related to greater WMH burden, consistent with a relative protective E2 effect on white matter integrity (Lyall et al., 2020). In a longitudinal study, WMH increased more over a 3-year period in E4 than non-E4 carriers, defined as having at least one e4 allele thus including heterozygous carriers (Cox et al., 2017). However, no effect of E4 carrier status (E2/E4, E3/E4, and E4/E4) on WMH burden was seen in a much smaller study (Lyall et al., 2015). In this latter study E2/E4 carriers were included, while they were not in the earlier study in which WMH was seen in both E4/E4 and E3/E4 carriers. Based on their relative protective effect of E2 versus the relative enhanced risk of E4, as compared to E3 (Farrer et al., 1997; Wisdom et al., 2011), including E2/E4 carriers can make the results harder to interpret.
Microstructural integrity disruption of the white matter, as measured by diffusion tensor imaging (DTI), is thought to be a sensitive indicator of early white matter damage that precedes macrostructural T2 WMH formation (Promjunyakul et al., 2018). Using DTI, decreased fractional anisotropy (indicating diminished WM directionality), greater radial diffusivity (indicating myelin disruption) and greater mean diffusivity (indicating greater water diffusion regardless of direction) are common indicators of diminished white matter integrity. DTI radial diffusivity seems especially increased in cognitively healthy E4/E4 carriers, suggesting a disruption in the myelin sheath rather than axonal damage (Operto et al., 2018). However, in different studies including heterozygous E4 carriers or only E3/E4 but not E2/E4 heterozygous carriers distinct alterations of white matter in E4 carriers have been reported. In 20–35 and 50–78 year-old E4 carriers, a reduction in fractional anisotropy and an increase in mean diffusivity, as compared to age-matched non E4 carriers, were reported (Heise et al., 2010). A decrease in fractional anisotropy in E4 versus non-E4 carriers were reported in people over 60 (Westlye et al., 2012) and between 49 and 79 years of age (Persson et al., 2006), respectively. However, in 21–70 year old E3/E4 versus E3/E3 carriers, so excluding E2/E4 carriers, an increase in mean and radial diffusivity were reported (Westlye et al., 2012). In general, those studies support that E4 affects white matter in an age-independent fashion. However, other studies support age-dependent E4 effects on the white matter (Ryan et al., 2011; Adluru et al., 2014). These divergent findings highlight the need for longitudinal and environmentally controlled human apoE animal studies.
In Alzheimer’s disease, WMH is more widespread in E4 carriers (E2/E4, E3/E4, and E4/E4) but more focal (posterior predominant) in non-E4 carriers (Slattery et al., 2017). In contrast, while WMH was not different between E4 and non-E4 AD patients in one study, WMH volume was associated with worse cognitive performance in all cognitive domains in E4 carriers only with AD or Lewy Body dementia (Mirza et al., 2019). On the other hand, in a combined cohort from cognitively healthy to probable AD patients, E4 homozygotes showed more WMH accumulation per year than non-E4 carriers (Sudre et al., 2017). In addition, in prodromal AD patients, E4 carriers had higher global, temporal, and occipital WMH and poorer memory than non -E4 carriers and E4 strengthened the inverse relationship between WMH and episodic memory (Kumar et al., 2022).
The relationship between WMH and risk of disease and biomarkers of disease seems apoE isoform-dependent. In a meta-analysis, E4 carrier status and E4 homozygosity was associated with increasing WMH and cerebral microbleeds (Schilling et al., 2013). In the same analyses, E2 carrier status was associated with increasing WMH and risk to develop a brain infarct (Schilling et al., 2013).
Amyloid β (Aβ) plaques (Pietroboni et al., 2020) are a hallmark of AD pathology. The AD biomarker tau, not Aβ, is associated with changes in anterior temporal WM integrity (Strain et al., 2018). Remarkably, while WMH is associated with axonal damage, tau independently contributed to the model and decline in white matter is associated with early tau accumulation (Strain et al., 2018).
In E4/E4 carriers with MCI or AD, there was a negative association between WMH and cerebrospinal levels of Aβ42 (Sharms et al., 2022). In contrast, in E3/E3 carriers, WMH was associated with lower levels of total and phosphorylated tau (Sharms et al., 2022). In E2 carriers, including those with MCI, more WMH is seen (Sharms et al., 2022). E2 carriers, like E4 carriers, show an association with WMH but, compared to E3, E2 reduces while E4 increases AD risk. These results suggest that risk to develop AD and to develop cognitive impairments due to WMH are two distinct phenomena. Consistent with this notion, severe WMH is associated with profound cognitive impairments in conditions that like seen in cerebral autosomal dominant arteriopathy with subcortical infarcts and leukoencephalopathy (CADASIL), a rare monogenic cerebral small vessel disease (Jouvent et al., 2020), that is not related to AD (Jolly et al., 2022). Especially in AD, apoE isoform-dependent pathologies related to cortical Aβ and hyperphosphorylated tau might be important to develop cognitive injury that is additive to that resulting from white matter injury alone.
White matter capillaries might play an important role in WMH. White matter capillary width is increased in AD, dementia with Lewy bodies, Parkinson’s disease with dementia, vascular dementia, mixed dementias, and post-stroke dementia (Hase et al., 2019). This was suggested to represent a compensatory change to retain white matter perfusion under hypoperfusion conditions (Hase et al., 2019). WMH and cognitive impairments are core features of CAA (Charidimou et al., 2022). In CAA, E4 carriers are at greater risk of Aβ40 deposition in the vasculature, while E2 carriers are at increased risk for vessel breakdown and subsequent cerebral hemorrhage (Greenberg et al., 2020). Amyloid related imaging abnormalities (ARIA) in the setting of anti-amyloid monoclonal antibody therapy for AD consist of punctate WMH (ARIA-E) and often co-localized cerebral microhemorrhages (ARIA-H) (Sperling et al., 2011). The most significant risk factor for ARIA-E in those participating in monoclonal antibody therapeutic trials is E4 carrier status (Sperling et al., 2011; Sevigny et al., 2016; van Dyck et al., 2023). Combined, these findings indicate a critical role of apoE isoform on vascular amyloid deposition and risk for related WMH and hemorrhage in both spontaneous CAA and therapeutic interventions that lead to greater amyloid deposition within the vasculature. Figure 1A illustrates potential pathways involved in WMH and cognitive injury in E4 carriers.
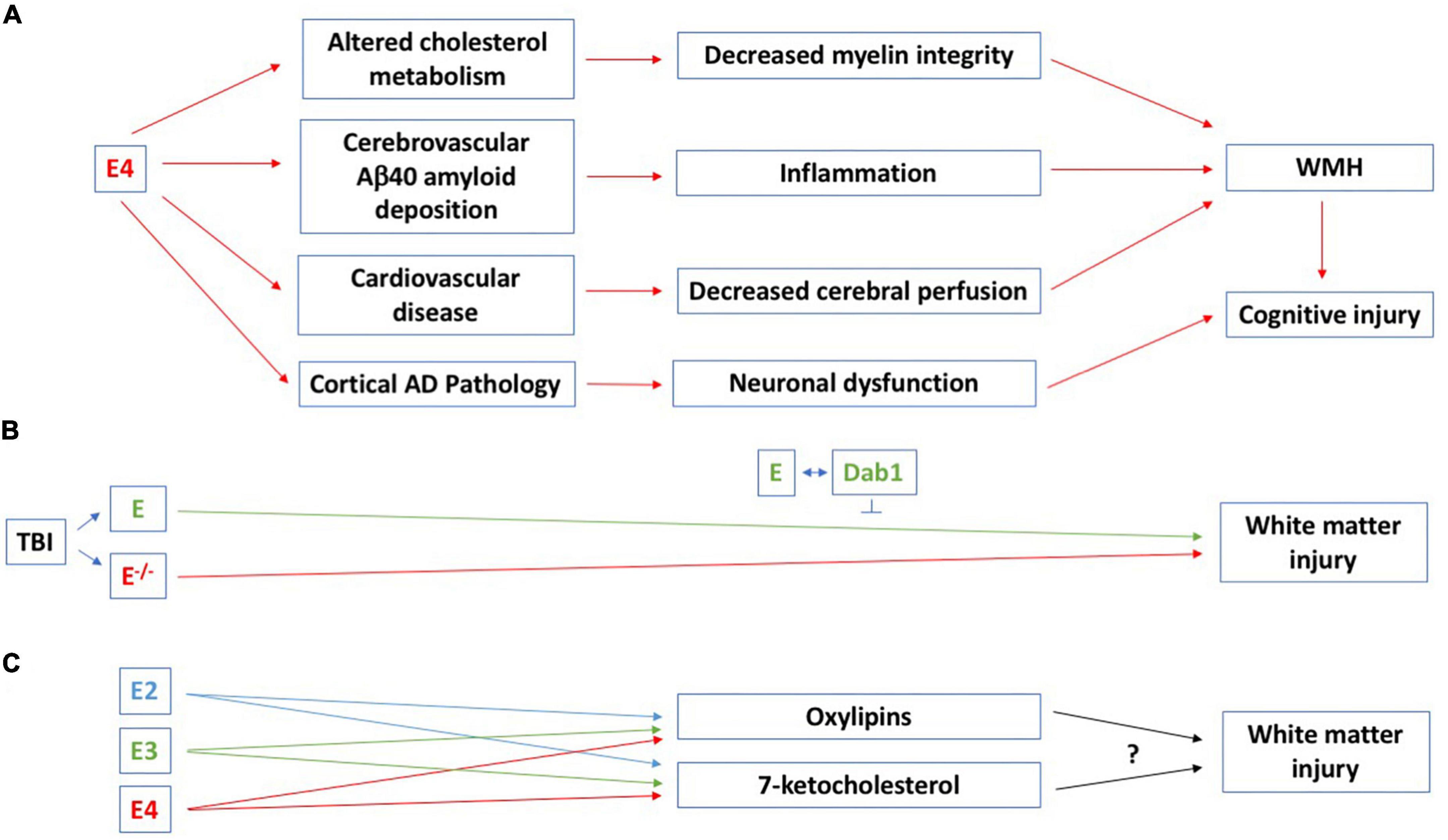
Figure 1. (A) Potential pathways involved in WMH and cognitive injury in E4 carriers. All comparisons are to E3/E3 carriers. In cognitively healthy adults (45–75 years of age), pathological WMH is seen in E4 homozygotes (Rojas et al., 2017; Operto et al., 2018) and in participants of the United Kingdom Biobank (mean age: 62 years of age), there is a greater WMH burden in E3/E4 and E4/E4 than E3/E3 carriers. WMH also increased more over a 3-year period in E4 than non-E4 carriers (Cox et al., 2017). E4 affects cholesterol metabolism and DTI radial diffusivity seems especially increased in cognitively healthy E4/E4 carriers, suggesting a disruption in the myelin sheath (Operto et al., 2018). In E4/E4 carriers with MCI or AD, there is a negative association between WMH and cerebrospinal levels of Aβ42 (Sharms et al., 2022). E4 is a risk factor for cardiovascular disease (Li et al., 2021) and effects on WMH and cognitive injury might involve the cardiovascular system and associated inflammation as well. Atherosclerosis and atrial fibrillation are associated with more WMH and this might involve decreased cerebral perfusion (Emrani et al., 2020). E4 is associated with pathological processes possibly linked to WMH and cognitive injury, including Aβ and tau pathologies. E4 also causes increased mitochondrial Ca2+ and reactive oxygen species (ROS) levels, mitochondrial dysfunction, and neurotoxicity (Pires and Rego, 2023), which might mediate this effect. (B) A role for apoE in white matter integrity in the controlled cortical impact model of traumatic brain injury was revealed 28 days following traumatic injury. The beneficial effects of apoE on white matter integrity involve intracellular adaptor protein Disabled-1 (Dab1) (Abadesco et al., 2014). (C) The effects of distinct human apoE isoforms on white matter integrity might be mediated by oxylipins and 7-ketocholesterol. In cognitively healthy hypertensive study participants, docosahexaenoic acid oxylipins are associated with white matter integrity (Silbert et al., 2020). APOE genotype modifies the plasma oxylipin response to Omega-3 Polyunsaturated Fatty Acid [eicosapentaenoic acid (EPA) and docosahexaenoic acid (DHA)] supplementation in cognitively healthy people, with E4 carriers showing the greatest increase in plasma oxylipin levels 12 months following the diet supplement (Saleh et al., 2021). For more details, see main text.
1.2. E4 and WMH in mice
To study E4 and WMH in mice, a bilateral carotid artery stenosis model of cerebral hypoperfusion was used in mice with targeted replacement (TR) of E3 or E4. In this mouse model Dr. Sullivan generated (Sullivan et al., 1997; Knouff et al., 1999), human apoE is expressed under control of a mouse apoE promoter. White matter blood flow, local hypoxia and white matter injury were more severely affected in E4 mice than E3 or wild-type mice (Koizumu et al., 2018). In addition, hippocampus-dependent spontaneous alternation in the Y maze and object recognition involving 24 hour delay between learning and memory testing were more severely affected in E4 mice than E3 or wild-type mice (Koizumu et al., 2018), supporting the likely relationship between WMH and cognition. Comparing E4 to E3 or wild-type mice, the following results were observed: (1) More demyelination in the corpus collosum; (2) more reduced levels of the myelin-associated glycoprotein; (3) a higher ratio of myelin basic protein to the axonal marker SM1312; and (4) more reduced levels of the oligodendrocyte marker Olig2. Consistent with these findings, a more profound loss of integrity of the nodes of Ranvier were observed in a model of cerebral hypoperfusion (Koizumu et al., 2018).
1.3. ApoE and white matter integrity
Studies in mice lacking apoE support a role for apoE in white matter integrity following in the controlled cortical impact model of traumatic brain injury. ApoE-deficient mice showed impaired white matter integrity, analyzed as reduced fractional anisotropy levels, 28 days following traumatic injury and this was associated with cognitive injury (Huang et al., 2020). This effect was delayed, as no genotype difference in fractional anisotropy was seen three days following traumatic brain injury. The beneficial effects of apoE on white matter integrity involve intracellular adaptor protein Disabled-1 (Dab1), which is normally phosphorylated when apoE binds the very-low density lipoprotein receptor and the apoE receptor 2 (Abadesco et al., 2014; Figure 1B). Reduced fractional anisotropy is also seen in completely denervated nerve segments compared to uninjured sciatic nerve and restored toward normal in regenerating nerve segments (Lehman et al., 2010) and associated with cognitive performance in the water maze test in mice 2 months following hypoxia-ischemia at day 9 (Cengiz et al., 2011).
1.4 ApoE, oxylipins, 7-ketocholesterol, and white matter integrity
Effects of distinct human apoE isoforms on white matter integrity might be mediated by oxylipins and 7-ketocholesterol (Figure 1C). Oxylipins are a class of bioactive lipid mediators derived from the oxidation of long-chain polyunsaturated fatty acids (PUFAs). They act as modulators of vascular tone and inflammation and are potential therapeutic targets in AD and related dementias (for a review, see Shinto et al., 2022). In cognitively healthy hypertensive study participants, docosahexaenoic acid oxylipins are associated with white matter integrity (Silbert et al., 2020). APOE genotype modifies the plasma oxylipin response to Omega-3 Polyunsaturated Fatty Acid [eicosapentaenoic acid (EPA) and docosahexaenoic acid (DHA)] supplementation in cognitively healthy people, with E4 carriers showing the greatest increase in plasma oxylipin levels 12 months following the diet supplement (Saleh et al., 2021).
Oxysterols are oxidized metabolites of cholesterol. They can penetrate the blood-brain barrier and modulate cholesterol metabolism in the brain (Björkhem, 2013). Levels of the oxysterol 7-ketocholesterol increase with disease progression in the frontal cortex of AD patients (Testa et al., 2016). Levels of 7-ketocholesterol in the cerebrospinal fluid are associated with white matter integrity in cognitively healthy people (Iriondo et al., 2020); higher levels of 7-ketocholesterol are associated with lower fractional anisotropy (Iriondo et al., 2020). In apoE TR mice, cortical levels of 7-ketocholesterol were increased following chronic variable stress, an animal model of post-traumatic stress disorder, in E4, but not E2 or E3, mice (Torres et al., 2022). Chronic variable stress increased 7-ketocholesterol levels in the liver of E2, E3, and E4 mice (Torres et al., 2022). In addition, independent of chronic variable stress, 7-ketocholesterol levels in plasma were much higher in E2 than E3 or E4 mice (Torres et al., 2022).
2. Conclusion
WMH is associated with cognitive injury in various neurological conditions. We hypothesize that apoE plays a critical role in modulating WMH via apoE isoform-dependent effects on oxylipins and 7-ketocholesterol, as well as amyloid related vascular injury, as seen in CAA (Figure 1). We recognize that as there are relatively few reported studies regarding some of the topics we discussed in this review. Therefore, future studies are warranted to determine the pathways involved in the relationship between apoE isoform, WMH, and cognitive injury.
Author contributions
Both authors have drafted, edit, and agreed to the published version of the manuscript.
Funding
This work was partially supported by the National Institutes of Health (RF1AG059088, R01 AG056712, R21 AG065914, and P30AG066518).
Conflict of interest
The authors declare that the research was conducted in the absence of any commercial or financial relationships that could be construed as a potential conflict of interest.
Publisher’s note
All claims expressed in this article are solely those of the authors and do not necessarily represent those of their affiliated organizations, or those of the publisher, the editors and the reviewers. Any product that may be evaluated in this article, or claim that may be made by its manufacturer, is not guaranteed or endorsed by the publisher.
References
Abadesco, A., Cilluffo, M., Yvone, G., Carpenter, E., Howell, B., and Phelps, P. (2014). Novel disabled-1-expressing neurons identified in adult brain and spinal cord. Eur. J. Neurosci. 39, 579–592. doi: 10.1111/ejn.12416
Adluru, N., Destiche, D., Lu, S. Y.-F., Doran, S., Birdsill, A. C., and Melah, K. E. (2014). White matter microstructure in late middle-age: effects of apolipoprotein E4 and parental family history of Alzheimer’s disease. Neuroimage Clin. 4, 730–742. doi: 10.1016/j.nicl.2014.04.008
Alber, J., Alladi, S., Bae, H.-J., Barton, D. A., Beckett, L. A., and Bell, J. M. (2019). White matter hyperintensities in vascular contributions to cognitive impairment and dementia (VCID): Knowledge gaps and opportunities. Alzheimer Dement. 5, 107–117. doi: 10.1016/j.trci.2019.02.001
Bernbaum, M., Menon, B., Fick, G., Smith, E., Goyal, M., Frayne, R., et al. (2015). Reduced blood flow in normal white matter predicts development of leukoaraiosis. J. Cereb. Blood Flow Metab. 35, 1610–1615. doi: 10.1038/jcbfm.2015.92
Björkhem, I. (2013). Five decades with oxysterols. Biochimie 95, 448–454. doi: 10.1016/j.biochi.2012.02.029
Block, F., and Dafotakis, M. (2017). Cerebral amyloid angiopathy in stroke medicine. Dtsch. Arztebl. Int. 114, 37–42. doi: 10.3238/arztebl.2017.0037
Boyle, P., Yu, F., Fleischman, D., Leurgans, S., Yang, J., and Wilson, R. S. (2016). White matter hyperintensities, incident mild cognitive impairment, and cognitive decline in old age. Ann. Clin. Translational Neurol. 3, 791–800. doi: 10.1002/acn3.343
Brickman, A., Siedlecki, K., Muraskin, J., Manly, J., Luchsinger, J., Yeung, L.-K., et al. (2011). White matter hyperintensities and cognition: testing the reserve hypothesis. Neurobiol. Aging 32, 1588–1598. doi: 10.1016/j.neurobiolaging.2009.10.013
Cengiz, P., Uluc, K., Kendigelen, P., Akture, E., Hutchinson, E., and Song, C. (2011). Chronic neurological deficits in mice after perinatal hypoxia and ischemia correlate with hemispheric tissue loss and white matter injury detected by MRI. Dev. Neurosci. 33, 270–279. doi: 10.1159/000328430
Charidimou, A., Boulouis, G., Frosch, M., Baron, J.-C., Pasi, M., and Albucher, J. (2022). The Boston criteria version 2.0 for cerebral amyloid angiopathy: a multicentre, retrospective, MRI–neuropathology diagnostic accuracy study. Lancet Neurol. 21, 714–725. doi: 10.1016/S1474-4422(22)00208-3
Charidimou, A., Martinez-Ramirez, S., Shoamanesh, A., Oliveira-Filho, J., Frosch, M., and Vashkevich, A. (2015). Cerebral amyloid angiopathy with and without hemorrhage. Neurology 84, 1206–1212. doi: 10.1212/WNL.0000000000001398
Cox, S., Ritchie, S., Dickie, D., Pattie, A., Royle, N. A., and Corley, J. (2017). Interaction of APOE e4 and poor glycemic control predicts white matter hyperintensity growth from 73 to 76. Neurobiol. Aging 54, 54–58. doi: 10.1016/j.neurobiolaging.2017.02.014
de Leeuw, F.-E., De Groot, J., Aachten, E., Oudkerk, M., Ramos, L., Heijboer, R., et al. (2001). Prevalence of cerebral white matter lesions in elderly people: a population based magnetic resonance imaging study. the rotterdam scan study. J. Neurol. Neurosurg Psychol. 70, 9–14. doi: 10.1136/jnnp.70.1.9
Debette, S., and Markus, H. (2010). The clinical importance of white matter hyperintensities on brain magnetic resonance imaging: systematic review and meta-analysis. BMJ 341:341. doi: 10.1136/bmj.c3666
Emrani, S., Arain, H., Demarshall, C., and Nuriel, T. (2020). APOE4 is associated with cognitive and pathological heterogeneity in patients with Alzheimer’s disease: a systematic review. Alzheimers Res. Ther. 12:141. doi: 10.1186/s13195-020-00712-4
Farrer, L. A., Cupples, L. A., Haines, J. L., Hyman, B., Kukull, W. A., Mayeux, R., et al. (1997). Effects of age, sex, and ethnicity on the association between apolipoprotein E genotype and Alzheimer disease. a meta-analysis. J. Am. Med. Assoc. 278, 1349–1356. doi: 10.1001/jama.1997.03550160069041
Garnier-Crussard, A., Bougacha, S., Wirth, M., André, C., Delarue, M., and Landeau, B. (2020). White matter hyperintensities across the adult lifespan: relation to age, Aβ load, and cognition. Alzheimer’s Res. Ther. 12:127. doi: 10.1186/s13195-020-00669-4
Giannisis, A., Patra, K., Edlund, A., Nieto, L., Benedicto-Gras, J., Moussaud, S., et al. (2022). Brain integrity is altered by hepatic APOE ε4 in humanized-liver mice. Mol. Psychiatry 1069, 3533–3543. doi: 10.1038/s41380-022-01548-0
Greenberg, S., Backskai, B., Hernandez-Guillamon, M., Pruzin, J., Sperling, R., and Van Veluw, S. (2020). Cerebral amyloid angiopathy and Alzheimer disease - one peptide, two pathways. Nat. Rev. Neurosci. 16, 30–42. doi: 10.1038/s41582-019-0281-2
Hase, Y., Ding, R., Harrison, G., Hawthorne, E., Li, M., and Chen, H. (2019). White matter capillaries in vascular and neurodegenerative dementias. Acta Neuropahol. Commun. 7:16. doi: 10.1186/s40478-019-0666-x
Hedden, T., Mormino, E., Amariglio, R., Younger, A., Schultz, A., Becker, J., et al. (2012). Cognitive profile of amyloid burden and white matter hyperintensities in cognitively normal older adults. J. Neurosci. 32, 16233–16242. doi: 10.1523/JNEUROSCI.2462-12.2012
Heise, V., Filippini, N., Ebmeier, K., and Mackay, C. (2010). The APOE ?4 allele modulates brain white matter integrity in healthy adults. Mol. Psychiatry 16, 908–916. doi: 10.1038/mp.2010.90
Huang, Z.-J., Cao, F., Wu, Y., Peng, J.-H., Zhong, J.-J., Jiang, Y., et al. (2020). Apolipoprotein E promotes white matter remodeling via the Dab1-dependent pathway after traumatic brain injury. CNS Neurosci. Ther. 26, 698–710. doi: 10.1111/cns.13298
Iriondo, A., Garcia-Sebastian, M., Arrospide, A., Aribba, M., Aurtenetxe, S., and Barandiaran, M. (2020). Cerebrospinal fluid 7-Ketocholesterol level is associated with Amyloid-β42 and white matter microstructure in cognitively healthy adults. J. Alzheimer’s Dis. 76, 643–656. doi: 10.3233/JAD-200105
Jangra, V., and Tople, J. (2022). Can Alzheimer’s disease be secondary to Type-2 diabetes mellitus? Cureus 14:e31273. doi: 10.7759/cureus.31273
Jolly, A., Nannoni, S., Edwards, H., Morris, R., and Markus, H. (2022). Prevalence and predictors of vascular cognitive impairment in patients with CADASIL. Neurology 99, e453–e461. doi: 10.1212/WNL.0000000000200607
Jouvent, E., Duering, M., and Chabriat, H. (2020). Cerebral autosomal dominant arteriopathy with subcortical infarcts and leukoencephalopathy. Stroke 51, 21–28. doi: 10.1161/STROKEAHA.119.024152
Kessler, K., Giannisis, A., Bial, G., Foquet, L., Nielsen, H., and Raber, J. (2023). Behavioral and cognitive performance of humanized APOEε3/ε3 liver mice in relation to plasma apolipoprotein E levels. Sci. Rep. 13:1728. doi: 10.1038/s41598-023-28165-3
Khalil, Y., Rabes, J.-P., Boileau, C., and Varret, M. (2021). APOE gene variants in primary dyslipidemia. Atherosclerosis 328, 11–22. doi: 10.1016/j.atherosclerosis.2021.05.007
Knouff, C., Hinsdale, M. E., Mezdour, H., Altenburg, M. K., Watanabe, M., Quarfordt, S. H., et al. (1999). ApoE structure determines VLDL clearance and atherosclerosis in mice. J. Clin. Invest. 103, 1579–1586. doi: 10.1172/JCI6172
Koizumu, K., Hattori, Y., Ahn, S., Buendia, I., Ciacciarelli, A., and Uekawa, K. (2018). Apoε4 disrupts neurovascular regulation and undermines white matter integrity and cognitive function. Nat. Commun. 9:3816. doi: 10.1038/s41467-018-06301-2
Kumar, D., Vipin, A., Wong, B., Ng, K., and Kandiah, N. (2020). Differential effects of confluent and nonconfluent white matter hyperintensities on functional connectivity in mild cognitive impairment. Brain Connect. 10, 547–554. doi: 10.1089/brain.2020.0784
Kumar, D., Yatawara, C., Wang, B., Wong, B., Tan, Y., Zailan, F., et al. (2022). APOE4 and confluent white matter hyperintensities have a synergistic effect on episodic memory impairment in prodromal dementia. J. Alzheimer’s Dis. 87, 1103–1114. doi: 10.3233/JAD-215556
Lampe, L., Kharabian-Masouleh, S., Kynast, J., Arelin, K., Steele, C. J., and Löffler, M. E. (2019). Lesion location matters: the relationships between white matter hyperintensities on cognition in the healthy elderly. J. Cereb. Blood Flow Metab. 39, 36–43. doi: 10.1177/0271678X17740501
Lee, S., Vigar, F., Zimmerman, M., Barkhede, A., Tosto, G., and Benzinger, T. L. (2016). White matter hyperintensities are a core feature of Alzheimer’s disease: evidence from the dominantly inherited Alzheimer network. Ann. Neurol. 79, 929–939. doi: 10.1002/ana.24647
Lehman, H., Zhang, J., Mori, S., and Sheikh, K. (2010). Diffusion tensor imaging to assess axonal regeneration in peripheral nerves. Exp. Neurol. 223, 238–244. doi: 10.1016/j.expneurol.2009.10.012
Li, M.-H., Kwok, M., and Schooling, M. (2021). Age and sex specific effects of APOE genotypes on ischemic heart disease and its risk factors in the UK Biobank. Sci. Rep. 11:9229. doi: 10.1038/s41598-021-88256-x
Liao, D., Cooper, L., Cai, J., Toole, J., Bryan, N., Hutchinson, R., et al. (1996). Presence and severity of cerebral white matter lesions and hypertension, its treatment, and its control. the ARIC study. atherosclerosis risk in communities study. Stroke 27, 2262–2270. doi: 10.1161/01.STR.27.12.2262
Lyall, D., Cox, S., Lyall, L., Celis-Morales, C., Cullen, B., and Mackay, D. F. (2020). Association between APOE e4 and white matter hyperintensity volume, but not total brain volume or white matter integrity. Brain Imaging Behav. 14, 1468–1476. doi: 10.1007/s11682-019-00069-9
Lyall, D., Munoz Maniega, S., Harris, S., Murray, C., and Lutz, M. W. (2015). APOE/TOMM 40 genetic loci, white matter hyperintensities, and cerebral microbleeds. Int. J. Stroke 10, 1297–1300. doi: 10.1111/ijs.12615
Mahley, R. W. (1988). Apolipoprotein E: cholesterol transport protein with expanding role in cell biology. Science 240, 622–630. doi: 10.1126/science.3283935
Mirza, S., Saeed, U., Knight, J. D., Stuss, D. T., and Keith, J. (2019). APOE ε4, white matter hyperintensities, and cognition in Alzheimer and Lewy body dementia. Neurology 93, e1807–e1819. doi: 10.1212/WNL.0000000000008377
Mortamais, M., Reynes, C., Brickman, A. M., Provenzano, F. A., Muraskin, J., and Portet, F. (2013). Spatial distribution of cerebral white matter lesions predicts progression to mild cognitive impairment and dementia. PLoS One 8:e56972. doi: 10.1371/journal.pone.0056972
Niemi, M., Hakkinen, T., Karttunen, T., Eskelinen, S., Kervinen, K., Savolainen, M., et al. (2002). Apolipoprotein E and colon cancer: expression in normal and malignant human intestine and effect on cultured human colonic adenocarcinoma cells. Eur. J. Intern. Med. 13, 37–43. doi: 10.1016/S0953-6205(01)00191-1
Operto, G., Cacciaglia, R., Grau-Rivera, O., and Falcon, C. (2018). White matter microstructure is altered in cognitively normal middle-aged APOE-ε4 homozygotes. Alzheimer’s Res. Ther. 10:48. doi: 10.1186/s13195-018-0375-x
Pantoni, L. (2010). Cerebral small vessel disease: from pathogenesis and clinical characteristics to therapeutic challenges. Lancet Neurol. 9, 689–701. doi: 10.1016/S1474-4422(10)70104-6
Persson, J., Larsson, A., Ingvar, M., Cruts, M., Van Broeckhoven, C., Adolfson, R., et al. (2006). Altered brain white matter integrity in healthy carriers of the APOE ε4 allele. a risk for AD? Neurology 66, 1029–1033. doi: 10.1212/01.wnl.0000204180.25361.48
Pietroboni, A., Colombi, A., Carandini, T., Scarpini, E., Galimbarti, D., and Bozzali, M. (2020). The role of Amyloid-β in white matter damage: possible common pathogenetic mechanisms in neurodegenerative and demyelinating diseases. J. Alzjeimers Dis. 78, 13–22. doi: 10.3233/JAD-200868
Pires, M., and Rego, A. (2023). Apoe4 and Alzheimer’s disease pathogenesis—mitochondrial deregulation and targeted therapeutic strategies. Int. J. Mol. Sci. 24:778. doi: 10.3390/ijms24010778
Prins, N., and Scheltens, P. (2015). White matter hyperintensities, cognitive impairment and dementia: an update. Nat. Rev. Neurosci. 11, 157–165. doi: 10.1038/nrneurol.2015.10
Promjunyakul, N., Dodge, H., Lahna, D., Boespflug, E., Kaye, J., Rooney, W., et al. (2018). Baseline NAWM structural integrity and CBF predict periventricular WMH expansion over time. Neurology 90, e2119–e2126. doi: 10.1212/WNL.0000000000005684
Promjunyakul, N., Lahna, D., Kaye, J., Dodge, H., Erten-Lyons, D., Rooney, W., et al. (2015). Characterizing the white matter hyperintensity penumbra with cerebral blood flow measures. Neuroimage Clin. 8, 224–229. doi: 10.1016/j.nicl.2015.04.012
Raber, J., Akana, S. F., Bhatnagar, S., Dallman, M. F., Wong, D., and Mucke, L. (2000). Hypothalamic-pituitary-adrenal function in Apoe–/– mice: possible role in behavioral and metabolic alterations. J. Neurosci. 20, 2064–2071. doi: 10.1523/JNEUROSCI.20-05-02064.2000
Rojas, S., Brugualat-Serrat, A., Gispter, J., Minguillón, C., and Tucholka, A. (2017). Higher prevalence of cerebral white matter hyperintensities in homozygous APOE-?4 allele carriers aged 45–75: results from the ALFA study. J. Cereb Blood Flow Metab. 38, 250–261. doi: 10.1177/0271678X17707397
Ryan, L., Walther, K., Bendin, B., Lue, L.-F., Walker, D., and Glisky, E. (2011). Age-related differences in white matter integrity and cognitive function are related to APOE status. NeuroImage 54, 1565–1577. doi: 10.1016/j.neuroimage.2010.08.052
Saleh, R., West, A., Ostermann, A., Schebb, N., Calder, P., and Minihane, A. (2021). APOE genotype modifies the plasma oxylipin response to Omega-3 polyunsaturated fatty acid supplementation in healthy individuals. Front. Nutr. 8:723813. doi: 10.3389/fnut.2021.723813
Santos-Ferreira, C., Baptista, R., Olivieira-Santos, M., Costa, R., Pereira Moura, J., and Goncalves, L. (2019). Apolipoprotein E2 genotype is associated with a 2-fold increase in the incidence of Type 2 diabetes mellitus: results from a long-term observational study. J. Lipids 2019:1698610. doi: 10.1155/2019/1698610
Schilling, S., Destefano, A., Sachdev, P., Choi, S., Mather, K. A., and DeCarli, C. D. (2013). APOE genotype and MRI markers of cerebrovascular disease: systematic review and meta-analysis. Neurology 81, 292–300. doi: 10.1212/WNL.0b013e31829bfda4
Schoemaker, D., Zanon Zotin, M., Chen, K., Igwe, K., Igwe, K. C., and Vila-Castelar, C. (2022). White matter hyperintensities are a prominent feature of autosomal dominant Alzheimer’s disease that emerge prior to dementia. Alzheimer’s Res Ther. 14:89. doi: 10.1186/s13195-022-01030-7
Sevigny, J., Ch’iao, P., Bussierre, R., Weinreb, P., Williams, L., Williams, L., et al. (2016). The antibody aducanumab reduces Aβ plaques in Alzheimer’s disease. Nature 537, 50–56. doi: 10.1038/nature19323
Sharms, M., Shams, S., Martola, J., and Cavallin, L. (2022). MRI markers of small vessel disease and the APOE allele in cognitive impairment. Front. Aging Neurosci. 14:897674. doi: 10.3389/fnagi.2022.897674
Shinto, L., Raber, J., Mishra, A., Roese, N., and Silbert, L. (2022). A review of oxylipins in Alzheimer’s disease and related dementias (ADRD): potential therapeutic targets for the modulation of vascular tone and inflammation. Metabolites 12:826. doi: 10.3390/metabo12090826
Silbert, L., Wall, R., Lahna, D., Dodge, H., Hagen, K., David, J., et al. (2020). Docosahexaenoic acid oxylipins are associated with white matter microstructural integrity in a cohort of cognitively normal, hypertensive young-old. Alzheimer Dement. 16:e044965. doi: 10.1002/alz.044965
Slattery, C., Zhang, J., Paterson, R., Carton, A., and Macpherson, K. (2017). ApoE influences regional white-matter axonal density loss in Alzheimer’s disease. Neurobiol. Aging 57, 8–17. doi: 10.1016/j.neurobiolaging.2017.04.021
Sperling, R., Aisen, P., Beckett, L., Bennett, D., Craft, S., Craft, S., et al. (2011). Toward defining the preclinical stages of Alzheimer’s disease: recommendations from the national institute on aging-Alzheimer’s association workgroups on diagnostic guidelines for Alzheimer’s disease. Alzheimer Dement. 7, 280–292. doi: 10.1016/j.jalz.2011.03.003
Strain, J., Smith, R., Beaumont, H., Roe, C., Gordon, B., Mishra, S., et al. (2018). Loss of white matter integrity reflects tau accumulation in Alzheimer disease defined regions. Neurology 91, e313–e318. doi: 10.1212/WNL.0000000000005864
Sudre, C., Cardoso, M., Frost, C., Barkhof, F., and Fox, N. (2017). APOE ε4 status is associated with white matter hyperintensities volume accumulation rate independent of AD diagnosis. Neurobiol. Aging 53, 65–75. doi: 10.1016/j.neurobiolaging.2017.01.014
Sullivan, P. M., Mezdour, H., Aratani, Y., Knouff, C., Najib, J., Reddick, R. L., et al. (1997). Targeted replacement of the mouse apolipoprotein E gene with the common human APOE3 allele enhances diet-induced hypercholesterolemia and atherosclerosis. J. Biol. Chem. 272, 17972–17980. doi: 10.1074/jbc.272.29.17972
Testa, G., Staurenghi, E., Zerbinati, C., Gargiulo, S., Iuliano, L., and Giaccone, G. (2016). Changes in brain oxysterols at different stages of Alzheimer’s disease: their involvement in neuroinflammation. Redox Biol. 10, 24–33. doi: 10.1016/j.redox.2016.09.001
Torres, E., Luo, J., Boehnlein, J., Towns, D., Kinzie, D., Debarber, A., et al. (2022). Apolipoprotein E isoform-specific changes related to stress and trauma exposure. Transl. Psychiatry 12:125. doi: 10.1038/s41398-022-01848-7
Tran, T., Corsini, S., Kellinggray, L., Hegarty, C., Le Gail, C., Narbad, A., et al. (2019). APOE genotype influences the gut microbiome structure and function in humans and mice: relevance for Alzheimer’s disease pathophysiology. FASEB J. 33, 8221–8231. doi: 10.1096/fj.201900071R
Turney, I., Chesebro, A., Renteria, M., Lao, P., Beato, J., Schupf, N., et al. (2020). APOE ε4 and resting-state functional connectivity in racially/ethnically diverse older adults. Alzheimer Dement. 12:e12094. doi: 10.1002/dad2.12094
van Dijk, E., Prins, N., Vrooman, H., Hofman, A., Koudstaal, P., and Breteler, M. (2008). Progression of cerebral small vessel disease in relation to risk factors and cognitive consequences. rotterdam scan study. Stroke 39, 2712–2719. doi: 10.1161/STROKEAHA.107.513176
van Dyck, C., Swanson, C., Aisen, P., Bateman, R., Chen, C., and Gee, M. (2023). Lecanemab in early Alzheimer’s disease. N. Engl. J. Med. 388, 9–21. doi: 10.1056/NEJMoa2212948
Vettore, M., De Marco, M., Pallucca, C., Bendini, M., Gallucci, M., and Venneri, A. (2021). White-matter hyperintensity load and differences in resting-state network connectivity based on mild cognitive impairment subtype. Front. Aging Neurosci. 13:737359. doi: 10.3389/fnagi.2021.737359
Westlye, L., Reinvang, I., Rootwelt, H., and Espeseth, T. (2012). Effects of APOE on brain white matter microstructure in healthy adults. Neurology 79, 1961–1969. doi: 10.1212/WNL.0b013e3182735c9c
Wisdom, N., Callahan, J., and Hawkins, K. (2011). The effects of apolipoprotein E on non-impaired cognitive functioning: a meta-analysis. Neurobiol. Aging 32, 63–74. doi: 10.1016/j.neurobiolaging.2009.02.003
Keywords: white matter hyperintensity, white matter integrity, apolipoprotein E, magnetic resonance imaging (MRI), cognition
Citation: Raber J and Silbert LC (2023) Role of white matter hyperintensity in effects of apolipoprotein E on cognitive injury. Front. Hum. Neurosci. 17:1176690. doi: 10.3389/fnhum.2023.1176690
Received: 01 March 2023; Accepted: 28 April 2023;
Published: 19 May 2023.
Edited by:
Björn H. Schott, Leibniz Institute for Neurobiology (LG), GermanyReviewed by:
Eric Goldwaser, NewYork-Presbyterian, United StatesCopyright © 2023 Raber and Silbert. This is an open-access article distributed under the terms of the Creative Commons Attribution License (CC BY). The use, distribution or reproduction in other forums is permitted, provided the original author(s) and the copyright owner(s) are credited and that the original publication in this journal is cited, in accordance with accepted academic practice. No use, distribution or reproduction is permitted which does not comply with these terms.
*Correspondence: Jacob Raber, raberj@ohsu.edu