- 1Department of Rehabilitation Medicine, Nagoya City University Graduate School of Medical Sciences, Nagoya, Japan
- 2Department of Neurosurgery, Nagoya City University Graduate School of Medical Sciences, Nagoya, Japan
- 3Department of Neurology and Neuroscience, Nagoya City University Graduate School of Medical Sciences, Nagoya, Japan
- 4Hitachi, Ltd., Research and Development Group, Center for Exploratory Research, Tokyo, Japan
Background: The Finger Tapping (F-T) test is useful for assessing motor function of the upper limbs in patients with idiopathic normal pressure hydrocephalus (iNPH). However, quantitative evaluation of F-T for iNPH has not yet been established. The purpose of this study was to investigate the usefulness of the quantitative F-T test and optimal measurement conditions as a motor evaluation and screening test for iNPH.
Methods: Sixteen age-matched healthy controls (mean age 73 ± 5 years; 7/16 male) and fifteen participants with a diagnosis of definitive iNPH (mean age 76 ± 5 years; 8/15 male) completed the study (mean ± standard deviation). F-T performance of the index finger and thumb was quantified using a magnetic sensing device. The performance of repetitive F-T by participants was recorded in both not timing-regulated and timing-regulated conditions. The mean value of the maximum amplitude of F-T was defined as M-Amplitude, and the mean value of the maximum velocity of closure of F-T was defined as cl-Velocity.
Results: Finger Tapping in the iNPH group, with or without timing control, showed a decrease in M-Amplitude and cl-Velocity compared to the control group. We found the only paced F-T with 2.0 Hz auditory stimuli was found to improve both M-Amplitude and cl-Velocity after shunt surgery.
Conclusion: The quantitative assessment of F-T with auditory stimuli at the rate of 2.0 Hz may be a useful and potentially supplemental screening method for motor assessment in patients with iNPH.
Highlights
- Patients with idiopathic normal pressure hydrocephalus (iNPH) have not only the lower limb dysfunction but also the upper limb.
- This is the first report of quantitative evaluation of finger tapping (F-T) movements in patients with iNPH under multiple conditions.
- The results showed particularly that patients had impaired hand dexterity compared to controls, and cut-off values were calculated.
- Quantitative assessment of F-T with 2.0 Hz auditory stimulation may be a useful and complementary screening method for motor assessment of patients with iNPH.
Introduction
Normal pressure hydrocephalus (NPH) was first described in 1965 by Hakim and Adams as a syndrome accompanied by a triad of progressive symptoms: gait disturbance, cognitive impairments and urinary incontinence (Adams et al., 1965). The assessment of gait is fundamental to diagnose idiopathic NPH (iNPH), because gait disturbance is typically the first clinical symptom (Ojemann et al., 1969). The assessment of gait after drainage is very important for predicting functional improvement after shunt surgery (Williams et al., 2008), but it is sometimes difficult if patients have heavily deteriorated motor function. However, gait assessment in iNPH is sometimes difficult because the gait is affected by various factors such as orthopedic diseases, motor weakness and psychiatric apathy (Kito et al., 2009; Allali et al., 2017). The CSF tap test, predictive of post-shunt improvement, involves repeated assessments of motor and cognitive function. Therefore, the ideal supplemental functional evaluation of the tap test should be as reproducible as possible and cause as little physical and mental to put burden on the patient as possible, such as falls.
The pattern of gait disturbance in NPH is often described as lower-body parkinsonism according to the guidelines for diagnosis (Espay et al., 2008). Kang et al. (2016) reported that parkinsonism of iNPH is present not only in the lower limbs but also the upper limbs. Previous studies reported that upper limb dysfunction in patients with iNPH was similar to that of bradykinesia symptoms in Parkinson’s disease (PD) (Krauss et al., 1997; Nowak et al., 2006). Repetitive index finger on thumb tapping is considered an appropriate evaluation of bradykinesia because upper limb impairments in PD are reported to be more apparent distally than proximally (Agostino et al., 1998; Nowak and Topka, 2006). Stegemöller et al. (2017) explained that repetitive movements in Parkinsonism are characterized by reductions in amplitude (hypokinesia), velocity (bradykinesia), and rhythmicity, with or without hesitations and arrests. Several previous studies have reported that patients with iNPH had upper limb dysfunction (Sørensen et al., 1986; Nowak and Topka, 2006; Tsakanikas et al., 2009; Hellström et al., 2012), and some found finger tapping (F-T) to be particularly useful in assessing motor function of iNPH (Lenfeldt et al., 2008; Liouta et al., 2017; Behrens et al., 2019). The assessment of motor function of the upper limbs might be helpful in diagnosing NPH to avoid falls and difficulties in gait assessment.
Although the usefulness of F-T test has widely been accepted, its quantitative evaluation for iNPH has not yet been established. Notably, previous studies reported that impairments in simple repetitive finger movements was observed at rates near and above 2.0 Hz in patients with PD (Pastor et al., 1992; Stegemöller et al., 2009). Based on these reports, the present study used 1.0 and 2.0 Hz auditory stimuli in the timing-regulated condition and spontaneous motor tempo (SMT) and maximum effort (as quickly and as big as possible) in the not timing-regulated condition (Christopher et al., 2008; Dawn et al., 2020).
This is the first report evaluating repetitive F-T of iNPH patients quantitatively with multiple conditions. The purpose of this study was to find the optimal conditions for quantitative evaluation of repetitive F-T to help assess the function of iNPH patients.
Materials and methods
Subjects
Sixteen age-matched healthy controls (mean age 73 ± 5 years; 7/16 male) and 15 participants with a diagnosis of definitive iNPH (mean age 76 ± 5 years; 8/15 male) completed the study (mean ± standard deviation). After shunt surgery, the clinical symptoms of patients with iNPH improved significantly and they received a definitive diagnosis of iNPH. All participants were right-handed and completed the Edinburgh Handedness Inventory and Mini-Mental State Examination Japanese (MMSE-J). We excluded participants with conditions such as severe hearing loss or other diseases that could interfere significantly with the test.
We used the iNPH Grading Scale (iNPHGS) to evaluate the triad of disease severity (Kubo et al., 2008). The iNPHGS consists of three domains: gait disturbance, cognitive impairment and urinary incontinence, each of which is scored according to five levels (0 to 4, respectively).
The walking ability of all patients was also measured for the severity of symptoms by the Timed Up and Go Test (TUG), which is accepted as a valid test to assess motor function (Podsiadlo and Richardson, 1991). The study protocol was approved as prospective study by the Nagoya City University Institutional Review Board, which is compliant with the guidelines in the Declaration of Helsinki. Informed consent was obtained for all participants (patients and controls) (ID: 60-18-0213).
Parameters of F-T
Finger-tapping performance of the paced index finger and thumb was quantified using a magnetic sensing device (UB-1, HITACHI Ltd., Tokyo, Japan) (Figure 1). Participants tapped their index finger on the thumb while wearing magnetic sensors. The device can automatically detect and record the amplitude, velocity and interval of each a finger tap. Since a previous study described repetitive movements in parkinsonism as being characterized by reductions in amplitude, velocity and rhythmicity (Stegemöller et al., 2017), we chose these parameters for evaluation of F-T in patients with iNPH. The mean of the maximum amplitude was defined as M-Amplitude, and the mean of the maximum velocity of the closed motion was defined as cl-Velocity. The variability was calculated as the coefficient of variation (CV) of each parameter, which were M-Amplitude, cl-Velocity, and tapping interval.
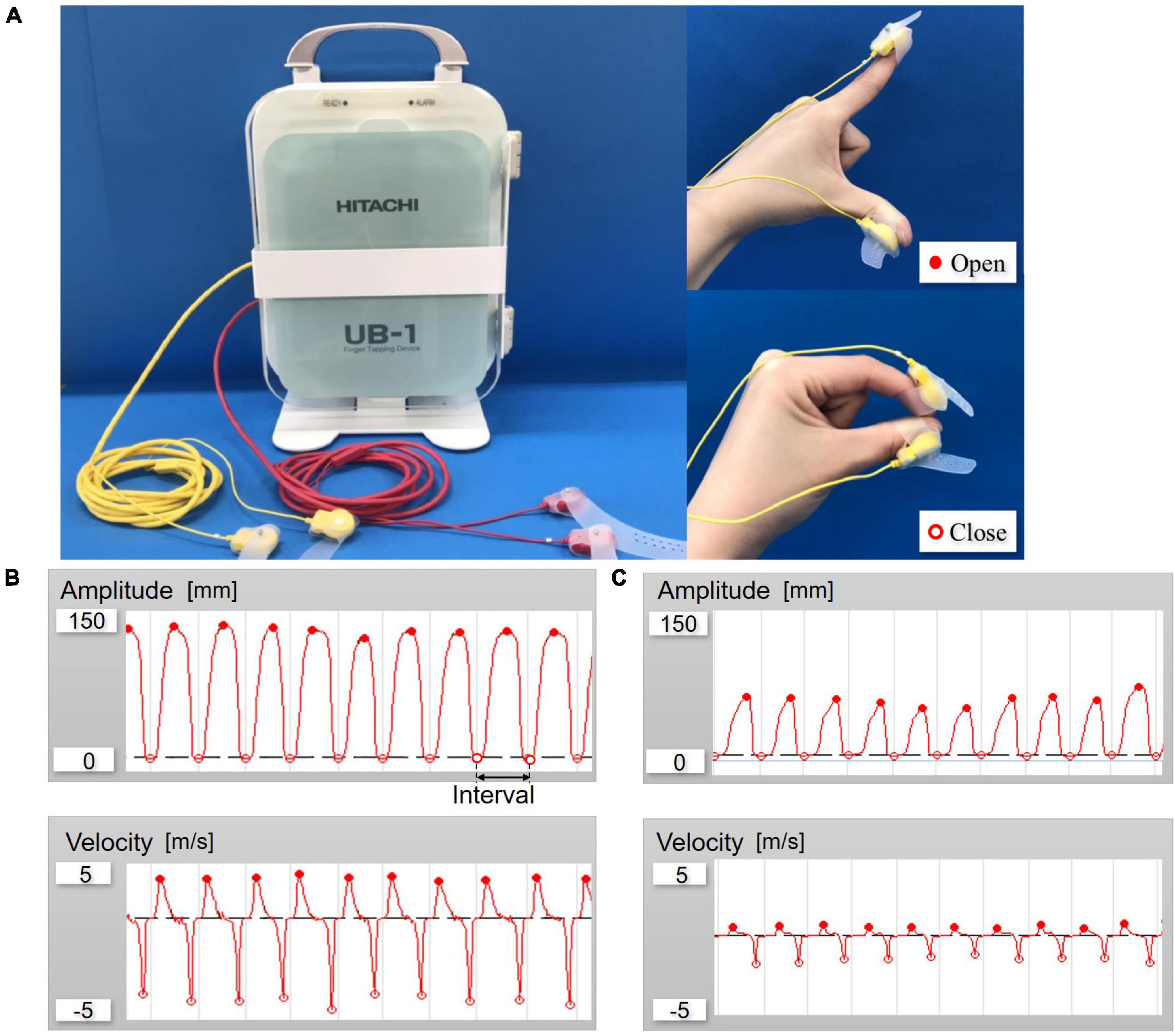
Figure 1. (A) Overall view of UB-1; Finger-tapping system with magnetic sensors and finger tap. (B) Example of waveforms control. (C) Example of waveforms patient with idiopathic normal pressure hydrocephalus (iNPH) (amplitude and velocity).
Recording of F-T
First, the maximum distance from index finger to thumb at rest was measured by each participant and defined as r-Amplitude as one of the calibrations before the F-T evaluation. We then recorded the performance of repetitive F-T both not timing-regulated and timing-regulated conditions by participants for 15 s each of the non-dominant and the dominant hand. F-T test was performed in the order of at a spontaneous pace, at rates of 1.0 and 2.0 Hz by auditory stimulation with a metronome, and at the maximum effort pace (as quickly and as big as possible). Participants were instructed to close their fingers together when auditory stimuli were given. Furthermore, the patients were evaluated for F-T before and after shunt surgery.
Statistics
We computed the sample size according to the of F-T test we considered most relevant in our investigation, finger oscillation test during which recorded the speed of tapping (Reitan and Wolfson, 1993). We calculated the effect size from the mean and standard deviation of the F-T speeds of controls and iNPH according to Lenfeldt et al. (2008), and be aimed to detect a change of more than 30% of it. To detect this decline with a two-tailed type I error of 0.05 and a power of 80%, the estimated sample size was 20 persons (10 persons in each group). In order to account for the possibility of some patients not completing the study, we aimed to enroll 15 subjects in each group. We used a two-way repeated measures analysis of variance (ANOVA) to compare on all parameters of the F-T test, with non-dominant and dominant hand as the within factor and disease as the between factor. We used a paired t-test for M-Amplitude and cl-Velocity, and Wilcoxon signed-rank test for CV to compare the parameters of the F-T test in patients before and after surgery with the mean values of the dominant and non-dominant hand, except for CV of cl-Velocity. Since the normality of M-Amplitude and cl-Velocity was confirmed by the Shapiro-Wilk test, the cut-off thresholds for the parameter of paced F-T were calculated from ROC analyses, using the area under the curve (AUC) and determined to a 95% confidence interval (CI). The Pearson correlation coefficient was calculated for continuous variables between the parameters of F-T test and other function in patients with iNPH. The results were expressed as mean ± standard deviation of the mean. Statistical analysis was completed with Statistical software JMP®10.0.2 (Statistical Analysis Software, SAS Institute Inc., Charlotte, NC, USA). p-values less than 0.05 were considered as statistically significant.
Results
Clinical characteristics
Characteristics of participants are provided in Table 1. There were no significant differences in age and gender between groups. However, MMSE-J scores were significantly lower in patients with iNPH as compared to healthy controls (P < 0.001). The mean iNPHGS of gait impairment scores improved from 2.00 ± 0.65 to 1.20 ± 1.10 (P < 0.01) and the mean iNPHGS of urination incontinence scores from1.46 ± 0.83 to 1.10 ± 0.90 (P < 0.05) after shunt surgery, respectively. The mean iNPHGS for cognitive impairment scores showed a tendency for improvement, from1.93 ± 0.80 to 1.80 ± 1.00 (P = 0.08).
F-T performance
The comparison of paced F-T performance between controls and patients is shown in Table 2.
The r-Amplitudes in both dominant and non-dominant hands were not significantly different between groups. In controls, r-Amplitude was 131.44 ± 13.42 mm (non-dominant hand)/123.91 ± 13.79 mm (dominant hand). In iNPH patients, the r-Amplitude was 137.20 ± 33.43 mm (non-dominant hand)/127.74 ± 20.63 mm (dominant hand). In frequency of taps, only the SMT condition showed significant difference between groups and maximum effort condition showed no significant difference by detected in the ANOVA. CVs of cl-Velocity in the dominant and non-dominant hands were significantly different for both controls and patients with iNPH, with the exception of the 1.0 Hz condition. There was no interaction effect (group × the dominant hand) observed in any parameter. There were significant differences between groups on almost all parameters of F-T. Finger tapping movements of the iNPH group were detected as being significantly impaired in amplitude and speed compared to the control group.
The change of F-T performance by shunt surgery
The comparison of paced F-T performance before and after shunt surgery in patients with iNPH is shown in Figure 2.
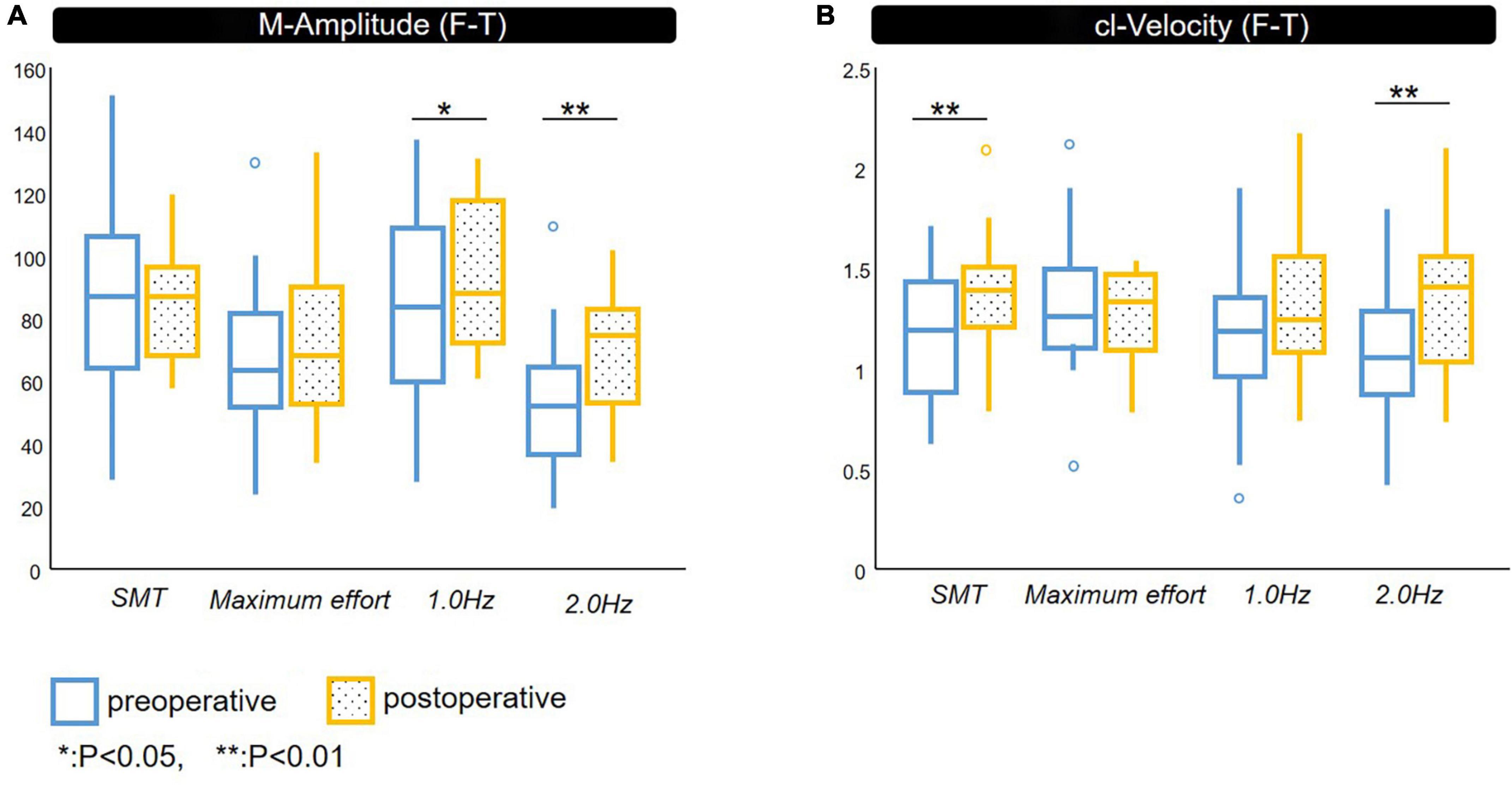
Figure 2. Spatiotemporal parameters of F-T. Comparison between pre- and post-shunt surgery with definite iNPH. (A) M-Amplitude of finger tapping, (B) cl-Velocity of finger tapping. preoperative, postoperative. iNPH, idiopathic normal pressure hydrocephalus; M-Amplitude, the mean of maximum amplitude; cl-Velocity, the line represents median values; *p < 0.05, **p < 0.01.
The M-Amplitude of F-T under 1.0 and 2.0 Hz conditions was significantly improved after shunt surgery.
The cl-Velocity of F-T showed significant improvement under SMT and 2.0 Hz conditions.
We did not identify significant improvement in CV after shunt surgery except for that of cl-Velocity of the non-dominant hand under 2.0 Hz condition.
ROC analysis
For discrimination between iNPH and normal controls, the optimal cut-off levels of the F-T parameters under 2.0 Hz auditory stimuli conditions were determined using ROC analyses (Table 3). The optical cut-off of M-Amplitude was 56.4 mm, with 73% sensitivity and 94% specificity in the non-dominant hand and 57.1 mm, with 60% sensitivity and 100% specificity in the dominant hand, respectively.
The cut-off score obtained in cl-Velocity was 1.20 m/s, with 73% sensitivity and 38% specificity in the non-dominant hand and 1.44 m/s, with 87% sensitivity and 75% specificity in the dominant hand, respectively. The M-Amplitude of the optimal cut-off value was 41.1% (non-dominant hand)/44.7% (dominant hand) in patients with iNPH, as compared to r-Amplitude.
Correlations between the parameters of F-T test and conventional assessment in patients with iNPH
The correlations between F-T parameters and MMSE-J and TUG under 1.0 and 2.0 Hz conditions are shown in Figure 3. Under the 1.0 Hz condition, both of the M-Amplitude and cl-Velocity correlated with TUG (Pearson’s correlation coefficient −0.65; P < 0.01 and −0.72; P < 0.01) in the non-dominant hand.
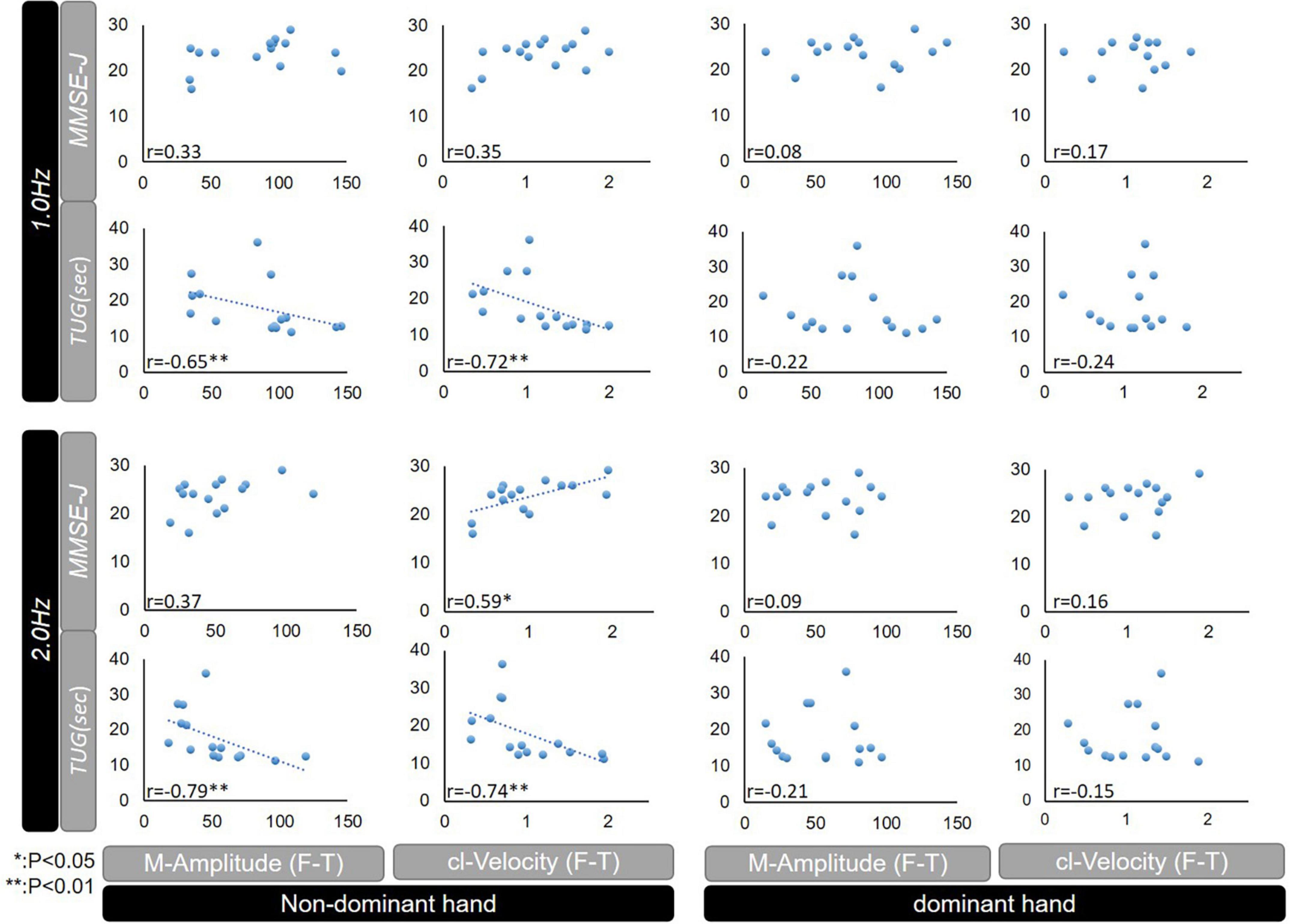
Figure 3. Correlations between MMSE-J or TUG, and F-T parameters (M-Amplitude or cl-Velocity) among patients with iNPH. MMSE-J, Mini Mental State Examination-Japanese; TUG, Timed up and go test; F-T, finger tapping; M-Amplitude, the mean of maximum amplitude; cl-Velocity, the maximum of closing movement velocity; iNPH, idiopathic normal pressure hydrocephalus; CV, coefficient of variation; NS, not significant; *p < 0.05, **p < 0.01.
Under the 2.0 Hz condition, both of the M-Amplitude and cl-Velocity correlated with TUG (Pearson’s correlation coefficient −0.79; P < 0.01 and −0.74; P < 0.01), and cl-Velocity was further correlated with MMSE-J (Pearson’s correlation coefficient 0.59; P < 0.05) in the non-dominant hand.
Discussion
The goal of this study was to investigate the optimal conditions for quantitative evaluation of repetitive F-T to help assess the function of iNPH patients. We evaluated repetitive simple F-T quantitatively in a control group and patients with iNPH. There are two main findings. First, the present study revealed that repetitive simple F-T was impaired in patients with iNPH compared to controls under the both not timing-regulated and timing-regulated conditions. Second, only the timing-regulated 2.0 Hz condition, post-operative improvement of the F-T test for iNPH was detected in this study for both M-Amplitude and cl-Velocity.
Regarding previous reports evaluating hand dexterity in iNPH, various methods including finger tapping have been reported. Lenfeldt et al. (2008) assessed manual dexterity by counting the total number of taps using sequential movement of four fingers (from the index to the little finger or reversed) and confirmed the improvement in the right hand after cerebrospinal fluid (CSF) drainage. Liouta et al. (2017) measured F-T speed according to the Halstead-Reitan neuropsychological test battery which uses a specially adapted manual tapper for the index finger and scored the number of taps (Reitan and Wolfson, 1993). They reported that the fine motor function of the right hand in iNPH improved after the lumbar puncture tap test. Behrens showed impairment of manual dexterity in iNPH using F-T, which improved after shunt surgery. The examiner assessed manual dexterity using four fingers on a small keyboard with digits to tap the digits in order and scored the correct taps (Behrens et al., 2014, 2019).
Based on these findings, all of these reports demonstrated the usefulness of the F-T test to assess motor function in iNPH. However, while these studies merely counted the number of finger taps, this study allowed us to measure F-T minutely and quantitatively by measuring the amplitude, velocity and other parameters in the present study.
Our rationale for choosing the 2.0 Hz rather than 1.0 Hz as the pacing frequency for timing-regulated of F-T is as follows. In behavioral and electrophysiological studies, the rate of about 2.0 Hz was shown to be a noteworthy frequency, and Mayville et al. (1999) provided evidence that a frequency of about 2.0 Hz is the band in which there is a shift from discrete to continuous control in repetitive finger movement with pacing stimuli (Toma et al., 2002). As Stegemöller et al. (2017) reported that in repetitive movements at frequencies slower than 2.0 Hz (e.g., 1.0 Hz), which are perceived as discrete movements, “control of discrete movements is considered to be preferentially mediated by cerebellar-premotor pathways,” while in repetitive movements at frequencies of about 2.0 Hz, which are perceived as continuous movements, “the control of continuous movement is mediated by the basal ganglia thalamocortical pathways.”
Based on these findings, the disturbance of repetitive movements at around 2.0 Hz, which are perceived as controlling continuous movements, reflects impairment from a dysfunction of the basal ganglia-thalamocortical network in PD (Ivry, 1996; Ivry and Spencer, 2004; Spencer and Ivry, 2005; Grahn and Brett, 2009; Stegemöller et al., 2017). Several reports have explained the implications of 2.0 Hz paced F-T in PD. Nowak et al. (2006) reported that fMRI findings suggest impairments of F-T in patients with PD reflect potential impairment in the supplementary motor area (SMA) (Nowak and Topka, 2006). Lenfeldt et al. (2008) suggested that the improvement of manual dexterity after CSF drainage in iNPH was related to the activation of SMA using fMRI. PD shares many characteristics with disturbances of iNPH mechanisms in the SMA related to frontal periventricular cortico-basal ganglia-thalamus-cortical pathways, which are connected with the impairment of finger movements with paced stimuli (Roland et al., 1980; Contreras-Vidal and Stelmach, 1995; Nowak and Topka, 2006; Witt et al., 2008; Genon et al., 2017; Liouta et al., 2017).
Previous studies have reported that the method of stimulus presentation is another important measurement condition. Witt et al. (2008) reported that all F-T tasks with pacing stimuli are commonly activated in the primary sensorimotor cortex, SMA, and anterior cerebellum by conjunction analysis, and especially, that the concordance within the dorsal premotor cortex (PMd) was lateralized based on task variation, with the auditorily-paced task in the right PMd. The right dorsal PMd is preferentially related to hand/finger movements and connected to both cognitive and motor regions in sequencing and rhythm-processing aspects common to finger movements, music, and language (Genon et al., 2017; Stegemöller et al., 2017). Therefore, using auditory paced F-T at the rate of 2.0 Hz to assess parkinsonism could be beneficial, because it is likely to provide high detectability of impairment.
In addition, the advantages of F-T may include the following. Paced F-T is easy for patients to perform in any position, sitting or lying down. Dawn et al. (2020) report that the timing-regulated condition is less susceptible to momentary affective states than the not timing-regulated condition, suggesting that it may be more appropriate to compare the performance of same subjects. According to Abe et al. (2017) hand dexterity may assess not only motor function but also cognitive function, and cross-sectional and longitudinal studies have shown that assessment of manual dexterity using pegs correlates with cognitive function in a healthy elderly. These reports suggest that the pacing F-T is a simple assessment, but that it may be used to assess not only motor function but also cognitive function.
There was another finding in this study, it was observed that CV of cl-Velocity in the repetitive F-T test differed between non-dominant and dominant hands. Previous imaging studies have reported that a hemispheric asymmetry in the functional activation of the motor cortex during contralateral and ipsilateral finger movements, especially in right-handed (Kim et al., 1993; Hayashi et al., 2008). Hayashi et al. (2008) reported that increase of contralateral activation and ipsilateral deactivation during the right-hand movement at high-frequency because the right-handed have the dominance of the left primary motor cortex. These studies suggest that the influence of non-dominant and dominant hand in right-handed should be taken into account in quantitative assessment of dexterous movements such as F-T test.
There are other barriers to the clinical application of this study. Quantitative evaluation of fingers is useful, but not as common as gait analysis. Previous studies reported quantitative evaluation of fingers using a 3D motion analyzer, which may be a potential alternative method (Horiba et al., 2019).
The limitation of our study is the small number of cases, further investigation is needed. Considering the possibility of applying it to tap tests and disease screening, the cut-off value obtained may be an indicator of the severity of impairment of hand dexterity in patients with NPH, which is an advantage of quantitative evaluation of F-T. Further studies with a larger number of subjects are needed to confirm the reproducibility of the repetitive 2.0 Hz F-T cut-off value and validate its usefulness as an indicator of motor impairment in iNPH. It may prove useful in revealing the features of upper limb parkinsonism in patients with iNPH in more detailed studies of finger tapping for differential clinical diagnosis.
Conclusion
Quantitative assessment of F-T with 2.0 Hz auditory stimulation may be a useful and potentially supplemental screening method for motor assessment in patients with iNPH.
Data availability statement
The raw data supporting the conclusions of this article will be made available by the authors, without undue reservation.
Ethics statement
The studies involving human participants were reviewed and approved by Nagoya City University. The patients/participants provided their written informed consent to participate in this study.
Author contributions
YS, MT, and MH conceived the idea of the study. YS, KS, and MH carried out the experiments. SK developed the statistical analysis plan and conducted statistical analyses. AK contributed to the interpretation of the results. TY and YN provided critical feedback and helped shape the research and analysis. YS drafted the original manuscript. MM, YU, and NM supervised the conduct of this study. All authors reviewed the manuscript draft and revised it critically on intellectual content and approved the final version of the manuscript to be published.
Conflict of interest
AK was employed by Hitachi, Ltd.
The remaining authors declare that the research was conducted in the absence of any commercial or financial relationships that could be construed as a potential conflict of interest.
Publisher’s note
All claims expressed in this article are solely those of the authors and do not necessarily represent those of their affiliated organizations, or those of the publisher, the editors and the reviewers. Any product that may be evaluated in this article, or claim that may be made by its manufacturer, is not guaranteed or endorsed by the publisher.
Abbreviations
iNPH, idiopathic normal pressure hydrocephalus; F-T, finger tapping; PD, Parkinson’s disease; SMT, spontaneous motor tempo; MMSE-J, Mini-Mental State Examination Japanese; iNPHGS, idiopathic normal pressure hydrocephalus Grading Scale; TUG, Time Up and Go Test; CV, coefficient of variation; AUC, area under the curve; CI, confidence interval; SD, standard deviation; ANOVA, analysis of variance; CSF, cerebrospinal fluid; SMA, supplementary motor area; PMd, dorsal premotor cortex; NS, not significant; M-Amplitude, the mean of maximum amplitude; cl-Velocity, the mean of maximum of closing movement velocity.
References
Abe, T., Soma, Y., and Kitano, N. (2017). Change in hand dexterity and habitual gait speed reflects cognitive decline over time in healthy older adults: a longitudinal study. J. Phys. Ther. Sci. 29, 1737–1741. doi: 10.1589/jpts.29.1737
Adams, R., Fisher, C., Hakim, S., Ojemann, R., and Sweet, W. (1965). Symptomatic occult hydrocephalus with normal cerebrospinal-fluid pressure — a treatable syndrome. N. Engl. J. Med. 273, 117–126. doi: 10.1056/NEJM196507152730301
Agostino, R., Berardelli, A., Currà, A., Accornero, N., and Manfredi, M. (1998). Clinical impairment of sequential finger movements in Parkinson’s disease. Mov. Disord. 13, 418–421. doi: 10.1002/mds.870130308
Allali, G., Laidet, M., Armand, S., Saj, A., Krack, P., Assal, F., et al. (2017). Apathy and higher level of gait control in normal pressure hydrocephalus. Int. J. Psychophysiol. 119, 127–131. doi: 10.1016/j.ijpsycho.2016.12.002
Behrens, A., Eklund, A., Elgh, E., Smith, C., Williams, M., Malm, J., et al. (2014). A computerized neuropsychological test battery designed for idiopathic normal pressure hydrocephalus. Fluids Barriers CNS. 11:22. doi: 10.1186/2045-8118-11-22
Behrens, A., Elgh, E., Leijon, G., Kristensen, B., Eklund, A., Malm, J., et al. (2019). The computerized general neuropsychological INPH Test revealed improvement in idiopathic normal pressure hydrocephalus after shunt surgery. J. Neurosurg. 132, 733–740. doi: 10.3171/2018.10.JNS18701
Christopher, G., Barbara, C., and Stephanie, R. (2008). Movement disorder society-sponsored revision of the unified Parkinson’s disease rating scale (MDS-UPDRS): scale presentation and clinimetric testing results. Mov. Disord. 23, 2129–2170. doi: 10.1002/mds.22340
Contreras-Vidal, J., and Stelmach, G. (1995). A neural model of basal ganglia-thalamocortical relations in normal and parkinsonian movement. Biol Cybern. 73, 467–476. doi: 10.1007/BF00201481
Dawn, R., Daniel, J., and Peter, J. (2020). Comparison of spontaneous motor tempo during finger tapping, toe tapping and stepping on the spot in people with and without Parkinson’s disease. J. Mov. Disord. 13, 47–56. doi: 10.14802/jmd.19043
Espay, A., Narayan, R., Duker, A., Barrett, E. Jr., and de Courten-Myers, G. (2008). Lower-body parkinsonism: reconsidering the threshold for external lumbar drainage. Nat. Clin. Pract. Neurol. 4, 50–55. doi: 10.1038/ncpneuro0688
Genon, S., Li, H., Fan, L., Müller, V., Cieslik, E., Hoffstaedter, F., et al. (2017). The right dorsal premotor mosaic: organization, functions, and connectivity. Cereb. Cortex. 27, 2095–2110. doi: 10.1093/cercor/bhw065
Grahn, J., and Brett, M. (2009). Impairment of beat-based rhythm discrimination in Parkinson’s disease. Cortex. 45, 54–61. doi: 10.1016/j.cortex.2008.01.005
Hayashi, M., Saito, D., Aramaki, Y., Asai, T., Fujibayashi, Y., Sadato, N., et al. (2008). Hemispheric asymmetry of frequency-dependent suppression in the ipsilateral primary motor cortex during finger movement: a functional magnetic resonance imaging study. Cereb Cortex. 18, 2932–2940. doi: 10.1093/cercor/bhn053
Hellström, P., Klinge, P., Tans, J., and Wikkelsø, C. (2012). The neuropsychology of iNPH: findings and evaluation of tests in the European multicentre study. Clin. Neurol. Neurosurg. 114, 130–134. doi: 10.1016/j.clineuro.2011.09.014
Horiba, M., Ueki, Y., Nojima, I., Shimizu, Y., Sahashi, K., Itamoto, S., et al. (2019). Impaired motor skill acquisition using mirror visual feedback improved by transcranial direct current stimulation (tDCS) in patients with Parkinson’s disease. Front. Neurosci. 13:602. doi: 10.3389/fnins.2019.00602
Ivry, R. (1996). The representation of temporal information in perception and motor control. Curr. Opin. Neurobiol. 6, 851–857. doi: 10.1016/s0959-4388(96)80037-7
Ivry, R., and Spencer, R. (2004). The neural representation of time. Curr. Opin. Neurobiol. 14, 225–232. doi: 10.1016/j.conb.2004.03.013
Kang, K., Choi, D., and Lee, H. (2016). Unusual idiopathic normal pressure hydrocephalus patient with marked asymmetric and upper body parkinsonism. Ann. Indian Acad. Neurol. 19, 245–248. doi: 10.4103/0972-2327.160057
Kim, S., Ashe, J., Hendrich, K., Ellermann, J., Merkle, H., Uðurbil, K., et al. (1993). Functional magnetic resonance imaging of motor cortex: hemispheric asymmetry and handedness. Science 261, 615–617. doi: 10.1126/science.8342027
Kito, Y., Kazui, H., Kubo, Y., Yoshida, T., Takaya, M., Wada, T., et al. (2009). Neuropsychiatric symptoms in patients with idiopathic normal pressure hydrocephalus. Behav. Neurol. 21, 165–174. doi: 10.3233/BEN-2009-0233
Krauss, K., Regel, J., Droste, D., Orszagh, M., Borremans, J., Vach, W., et al. (1997). Movement disorders in adult hydrocephalus. Mov. Disord. 12, 53–60. doi: 10.1002/mds.870120110
Kubo, Y., Kazui, H., Yoshida, T., Kito, Y., Kimura, N., Tokunaga, H., et al. (2008). Validation of grading scale for evaluating symptoms of idiopathic normal-pressure hydrocephalus. Dement. Geriatr. Cogn. Disord. 25, 37–45. doi: 10.1159/000111149
Lenfeldt, N., Larsson, A., Nyberg, L., Andersson, M., Birgander, R., Eklund, A., et al. (2008). Idiopathic normal pressure hydrocephalus: increased supplementary motor activity accounts for improvement after CSF drainage. Brain. 131(Pt. 11), 2904–2912. doi: 10.1093/brain/awn232
Liouta, E., Gatzonis, S., Kalamatianos, T., Kalyvas, A., Koutsarnakis, C., Liakos, F., et al. (2017). Finger tapping and verbal fluency post-tap test improvement in INPH: its value in differential diagnosis and shunt-treatment outcomes prognosis. Acta Neurochir. 159, 2301–2307. doi: 10.1007/s00701-017-3301-2
Mayville, J., Bressler, S., Fuchs, A., and Kelso, J. (1999). Spatiotemporal reorganization of electrical activity in the human brain associated with a timing transition in rhythmic auditory-motor coordination. Exp. Brain Res. 127, 371–381. doi: 10.1007/s002210050805
Nowak, D., and Topka, H. (2006). Broadening a classic clinical triad: the hypokinetic motor disorder of normal pressure hydrocephalus also affects the hand. Exp. Neurol. 198, 81–87. doi: 10.1016/j.expneurol.2005.11.003
Nowak, D., Gumprecht, H., and Topka, H. (2006). CSF drainage ameliorates the motor deficit in normal pressure hydrocephalus: evidence from the analysis of grasping movements. J. Neurol. 253, 640–647. doi: 10.1007/s00415-006-0086-2
Ojemann, R., Fisher, C., Adams, R., Sweet, W., and New, P. (1969). Further experience with the syndrome of “Normal” pressure hydrocephalus. J. Neurosurg. 31, 279–294. doi: 10.3171/jns.1969.31.3.0279
Pastor, M., Jahanshahi, M., Artieda, J., and Obeso, J. (1992). Performance of repetitive wrist movements in Parkinson’s disease. Brain 115(Pt. 3), 875–891. doi: 10.1093/brain/115.3.875
Podsiadlo, D., and Richardson, S. (1991). The timed “Up & Go”: a test of basic functional mobility for frail elderly persons. J. Am. Geriatr. Soc. 39, 142–148. doi: 10.1111/j.1532-5415.1991.tb01616.x
Reitan, R., and Wolfson, D. (1993). The Halstead-Reitan neuropsychological test battery: theory and clinical applications. Tucson: Neuropsychology Press.
Roland, P., Larsen, B., Lassen, N., and Skinhøj, E. (1980). Supplementary motor area and other cortical areas in organization of voluntary movements in man. J. Neurophysiol. 43, 118–136. doi: 10.1152/jn.1980.43.1.118
Sørensen, P., Jansen, E., and Gjerris, F. (1986). Motor disturbances in normal-pressure hydrocephalus. Special reference to stance and gait. Arch. Neurol. 43, 34–38. doi: 10.1001/archneur.1986.00520010030016
Spencer, R., and Ivry, R. (2005). Comparison of patients with Parkinson’s disease or cerebellar lesions in the production of periodic movements involving event-based or emergent timing. Brain Cogn. 58, 84–93. doi: 10.1016/j.bandc.2004.09.010
Stegemöller, E., Allen, D., Simuni, T., and MacKinnon, C. (2017). Altered premotor cortical oscillations during repetitive movement in persons with Parkinson’s disease. Behav. Brain Res. 317, 141–146. doi: 10.1016/j.bbr.2016.09.036
Stegemöller, E., Simuni, T., and MacKinnon, C. (2009). Effect of movement frequency on repetitive finger movements in patients with Parkinson’s disease. Mov. Disord. 24, 1162–1169. doi: 10.1002/mds.22535
Toma, K., Mima, T., Matsuoka, T., Gerloff, C., Ohnishi, T., Koshy, B., et al. (2002). Movement rate effect on activation and functional coupling of motor cortical areas. J. Neurophysiol. 88, 3377–3385. doi: 10.1152/jn.00281.2002
Tsakanikas, D., Katzen, H., Ravdin, L., and Relkin, N. (2009). Upper extremity motor measures of tap test response in normal pressure hydrocephalus. Clin. Neurol. Neurosurg. 111, 752–757. doi: 10.1016/j.clineuro.2009.07.017
Williams, M., Thomas, G., de Lateur, B., Imteyaz, H., Rose, J., Shore, W., et al. (2008). Objective assessment of gait in normal-pressure hydrocephalus. Am. J. Phys. Med. Rehabil. 87, 39–45. doi: 10.1097/PHM.0b013e31815b6461
Keywords: idiopathic normal pressure hydrocephalus, finger tapping, diagnosis, 2.0 Hz, cut-off value
Citation: Shimizu Y, Tanikawa M, Horiba M, Sahashi K, Kawashima S, Kandori A, Yamanaka T, Nishikawa Y, Matsukawa N, Ueki Y and Mase M (2023) Clinical utility of paced finger tapping assessment in idiopathic normal pressure hydrocephalus. Front. Hum. Neurosci. 17:1109670. doi: 10.3389/fnhum.2023.1109670
Received: 28 November 2022; Accepted: 07 February 2023;
Published: 23 February 2023.
Edited by:
Etsuro Mori, Osaka University, JapanReviewed by:
Kenji Yoshiyama, Osaka University, JapanShigenori Kanno, Tohoku University, Japan
Noriaki Hattori, University of Toyama, Japan
Copyright © 2023 Shimizu, Tanikawa, Horiba, Sahashi, Kawashima, Kandori, Yamanaka, Nishikawa, Matsukawa, Ueki and Mase. This is an open-access article distributed under the terms of the Creative Commons Attribution License (CC BY). The use, distribution or reproduction in other forums is permitted, provided the original author(s) and the copyright owner(s) are credited and that the original publication in this journal is cited, in accordance with accepted academic practice. No use, distribution or reproduction is permitted which does not comply with these terms.
*Correspondence: Mitsuhito Mase, mitmase@med.nagoya-cu.ac.jp; Yoko Shimizu,
otyokos@med.nagoya-cu.ac.jp