- 1Department of Regenerative Systems Neuroscience, Human Brain Research Center, Kyoto University Graduate School of Medicine, Kyoto, Japan
- 2Department of Human Health Sciences, Kyoto University Graduate School of Medicine, Kyoto, Japan
- 3Division of Neurobiology and Physiology, Department of Neuroscience, Kyoto University Graduate School of Medicine, Kyoto, Japan
- 4Department of Neurology, Kyoto University Graduate School of Medicine, Kyoto, Japan
- 5Department of Electronics and Bioinformatics, Meiji University, Tokyo, Kanagawa, Japan
- 6The Graduate School of Core Ethics and Frontier Sciences, Ritsumeikan University, Kyoto, Japan
- 7Department of Rehabilitation and Physical Medicine, Hokkaido University Hospital, Sapporo, Japan
Progressive supranuclear palsy (PSP) is characterized by recurrent falls caused by postural instability, and a backward gait is considered beneficial for postural instability. Furthermore, a recent approach for rehabilitation combined with gait-oriented synchronized stimulation using non-invasive transcranial patterned stimulation could be promising for balance function. Here, we present a case of PSP with backward gait training combined with gait-synchronized transcranial alternating current stimulation (tACS). A 70-year-old woman with PSP-Richardson’s syndrome underwent backward gait training combined with synchronized cerebellar tACS. Initially, she underwent short-term intervention with combined training of backward gait with synchronized cerebellar tACS, asynchronized, or sham stimulation according to the N-of-1 study design. Synchronized tACS training demonstrated a decrease in postural instability, whereas asynchronized or sham stimulation did not. The additional long-term interventions of combined backward gait training with synchronized cerebellar tACS demonstrated further decrease in postural instability with improvements in gait speed, balance function, and fall-related self-efficacy in daily life. The present case describes a novel approach for motor symptoms in a patient with PSP. Backward gait training with synchronized cerebellar tACS may be a promising therapeutic approach.
1. Introduction
Progressive supranuclear palsy (PSP) is characterized by the rapid deterioration of Parkinsonism with supranuclear palsy and frontal lobe dysfunction (Höglinger et al., 2017). Postural instability is one of the symptomatic hallmarks of typical PSP with Richardson syndrome, which is closely related to quality of life (Brown et al., 2020). Although the symptoms affect activities of daily living, the effects of medication, including dopaminergic replacement therapies, are limited, and the development of training to maintain motor functions, including postural stability, is necessary.
Few studies have been conducted on rehabilitation approaches for the motor symptoms of PSP. Previous research has reported that postural reaction training with eye movements or visual awareness three times per week for 4 weeks moderately improved gait speed evaluated by 8 feet walk test (Zampieri and Di Fabio, 2008). The case report showed that 2.5-year exercise programs, including two 14 weeks of forward and backward walking training using a bodyweight-supported treadmill, maintained balance function in a patient with mixed PSP and cortico-basal degeneration (Steffen et al., 2007). Another case report showed that treadmill training for 8 weeks improved balance and gait, leading to a decrease in falls (Suteerawattananon et al., 2002). Although rehabilitation programs might be helpful in delaying the deterioration of motor symptoms, their effects seem to be limited in PSP patients (Intiso et al., 2018).
Recent rehabilitation strategies using non-invasive brain stimulation (NIBS) can be effective for gait and balance function (Forogh et al., 2017; Koganemaru et al., 2017; Krogh et al., 2022; Spiandor et al., 2022). While NIBS using anodal transcranial direct current stimulation enhances neuronal activities, NIBS using transcranial alternating current stimulation (tACS) synchronizes specific neuronal networks in a frequency and phase-dependent manner (Ali et al., 2013). The synchronization of widespread neuronal activities facilitates the transfer of information to remote areas and increases the possibility of inducing specific timing-dependent plasticity due to enhanced coincidental firing of pre- and post-synaptic neurons (Fell and Axmacher, 2011). Recently, gait-synchronized tACS over the foot area of the affected primary motor cortex (M1) improved gait and balance functions in post-stroke patients (Koganemaru et al., 2019; Kitatani et al., 2020). Cerebellar tACS has also been reported to synchronize gait cycles in healthy subjects, suggesting that the activity of gait-related neuronal networks interconnected with the cerebellar cortices was synchronized and facilitated by stimulation (Koganemaru et al., 2018, 2020).
For diseases presenting with Parkinsonism, including PSP, subclinical cerebellar involvement is reported as hypometabolism and pathological protein accumulation in cerebellar tissues (Kovacs et al., 2020) and functional impairments indicated by reduced cerebellar inhibition (CBI) (Shirota et al., 2010). The cerebellum regulates posture and balance during movements in coordination with the brainstem, which is disrupted in patients with Parkinsonism (Kurz et al., 2012; Hoogkamer et al., 2014; Peterson et al., 2014; Takakusaki, 2017). During gait, the cerebellum shows rhythmic bursts to produce step cycles and to maintain the balance of the gait-cycle-dependent swaying body in animals and possibly humans (Mori, 1987; Fukuyama et al., 1997; Mori et al., 1999). Cerebellar dysfunction leads to an inability to maintain balance during gait, resulting in a wide-based gait that enlarges the base of support in pure cerebellar dysfunction (Morton and Bastian, 2004). Compared to forward gait, backward gait is more specifically influenced by cerebellar activities that exert anticipatory postural adjustments without visual monitoring of steps and an external space (Timmann and Horak, 2001; Hoogkamer et al., 2017; Aman et al., 2018; Myers et al., 2018). Thus, backward gait training is effective in improving posture and balance, and decreasing falls (DeMark et al., 2019).
Therefore, we hypothesized that backward gait training combined with gait-synchronized tACS on the cerebellum would improve balance dysfunction, postural instability, and cerebellar activity in patients with PSP and systematically compared the short-term effects of three interventions: gait-synchronized tACS, gait-asynchronized tACS, and gait with sham tACS in a case of PSP–Richardson syndrome.
2. Case description
A 70-year-old woman without any medical history was referred to the hospital with difficulty in walking and recurrent falls that had deteriorated within a year. Neurological examination revealed supranuclear gaze palsy, truncal-dominant rigidity, and severe postural instability. The patient did not show any dystonia in the upper and lower extremities on the both sides. 123I-ioflupane single-photon emission computed tomography (Dat-SPECT) demonstrated bilateral depletion of dopamine transporters in the striatum, and MRI revealed atrophy of the midbrain tegmentum. 123I-IMP (N-isopropyl-p-123I-iodoamphetamine) SPECT revealed hypometabolism in both frontal lobes. The compound of levodopa and carbidopa was administered at a total dose of 450 mg/day, which was not effective for motor symptoms. Including levodopa-resistant motor symptoms, the patient was diagnosed with probable PSP-Richardson syndrome according to the clinical criteria (Höglinger et al., 2017). The participant was enrolled in this study a year and seven months after the onset of postural instability. The study protocol was approved by the Hokkaido University Certified Review Board of Japan (No. CRB1180001), and written informed consent was obtained from the patient (Table 1).
3. Diagnostic assessment
3.1. Clinical measurements
Clinical evaluations were performed before and after intervention. For the short-term evaluations, assessments included the timed up and go test (TUG), the mini-Balance Evaluation Systems Test (mini-BESTest) (Horak et al., 2009), and the Visual Analog Scale (VAS; score was determined by the distance on the 10 cm line, in which “0” indicated the worst condition and “10” indicated the best condition for the subjective judgment to the general motor function). For the long-term follow-ups, the Progressive Supranuclear Palsy Rating Scale (PSPRS) (Golbe and Ohman-Strickland, 2007), Fall Efficacy Scale (FES) (Tinetti et al., 1990), modified FES (Hill et al., 1996; Delbaere et al., 2011), and Activities-Specific Balance Confidence (ABC) Scale (Powell and Myers, 1995) were assessed. All the clinical evaluations were double-blinded.
3.2. Cerebellar brain inhibition (CBI)
To assess right cerebellar function, CBI was measured using the paired transcranial magnetic stimulation (TMS) technique (Magstim® BiStim2, Magstim Co. Ltd., UK) (Ugawa et al., 1994) for long-term evaluation. TMS pulse on the left primary motor cortex with intensity to evoke ∼1.0 mV peak-to-peak amplitude (SI 1 mV) which was preceded by the stimulation on the right cerebellar hemisphere at 90% intensity of resting motor threshold with an inter-stimulus interval (ISI) varying between 3, 5, and 10 ms were applied for 15 times for each ISIs. The figure-eight coils were placed on the primary motor cortex and cerebellum (Benussi et al., 2017). Motor evoked potentials were recorded using electrodes placed on the right first dorsal interosseous (FDI) muscle.
3.3. Intervention with tACS procedure
tACS was administered using a DC stimulator (NeuroConn DC, GmbH, Germany), according to a previously described gait-synchronized stimulation (Koganemaru et al., 2019). The stimulus electrode was placed on the right cerebellum (5 × 5 cm), which was determined with a neuro-navigation system using her head MRI scan (Brainsight Brainbox Ltd., UK). We chose the right side due to more severe motor symptoms on the right side compared with the left side. A reference electrode (7 × 5 cm) was placed on the left shoulder.
The current waveform was a sinusoidal wave with a peak-to-peak amplitude of 3 mA (−1.5 ∼ +1.5 mA) computed with an external computer, and the waveform was applied to the DC stimulator. Prior to the first intervention, we determined the stimulus frequency of the tACS by calculating the gait frequency (Hz) [= 1/one gait cycle time (s)] during a 30-s backward walking at a self-speed. After that, we gave the tACS with the determined frequency during a 2-min backward walking to detect the phase of tACS to synchronize the timing of the gait cycle of the right leg (the timing of the right heel contact) using a flat pressure sensor attached to the right heel (MF01-N-221-A01, Switch Science Inc., Japan). This was because the synchronized phase of cerebellar tACS was individually different, according to a previous study (Koganemaru et al., 2020). For the calculation of the synchronized phase, all instantaneous phases of the tACS at every right heel contact were determined and summarized as a histogram with 18 discrete bins (20° each). The mean phase of the bin representing the maximum number of right heel contacts was regarded as the synchronized phase.
First, we evaluated the effects of short-term intervention of backward gait training combined with cerebellar tACS according to the N-of-1 study design (Guyatt et al., 1990). We performed a combination of sham stimulation (Intervention A), gait-synchronized cerebellar tACS (Intervention B), and cerebellar tACS asynchronized with gait using the inverted phase as a control condition (Intervention C). The order of the interventions was A–C, with an interval of more than a week between the interventions. One session of backward training comprised a 4-min self-paced backward gait on the treadmill using Kineassist with truncal belts (Woodway USA, Inc.) to prevent falls and a 1-min break, and four sessions were performed in each intervention. In addition, the long-term intervention was performed with 10 times of the backward gait training combined with gait-synchronized cerebellar tACS twice a week for 5 weeks. The medication with a compound of levodopa and carbidopa (150 mg, three times a day) was continued during the interventions.
4. Results
The patient did not report any perception during all the stimulation conditions in the short-term and long-term interventions. There was neither side effect nor unanticipated event during all the interventions.
4.1. Results for the short-term intervention
The self-paced speed of the backward gait on the treadmill was 0.3 m/sec and the stimulus frequency of the tACS was 0.97 Hz. The phase of cerebellar tACS to synchronize the gait cycle of the right leg was 10° (Figure 1), which was used in Intervention B. The inverted phase (190°) was used as the control condition in Intervention C.
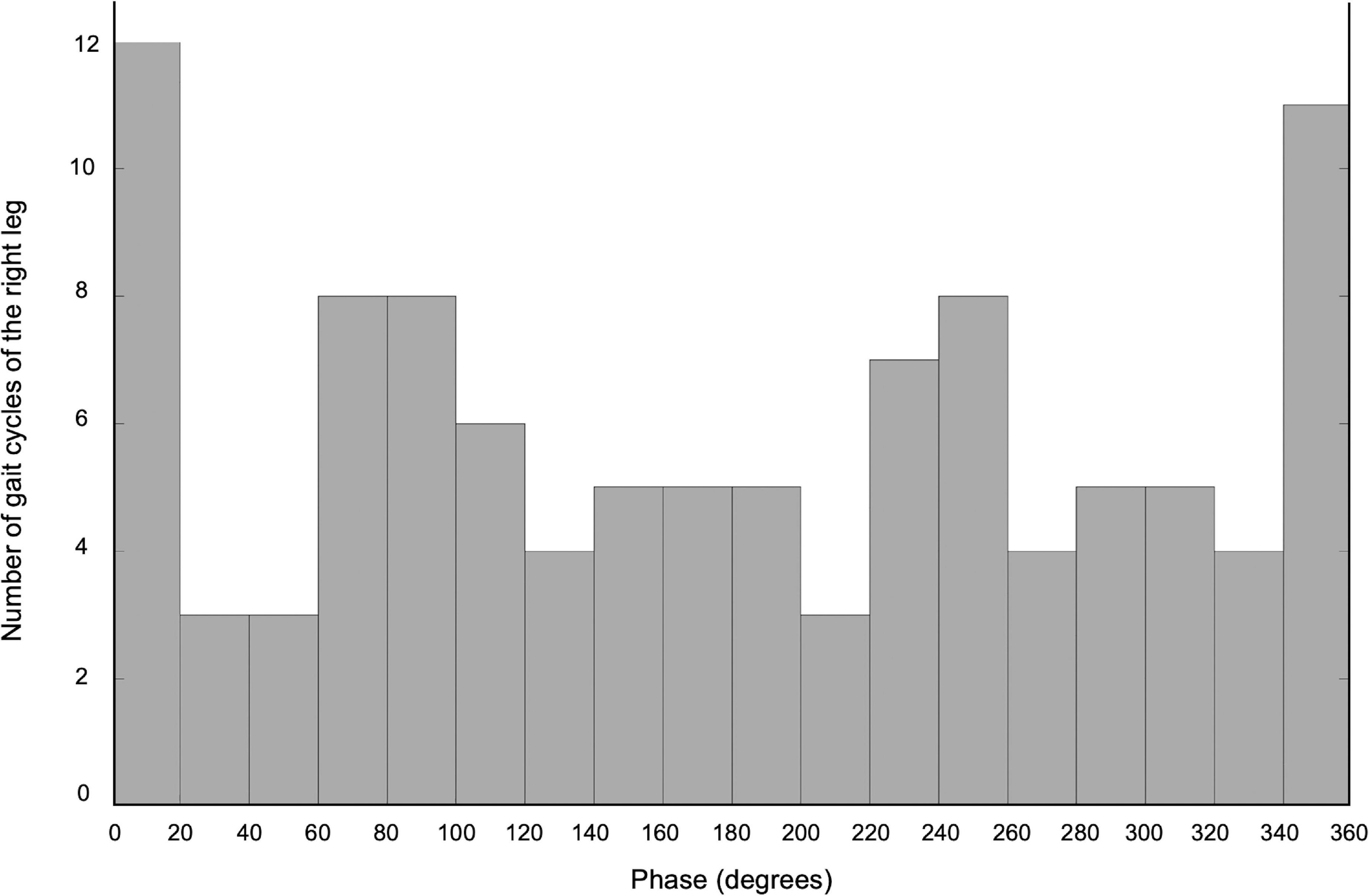
Figure 1. The histogram of the number of gait cycles of the right leg during the tACS X-axis demonstrates the phase of the cerebellar tACS, and Y-axis shows the number of gait cycles of the right leg.
The short-term intervention elucidated that Intervention B improved the time of TUG and the total score of the mini-BES test, whereas interventions A and C did not (Table 2 and Figure 2A, the baselines of TUG:10.91, 13.84 and 8.82 s, mini-BESTest:17, 15, and 17 in Interventions A–C, respectively. The VAS revealed the largest improvement in general motor symptoms in Intervention B (Figure 2A; baseline points were 7.6, 8.2, and 8.8 in Interventions A–C, respectively).
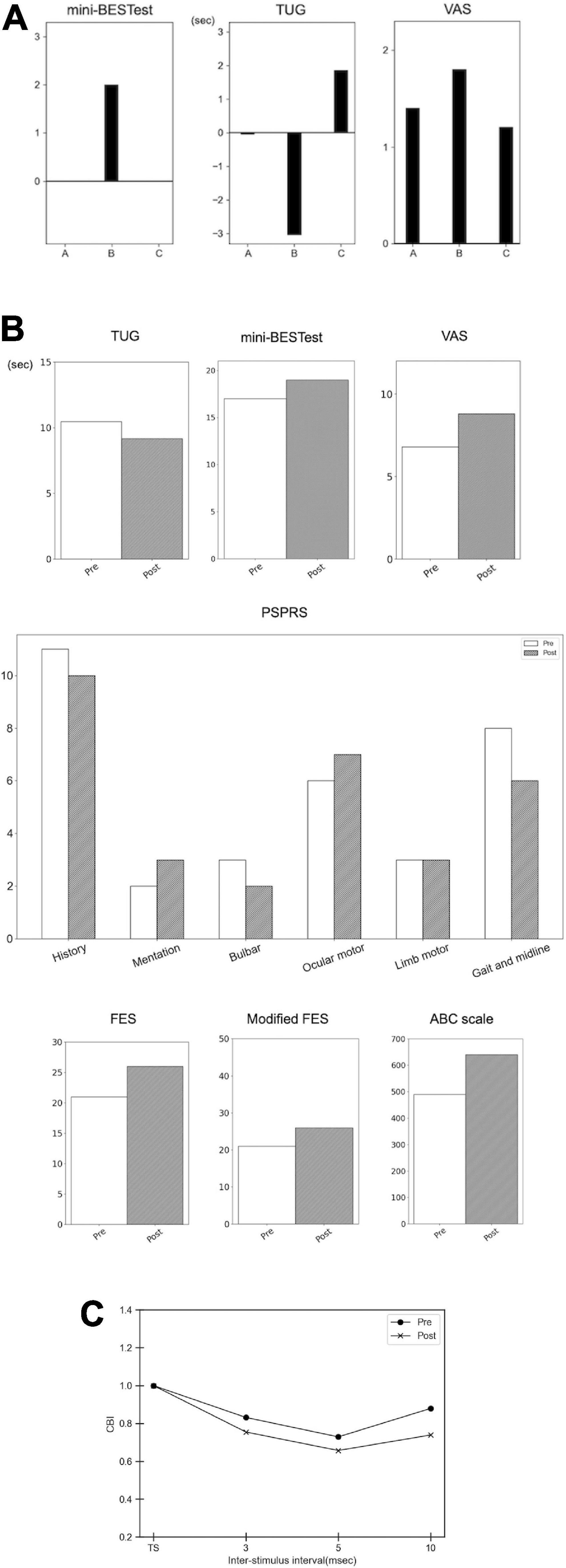
Figure 2. Results of the short-term intervention, long-term intervention, and cerebellar brain inhibition. (A) Results of the short-term intervention. The bar graph demonstrates the difference in the pre- and post-intervention (subtraction of the scores, i.e., post-minus pre-interventions). Intervention A, sham stimulation; intervention B, gait-synchronized cerebellar tACS; and intervention C, gait-asynchronized cerebellar tACS. (B) Results of the long-term intervention. The bar graph demonstrates the scores of mini-BESTest, VAS, the time for TUG (seconds), the sub-scores of Progressive Supranuclear Palsy Rating Scale (PSPRS), Fall Efficacy Scale (FES), modified FES, and Activities-Specific Balance Confidence (ABC) Scale in the pre- and post-interventions. (C) Cerebellar brain inhibition (CBI). The X-axis shows the inter-stimulus intervals, and the Y-axis demonstrates the CBI. TS, test stimulus.
4.2. Results for the additional long-term intervention
In addition, we evaluated the long-term intervention of backward gait training combined with gait-synchronized tACS in an observational study. We found improvements in gait and balance functions evaluated using TUG and mini-BESTest (Table 2 and Figure 2B). PSPRS was also improved, especially in the subscale of the “Gait and midline,” along with the VAS improvement for general symptoms (Figure 2B). The FES and modified FES scores increased after long-term intervention. The ABC scale also showed an increase in scores: 490 at pre-intervention and 640 at post-intervention (Figure 2B). CBI using paired TMS of the left M1 and right cerebellum was improved at inter-stimulus interval of 3,5, and 10 ms, suggesting that the function of the right cerebellum was recovered (Figure 2C).
5. Discussion
The present case demonstrates the potential therapeutic effect of backward gait training with synchronized cerebellar tACS in a patient with PSP. The short-term evaluation showed that training with the synchronized tACS seemed effective in improving the balance functions evaluated by TUG and the mini-BESTest, whereas sham- or asynchronized- tACS combined with backward gait did not improve them. The long-term intervention of synchronized tACS combined with backward gait training also improved gait speed and postural instability, as evaluated by TUG and mini-BESTest, and the general motor symptoms of PSP evaluated by PSPRS. The self-efficacy related to fall prevention showed an improvement in the scores for FES, modified FES, and ABC scales. Cerebellar function evaluated by the CBI was improved on the stimulated right side.
There are several approaches for the application of NIBS to the cerebellum in patients with PSP. However, their therapeutic effects on motor symptoms remain limited. One session of intermittent theta burst TMS (iTBS) on the cerebellum improved postural instability, but its long-term effects were not investigated (Pilotto et al., 2021). In an observational study, iTBS on the cerebellum improved only dysarthria, as evaluated by the PSPRS (Brusa et al., 2014).
NIBS can enhance motor function recovery in combination with rehabilitation programs (Koganemaru et al., 2015). Therefore, it would be appropriate to combine motor training with specific functions. Backward gait training has been reported to improve balance function as well as gait capacity in post-stroke patients (Wen and Wang, 2022), and to improve the stride length reflecting the gait-related balance function, compared with forward gait training in patients with Parkinson’s disease (Grobbelaar et al., 2017). Therefore, the combination of tACS of the cerebellum with backward gait training may have improved cerebellar motor control related to balance stability, resulting in improved axial symptoms of PSP in this case. Previous reports have demonstrated cerebellar dysfunction by reduced CBI in PSP patients (Shirota et al., 2010; Benussi et al., 2019). Cerebellar iTBS to induce LTP effects improved CBI without any improvement in balance function (Brusa et al., 2014). In the present case, CBI was improved at the stimulated side after long-term intervention. This suggests that improvement of cerebellar function may correlate with motor improvement due to cerebellar stimulation combined with specific training requiring cerebellar control.
The self-efficacy scores related to preventing falls showed an increase, as represented in the scores for the FES, modified FES, and ABC scales. The increase in scores may reflect a reduced risk of falls in daily living. The frequency of falls remained at zero during the interventions. The increased subjective confidence in preventing falls might have been related to the prevention of falls.
The short-term evaluation with asynchronized-tACS with the inverted phase demonstrated the deterioration of TUG. A previous report showed that asynchronized tACS over the regions between fronto-parietal areas with the inverted phase deteriorated the cognitive performance by decoupling relevant brain rhythm (Polanía et al., 2012). Similarly, it may be possible that the cerebellar function was temporally exacerbated due to decoupling the cerebellar activity with the gait rhythm to deteriorate the gait and balance function in the TUG test. Detailed assessments of cerebellar function are required in the stimulation with the inverted phase during the gait.
We applied the measurement of the mini-BESTest. The mini-BESTest is considered sensitive in the assessment of Parkinson’s disease to discriminate between fallers and non-fallers (Leddy et al., 2011), suggesting its high sensitivity of balance dysfunction in patients with Parkinsonism. Although some items (e.g., lateral push and release, standing on foam with eyes closed) are hard to perform in PSP-Richardson syndrome (Dale et al., 2022), this patient could complete almost all the assessments. The “reactive postural control” sub-scores of the mini-BESTest showed improvements only in the intervention B (real stimulation) of the short-term intervention. Therefore, we considered that the mini-BESTest could appropriately evaluate for the improvement of the balance function in this case.
The long-term intervention was performed as an additional observational study. A previous observational study and case reports showed an improvement of the balance function or fall tendency in PSP patients by the long-term exercise program (Matsuda et al., 2022) or gait training (Suteerawattananon et al., 2002; Steffen et al., 2007). Therefore, the practice effect may affect the performance after the intervention in this case. A comparative study is warranted by conducting long-term backward gait trainings with or without the gait-synchronized brain stimulation.
The spatial resolution of the tACS is limited (Yang et al., 2021) and the scalp-applied currents are attenuated to 25% by soft tissue and the skull (Miranda et al., 2006; Wagner et al., 2007; Vöröslakos et al., 2018). However, we confirmed the peak intensity of the electric field on the right cerebellum and less distribution on other brain areas by simulating the electric field distribution in the patient’s head MRI with the current electrode montage (Saturnino et al., 2019; Puonti et al., 2020).
As typical PSP cases demonstrate mild atrophy of the brainstem and cerebellum (Mimuro and Yoshida, 2020), this case showed mild cerebellar atrophy. Meanwhile, the peak of electrical field was simulated on the right cerebellum and the cerebellar-brain inhibition was enhanced after the long-term intervention. Therefore, we considered that the application of tACS with the current electrode montage could modify the cerebellar activity.
In normal gait, the cerebellum plays a pivotal role in predictive feedforward adaptation to novel terrains and environments (Takakusaki, 2013; Pisotta and Molinari, 2014). A previous study showed that patients with cerebellar impairment had a difficulty or inability in feedforward prediction and adaptation relating to the lower-limb and posture controls during the gait on the split-belt treadmill (Morton and Bastian, 2006). Compared to forward gait, backward gait needs more precise feedforward postural and lower-limb adaptation by the cerebellum due to the controlling the body and limbs without visual information (Klous et al., 2011). Then, the present intervention with cerebellar tACS and backward gait training may have contributed to improvements of the predictive feedforward adaptation by the cerebellum.
The present case demonstrates that backward gait training combined with synchronized cerebellar tACS can be a promising treatment for improving the motor symptoms of PSP.
Author’s note
The patient stated that she hoped that the present findings may contribute to the development of new strategies for the treatment of PSP.
Data availability statement
The raw data supporting the conclusions of this article will be made available by the authors, without undue reservation.
Ethics statement
The studies involving human participants were reviewed and approved by Hokkaido University Certified Review Board of Japan. The patient provided her written informed consent to participate in this study. Written informed consent was obtained from her for the publication of any potentially identifiable images or data included in this article.
Author contributions
AS and SK designed the study, collected and interpreted data, and contributed to the initial draft of the manuscript. AS, AO, TMiy, YN, YM, KO, and YO contributed to data collection and analysis. TMim and RT contributed to the interpretation and critically reviewed the manuscript. All authors approved the final version of the manuscript.
Funding
This work was supported by Grants-in-Aid for Scientific Research (B) (21H03308), Grants-in-Aid for Exploratory Research (20K21770) (SK), Grants-in-Aid for Scientific Research on Innovative Areas (22H04788), Grants-in-Aid for Exploratory Research (21K19745), and Grants-in-Aid for Scientific Research (A) (19H01091) (TMim).
Conflict of interest
The authors declare that the research was conducted in the absence of any commercial or financial relationships that could be construed as a potential conflict of interest.
Publisher’s note
All claims expressed in this article are solely those of the authors and do not necessarily represent those of their affiliated organizations, or those of the publisher, the editors and the reviewers. Any product that may be evaluated in this article, or claim that may be made by its manufacturer, is not guaranteed or endorsed by the publisher.
References
Ali, M. M., Sellers, K. K., and Fröhlich, F. (2013). Transcranial alternating current stimulation modulates large-scale cortical network activity by network resonance. J. Neurosci. 33, 11262–11275. doi: 10.1523/JNEUROSCI.5867-12.2013
Aman, J. E., Valberg, S. J., Elangovan, N., Nicholson, A., Lewis, S. S., and Konczak, J. (2018). Abnormal locomotor muscle recruitment activity is present in horses with shivering and purkinje cell distal axonopathy. Equine Veter. J. 50, 636–643. doi: 10.1111/evj.12813
Benussi, A., Dell’Era, V., Cantoni, V., Turrone, R., Pilotto, A., Alberici, A., et al. (2019). Stimulation over the cerebellum with a regular figure-of-eight coil induces reduced motor cortex inhibition in patients with progressive supranuclear palsy. Brain Stimulat. 12, 1290–1297. doi: 10.1016/j.brs.2019.05.017
Benussi, A., Dell’Era, V., Cotelli, M. S., Turla, M., Casali, C., Padovani, A., et al. (2017). Long term clinical and neurophysiological effects of cerebellar transcranial direct current stimulation in patients with neurodegenerative ataxia. Brain Stimulat. 10, 242–250. doi: 10.1016/j.brs.2016.11.001
Brown, F. S., Rowe, J. B., Passamonti, L., and Rittman, T. (2020). Falls in progressive supranuclear palsy. Movement Dis. Clin. Pract. 7, 16–24. doi: 10.1002/mdc3.12879
Brusa, L., Ponzo, V., Mastropasqua, C., Picazio, S., Bonnì, S., Lorenzo, F. D., et al. (2014). Theta burst stimulation modulates cerebellar-cortical connectivity in patients with progressive supranuclear palsy. Brain Stimulat. 7, 29–35. doi: 10.1016/j.brs.2013.07.003
Dale, M. L., Prewitt, A. L., Harker, G. R., McBarron, G. E., and Mancini, M. (2022). Perspective: balance assessments in progressive supranuclear palsy: lessons learned. Front. Neurol. 13:1–7. doi: 10.3389/fneur.2022.801291
Delbaere, K., Smith, S. T., and Lord, S. R. (2011). Development and initial validation of the iconographical falls efficacy scale. J. Gerontol. Series Biol. Sci. Med. Sci. 66, 674–680. doi: 10.1093/gerona/glr019
DeMark, L., Fox, E. J., Spigel, P. M., Osborne, J., and Rose, D. K. (2019). Clinical application of backward walking training to improve walking function, balance, and fall-risk in acute stroke: a case series. Top. Stroke Rehabilit. 26, 497–502. doi: 10.1080/10749357.2019.1641011
Fell, J., and Axmacher, N. (2011). The role of phase synchronization in memory processes. Nat. Rev. Neurosci. 12, 105–118. doi: 10.1038/nrn2979
Forogh, B., Ahadi, T., Nazari, M., Sajadi, S., Latif, L. A., Hejazi, S. M., et al. (2017). The effect of repetitive transcranial magnetic stimulation on postural stability after acute stroke: a clinical trial. Basic Clin. Neurosci. 8, 405–412. doi: 10.18869/nirp.bcn.8.5.405
Fukuyama, H., Ouchi, Y., Matsuzaki, S., Nagahama, Y., Yamauchi, H., Ogawa, M., et al. (1997). Brain functional activity during gait in normal subjects: a SPECT study. Neurosci. Lett. 228, 183–186. doi: 10.1016/S0304-3940(97)00381-9
Golbe, L. I., and Ohman-Strickland, P. A. (2007). A clinical rating scale for progressive supranuclear palsy. Brain 130, 1552–1565. doi: 10.1093/brain/awm032
Grobbelaar, R., Venter, R., and Welman, K. E. (2017). Backward compared to forward over ground gait retraining have additional benefits for gait in individuals with mild to moderate parkinson’s disease: a randomized controlled trial. Gait Posture 58, 294–299. doi: 10.1016/j.gaitpost.2017.08.019
Guyatt, G. H., Keller, J. L., Jaeschke, R., Rosenbloom, D., Adachi, J. D., and Newhouse, M. T. (1990). The n -of-1 randomized controlled trial: clinical usefulness. Ann. Int. Med. 112:293. doi: 10.7326/0003-4819-112-4-293
Hill, K. D., Schwarz, J. A., Kalogeropoulos, A. J., and Gibson, S. J. (1996). Fear of falling revisited. Arch. Phys. Med. Rehabilit. 77, 1025–1029. doi: 10.1016/S0003-9993(96)90063-5
Höglinger, G. U., Respondek, G., Stamelou, M., Kurz, C., Josephs, K. A., Lang, A. E., et al. (2017). Clinical diagnosis of progressive supranuclear palsy: the movement disorder society criteria. Mov. Dis. 32, 853–864. doi: 10.1002/mds.26987
Hoogkamer, W., Meyns, P., and Duysens, J. (2014). Steps forward in understanding backward gait: from basic circuits to rehabilitation. Exerc. Sport Sci. Rev. 42, 23–29. doi: 10.1249/JES.0000000000000000
Hoogkamer, W., Potocanac, Z., Van Calenbergh, F., and Duysens, J. (2017). Quick foot placement adjustments during gait are less accurate in individuals with focal cerebellar lesions. Gait Posture 58, 390–393. doi: 10.1016/j.gaitpost.2017.08.037
Horak, F. B., Wrisley, D. M., and Frank, J. (2009). The balance evaluation systems test (BESTest) to differentiate balance deficits. Phys. Ther. 89, 485–498.
Intiso, D., Bartolo, M., Santamato, A., and Rienzo, F. D. (2018). The role of rehabilitation in patients with progressive supranuclear palsy: a narrative review. PM R 10, 636–645. doi: 10.1016/j.pmrj.2017.12.011
Kitatani, R., Koganemaru, S., Maeda, A., Mikami, Y., Matsuhashi, M., Mima, T., et al. (2020). Gait-combined transcranial alternating current stimulation modulates cortical control of muscle activities during gait. Eur. J. Neurosci. 52, 4791–4802.
Klous, M., Mikulic, P., and Latash, M. L. (2011). Two aspects of feedforward postural control: anticipatory postural adjustments and anticipatory synergy adjustments. J. Neurophysiol. 105, 2275–2288. doi: 10.1152/jn.00665.2010
Koganemaru, S., Fukuyama, H., and Mima, T. (2015). Two is more than one: how to combine brain stimulation rehabilitative training for functional recovery? Front. Syst. Neurosci. 9:1–8. doi: 10.3389/fnsys.2015.00154
Koganemaru, S., Goto, F., Arai, M., Toshikuni, K., Hosoya, M., Wakabayashi, T., et al. (2017). Effects of vestibular rehabilitation combined with transcranial cerebellar direct current stimulation in patients with chronic dizziness: an exploratory study. Brain Stimulat. 10, 576–578. doi: 10.1016/j.brs.2017.02.005
Koganemaru, S., Kitatani, R., Fukushima-Maeda, A., Mikami, Y., Okita, Y., Matsuhashi, M., et al. (2019). Gait-synchronized rhythmic brain stimulation improves poststroke gait disturbance: a pilot study. Stroke 50, 3205–3212. doi: 10.1161/STROKEAHA.119.025354
Koganemaru, S., Mikami, Y., Maezawa, H., Matsuhashi, M., Ikeda, S., Ikoma, K., et al. (2018). Anodal transcranial patterned stimulation of the motor cortex during gait can induce activity-dependent corticospinal plasticity to alter human gait. PLoS One 13:1–19. doi: 10.1371/journal.pone.0208691
Koganemaru, S., Mikami, Y., Matsuhashi, M., Truong, D. Q., Bikson, M., Kansaku, K., et al. (2020). Cerebellar transcranial alternating current stimulation modulates human gait rhythm. Neurosci. Res. 156, 265–270. doi: 10.1016/j.neures.2019.12.003
Kovacs, G. G., Lukic, M. J., Irwin, D. J., Arzberger, T., Respondek, G., Lee, E. B., et al. (2020). Distribution patterns of tau pathology in progressive supranuclear palsy. Acta Neuropathol. 140, 99–119. doi: 10.1007/s00401-020-02158-2
Krogh, S., Jønsson, A. B., Aagaard, P., and Kasch, H. (2022). Efficacy of repetitive transcranial magnetic stimulation for improving lower limb function in individuals with neurological disorders: a systematic review and meta-analysis of randomized sham-controlled trials. J. Rehabilit. Med. 54:1097. doi: 10.2340/jrm.v53.1097
Kurz, M. J., Wilson, T. W., and Arpin, D. J. (2012). Stride-time variability and sensorimotor cortical activation during walking. NeuroImage 59, 1602–1607. doi: 10.1016/j.neuroimage.2011.08.084
Leddy, A. L., Crowner, B. E., and Earhart, G. M. (2011). Utility of the mini-BESTest, BESTest, and BESTest sections for balance assessments in individuals with parkinson disease. J. Neurol. Phys. Ther. 35, 90–97. doi: 10.1097/NPT.0b013e31821a620c
Matsuda, N., Takamatsu, Y., and Aiba, I. (2022). Effect of therapeutic exercise on the balance of patients with progressive supranuclear palsy: a pilot study. Front. Neurol. 13:955893. doi: 10.3389/fneur.2022.955893
Mimuro, M., and Yoshida, M. (2020). Chameleons and mimics: progressive supranuclear palsy and corticobasal degeneration. Neuropathology 40, 57–67. doi: 10.1111/neup.12590
Miranda, P. C., Lomarev, M., and Hallett, M. (2006). Modeling the current distribution during transcranial direct current stimulation. Clin. Neurophysiol. 117, 1623–1629. doi: 10.1016/j.clinph.2006.04.009
Mori, S. (1987). Integration of posture and locomotion in acute decerebrate cats and in awake, freely moving cats. Prog. Neurobiol. 28, 161–195. doi: 10.1016/0301-0082(87)90010-4
Mori, S., Matsui, T., Kuze, B., Asanome, M., Nakajima, K., and Matsuyama, K. (1999). Stimulation of a restricted region in the midline cerebellar white matter evokes coordinated quadrupedal locomotion in the decerebrate cat. J. Neurophysiol. 82, 290–300. doi: 10.1152/jn.1999.82.1.290
Morton, S. M., and Bastian, A. J. (2004). Cerebellar control of balance and locomotion. Neuroscientist 10, 247–259. doi: 10.1177/1073858404263517
Morton, S. M., and Bastian, A. J. (2006). Cerebellar contributions to locomotor adaptations during splitbelt treadmill walking. J. Neurosci. 26, 9107–9116. doi: 10.1523/JNEUROSCI.2622-06.2006
Myers, P. S., McNeely, M. E., Pickett, K. A., Duncan, R. P., and Earhart, G. M. (2018). Effects of exercise on gait and motor imagery in people with parkinson disease and freezing of gait. Parkins. Related Dis. 53, 89–95. doi: 10.1016/j.parkreldis.2018.05.006
Peterson, D. S., Pickett, K. A., Duncan, R. P., Perlmutter, J. S., and Earhart, G. M. (2014). Brain activity during complex imagined gait tasks in parkinson disease. Clin. Neurophysiol. 125, 995–1005. doi: 10.1016/j.clinph.2013.10.008
Pilotto, A., Rizzetti, M. C., Lombardi, A., Hansen, C., Biggi, M., Verzeroli, G., et al. (2021). Cerebellar RTMS in PSP: a double-blind sham-controlled study using mobile health technology. Cerebellum 20, 662–666. doi: 10.1007/s12311-021-01239-6
Pisotta, I., and Molinari, M. (2014). Cerebellar contribution to feedforward control of locomotion. Front. Hum. Neurosci. 8:1–5. doi: 10.3389/fnhum.2014.00475
Polanía, R., Nitsche, M. A., Korman, C., Batsikadze, G., and Paulus, W. (2012). The importance of timing in segregated theta phase-coupling for cognitive performance. Curr. Biol. 22, 1314–1318. doi: 10.1016/j.cub.2012.05.021
Powell, L. E., and Myers, A. M. (1995). The activities-specific balance confidence (ABC) scale. J. Gerontol. 50A, M28–M34.
Puonti, O., Leemput, K. V., Saturnino, G. B., Siebner, H. R., Madsen, K. H., and Thielscher, A. (2020). Accurate and robust whole-head segmentation from magnetic resonance images for individualized head modeling. NeuroImage 219:117044. doi: 10.1016/j.neuroimage.2020.117044
Saturnino, G. B., Puonti, O., Nielsen, J. D., Antonenko, D., Madsen, K. H., and Thielscher, A. (2019). SimNIBS 2.1: a comprehensive pipeline for individualized electric field modelling for transcranial brain stimulation. Cham: Springer International Publishing.
Shirota, Y., Hamada, M., Hanajima, R., Terao, Y., Matsumoto, H., Ohminami, S., et al. (2010). Cerebellar dysfunction in progressive supranuclear palsy: a transcranial magnetic stimulation study. Mov. Dis. 25, 2413–2419. doi: 10.1002/mds.23298
Spiandor, V., Cezar, P., Santos, R., Orcioli-silva, D., Teresa, L., Gobbi, B., et al. (2022). Transcranial direct current stimulation for balance rehabilitation in neurological disorders: a systematic review and meta-analysis. Ageing Res. Rev. 8, 1568–1637. doi: 10.1016/j.arr.2022.101736
Steffen, T. M., Boeve, B. F., Mollinger-riemann, L. A., and Petersen, C. M. (2007). Long-term locomotor training for gait and balance in a patient with mixed progressive supranuclear palsy and corticobasal degeneration. Phys. Ther. 87:8. doi: 10.2522/ptj.20060166
Suteerawattananon, M., MacNeill, B., and Protas, E. J. (2002). Supported treadmill training for gait and balance in a patient with progressive supranuclear palsy. Phys. Ther. 82, 485–495. doi: 10.1093/ptj/82.5.485
Takakusaki, K. (2013). Neurophysiology of gait: from the spinal cord to the frontal lobe. Mov. Dis. 28, 1483–1491. doi: 10.1002/mds.25669
Takakusaki, K. (2017). Functional neuroanatomy for posture and gait control. J. Mov. Dis. 10, 1–17. doi: 10.14802/jmd.16062
Timmann, D., and Horak, F. B. (2001). Perturbed step initiation in cerebellar subjects: 2. modification of anticipatory postural adjustments. Exp. Brain Res. 141, 110–120. doi: 10.1007/s002210100858
Tinetti, M. E., Richman, D., and Powell, L. (1990). Falls efficacy as a measure of fear of falling. J. Gerontol. 45, 239–243. doi: 10.1093/geronj/45.6.P239
Ugawa, Y., Genba-Shimizu, K., Rothwell, J. C., Iwata, M., and Kanazawa, I. (1994). Suppression of motor cortical excitability by electrical stimulation over the cerebellum in ataxia. Ann. Neurol. 36, 90–96. doi: 10.1002/ana.410360117
Vöröslakos, M., Takeuchi, Y., Brinyiczki, K., Zombori, T., Oliva, A., Fernández-Ruiz, A., et al. (2018). Direct effects of transcranial electric stimulation on brain circuits in rats and humans. Nat. Commun. 9:483. doi: 10.1038/s41467-018-02928-3
Wagner, T., Fregni, F., Fecteau, S., Grodzinsky, A., Zahn, M., and Pascual-Leone, A. (2007). Transcranial direct current stimulation: a computer-based human model study. NeuroImage 35, 1113–1124. doi: 10.1016/j.neuroimage.2007.01.027
Wen, H., and Wang, M. (2022). Backward walking training impacts positive effect on improving walking capacity after stroke: a meta-analysis. Int. J. Environ. Res. Public Health 19:370. doi: 10.3390/ijerph19063370
Yang, D., Shin, Y., and Hong, K. S. (2021). Systemic review on transcranial electrical stimulation parameters and EEG/FNIRS features for brain DISEASES. Front. Neurosci. 15:629323. doi: 10.3389/fnins.2021.629323
Keywords: non-invasive brain stimulation (NIBS), transcranial alternating current stimulation, cerebellum, rehabilitation, entrainment, backward gait, progressive supranuclear palsy, Parkinsonism
Citation: Shima A, Tanaka K, Ogawa A, Omae E, Miyake T, Nagamori Y, Miyata Y, Ohata K, Ono Y, Mima T, Takahashi R and Koganemaru S (2023) Case report: Backward gait training combined with gait-synchronized cerebellar transcranial alternating current stimulation in progressive supranuclear palsy. Front. Hum. Neurosci. 17:1082555. doi: 10.3389/fnhum.2023.1082555
Received: 28 October 2022; Accepted: 06 February 2023;
Published: 22 February 2023.
Edited by:
Stephane Perrey, Université de Montpellier, FranceReviewed by:
Fay Horak, Oregon Health and Science University, United StatesJared Skinner, Appalachian State University, United States
Copyright © 2023 Shima, Tanaka, Ogawa, Omae, Miyake, Nagamori, Miyata, Ohata, Ono, Mima, Takahashi and Koganemaru. This is an open-access article distributed under the terms of the Creative Commons Attribution License (CC BY). The use, distribution or reproduction in other forums is permitted, provided the original author(s) and the copyright owner(s) are credited and that the original publication in this journal is cited, in accordance with accepted academic practice. No use, distribution or reproduction is permitted which does not comply with these terms.
*Correspondence: Satoko Koganemaru, a29nYW5lQGt1aHAua3lvdG8tdS5hYy5qcA==