- 1Neuromechanics Laboratory, Department of Biomedical Engineering, Emory University & Georgia Institute of Technology, Atlanta, GA, United States
- 2College of Arts and Sciences, Emory University, Atlanta, GA, United States
- 3Department of Medicine, Division of Geriatrics and Gerontology, Emory University School of Medicine, Atlanta, GA, United States
- 4Department of Neurology, Emory University School of Medicine, Atlanta, GA, United States
- 5Department of Biomedical Informatics, Emory University School of Medicine, Atlanta, GA, United States
- 6Department of Music, Emory University College of Arts and Sciences, Atlanta, GA, United States
- 7Department of Rehabilitation Medicine, Emory University School of Medicine, Atlanta, GA, United States
- 8Emory University School of Nursing, Atlanta, GA, United States
- 9Atlanta VA Center for Visual & Neurocognitive Rehabilitation, Atlanta, GA, United States
- 10Birmingham/Atlanta VA Geriatric Research Education and Clinical Center, Atlanta, GA, United States
Introduction: Dance-based therapies are an emerging form of movement therapy aiming to improve motor and cognitive function in older adults with mild cognitive impairments (MCIs). Despite the promising effects of dance-based therapies on function, it remains unclear how age-related declines in motor and cognitive function affect movement capacity and influence which movements and rhythms maximize dance therapy efficacy. Here, we evaluated the effects of age and MCI on the ability to accurately modulate spatial (i.e., joint kinematics), temporal (i.e., step timing), and spatiotemporal features of gait to achieve spatial and temporal targets during walking.
Methods: We developed novel rhythmic movement sequences—nine spatial, nine temporal, and four spatiotemporal—that deviated from typical spatial and temporal features of walking. Healthy young adults (HYA), healthy older adults (HOA), and adults with MCI were trained on each gait modification before performing the modification overground, with kinematic data recorded using wearable sensors.
Results: HOA performed spatial (p = 0.010) and spatiotemporal (p = 0.048) gait modifications less accurately than HYA. Individuals with MCI performed spatiotemporal gait modifications less accurately than HOA (p = 0.017). Spatial modifications to the swing phase of gait (p = 0.006, Cohen’s d = −1.3), and four- and six-step Duple rhythms during temporal modifications (p ≤ 0.030, Cohen’s d ≤ 0.9) elicited the largest differences in gait performance in HYA vs. HOA and HOA vs. MCI, respectively.
Discussion: These findings suggest that age-related declines in strength and balance reduce the ability to accurately modulate spatial gait features, while declines in working memory in individuals with MCI may reduce the ability to perform longer temporal gait modification sequences. Differences in rhythmic movement sequence performance highlight motor and cognitive factors potentially underlying deficits in gait modulation capacity, which may guide therapy personalization and provide more sensitive indices to track intervention efficacy.
Introduction
Dance-based therapy, which purposefully sets movements to music, is an emerging form of movement therapy for individuals with mild cognitive impairments (MCIs), a precursor to dementia or Alzheimer’s involving reduced attention, executive function, spatial cognition, and working memory, that elicits improvements in cognitive function in individuals with MCI (Gauthier et al., 2006; Lazarou et al., 2017; Sanford, 2017; Zhu et al., 2018, 2020). Dance-based therapy is a logical approach for improving cognitive function, as exercise or general physical activity are among the few interventions shown to improve cognitive function in individuals with MCI (Geda et al., 2010; Thom and Clare, 2011). Specifically, Thom and Clare (2011) noted that physical exercise programs that target functional tasks, aerobic endurance, and balance are likely needed to maximize the functional benefits of therapy, all of which are challenged during the dance. Currently, it is unclear how to personalize dance-based therapy parameters for individuals with MCI. Studies of dance-based therapy use different music, dance types, and study designs (Hackney and Earhart, 2009; McKee and Hackney, 2013; Lazarou et al., 2017; Zhu et al., 2020). Improving our understanding of the relationships between individual-specific deficits associated with MCI and the ability to perform different movements during dance-based therapy is needed to inform the objective design of personalized dance-based therapies for individuals with MCI.
While a major goal of dance-based therapies is to maximize the motor and cognitive benefits of moving to music, how to do so for a given population needs better clarity. Music type and tempo, and the dance type or steps are basic parameters that can influence the difficulty of performing the therapy routine. Therapies often rely on trial and error to refine a protocol, rather than being grounded in a principled data-driven understanding of myriad factors that may impact dance-therapy performance, including individual differences in motor control and cognitive function (McKee and Hackney, 2013; Lazarou et al., 2017; Zhu et al., 2018). Selecting an appropriate level of challenge is fundamental to maximizing the motor and cognitive effects of therapy (Guadagnoli and Lee, 2004). Given the heterogeneity of cognitive deficits in MCI (Gauthier et al., 2006) and age-related declines in motor function (Booth et al., 1994), understanding how both motor and cognitive deficits impact the ability to perform challenging rhythmic movement patterns may both enable objective personalization of dance-based therapy parameters and provide mechanistic insights into the impacts of MCI on mobility.
Though not yet quantified in the context of dance-based therapy, dance can be considered a series of Rhythmic Movement Sequences (RMS): rhythmic patterns involving modifications to spatial and temporal components of normal stepping (Rallis et al., 2018). Importantly, spatial and temporal features of movement correspond to and may challenge, distinct neural representations (e.g., the premotor cortex) and multiple aspects of biomechanics function (e.g., strength, balance, joint coordination; Wilson and Kwon, 2008; Kornysheva and Diedrichsen, 2014). A central premise of this work is that evaluating the capacity for modulating spatial and temporal movement features will shed light on motor and cognitive mechanisms that may inform future therapy individualization and enhance the efficacy of dance and music-based movement therapy in individuals with MCI. Spatial modifications of movement during dance involve altering the magnitude and coordination of hip, knee, and ankle kinematics (Ivanenko et al., 2005; Wilson and Kwon, 2008). Voluntarily altering coordination relative to highly stereotyped patterns during locomotion requires the motor capacity to achieve joint excursions and increased cortical demands (Ivanenko et al., 2005; Cohen et al., 2016; Hortobágyi et al., 2016; Rucco et al., 2017). Consequently, both motor and cognitive deficits may impact individuals’ abilities to perform modifications to the spatial components of movement. Muscle function and motor control change with age, and correspond to reductions in overall motor function in older adults (Booth et al., 1994; Schloemer et al., 2017). Therefore, age-related deficits in motor function may impact individuals’ abilities to modify spatial components of movement. Because spatial modifications also require mapping perceived biomechanical targets to motor actions, we expect these modifications to challenge aspects of cognitive function that are impaired in individuals with MCI, such as spatial attention, executive function, and spatial cognition (Gauthier et al., 2006; Rucco et al., 2017). However, how these motor and cognitive deficits impact individuals’ abilities to voluntarily modulate spatial aspects of movement remains unclear.
Temporal modifications of movement involve altering step timing to produce rhythmic sequences of steps, which may challenge cognitive function (Kornysheva and Diedrichsen, 2014). During dance therapy, temporal modifications can be elicited by altering the music’s rhythm and meter, two fundamental components of music (Hackney et al., 2007a; Hackney and Earhart, 2009). In the context of stepping or walking, rhythm defines the pattern of temporal stimuli, such as taking two quick (q) steps followed by two slow (S) steps. Meter is a perceived quantity that individuals anticipate and to which they entrain their movements. Unimpaired walking typically involves evenly recurring steps that are approximately rhythmically and metrically constant, such that modifying temporal features during RMS would represent a deviation from normal gait rhythm and meter. Because temporal modifications require attention and the ability to perceive rhythmic cues and map them to motor actions, we expect it to challenge aspects of cognitive function that are impaired in individuals with MCI, such as attention and working memory (Gauthier et al., 2006; Grahn, 2012; Sanford, 2017). Because temporal modifications can be performed with normal joint coordination patterns, they are less likely to challenge motor function than spatial modifications. Therefore, we expect spatial modifications to movement during RMS to challenge both motor and cognitive function, while temporal modifications should primarily challenge cognitive function. However, the extent to which age-related motor and cognitive deficits impact the ability to modulate spatial and temporal aspects of movement is unclear.
In this study, we leveraged biomechanical analysis, prototypical dance movements, and music theory to systematically investigate the effects of age-related differences in motor and cognitive function on the ability to perform spatial and temporal modifications to walking in individuals with MCI. We hypothesized that differences in motor and cognitive function would impact individuals’ abilities to accurately modulate spatial and temporal features of walking, similar to those performed during dance-based therapies. Specifically, we predicted that, when performing RMS gait modifications of varying difficulty, healthy older adults (HOA) would perform spatial and spatiotemporal gait modifications significantly less accurately than healthy young adults (HYA), suggesting an effect associated with aging-related differences in motor function. Similarly, we predicted that individuals with MCI would perform temporal and spatiotemporal RMS less accurately than HOA, suggesting an effect associated with impaired cognitive function.
Materials and methods
This study was approved by the Emory University Institutional Review Board (STUDY00003507). All participants gave written informed consent prior to participation.
Study design and visits
We performed an observational cross-sectional study in healthy younger and older adults, and older adults with MCI. All outcomes were collected during a single 2–4 h study visit for each participant. Participants who were unable to complete the protocol in a single visit completed it during one additional visit within 1 week.
Participants
A total of 37 participants were recruited for the study, including younger adults (HYA, N = 13), older adults (HOA, N = 12), and older adults with MCI (N = 12 Table 1). One HYA participant failed to complete the protocol and was excluded from the analysis. For all participants, inclusion criteria included the ability to walk 20 m without an assistive device, completion of at least 6 years of education or good work history, no hospitalizations in the last 60 days, and proficiency in the English language. HYA participants ages 18–35 years were included in this study and HOA participants ages 55 years and older were included. Additional inclusion criteria for participants with MCI included amnestic MCI defined using the Alzheimer’s Disease Neuroimaging Initiative criteria and reduced executive function, working memory, and spatial cognition according to standard clinical assessments (Phillips et al., 2001; Vandierendonck et al., 2004; Mueller et al., 2005; Bowie and Harvey, 2006; Hackney et al., 2013).
Motor and cognitive assessments
To characterize participants’ motor and cognitive function, trained personnel administered the following motor and cognitive assessments: Montreal Cognitive Assessment (MoCA), Reverse Corsi Blocks test, Tower of London test, Trail Making Test, Four-Square Step Test, Body Position Spatial Task, simple, manual, and cognitive Timed Up-and-Go (TUG), and the 30-s Chair Stand (Lundin-Olsson et al., 1998; Jones et al., 1999; Morris et al., 2001; Phillips et al., 2001; Dite and Temple, 2002; Vandierendonck et al., 2004; Nasreddine et al., 2005; Bowie and Harvey, 2006; Hackney et al., 2013). The average (±1 SD) scores for each clinical assessment and corresponding p-values denoting the probability of statistical differences in each score were computed using the software package R (Table 2). Older adults and individuals with MCI were well-matched in age and sex. As expected, the MCI participants trended toward worse performance on the tests of motor and cognitive function. HYA participants performed better than HOA or MCI participants on all assessments of motor and cognitive function. For comparison to the tempi of temporal and spatiotemporal gait modifications, we also recorded participants’ stride frequencies at their preferred walking speeds.
RMS gait modifications
Participants performed normal walking at their self-selected and fast speeds, and 22 RMS consisting of nine spatial, nine temporal, and four spatiotemporal gait modifications, described below. Participants were randomly assigned to perform either the spatial or temporal modifications first. Spatiotemporal modifications always occurred after spatial and temporal modification trials. Experimental conditions within each sub-group (spatial, temporal, or spatiotemporal) were randomized.
We defined 64 spatial, temporal, and spatiotemporal gait modifications, constituting a movement library, from which we selected nine spatial, nine temporal, and four spatiotemporal gait modifications of varying levels of difficulty. The selected biomechanical (i.e., spatial) and temporal features of each modification are described in Figure 1A. The figure shows the kinematic configuration of each movement and lists the target variables. Briefly, spatial modifications altered the magnitude of the hip, knee, and ankle movements and their coordination relative to normal walking (Ivanenko et al., 2005). We selected three stance-phase modifications (ST), three swing-phase modifications (SW), and three combined modifications in both the swing and stance phases (SWST). Participants were provided target joint angles for each modification, such as flexing the hip and knee to 90 degrees during the swing; and watched a video of an expert dancer performing the movements. During ST and SW trials, participants modified stance and swing-phase gait kinematics, respectively, in both legs asymmetrically. During SWST trials, participants modified both swing and stance only in the left leg.
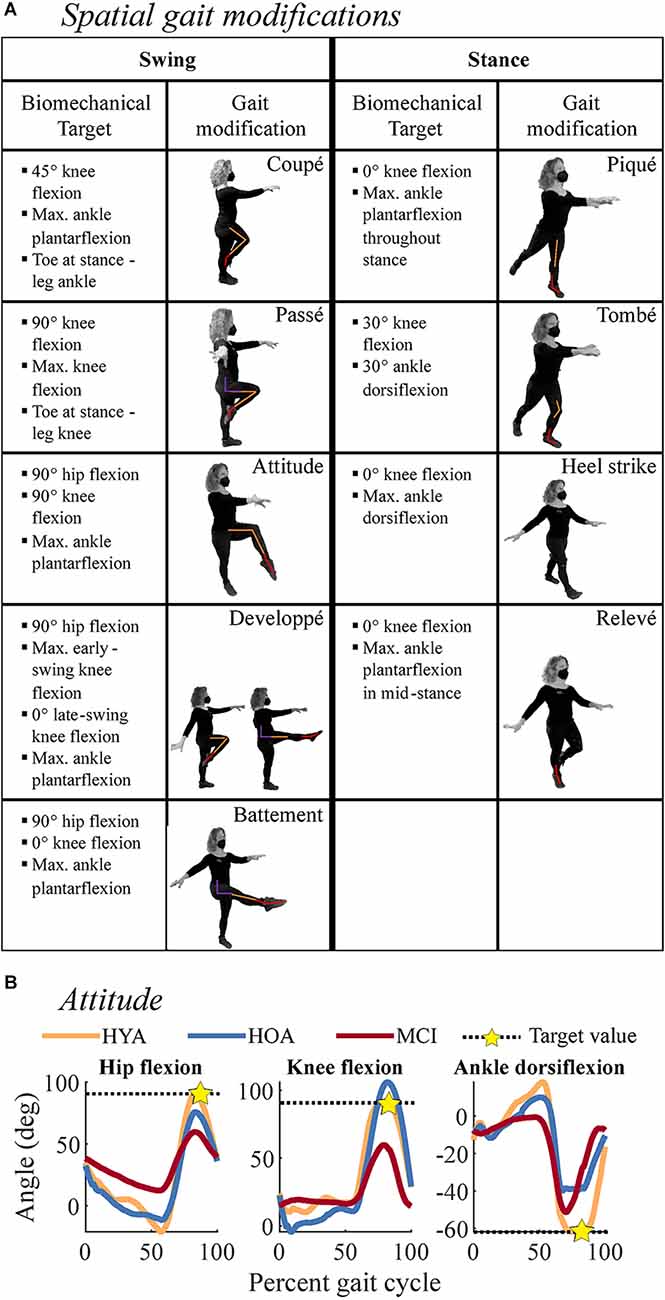
Figure 1. Descriptions of subcomponents of the spatial gait modifications. or rhythmic movement sequences (RMS) evaluated in the current study. (A) Spatial gait modifications were derived from ballet steps, noted in the boxes containing movement depictions. The left two columns correspond to swing-phase modifications, while the right two columns correspond to stance-phase modifications. Each depiction shows the modification where biomechanical targets were applied. Colored lines on each person denote biomechanical targets at the hip (purple), knee (orange), and ankle (red) kinematics that were used to evaluate movement performance. Bulleted lists describe the biomechanical targets for each gait modification, which were used to quantify movement performance. Bullets denote the biomechanical targets for each gait modification. Modifications were performed in pairs during spatial trials. (B) Example of biomechanical targets for the Attitude gait modification. Black dashed lines denote biomechanical target values and gold stars denote where the target value was estimated. For example, during the Attitude modification, the swing-leg hip (left) and knee (middle) should be flexed to 90 degrees, and the ankle (right) should be maximally plantarflexed. Colored lines denote exemplary HYA (orange), HOA (blue), and MCI (red) participants. These participants highlight reduced RMS performance at the hip and knee in MCI and at the ankle in HOA and MCI.
Temporal modifications altered the rhythmic patterns of movement, consisting of two, four, or six steps taken at half-beat (“and;” denoted &), one-beat (“quick;” denoted q), and two-beat (“slow;” denoted S) intervals (Figure 2). The music notation used in Figure 2 indicates the sequence and duration of steps, with closed (black) notes corresponding to a step spanning one beat and open (white) notes denoting steps spanning two beats. Performance was evaluated by each participant’s ability to take steps matching the beat and in the correct sequence of quick and slow steps.
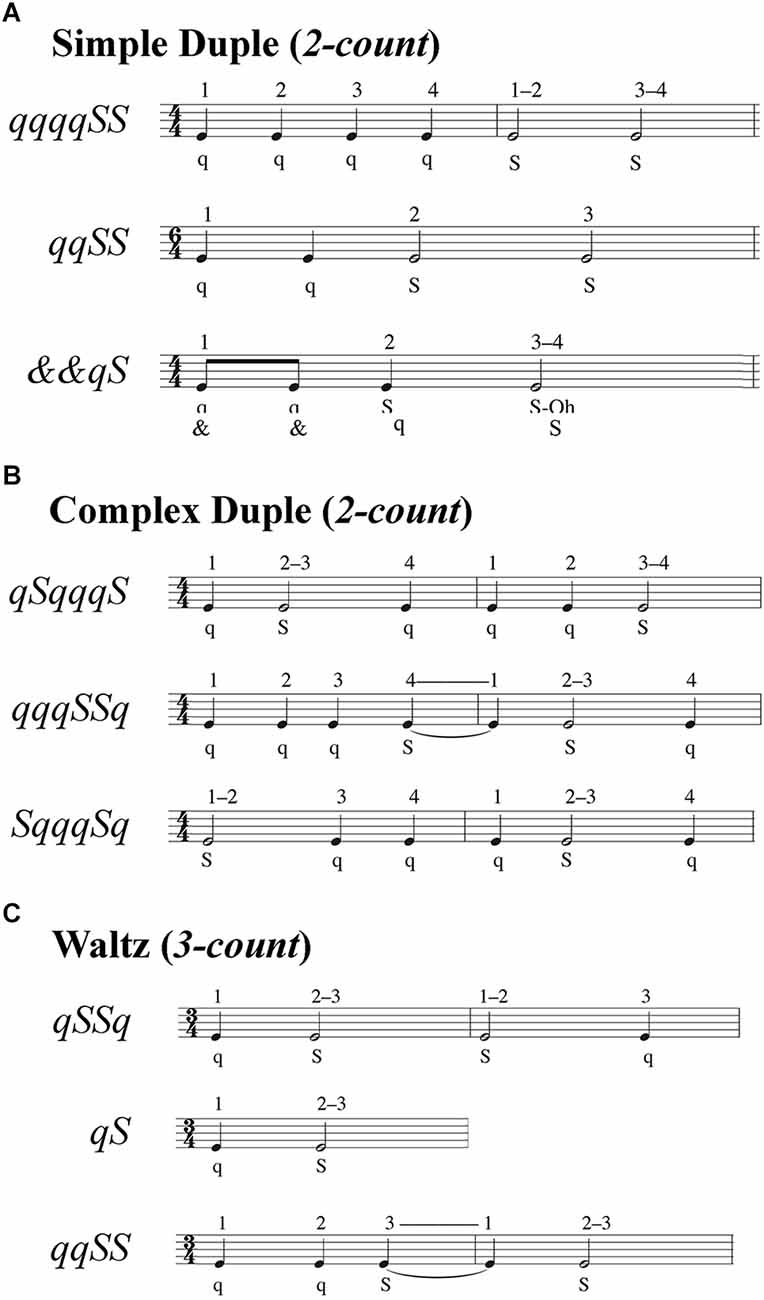
Figure 2. Step sequences for temporal and spatiotemporal gait modifications. Step sequences consisted of two, four, or six steps. Each sequence specified a combination of very quick (& = half-beat), quick (q = one beat), and slow (S = two beats) steps. The numbers above the musical bars show the beat count for each rhythm. (A) Simple duple sequences used a two-count rhythm in which the strong beat was the downbeat. (B) Complex duple sequences also used a two-count rhythm, but the strong beat was not the downbeat. (C) Waltz sequences used a three-count rhythm, such that sequences spanned either one or two measures.
Temporal modifications were split into three sub-groups: three simple duple, three complex duple, and three waltz modifications. Duple music (Figures 2A,B) had a tempo of 100 beats per minute (bpm) for all participants, while Waltz music (Figure 2C) had a tempo of 134 bpm for HYA but was reduced to 104 bpm for the HOA and MCI groups. The faster Waltz tempo for the HYA group was due to an error in coding the tempi for the first eight participants. We corrected the tempo for the HOA and MCI to ensure that all participants could perform the temporal modifications. We used the same tempi across all participants, rather than personalizing the tempi to each participant’s preferred cadence to avoid giving participants different amounts of time to process and remember auditory input, which could alter cognitive demands for each participant (Gauthier et al., 2006; Persad et al., 2008; Montero-Odasso et al., 2012). The simple duple meter has a two-count grouping (1–2) where the downbeat is the strong beat. In more complex duple meters, the emphasized beat falls on the weak beat, which defies Western music listeners’ expectations. Consequently, we expected the complex duple to be more challenging than the simple duple. The waltz is in a compound duple meter (a fast two-count where each is subdivided into three sub-beats; 1-2-3, 1-2-3) or simple triple (three-count; 1-2-3) meter. Waltz meters, unlike simple and complex duple meters, in which synchronization of movement to music is easier because the same foot always lands on the downbeat, the three-count of waltz rhythms causes the foot landing on the downbeat to alternate every measure (three steps). Consequently, individuals accustomed to Western music traditions may have greater difficulty synchronizing movements to the triple meter of the waltz than to duple meters. The movements within each sub-group varied in difficulty. During duple trials, participants were cued by a simplified Libertango (Piazzolla, 1974), with superfluous musical accents and cues eliminated to improve the ability of participants with limited musical experience to identify temporal patterns in the music. During waltz trials, participants were cued by a modified version of Shostakovich Waltz No. 2 (Shostakovich, 1938). These musical pieces are much more complex than a metronome beat used in locomotion studies, as the attention required to dissociate meter and rhythm from superfluous auditory information can alter individuals’ abilities to entrain to the tempo (Styns et al., 2007; Moumdjian et al., 2019). For each modification, participants were provided a sequence of “quick” and “slow” beats and practiced the sequence by following an instructional video: first clapping, then tapping the foot, then shifting weight between the legs, then stepping in place, then walking (Hackney and Earhart, 2010).
Spatiotemporal modifications combined spatial and temporal modifications of varying difficulty (Figures 1 and 2). We selected these movements to vary the relative motor and cognitive challenges of the movements. Participants were allowed to review spatial and temporal instructional videos if needed. Following instructions for all modifications, the assessors would briefly practice (3–5 sequences) the modifications with the participant to confirm understanding. Data were collected immediately after an understanding was confirmed. Names and descriptions of all gait modifications can be found in the Supplementary Material, with reference to Figures 1 and 2.
During each trial, participants wore 15 inertial measurement units (IMUs) in a standard configuration (APDM, Inc., Portland, USA). We used validated commercial software associated with the IMUs to estimate lower-limb hip, knee, and ankle flexion angles over the gait cycle (Mancini and Horak, 2016; Washabaugh et al., 2017). Participants began each trial in a quiet stance until hearing a tone, then began walking in a modified gait pattern defined by the trial’s spatial, temporal, or spatiotemporal RMS. When physically capable, participants walked overground along an 11 m walkway four times for each trial, providing 15–30 (spatial) or 15–60 (temporal) strides for each trial. For participants with reduced physical capacity, we iteratively reduced the number of walkway lengths traveled from four lengths down to one length based on each participant’s physical capacity. We ensured that each participant performed at least 15 strides for spatial modifications and 10 sequences for temporal and spatiotemporal modifications. However, all participants performed the same gait modifications, regardless of the distance walked. Participants were allowed to rest as needed and were provided snacks and water between trials. All assessments were administered by trained personnel.
RMS performance
For each modification, we defined biomechanical (spatial and spatiotemporal trials) and temporal (temporal and spatiotemporal trials) targets, defined a priori in our movement library (Figure 1A). Biomechanical targets matched those provided during instruction. Temporal targets corresponded to the step sequence of quick and slow steps. RMS performance was defined as a percent error relative to biomechanical and temporal targets: the error relative to each target value, normalized by the magnitude of the corresponding target (Figure 1B). For example, the hip flexion angle target for the attitude movement, the star in Figure 1B, is 90 degrees during the swing phase of gait. In swing, the knee should be flexed to 90 degrees, and the ankle maximally plantarflexed. The targets for each spatial modification are listed in Figure 1A. Therefore, RMS performance reflected the accuracy with which each participant performed a gait modification, with lower percent error reflecting more accurate (i.e., better) performance. For each trial and participant, we averaged across each trial’s biomechanical and temporal targets [Equation (1)]. For each gait modification class (spatial, temporal, spatiotemporal), we computed the error the ith participant made on the jth trial of the kth target variable (e.g., peak knee flexion angle) as follows:
where xi, j, k denotes the ith participant’s outcome for the jth trial and kth target variable (e.g., peak knee flexion angle), and is a vector of outcomes over all available strides for that trial. The variable x target, j, k denotes the value of the jth trial and kth target variable. The variable J represents the number of trials included in each modification class and K represents the number of pre-specified target variables for each gait modification (spatial: nine modifications, 2–3 target variables per trial; temporal: nine modifications, one target variable per trial; spatiotemporal: four modifications, 3–4 target variables per trial). Percent error for each target (multiple targets per trial; see Figures 1 and 2) was averaged to compute a single error value for the trial. When multiple trials were included in a single analysis (e.g., all spatial trials), the percent errors were averaged across trials. We computed RMS performance outcomes using all available data from each trial, regardless of trial length.
Statistical analysis
Group differences in demographic and clinical characteristics were assessed with omnibus analysis of variance (ANOVA) and Chi-squared test, as appropriate. Significant results (α = 0.05) were followed by the post-hoc independent sample t-tests for differences between the HYA and HOA groups, and between the HOA and MCI groups. Based on our hypotheses, we only compared the HYA and HOA groups, and the HOA and MCI groups.
We examined differences in RMS performance across groups using separate fixed effects linear models for each outcome variable. To test for group differences in RMS performance between the HYA and HOA groups, and between HOA and MCI groups, we used linear models containing indicator variables denoting the HYA and MCI groups, with HOA as the reference group (Equation (2). Significant coefficients (Wald Tests, α = 0.05) corresponding to the HYA (βHYA) or MCI variables (βMCI) would indicate significant differences in performance between the HOA group and the HYA or MCI groups, respectively. Separate linear models were fit for each of the three gait modification classes (spatial, temporal, spatiotemporal). The sample size for each model was N = 36. Because we were not interested in comparisons between HYA and MCI, we parameterized linear models so that the coefficients would directly test the contrasts of interest.
Where the error is a 36 × 1 vector with one sample for each participant. The variable β0 denotes the average percent error of the HOA group, βHYA and βMCI are coefficients corresponding to the magnitude of differences between the HYA and HOA groups and the HOA and MCI groups, respectively. Variables IHYA and IMCI are indicator variables corresponding to the respective coefficients. The variable ε is an error term. Linear models were fit using fitlm in MATLAB 2021b (Mathworks Ltd, Natick, USA), which estimates coefficients using least-squares regression and determines the coefficients’ p-values using Wald tests. To determine whether specific types of gait modifications were differentiated between groups, we repeated the above analysis for gait modification subclasses (e.g., swing, simple duple) and individual gait modifications (e.g., attitude-developpe, qqqqSS; Figures 1A and 2A).
To determine which movements best distinguished between groups, we computed effects sizes (Cohen’s d; vs. HOA) and the average difference (Δ vs. HOA) for each gait modification class (spatial, temporal, spatiotemporal). To determine whether specific gait modifications were differentiated between groups, we repeated the above analysis for gait modification subclasses (e.g., swing, simple duple).
As an exploratory investigation to identify potential correlates of RMS performance, we computed separate Pearson correlations between each of the clinical assessments of motor and cognitive function (Table 2) against spatial, temporal, and spatiotemporal gait modification performance, with N = 36 samples per correlation.
Results
Study compliance and participants
Data were collected from 37 participants (12 per group, plus one additional HYA participant). The additional HYA participant was unable to follow directions when performing rhythmic movement sequences and was excluded from the analysis. One MCI participant failed to complete the protocol due to fatigue and completed the protocol in a follow-up visit. One MCI participant was only able to complete temporal trials due to fatigue and balance concerns. Across the 36 participants included in the analysis, only 19 trials (less than 2% of all trials) were omitted due to sensor errors not detected during the experiment. Most participants completed the full four walkway lengths of the RMS protocol. Across groups, 24 participants completed four walkway lengths, 10 participants completed two-to-three lengths (five HOA, five MCI), and only two participants (one HOA, one MCI) completed a single walkway length.
Demographic characteristics for each participant group are shown in Table 1. Participants exhibited similar demographic characteristics, as expected. Clinical characteristics for each participant group are shown in Table 2. Individuals with an MCI diagnosis trended toward worse performance than HOA on the Trail Making Test (p = 0.099; independent-samples t-tests), cognitive (p = 0.084), and manual (p = 0.067) TUG tests. HOA performed worse than HYA on the Reverse Corsi Blocks (p = 0.003), Body Position Spatial Task (p = 0.028), and the Four-Square Step Test (p = 0.002). All three groups walked with similar cadences.
RMS performance was reduced with age
When averaging across gait modifications within each modification class (spatial, temporal, spatiotemporal), regression analysis revealed that the HYA group performed spatial gait modifications significantly more accurately than the HOA group (p = 0.010; d = 1.1, Δ = 4.0%; Figure 3). HYA participants also performed spatiotemporal gait modifications more accurately than HOA participants (p = 0.048; d = 0.8, Δ = 2.1%). Conversely, HYA participants did not perform temporal gait modifications significantly more accurately than HOA participants.
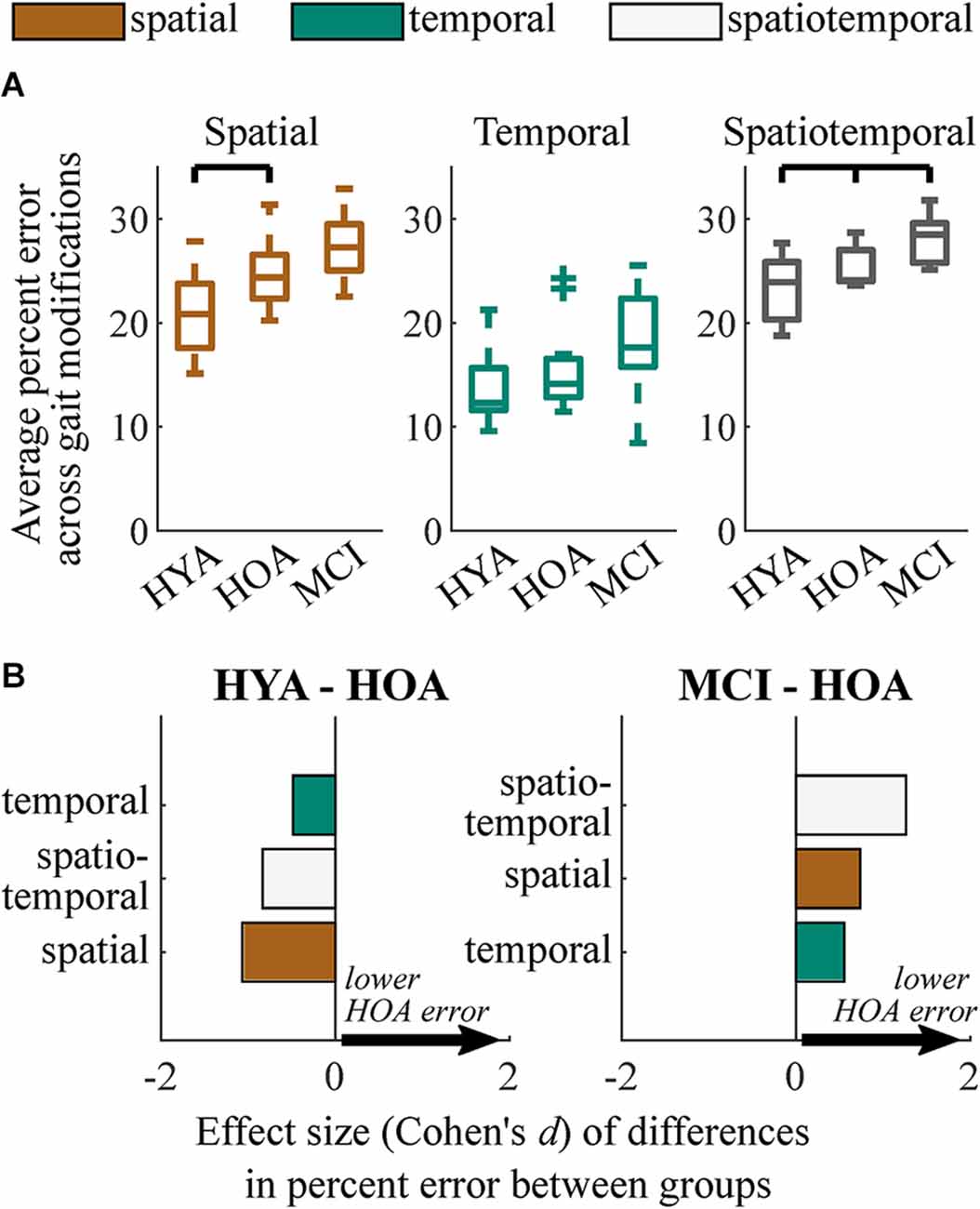
Figure 3. Summary of group performance on gait modifications. (A) Boxplots showing group performance on spatial, temporal, and spatiotemporal trials. Each sample consists of the average percent error relative to target values across nine gait modifications for spatial and temporal trials and four gait modifications for spatiotemporal trials. (B) Effect sizes of differences in performance (Cohen’s d) between HYA and HOA groups (left) and HOA and MCI groups (right) on spatial (orange), temporal (green), and spatiotemporal (white) gait modifications. Positive values (arrows) imply that the HOA group exhibited lower error on gait modifications. We did not test for differences between the HYA and MCI groups.
Within movement subclasses (e.g., swing, simple-duple), differences in spatial performance between the HYA and HOA groups were largest in modifications to the swing phase of gait (p = 0.006, d = −1.3, Δ = 5.8%; Figures 4A,B). Differences in gait modification performance in both the stance phase (p = 0.074, d < 0.8, Δ = 2.2%) and the combined swing-stance modifications (p = 0.054, d < 0.9, Δ = 2.8%) had smaller effects that did not reach significance. In particular, deficits in gait modification accuracy in HOA were largest in swing-phase modifications that involved large hip flexion ranges of motion (i.e., attitude, developpé, and battement; see modification descriptions in Figure 1A and Supplementary Material), with large effect sizes (p < 0.001; d > 0.9; Δ = 4.5%). These differences are exemplified by the attitude-developpé modification shown in Figure 4C.
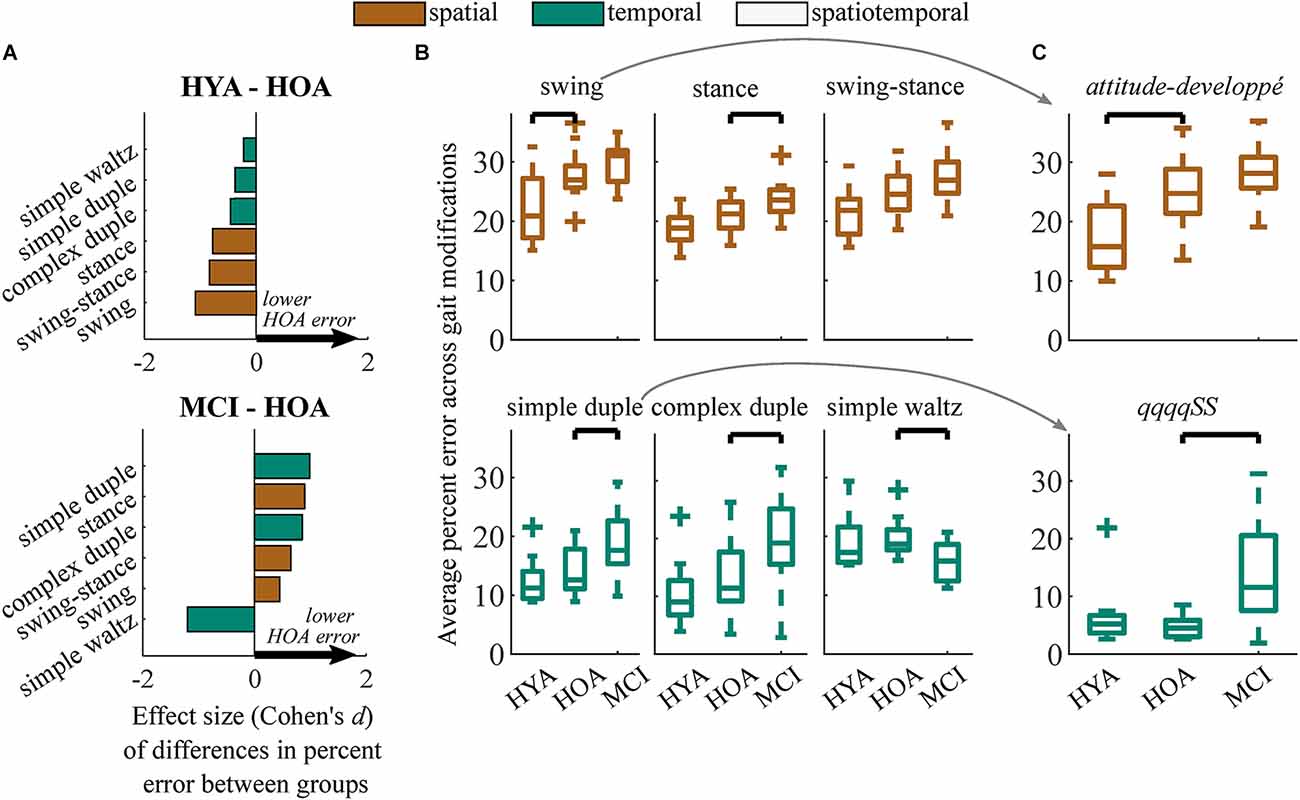
Figure 4. (A) Effect sizes of differences in performance (Cohen’s d) between HYA and HOA groups (top) and HOA and MCI groups (bottom) in each gait modification subclass. Spatial and temporal modifications are denoted in orange and green, respectively. Positive values (arrows) imply that the HOA group exhibited lower error on gait modifications compared to either group. (B) Boxplots showing distributions of gait modification accuracy (percent error vs. targets) for spatial (top) and temporal (bottom) gait modifications. (C) Results from exemplary gait modifications that elicited the largest between-group effect sizes. The attitude-developpé modification (top) elicited the largest difference in spatial performance between the HYA and HOA groups. The simple duple modification, qqqqSS, elicited the largest difference in temporal performance between the HOA and MCI groups but did not differentiate between the HYA and HOA groups. We did not test for differences between the HYA and MCI groups.
RMS performance was reduced in older adults with mild cognitive impairments
Unlike differences in the HYA and HOA groups, the HOA group performed only spatiotemporal gait modifications significantly more accurately than the MCI group (p = 0.017; d = 1.3, Δ = 2.6%; Figure 3A). While many participants with MCI exhibited reduced spatial and temporal modification accuracy compared to the HOA group, these differences did not reach statistical significance. Temporal accuracy was highly variable in both groups, with some participants with MCI performing temporal modifications more accurately than those in the HOA group. However, the HOA and MCI groups still exhibited a moderate differential effect size (p = 0.16; d = 0.6; Δ = 2.4%).
Differences in spatiotemporal modification accuracy were driven by HOA performing the simple duple (p = 0.012, d = 1.0, Δ = 4.9%), complex duple (p = 0.030, d = 0.9, Δ = 6.5%), and stance (p = 0.032, d = 0.9, Δ = 2.6%) gait modifications more accurately than MCI participants (Figures 4A,B). An example of group differences in simple duple modification accuracy is shown in Figure 4C, in which participants with MCI struggled to perform the six-step temporal sequence qqqqSS. Surprisingly, the MCI group performed the waltz gait modifications significantly more accurately than the HOA group (p = 0.013, d = 1.2, Δ = −4.0%). Datasheets containing clinical and demographic characteristics, effect sizes, and average differences in percent error between groups for each gait modification can be found in the Supplementary Material.
Associations of motor and cognitive function with RMS performance: preliminary evidence
Exploratory correlation analyses identified non-negligible correlations in 27/29 combinations of variables tested [Table 3; asterisks designate effect sizes with cutoff values according to Cohen (1992)]. The strongest correlations were between spatial modification performance and: Reverse Corsi Blocks product score (Pearson’s r = −0.50), Four-Square Step Test completion time (r = 0.55), and Body Position Spatial Task product score (r = −0.55; Table 3). In the table, asterisks denote effect sizes for each correlation (Cohen, 1992). Temporal gait modifications exhibited at most moderate correlations with clinical assessments: Reverse Corsi Blocks product score (r = −0.31), Four-Square Step Test completion time (r = 0.29), and Body Position Spatial Task product score (r = −0.28). Spatiotemporal gait modifications exhibited the strongest correlations with the Four-Square Step Test completion time (r = 0.59), and Body Position Spatial Task product score (r = −0.69).
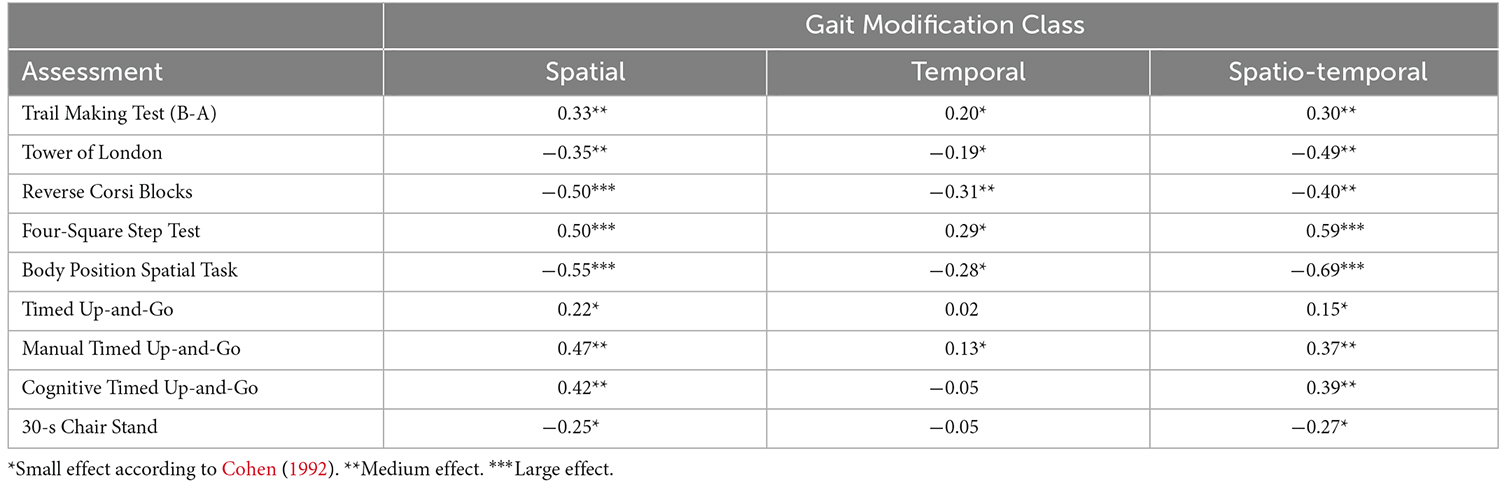
Table 3. Correlations (Pearson’s r) between RMS performance in each of three gait modification classes clinical outcomes.
Discussion
This study leveraged biomechanical analysis and music theory to investigate the impacts of age and cognitive function on individuals’ abilities to flexibly modulate spatial and temporal aspects of their gait. Age-related declines in motor function (HYA vs. HOA) appear to reduce individuals’ capacities to flexibly modulate spatial aspects of gait, though cognitive deficits (MCI vs. HOA) may also contribute to reductions in this capacity. While temporal modification performance was more variable, cognitive deficits appear to reduce individuals’ abilities to modulate temporal aspects of gait. Unsurprisingly, both age-related declines in motor and cognitive function appear to reduce individuals’ abilities to flexibly modulate spatiotemporal aspects of gait, as would be done in music or dance therapy. By testing a broad set of spatial and temporal movement patterns, our novel experimental framework revealed group differences in movement performance that would not be revealed by normal walking or by a single set of gait modifications (e.g., backward walking, fast walking). Given the importance of selecting an appropriate level of challenge during rehabilitation, our findings suggest that both motor and cognitive function, as well as movement types, should be considered when designing complex movement, music-movement, or dance-based therapies for individuals with MCI as others have recommended (Guadagnoli and Lee, 2004).
Motor and, possibly, cognitive deficits reduce the ability to accurately modulate spatial gait features
Reduced performance on spatial and spatiotemporal, but not temporal, gait modifications in HOA relative to HYA suggests that age-related declines in motor function may limit individuals’ abilities to modulate spatial components of gait. Aging is associated with reductions in strength and balance (Schloemer et al., 2017; Reimann et al., 2020). These findings are consistent with the observed large deficits in gait modification performance during swing-phase modifications in HOA relative to HYA, which often involved large hip ranges of motion. Additionally, a post-hoc analysis of individual gait modifications revealed that swing and swing-stance modifications comprised five of the six modifications eliciting the largest difference in performance between HOA and HYA. Reduced swing modification performance contrasts with performance noted during the stance modifications, which challenged strength more than balance because stance modification targets could be achieved during double-limb support. Consequently, modifications to the swing-phase of gait or dance steps may be critical to personalizing exercise programs based on individuals’ motor function during dance-based therapy (Bauby and Kuo, 2000; Kuo, 2007). Note that the trend toward reduced spatial modification performance in the MCI compared to the HOA group supported a potential effect of cognitive function on the ability to modulate spatial components of movement (Cohen et al., 2016; Rucco et al., 2017), which may emerge with a larger sample size.
Age-related declines in strength may impact individuals’ abilities to perform plantarflexion during stance modifications (Browne and Franz, 2018): multiple HOA and MCI participants were unable to generate sufficient plantarflexor moments to raise their heel during single-limb support in the relevé and piqué stance modifications. Plantarflexor muscle properties are altered and activation capacity is reduced in older adults, which may limit ankle torque production capacity during gait modifications (Morse et al., 2004; Conway and Franz, 2020). Dance-based movements that require plantarflexor moment generation during stance may, therefore, challenge a prominent age-related deficit in HOA and could be good targets for personalization during dance-based therapy.
Our assumption that differences in RMS performance between HYA and HOA stemmed primarily from differences in motor function was supported by worse performance on assessments of motor function (e.g., TUG, Four-Square Step Test) in HOA compared to HYA. However, some assessments involving aspects of cognitive function also differed between HYA and HOA (e.g., Body Position Spatial Task) and were correlated with spatial modification performance. Therefore, it is possible that differences in cognitive function also impact individuals’ abilities to modulate spatial aspects of gait.
Cognitive deficits may reduce the ability to accurately modulate temporal gait features
We observed a moderate effect toward individuals with MCI exhibiting worse performance on temporal gait modifications than those in the HOA group, suggesting that cognitive deficits associated with MCI reduce the ability to flexibly modulate temporal features of gait. Such deficits correspond to the inability to entrain stepping movements into rhythms during dance classes. For example, individuals with MCI exhibited worse performance for 6-step temporal sequences (vs. 2-step or 4-step sequences), particularly during the complex duple modifications. Accurately performing longer sequences places greater demands on working memory, which is reduced in individuals with MCI (see Reverse Corsi Blocks Test in Table 2, Vandierendonck et al., 2004; Montero-Odasso et al., 2012). An alternative explanation for poor performance on some temporal modifications is that reduced motor-cognitive integration in individuals with MCI may limit individuals’ abilities to use the musical cues to adopt the proper timing for the assigned movement (Kluger et al., 1997; Hackney et al., 2017).
Waltz modifications represented a surprising case in which individuals with MCI performed modifications more accurately than HOA. Specifically, individuals with MCI performed the quick-Slow (qS) modification better than HOA (post-hoc analysis: Cohen’s d = 2.8). Waltz sequences were either two- or four-step, reducing their challenge to working memory compared to the six-step Duple sequences and possibly making these modifications easier for individuals with MCI (Montero-Odasso et al., 2012). Additionally, the three-count of Waltz consisted of one quick (q; 33% of the stride) and one Slow (S; 67% of the stride) step in each measure. This division of 33% and 67% of a stride is similar to the approximate percentages of the swing (40%) and stance (60%) phases during normal walking, respectively (Winter, 1984). Consequently, Waltz may have represented a smaller deviation from normal walking, enabling greater automaticity and reduced cortical demand than Duple modifications (Clark, 2015). Increased automaticity, however, would only explain a lack of deficit in Waltz performance in individuals with MCI. Better Waltz performance in MCI compared to HOA may stem from differences in music or dance experience, which represents an interesting area of future research.
Our decision to use fixed tempi for all participants is important for our interpretation of differences in temporal modification performance. Using a fixed tempo, rather than setting the tempi relative to each participant’s preferred cadence (Moumdjian et al., 2019), ensured that all participants had the same amount of time to perceive and respond to the musical cues. Personalizing the tempi to cadence would likely reduce differences in performance due to cognitive function because individuals with slower cadences would have more time to perceive the musical cues and determine motor commands to execute. Rather, we selected tempi near 100 bpm, below most participants’ preferred cadences. Therefore, differences in temporal modification performance are likely driven by participants’ abilities to perceive cues and step in the correct patterns, rather than their physical capacity to match the tempi. This expectation is supported by HYA performing Waltz modifications with similar accuracy to HOA, despite stepping at a roughly 30% faster tempo. Such a fast cadence could increase demands on motor function but did not obviously reduce HYA performance. Future studies can build upon our current findings to evaluate the effect of varying tempi and movement speeds.
Motor and cognitive deficits reduced the ability to perform spatiotemporal modifications in individuals with MCI
Large deficits in spatiotemporal performance in individuals with MCI compared to HOA were expected and further emphasize the impacts of cognitive deficits on the ability to voluntarily modulate gait biomechanics. Spatiotemporal gait modifications appear to elicit larger differences in movement performance between HOA and MCI than spatial or temporal modifications. Motor-cognition is adversely affected by MCI, and the requirement to modulate both spatial and temporal parameters affected those with MCI more than HOA (Hackney et al., 2017). Additionally, reduced set-shifting capacity in MCI compared to HOA—revealed by large differences in the A and B components of the Trail Making Task (Bowie and Harvey, 2006)—may explain reduced spatiotemporal performance in MCI. For example, individuals with MCI may struggle to identify tempos in the music while remembering gait modifications. Participants were instructed to equally prioritize the temporal or spatial components of the spatiotemporal gait modifications. However, some participants in the MCI group reported “giving up” on either the spatial or temporal components of the modifications. Future investigation into which aspects of RMS gait modifications are prioritized with age and cognitive decline may provide further insight into mechanisms impacting specific features of movement performance (Earhart, 2013).
Potential correlates of RMS performance
Our results show that spatial, temporal, and spatiotemporal gait modifications may be correlated with assessments of visuospatial working memory (Reverse Corsi Blocks), motor-cognitive integration, balance and mobility (Four-Square Step Test), and whole body spatial memory (Body Position Spatial Task; Dite and Temple, 2002; Vandierendonck et al., 2004; Hackney et al., 2013). These correlations suggest potential motor and cognitive contributions to spatial and temporal aspects of RMS performance and may be useful as design variables for dance-based therapy classes. However, these findings should be considered with extreme caution because in this preliminary study, we were not able to rigorously validate the robustness of these correlations, which will be a valuable focus of future larger-sample studies. Our findings here can pilot future trials with larger sample sizes and specific hypotheses about which aspects of motor or cognitive function impact RMS performance.
Clinical implications
Beyond advancing our understanding of how age and cognition impact the ability to flexibly modulate gait, our findings have implications for dance-based therapies for individuals with MCI. Our results showing distinct motor control deficits related to aging vs. cognitive impairment inform clinical understanding of the mechanisms underlying impaired gait performance in HOA and MCI. Creative dance-based movement therapies aim to challenge both motor and cognitive function and have shown positive impacts on spatial cognition, executive function, balance, and mobility in individuals with Parkinson’s and MCI (Hackney et al., 2007a,b; McKee and Hackney, 2013; Zhu et al., 2020). Our results show that different temporal features of music and spatial components of movements challenge distinct motor (e.g., strength or balance) and cognitive (e.g., working memory, set-shifting, or motor-cognitive integration) features (Thom and Clare, 2011; Montero-Odasso et al., 2012; Earhart, 2013; Browne and Franz, 2018). Consequently, personalizing music and dance selections during therapy based on an individual’s motor and cognitive function may maximize therapeutic effects. Specifically, selecting music and dance parameters that optimize the level of challenge for an individual may maximize benefits while retaining motivation (Guadagnoli and Lee, 2004; Hackney et al., 2007a). For example, our results suggest that teaching spatial or temporal modifications alone may be an appropriate level of challenge for individuals with MCI, before progressing to spatiotemporal modifications (Hackney and Earhart, 2010). However, we evaluated only the immediate relationship between age-related declines in motor and cognitive function and RMS performance. With repeated practice, individuals with MCI may improve RMS performance levels to near those of HOA or HYA participants (McKee and Hackney, 2013). These learning or longitudinal effects are beyond the scope of this study but are interesting areas of future research that could inform personalized design and data-driven progression of dance-based and music-based movement therapies.
Limitations
The following limitations constrain the generalizability of our findings. First, our modest sample size of twelve participants per group lacked the statistical power to identify all differences in movement performance, particularly during temporal gait modifications. Nonetheless, our data revealed differences in movement performance for spatial and spatiotemporal gait modifications and showed moderate differential effect sizes (HOA vs. MCI) during temporal modification performance. Secondly, while protocol administrators verified that participants understood each gait modification similarly before collecting data, understanding may have improved over the first few trials, potentially improving performance in later trials. However, we used a block randomization strategy to account for changes in comprehension of the movements.
Our findings should be viewed in the context of a broader set of constructs—including and beyond the motor and cognitive constraints studied here—that could impact movement performance during movement therapy. The specific gait modifications selected sensory stimuli (music type or tempo). walking speed, or even individuals’ relationships to music and dance could impact RMS performance. For example, music with an easier-to-perceive downbeat may alter the observed relationships between cognitive function and spatiotemporal RMS performance in individuals with lesser music experience. Our group-level analyses across diverse movement classes provide an early step toward understanding how dance-based RMS challenge specific aspects of motor and cognitive function. Larger sample sizes would enable the discovery of specific factors underlying RMS performance in individuals with MCI to be discovered from a broader set of constructs affecting movement.
Conclusions
We developed and implemented an innovative RMS gait modifications protocol to evaluate the impacts of age-related declines in motor and cognitive function on the ability to accurately modulate spatial and temporal features of gait. By improving our understanding of motor and cognitive impacts on the ability to modulate gait, this study represents an early step toward systematically personalized dance-based movement therapies. Furthermore, this study lays the foundation for future evaluation of the ability of RMS to: (1) help diagnose cognitive-motor impairments by providing insight into mechanisms of movement performance deficits; and (2) track intervention efficacy by quantifying changes in the accuracy of spatial and temporal features of gait modifications following therapy. Future studies identifying clinical and mechanistic predictors of RMS performance will advance our ability to provide quantitative metrics that assist clinicians in selecting movement therapy parameters to maximize treatment efficacy.
Data availability statement
The original contributions presented in the study are included in the article/Supplementary material, further inquiries can be directed to the corresponding author.
Ethics statement
The studies involving human participants were reviewed and approved by Emory University Institutional Review Board. The patients/participants provided their written informed consent to participate in this study.
Author contributions
MR, MH, JM, LE, and TK obtained funding for the study. MH, TK, LE, MR, and AS defined the study design and data collection protocol. MR, AS, and KC were skilled assessors who performed data collection. MH, JM, TK, LE, and MR supervised the study and planned data analysis. MR and JM performed data analysis. MR drafted the original manuscript. All authors provided intellectual critique and manuscript revisions. All authors contributed to the article and approved the submitted version.
Funding
Research reported in this manuscript was supported by the National Institute of Child Health and Human Development and the National Institute on Aging of the National Institutes of Health under award numbers F32HD108927 and R01AG062691, respectively. This research was also supported by Emory University through a Goizueta Alzheimer’s Disease Research Center CEP Innovation Accelerator Seed Grant, an award from the Emory University Senior Vice President of Research at the Intersection Fund.
Acknowledgments
We thank the participation of our volunteers, and T. Prusin and C. Carroll-Sauer for their assistance in data collection and participant recruitment.
Conflict of interest
The authors declare that the research was conducted in the absence of any commercial or financial relationships that could be construed as a potential conflict of interest.
Publisher’s note
All claims expressed in this article are solely those of the authors and do not necessarily represent those of their affiliated organizations, or those of the publisher, the editors and the reviewers. Any product that may be evaluated in this article, or claim that may be made by its manufacturer, is not guaranteed or endorsed by the publisher.
Supplementary material
The Supplementary Material for this article can be found online at: https://www.frontiersin.org/articles/10.3389/fnhum.2023.1040930/full#supplementary-material.
References
Bauby, C. E., and Kuo, A. D. (2000). Active control of lateral balance in human walking. J. Biomech. 33, 1433–1440. doi: 10.1016/s0021-9290(00)00101-9
Booth, F. W., Weeden, S. H., and Tseng, B. S. (1994). Effect of aging on human skeletal muscle and motor function. Med. Sci. Sports Exerc. 26, 556–560.
Bowie, C. R., and Harvey, P. D. (2006). Administration and interpretation of the trail making test. Nat. Protoc. 1, 2277–2281. doi: 10.1038/nprot.2006.390
Browne, M. G., and Franz, J. R. (2018). More push from your push-off: joint-level modifications to modulate propulsive forces in old age. PLoS One 13:e0201407. doi: 10.1371/journal.pone.0201407
Clark, D. J. (2015). Automaticity of walking: functional significance, mechanisms, measurement and rehabilitation strategies. Front. Hum. Neurosci. 9:246. doi: 10.3389/fnhum.2015.00246
Cohen, J. A., Verghese, J., and Zwerling, J. L. (2016). Cognition and gait in older people. Maturitas 93, 73–77. doi: 10.1016/j.maturitas.2016.05.005
Conway, K. A., and Franz, J. R. (2020). Shorter gastrocnemius fascicle lengths in older adults associate with worse capacity to enhance push-off intensity in walking. Gait Posture 77, 89–94. doi: 10.1016/j.gaitpost.2020.01.018
Dite, W., and Temple, V. A. (2002). A clinical test of stepping and change of direction to identify multiple falling older adults. Arch. Phys. Med. Rehabil. 83, 1566–1571. doi: 10.1053/apmr.2002.35469
Earhart, G. M. (2013). Dynamic control of posture across locomotor tasks. Mov. Disord. 28, 1501–1508. doi: 10.1002/mds.25592
Gauthier, S., Reisberg, B., Zaudig, M., Petersen, R. C., Ritchie, K., Broich, K., et al. (2006). Mild cognitive impairment. Lancet 367, 1262–1270. doi: 10.1016/S0140-6736(06)68542-5
Geda, Y. E., Roberts, R. O., Knopman, D. S., Christianson, T. J. H., Pankratz, V. S., Ivnik, R. J., et al. (2010). Physical exercise, aging and mild cognitive impairment: a population-based study. Arch. Neurol. 67, 80–86. doi: 10.1001/archneurol.2009.297
Grahn, J. A. (2012). Neural mechanisms of rhythm perception: current findings and future perspectives. Top. Cogn. Sci. 4, 585–606. doi: 10.1111/j.1756-8765.2012.01213.x
Guadagnoli, M. A., and Lee, T. D. (2004). Challenge point: a framework for conceptualizing the effects of various practice conditions in motor learning. J. Motil. Behav. 36, 212–224. doi: 10.3200/JMBR.36.2.212-224
Hackney, M. E., and Earhart, G. M. (2009). Effects of dance on movement control in Parkinson’s disease: a comparison of argentine tango and american ballroom. J. Rehabil. Med. 41, 475–481. doi: 10.2340/16501977-0362
Hackney, M. E., and Earhart, G. M. (2010). Recommendations for implementing tango classes for persons with Parkinson disease. Am. J. Dance Ther. 32, 41–52. doi: 10.1007/s10465-010-9086-y
Hackney, M. E., Hall, C. D., Echt, K. V., and Wolf, S. L. (2013). Dancing for balance: feasibility and efficacy in oldest-old adults with visual impairment. Nurs. Res. 62, 138–143. doi: 10.1097/NNR.0b013e318283f68e
Hackney, M. E., Kantorovich, S., and Earhart, G. M. (2007a). A study on the effects of argentine tango as a form of partnered dance for those with Parkinson disease and the healthy elderly. Am. J. Dance Ther. 29, 109–127. doi: 10.1007/s10465-007-9039-2
Hackney, M. E., Kantorovich, S., Levin, R., and Earhart, G. M. (2007b). Effects of tango on functional mobility in Parkinson’s disease: a preliminary study. J. Neurol. Phys. Ther. 31, 173–179. doi: 10.1097/NPT.0b013e31815ce78b
Hackney, M. E., Nocera, J., Creel, T., Riebesell, M. D., and Kesar, T. (2017). “Exercise and balance in older adults with movement disorders,” in Locomotion and Posture in Older Adults, eds F. Barbieri and R. Vitório (Cham: Springer), 323–346. doi: 10.1007/978-3-319-48980-3_21
Hortobágyi, T., Rider, P., Gruber, A. H., and DeVita, P. (2016). Age and muscle strength mediate the age-related biomechanical plasticity of gait. Eur. J. Appl. Physiol. 116, 805–814. doi: 10.1007/s00421-015-3312-8
Ivanenko, Y. P., Cappellini, G., Dominici, N., Poppele, R. E., and Lacquaniti, F. (2005). Coordination of locomotion with voluntary movements in humans. J. Neurosci. 25, 7238–7253. doi: 10.1523/JNEUROSCI.1327-05.2005
Jones, C. J., Rikli, R. E., and Beam, W. C. (1999). A 30-s chair-stand test as a measure of lower body strength in community-residing older adults. Res. Q. Exerc. Sport 70, 113–119. doi: 10.1080/02701367.1999.10608028
Kluger, A., Gianutsos, J. G., Golomb, J., Ferris, S. H., George, A. E., Franssen, E., et al. (1997). Patterns of motor impairment in normal aging, mild cognitive decline and early Alzheimer’s disease. J. Gerontol. B Psychol. Sci. Soc. Sci. 52, P28–P39. doi: 10.1093/geronb/52b.1.p28
Kornysheva, K., and Diedrichsen, J. (2014). Human premotor areas parse sequences into their spatial and temporal features. eLife 3:e03043. doi: 10.7554/eLife.03043
Kuo, A. D. (2007). The six determinants of gait and the inverted pendulum analogy: a dynamic walking perspective. Hum. Mov. Sci. 26, 617–656. doi: 10.1016/j.humov.2007.04.003
Lazarou, I., Parastatidis, T., Tsolaki, A., Gkioka, M., Karakostas, A., Douka, S., et al. (2017). International ballroom dancing against neurodegeneration: a randomized controlled trial in Greek community-dwelling elders with mild cognitive impairment. Am. J. Alzheimers Dis. Other Demen. 32, 489–499. doi: 10.1177/1533317517725813
Lundin-Olsson, L., Nyberg, L., and Gustafson, Y. (1998). Attention, frailty and falls: the effect of a manual task on basic mobility. J. Am. Geriatr. Soc. 46, 758–761. doi: 10.1111/j.1532-5415.1998.tb03813.x
Mancini, M., and Horak, F. B. (2016). Potential of APDM mobility lab for the monitoring of the progression of Parkinson’s disease. Expert Rev. Med. Devices 13, 455–462. doi: 10.1586/17434440.2016.1153421
McKee, K. E., and Hackney, M. E. (2013). The effects of adapted tango on spatial cognition and disease severity in Parkinson’s disease. J. Mot. Behav. 45, 519–529. doi: 10.1080/00222895.2013.834288
Montero-Odasso, M., Muir, S. W., and Speechley, M. (2012). Dual-task complexity affects gait in people with mild cognitive impairment: the interplay between gait variability, dual tasking and risk of falls. Arch. Phys. Med. Rehabil. 93, 293–299. doi: 10.1016/j.apmr.2011.08.026
Morris, S., Morris, M. E., and Iansek, R. (2001). Reliability of measurements obtained with the timed “Up & Go” test in people with Parkinson disease. Phys. Ther. 81, 810–818. doi: 10.1093/ptj/81.2.810
Morse, C. I., Thom, J. M., Davis, M. G., Fox, K. R., Birch, K. M., and Narici, M. V. (2004). Reduced plantarflexor specific torque in the elderly is associated with a lower activation capacity. Eur. J. Appl. Physiol. 92, 219–226. doi: 10.1007/s00421-004-1056-y
Moumdjian, L., Moens, B., Maes, P. J., Van Nieuwenhoven, J., Van Wijmeersch, B., Leman, M., et al. (2019). Walking to music and metronome at various tempi in persons with multiple sclerosis: a basis for rehabilitation. Neurorehabil. Neural Repair 33, 464–475. doi: 10.1177/1545968319847962
Mueller, S. G., Weiner, M. W., Thal, L. J., Petersen, R. C., Jack, C., Jagust, W., et al. (2005). The Alzheimer’s disease neuroimaging initiative. Neuroimaging Clin. N Am 15, 869–877. doi: 10.1016/j.nic.2005.09.008
Nasreddine, Z. S., Phillips, N. A., Bédirian, V., Charbonneau, S., Whitehead, V., Collin, I., et al. (2005). The montreal cognitive assessment, MoCA: a brief screening tool for mild cognitive impairment. J. Am. Geriatr. Soc. 53, 695–699. doi: 10.1111/j.1532-5415.2005.53221.x
Persad, C. C., Jones, J. L., Ashton-Miller, J. A., Alexander, N. B., and Giordani, B. (2008). Executive function and gait in older adults with cognitive impairment. J. Gerontol. A Biol. Sci. Med. Sci. 63, 1350–1355. doi: 10.1093/gerona/63.12.1350
Phillips, L. H., Wynn, V. E., McPherson, S., and Gilhooly, K. J. (2001). Mental planning and the tower of london task. Q. J. Exp. Psychol. A 54, 579–597. doi: 10.1080/713755977
Rallis, I., Doulamis, N., Doulamis, A., Voulodimos, A., and Vescoukis, V. (2018). Spatio-temporal summarization of dance choreographies. Comput. Graphics 73, 88–101. doi: 10.1016/j.cag.2018.04.003
Reimann, H., Ramadan, R., Fettrow, T., Hafer, J. F., Geyer, H., and Jeka, J. J. (2020). Interactions between different age-related factors affecting balance control in walking. Front. Sports Act. Living 2:94. doi: 10.3389/fspor.2020.00094
Rucco, R., Agosti, V., Jacini, F., Sorrentino, P., Varriale, P., De Stefano, M., et al. (2017). Spatio-temporal and kinematic gait analysis in patients with Frontotemporal dementia and Alzheimer’s disease through 3D motion capture. Gait Posture 52, 312–317. doi: 10.1016/j.gaitpost.2016.12.021
Sanford, A. M. (2017). Mild cognitive impairment. Clin. Geriatr. Med. 33, 325–337. doi: 10.1016/j.cger.2017.02.005
Schloemer, S. A., Thompson, J. A., Silder, A., Thelen, D. G., and Siston, R. A. (2017). Age-related differences in gait kinematics, kinetics and muscle function: a principal component analysis. Ann. Biomed. Eng. 45, 695–710. doi: 10.1007/s10439-016-1713-4
Shostakovich, D. (1938). “Waltz No. 2” from Suite for Jazz Orchestra No. 2. [CюиTa для джa3oBoгo opκecTpa№2]. Berlin: Boosey & Hawkes/Sikorski.
Styns, F., van Noorden, L., Moelants, D., and Leman, M. (2007). Walking on music. Hum. Mov. Sci. 26, 769–785. doi: 10.1016/j.humov.2007.07.007
Thom, J. M., and Clare, L. (2011). Rationale for combined exercise and cognition-focused interventions to improve functional independence in people with dementia. Gerontology 57, 265–275. doi: 10.1159/000322198
Vandierendonck, A., Kemps, E., Fastame, M. C., and Szmalec, A. (2004). Working memory components of the Corsi blocks task. Br. J. Psychol. 95, 57–79. doi: 10.1348/000712604322779460
Washabaugh, E. P., Kalyanaraman, T., Adamczyk, P. G., Claflin, E. S., and Krishnan, C. (2017). Validity and repeatability of inertial measurement units for measuring gait parameters. Gait Posture 55, 87–93. doi: 10.1016/j.gaitpost.2017.04.013
Wilson, M., and Kwon, Y.-H. (2008). The role of biomechanics in understanding dance movement: a review. J. Dance Med. Sci. 12, 109–116.
Winter, D. A. (1984). Kinematic and kinetic patterns in human gait: variability and compensating effects. Hum. Mov. Sci. 3, 51–76. doi: 10.1016/0167-9457(84)90005-8
Zhu, Y., Wu, H., Qi, M., Wang, S., Zhang, Q., Zhou, L., et al. (2018). Effects of a specially designed aerobic dance routine on mild cognitive impairment. Clin. Interv. Aging 13, 1691–1700. doi: 10.2147/CIA.S163067
Keywords: mild cognitive impairment, dance therapy, gait analysis, biomechanics, music, rhythm, gait modifications
Citation: Rosenberg MC, Slusarenko A, Cao K, Mckay JL, Emmery L, Kesar TM and Hackney ME (2023) Motor and cognitive deficits limit the ability to flexibly modulate spatiotemporal gait features in older adults with mild cognitive impairment. Front. Hum. Neurosci. 17:1040930. doi: 10.3389/fnhum.2023.1040930
Received: 09 September 2022; Accepted: 19 January 2023;
Published: 15 February 2023
Edited by:
Jiao Liu, Fujian University of Traditional Chinese Medicine, ChinaReviewed by:
Augusto Fusco, Agostino Gemelli University Polyclinic (IRCCS), ItalyAmbra Bisio, University of Genoa, Italy
Copyright © 2023 Rosenberg, Slusarenko, Cao, Mckay, Emmery, Kesar and Hackney. This is an open-access article distributed under the terms of the Creative Commons Attribution License (CC BY). The use, distribution or reproduction in other forums is permitted, provided the original author(s) and the copyright owner(s) are credited and that the original publication in this journal is cited, in accordance with accepted academic practice. No use, distribution or reproduction is permitted which does not comply with these terms.
*Correspondence: Michael C. Rosenberg, mcrose3@emory.edu