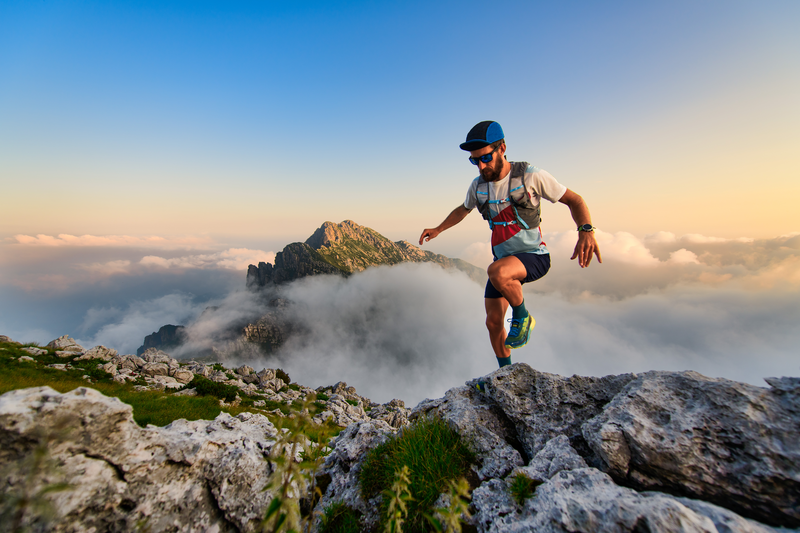
95% of researchers rate our articles as excellent or good
Learn more about the work of our research integrity team to safeguard the quality of each article we publish.
Find out more
ORIGINAL RESEARCH article
Front. Hum. Neurosci. , 03 October 2022
Sec. Cognitive Neuroscience
Volume 16 - 2022 | https://doi.org/10.3389/fnhum.2022.950893
This article is part of the Research Topic Exercise, Nutrition, and Cognitive Function: Implications on Health Promotion and Performance Improvement View all 11 articles
Physical activity is critical for maintaining cognitive and brain health. Previous studies have indicated that the effect of physical activity on cognitive and brain function varies between individuals. The present study aimed to examine whether mind wandering modulated the relations between physical activity and resting-state hippocampal functional connectivity. A total of 99 healthy adults participated in neuroimaging data collection as well as reported their physical activity in the past week and their propensity to mind wandering during typical activities. The results indicated that mind wandering was negatively related to the resting-state functional connectivity between hippocampus and right inferior occipital gyrus. Additionally, for participants with higher level of mind wandering, physical activity was negatively related to hippocampal connectivity at left precuneus and right precentral gyrus. In contrast, such relations were positive at right medial frontal gyrus and bilateral precentral gyrus for participants with lower level of mind wandering. Altogether, these findings indicated that the relations between physical activity and hippocampal functional connectivity vary as a function of mind wandering level, suggesting that individual differences are important to consider when we aim to maintain or improve cognitive and brain health through increasing physical activity.
There is no doubt that physical activity is critical for maintaining cognitive and brain health throughout the lifespan (Hillman et al., 2008; Duzel et al., 2016). Physical activity is also known as a “medicine” due to its protective effects against cognitive declines (American College of Sports Medicine, 2009). Many studies have found that increased physical activity is associated with better cognitive performance, such as attention, visuospatial processing, cognitive control, and memory (Colcombe and Kramer, 2003; Donnelly et al., 2016; de Greeff et al., 2018). However, the neural mechanisms underlying the effect of physical activity on cognitive functions are still not clear. To address the issue, many neuroimaging studies have focused on the changes in neural structure and function associated with physical activity (Colcombe et al., 2004, 2006; Pontifex et al., 2011; Chen et al., 2019; Horowitz et al., 2020). Of all the documented brain structure and functional changes that responds to physical activity, robust changes have been observed in the hippocampus (Clark et al., 2012; Prakash et al., 2015; Rendeiro and Rhodes, 2018).
Hippocampus plays a critical role in integrating information during learning, memory consolidation, and retrieval (Tulving and Markowitsch, 1998; Lavenex and Lavenex, 2013). It has been shown in rodent models that increases in physical activity are associated with increased hippocampal neurogenesis (van Praag et al., 2005) and enhanced hippocampal long-term potentiation (van Praag et al., 1999; Liu et al., 2011). Moreover, physical activity has been found to modify the hippocampus at the molecular and morphological level (van Praag et al., 1999; Stranahan et al., 2007; Liu et al., 2011), and these modifications are thought to contribute to the improvements in hippocampal-dependent memory, learning, and other cognitive functions (Rendeiro and Rhodes, 2018).
Similarly, researchers also sought to understand the influence of physical activity on the hippocampus in humans (Erickson et al., 2009, 2011; Ruscheweyh et al., 2011; Chaddock et al., 2016). For instance, randomized controlled clinical trials indicated that people with higher physical activity or better fitness showed greater hippocampal volume (Chaddock et al., 2010, 2016). Such exercise-related changes in hippocampal volume were related to subsequent changes in memory performance (Erickson et al., 2009, 2011; Maass et al., 2015). The profound effect of physical activity on hippocampal structure has led researchers to explore how physical activity may impact hippocampal function, as measured by brain activation (Pereira et al., 2007; Burdette et al., 2010; Voelcker-Rehage et al., 2011), and functional connectivity between the hippocampus and other brain regions (Burdette et al., 2010; Chaddock et al., 2010; Voss et al., 2010; Prakash et al., 2011; Ikuta et al., 2019). For example, level of vigorous physical activity was significantly associated with right hippocampal-orbitofrontal connectivity during resting state (Ikuta et al., 2019). Furthermore, an intervention study indicated that 12-month physical exercise training in old adults increased the negative connection between prefrontal regions and anterior left hippocampus region during resting state (Voss et al., 2010). Another study found that the group who received 4-month aerobic exercise training showed greater connectivity between hippocampus and anterior cingulate cortex than the control group (Burdette et al., 2010).
However, whether individuals can get cognitive benefits from physical activity and how much benefit they can get vary from person to person (Madden et al., 1989; Rhodes and Smith, 2006; Fedewa and Ahn, 2011). The routes by which physical activity affects learning and memory are complex and may likely be moderated by many factors, including age, gender, health status, cognitive level, and numerous psychosocial factors (Tomporowski et al., 2011). For example, a meta-analysis study showed that children of different ages may get various degrees of cognitive benefits from physical activity, indicating that age may be an important moderator of the benefits of physical activity (Benjamin and Jennifer, 2003). Moreover, the relations of physical activity to cognitive functions and hippocampal volume differ by gender, with females obviously benefiting from physical activity to a greater extent than males (Barha et al., 2020). Therefore, before physical activity can be prescribed as “medicine” for the brain, it is important to better understand the factors contributing to this variation.
Mind wandering, as an important cognitive function supported by hippocampus (Faber and Mills, 2018; Mills et al., 2018), may moderate the relations of physical activity to learning and memory. Mind wandering is defined as thoughts that were not tied to concurrent perceptions, which occurs when the attention shifts away from the present situation to one’s inner thought (Singer, 1966; Smallwood et al., 2003). The resting-state functional connectivity within default mode network (DMN), within which the hippocampus is a node, has been shown to be closely related to self-generated thoughts that involved in mind wandering (Christoff et al., 2009; Andrews-Hanna, 2011; Xu et al., 2014; Ellamil et al., 2016; Mittner et al., 2016). For example, hippocampus was more activated during mind wandering than at rest, suggesting that mind wandering may induce the activation of hippocampus (Xu et al., 2014).
Furthermore, previous studies also suggested that mind wandering was related to future physical activity (Smallwood and Andrews-Hanna, 2013; Fanning et al., 2016). For instance, present-moment mind wandering was positively associated with future moderate-to-vigorous physical activity, indicating that the nature of one’s mind wandering may impact the ability to plan for or engage in the goal directed behavior (Fanning et al., 2016). Although few studies have directly tested the relations between physical activity and mind wandering, previous studies have repeatedly found that increasing physical activity improves sustained attention (Kumar et al., 2015; Luque-Casado et al., 2015; Ciria et al., 2017). For example, an ERP study found that higher aerobic fitness was related to neuroelectric activity, demonstrating a better overall sustained attention and the ability to allocate attentional resources (Luque-Casado et al., 2015). Therefore, mind wandering, as a cognitive activity supported by hippocampus, is also closely related to physical activity.
To sum, previous studies have shown that physical activity, mind wandering and hippocampal functions are related to each other (Voelcker-Rehage et al., 2011; Smallwood and Andrews-Hanna, 2013; Faber and Mills, 2018). Specifically, physical activity not only has positive impact on hippocampal functions, but also affects mind wandering (Luque-Casado et al., 2015; Chaddock et al., 2016). Additionally, mind wandering also affects hippocampal activity due to its role in inducing hippocampal activation (Xu et al., 2014; Ellamil et al., 2016). However, it is still unknown that whether mind wandering plays a role in the effect of physical activity on hippocampal functions. To provide insight into this question, the purpose of this study is to test whether mind wandering modulated the relations between physical activity and hippocampal functional connectivity measured during resting state. Specifically, we hypothesized that the relations between physical activity and hippocampal functional connectivity vary as a function of individual’s mind wandering level.
A total of 105 college students were recruited from Zhejiang University at Hangzhou in China (mean age = 22.78, SD = 2.91, 49 female). Six participants were excluded from final analyses due to excessive head motion during fMRI scanning (n = 1) or incomplete questionnaires (n = 5). All participants were healthy without adverse health conditions, physical disabilities, or neurological disorders. This study was approved by the research ethics review board of Zhejiang University. Participants signed consent forms before participating in the study.
Participants completed the short version of International Physical Activity Questionnaire (IPAQ) to measure their physical activity level during the last 7 days (Hagströmer et al., 2006). The items in the questionnaire were structured to measure the volume of vigorous-intensity activity, moderate-intensity activity, and walking per week. These activities were weighted by their energy requirements defined in MET (Metabolic Equivalent Task) to generate a score in MET-minutes, which is calculated by multiplying the MET score of an activity by the minutes performed. Total physical activity (MET-min/week) was calculated by the summation of vigorous, moderate activity, and walking in MET-minutes over a week. This summation score was used as a continuous variable to measure the physical activity level of participants in the current study.
The Mind Wandering Questionnaire (MWQ) (Mrazek et al., 2013) is a 5-item self-report scale, which was used to measure the propensity to mind wandering during typical activities (Cronbach’s α = 0.85). We used the Chinese version of the MWQ, which was verified to be a suitable tool to measure the trait level of mind-wandering (Luo et al., 2016). The questionnaire is a 6-point Likert scale, ranging from 1 (Never) to 6 (Always), with participants rating these items based on how often they experienced the particular situation (e.g., “I find myself listening with one ear, thinking about something else at the same time” or “I mind-wander during lectures or presentations”). Higher scores represent greater propensity to mind wandering.
Participants were scanned in a Siemens 3.0T scanner (MAGNETOM Prisma, Siemens Healthcare, Erlangen, Germany) with a 20-channel coil in the Brain Imaging Science and Technology Center at Huajiachi Campus of Zhejiang University. They were asked to maintain their gaze at the fixation square in the center of the screen while they could blink as usual. Additionally, we explained to participants how subtle movements could affect data quality and asked them to remain as still as a statue to minimize head movement. The high-resolution structural images were acquired using a T1-weighted magnetization prepared-rapid gradient-echo sequence with the following parameters: TR = 2300 ms, TE = 2.32 ms, slice thickness = 0.9 mm, voxel size = 0.90 × 0.90 × 0.90 mm3, voxel matrix = 256 × 256, flip angle = 8°, and field of view = 240 mm2, duration of 7 min and 26 s. Then, a total of 480 whole-brain resting-state volumes were collected using a T2-weighted gradient echo planar imaging sequence: TR = 1000 ms, TE = 34 ms, slice thickness = 2.5 mm, voxel size = 2.50 × 2.50 × 2.50 mm3, voxel matrix = 92 × 92, flip angle = 50°, field of view = 230 mm2, slices = 52, and duration of 8 min.
The following steps were carried out to preprocess the data: (1) Slice timing correction and head motion correction were performed using AFNI.1 (2) Tissue segmentation was conducted to extract brains using SPM12.2 ANTs3 was used to co-register and normalize structural and functional images from original space to MNI space. (3) All functional images were spatially smoothed using a 5 mm full-width-at-half-maximum Gaussian kernel. (4) Nuisance variable regression was conducted using six-rigid head motion and their forward derivate as well as the first five principal components from white matter and cerebral spinal fluid (CSF) separately. (5) A band-pass filtering (0.01–0.1 Hz) was applied.
Since the resting-state functional connectivity could be influenced by small volume-to-volume head movements, we first calculated the framewise displacement (FD) of each volume to quantify the head motion. Any volume with FD ≥ 0.5 mm as well as 1 back and 1 forward volumes were scrubbed to minimize the effect of head motion. The mean FD of all participants included in the final statistical analyses was from 0.09 to 0.30 (mean FD = 0.153, SD = 0.038) with data length ≥ 7 min.
Then we performed resting-state functional connectivity analyses by using AFNI. We obtained the hippocampal seed regions from the Harvard–Oxford subcortical structure probabilistic atlas4 thresholded at 25%. With the uncal apex served as the border between anterior and posterior hippocampus (Duvernoy, 2005), the hippocampus was divided into anterior and posterior segments using manual identification of standard anatomical landmarks by using 1-mm MNI152 template.5 Therefore, left anterior, right anterior, left posterior, and right posterior hippocampus were used as seed regions. The functional connectivity between the time series of the seed regions and the other regions throughout the whole brain was calculated to generate the individual resting-state functional connectivity map (r-map). Then, by using Fisher’s r-to-z transformation, the r-maps were converted into z-maps to obtain the normally distributed values of the connectivity maps.
Statistical analyses were conducted using 3dLME program within AFNI. To identify whether there were interactions between physical activity and mind wandering in predicting the functional connectivity between hippocampus and other brain regions, we added physical activity, mind wandering, and their interaction as independent variables in the fixed effect model. Since previous studies have found functional separation of hippocampus in different subregions and hemispheres (Strange et al., 1999; Iglói et al., 2010; Shipton et al., 2014; Robinson et al., 2015; Persson et al., 2018), we included the Subregions (anterior vs. posterior) and Hemispheres (left vs. right) as within-subject covariables. Random effects were also added to the model. The 3dClustSim in AFNI indicated that when puncorrected < 0.001, only clusters with a minimum of 23 voxel size were viewed as significant with multiple comparison correction (puncorrected < 0.001). We mainly reported the results involving physical activity, mind wandering, or both.
Table 1 showed the characteristics of participants who contributed both fMRI and questionnaires data. There was no significant correlation between physical activity and mind wandering (p = 0.094). Additionally, both of them were not significant related to mean FD (r = −0.048, p = 0.637; r = −0.004, p = 0.966). Therefore, mean FD was not included as covariate when we tested brain-behavioral relations below.
There was a significant main effect of mind wandering at right inferior occipital gyrus (Cluster size = 30; x = 45, y = −65, z = −14), suggesting that mind wandering was negatively related to the resting-state functional connectivity between hippocampus and right inferior occipital gyrus (Figure 1). Physical activity was not significantly related to hippocampal functional connectivity at any brain region. However, there were interactions between physical activity and mind wandering in predicting hippocampal functional connectivity at left precuneus, precentral gyrus, left superior frontal gyrus, and right medial frontal gyrus (Table 2).
Figure 1. Mind wandering was negatively correlated with functional connectivity between hippocampus and right inferior occipital gyrus.
To understand these interactions, we separated all participants into high (48 subjects, 22 females, mean age = 22.71, SD = 2.75) and low groups (51 subjects, 27 females, mean age = 22.75, SD = 3.05) according to the mean scores of their mind wandering (i.e., 16.73). Then, for each group, we conducted whole-brain search analyses to test whether physical activity was related to hippocampal functional connectivity at each brain region showing significant interaction. The results indicated that in high mind wandering group, physical activity was negatively related to hippocampal functional connectivity at left precuneus and right precentral gyrus (Figure 2). In contrast, in low mind wandering group, there were positive correlations between physical activity and hippocampal functional connectivity at right medial frontal gyrus, right precentral gyrus, and left precentral gyrus (Figure 3 and Table 3). However, the relations between physical activity and hippocampal functional connectivity at left superior frontal gyrus did not survive from multiple comparison correction in both low and high mind wandering groups.
Figure 2. Negative relations between physical activity and hippocampal functional connectivity at left precuneus and right precentral gyrus in high mind wandering group.
Figure 3. Positive relations between physical activity and hippocampal functional connectivity at right medial frontal gyrus, right precentral gyrus, and left precentral gyrus in low mind wandering group.
Table 3. Mind wandering modulated the relationship between physical activity and hippocampal functional connectivity.
The current study aimed to examine whether mind wandering modulated the relations between physical activity and hippocampal functional connectivity. First, we found that mind wandering was negatively related to the resting-state functional connectivity between hippocampus and right inferior occipital gyrus. Additionally, we found there was significant interaction between physical activity and mind wandering in predicting the resting-state hippocampal functional connectivity at left precuneus, precentral gyrus, left superior frontal gyrus, and right medial frontal gyrus. Specifically, for participants with higher level of mind wandering, there was negative relation between physical activity and hippocampal functional connectivity at left precuneus and right precentral gyrus. For participants with lower level of mind wandering, physical activity was positively related to hippocampal functional connectivity at right medial frontal gyrus and bilateral precentral gyrus. These findings supported our hypothesis that mind wandering modulated the relations between physical activity and hippocampal functional connectivity.
Our study found the significant interaction between physical activity and mind wandering in predicting hippocampal functional connectivity at left precuneus, right medial frontal gyrus, and bilateral precentral gyrus. These brain regions, similar to hippocampus, are closely related to physical activity or/and mind wandering (Schneider et al., 2009; Vago and David, 2012; Boccia et al., 2015; Christoff et al., 2016; Thielen et al., 2016). For example, the DMN, which includes precuneus and medial frontal gyrus as nodes, has been shown to be positively activated during mind wandering (Buckner et al., 2008; Andrews-Hanna, 2011). Additionally, a meta-analysis study showed that compared with controls, the meditators, who would experience more mind wandering, had greater activation in precuneus, precentral gyrus, and medial frontal gyrus during meditation (Boccia et al., 2015).
Meanwhile, numerous studies also found that during both resting and task states, people with higher physical activity or fitness showed greater activation at precuneus, precentral gyrus, and medial frontal gyrus than people with lower physical activity or fitness (Casey et al., 2000; Schneider et al., 2009; Voelcker-Rehage et al., 2010; Smith et al., 2011; Kimura et al., 2013; Thielen et al., 2016). For example, compared to the controls, people who received aerobic physical activity intervention showed greater activation at precuneus (Thielen et al., 2016), precentral gyrus (Voelcker-Rehage et al., 2010; Smith et al., 2011), and medial frontal gyrus (Casey et al., 2000; Kimura et al., 2013) during performing tasks that were highly demanding. Altogether, previous studies suggest that left precuneus, right medial frontal gyrus, and bilateral precentral gyrus may co-activate with hippocampus to support mind wandering and the functions of these brain regions may also be influenced by physical activity.
Furthermore, we found that the relations between physical activity and hippocampal functional connectivity vary as a function of mind wandering level. Previous studies have indicated that maintaining appropriate mind wandering level is critical in our daily life (Smallwood and Andrews-Hanna, 2013). Too much mind wandering may cause us to be dissociated from the external environment, which may result in deteriorated task performance, such as the performance in working memory task (McVay and Kane, 2010) and reading comprehension task (Smallwood et al., 2008; Unsworth and McMillan, 2013). However, a certain amount of mind wandering is also beneficial in some situations. For example, we also benefit from mind wandering in tasks or environments that require divergent thinking, such as creativity (Baird et al., 2012), problem solving (Smeekens and Kane, 2016), and successful management of long-term goals (Smallwood et al., 2013). Therefore, mind wandering enables us to reach balance in allocating attention to processing internal and external stimuli.
Previous studies also indicated that physical activity is related to the allocation of attentional resources (Hillman et al., 2006; Luque-Casado et al., 2015; Stillman et al., 2018). For example, physical activity had a larger effect on behavioral performance during task switch vs. repeat (Hillman et al., 2006), suggesting that physical activity affects the processing of information in tasks that have great demands on attentional resource (Kramer et al., 1999; Colcombe and Kramer, 2003). Additionally, another ERP study indicated that fitness was positively related to the neuroelectric activity that measures sustained attention, suggesting that individuals with higher fitness are more capable of allocating attentional resources over time (Luque-Casado et al., 2015).
Therefore, in terms of the findings that individuals with high vs. low mind wandering showed opposite relations between physical activity and hippocampal functional connectivity, we propose that physical activity may help individuals maintain appropriate level of mind wandering through affecting the interactions between hippocampus and other brain regions. Specifically, for people with lower level of mind wandering, physical activity may help intensify the functional connectivity between brain regions within networks of mind wandering. In contrast, for people with higher level of mind wandering, physical activity may reduce the connectivity between regions of brain networks that support mind wandering (Stillman et al., 2018). Although such interpretation needs more studies to verify, we further propose that when physical activity is considered as a kind of “medicine,” individual differences are important to consider. In other words, the effect of physical activity may vary substantially between different people.
We found that mind wandering level was associated with the functional connectivity between hippocampus and right inferior occipital gyrus. The occipital gyrus participates in processing visual information and has been suggested to be important for object recognition (Gauthier et al., 2000; Sato et al., 2014; Jacques et al., 2019). Visual information is preliminarily processed and integrated in inferior frontal gyrus and then reprocessed in higher-level cognitive systems, such as the memory center for which hippocampus is a critical structure (Stone, 1983; Eichenbaum et al., 1992; Andersen et al., 2006). It has been suggested that compared to people with lower level of mind wandering, people with higher level of mind wandering focus more on processing internal information, but ignore external information or process it at superficial level without sending them to higher-order cognitive systems (i.e., hippocampal memory system) (Hasenkamp et al., 2012). As a result, for people with higher level of mind wandering, the connectivity between hippocampus and inferior occipital gyrus may become weaker compared to the ones with lower level of mind wandering.
Previous studies showed that physical activity, hippocampal function, and mind wandering were related to each other. Our study contributed to establishing the modulating role of mind wandering in the relations between physical activity and resting-state hippocampal functional connectivity. However, the current study still had several limitations. First, the study design did not allow us to test the causal relations between physical activity, mind wandering, and hippocampal functions. Additionally, physical activity and mind wandering were measured by self-reported, which is subjective and maybe biased (Randy et al., 2003). Therefore, future studies need to include more objective measurements and use study designs that allow us to test the causal effect of physical activity on mind wandering and the related brain functions.
To summarize, this study established the modulating role of mind wandering on the relations between physical activity and resting-state hippocampal functional connectivity at precuneus, precentral gyrus, and medial frontal gyrus. Specifically, for individuals with higher level of mind wandering, physical activity was negatively related to hippocampal functional connectivity at left precuneus and right precentral gyrus; in contrast, for individuals with lower level of mind wandering, physical activity was positively related to hippocampal functional connectivity at right medial frontal gyrus and bilateral precentral gyrus. We interpreted such findings as that physical activity may help maintain an appropriate level of mind wandering by affecting the interaction between hippocampus and other brain regions. Therefore, the current study provides insight into the variations between individuals on the relations between physical activity and brain functions, implying that individual differences are important to consider when we aim to maintain or improve neurocognitive health through increasing physical activity.
The raw data supporting the conclusions of this article will be made available by the authors, without undue reservation.
The studies involving human participants were reviewed and approved by Zhejiang University. The patients/participants provided their written informed consent to participate in this study.
DS drafted the initial manuscript, carried out data analysis, interpreted results, and critically revised the manuscript for important intellectual content. FG conceived and designed the study, contributed to acquisition, analysis and interpretation of the data, drafted, reviewed, and critically revised the manuscript for important intellectual content. XH contributed to data collection and critically revised the manuscript for important intellectual content. KH and YH contributed to conception of the study and critically revised the manuscript for important intellectual content. All authors approved the final manuscript as submitted.
This work was supported by the MOE (Ministry of Education in China) Project of Humanities and Social Sciences (20YJA190002), National Natural Science Foundation of China (General Program; 81971245), and the Fundamental Research Funds for the Central Universities.
We thank our participants and research assistants. Especially, we also thank Jiawen Zhang and Binyu Teng for helping with data collection.
The authors declare that the research was conducted in the absence of any commercial or financial relationships that could be construed as a potential conflict of interest.
All claims expressed in this article are solely those of the authors and do not necessarily represent those of their affiliated organizations, or those of the publisher, the editors and the reviewers. Any product that may be evaluated in this article, or claim that may be made by its manufacturer, is not guaranteed or endorsed by the publisher.
American College of Sports Medicine (2009). ACSM’s exercise is medicine: A clinician’s guide to exercise prescription. Philadelphia, PA: Lippincott Williams and Wilkins.
Andersen, P., Morris, R., Amaral, D., Bliss, T., and O’Keefe, J. (2006). The hippocampus book. Oxford: Oxford University Press.
Andrews-Hanna, J. (2011). The brain’s default network and its adaptive role in internal mentation. Neuroscientist 18, 251–270. doi: 10.1177/1073858411403316
Baird, B., Smallwood, J., Mrazek, M. D., Kam, J. W. Y., Franklin, M. S., and Schooler, J. W. (2012). Inspired by distraction: Mind wandering facilitates creative incubation. Psychol. Sci. 23, 1117–1122. doi: 10.1177/0956797612446024
Barha, C. K., Best, J. R., Rosano, C., Yaffe, K., Catov, J. M., Liu-Ambrose, T., et al. (2020). Sex-specific relationship between long-term maintenance of physical activity and cognition in the health ABC study: Potential role of hippocampal and dorsolateral prefrontal cortex volume. J. Gerontol. A Biol. Sci. Med. Sci. 75, 764–770. doi: 10.1093/gerona/glz093
Benjamin, A. S., and Jennifer, L. E. (2003). The relationship between physical activity and cognition in children: A meta-analysis. Pediatr. Exerc. Sci. 15, 243–256. doi: 10.1123/pes.15.3.243
Boccia, M., Piccardi, L., and Guariglia, P. (2015). The meditative mind: A comprehensive meta-analysis of MRI studies. Bio. Med. Res. Int. 2015:419808. doi: 10.1155/2015/419808
Buckner, R. L., Andrews-Hanna, J. R., and Schacter, D. L. (2008). The brain’s default network. Ann. N. Y. Acad. Sci. 1124, 1–38. doi: 10.1196/annals.1440.011
Burdette, J. H., Laurienti, P. J., Espeland, M. A., Morgan, A., Telesford, Q., Vechlekar, C. D., et al. (2010). Using network science to evaluate exercise-associated brain changes in older adults. Front. Aging Neurosci. 2:23. doi: 10.3389/fnagi.2010.00023
Casey, B. J., Thomas, K. M., Welsh, T. F., Badgaiyan, R. D., Eccard, C. H., Jennings, J. R., et al. (2000). Dissociation of response conflict, attentional selection, and expectancy with functional magnetic resonance imaging. Proc. Natl. Acad. Sci. U.S.A. 97, 8728–8733. doi: 10.1073/pnas.97.15.8728
Chaddock, L., Erickson, I., Prakash, R. S., Kim, J. S., Voss, M. W., VanPatter, M., et al. (2010). A neuroimaging investigation of the association between aerobic fitness, hippocampal volume, and memory performance in preadolescent children. Brain Res. 1358, 172–183. doi: 10.1016/j.brainres.2010.08.049
Chaddock, L., Erickson, K., Chappell, M., Johnson, C., Kienzler, C., Knecht, A., et al. (2016). Aerobic fitness is associated with greater hippocampal cerebral blood flow in children. Dev. Cogn. Neurosci. 20, 52–58. doi: 10.1016/j.dcn.2016.07.001
Chen, K., Zheng, Y., Wei, J., Ouyang, H., Huang, X., Zhang, F., et al. (2019). Exercise training improves motor skill learning via selective activation of mTOR. Sci. Adv. 5:eaaw1888. doi: 10.1126/sciadv.aaw1888
Christoff, K., Gordon, A., Smallwood, J., Smith, R., and Schooler, J. (2009). Experience sampling during fMRI reveals default network and executive system contributions to mind wandering. Proc. Natl. Acad. Sci. U.S.A. 106, 8719–8724. doi: 10.1073/pnas.0900234106
Christoff, K., Irving, Z. C., Fox, K. C. R., Spreng, R. N., and Andrews-Hanna, J. R. (2016). Mind-wandering as spontaneous thought: A dynamic framework. Nat. Rev. Neurosci. 17, 718–731. doi: 10.1038/nrn.2016.113
Ciria, L. F., Perakakis, P., Luque-Casado, A., Morato, C., and Sanabria, D. (2017). The relationship between sustained attention and aerobic fitness in a group of young adults. PeerJ 5:e3831. doi: 10.7717/peerj.3831
Clark, P., Bhattacharya, T., Miller, D., Kohman, R., DeYoung, E., and Rhodes, J. (2012). New neurons generated from running are broadly recruited into neuronal activation associated with three different hippocampus-involved tasks. Hippocampus 22, 1860–1867. doi: 10.1002/hipo.22020
Colcombe, S. J., and Kramer, A. F. (2003). Fitness effects on the cognitive function of older adults: A meta-analytic study. Psychol. Sci. 14, 125–130. doi: 10.1111/1467-9280.t01-1-01430
Colcombe, S. J., Erickson, K. I., Scalf, P. E., Kim, J. S., Prakash, R., McAuley, E., et al. (2006). Aerobic exercise training increases brain volume in aging humans. J. Gerontol. A Biol. Sci. Med. Sci. 61, 1166–1170. doi: 10.1093/gerona/61.11.1166
Colcombe, S. J., Kramer, A. F., Erickson, K. I., Scalf, P., McAuley, E., Cohen, N. J., et al. (2004). Cardiovascular fitness, cortical plasticity, and aging. Proc. Natl. Acad. Sci. U.S.A. 101:3316. doi: 10.1073/pnas.0400266101
de Greeff, J. W., Bosker, R. J., Oosterlaan, J., Visscher, C., and Hartman, E. (2018). Effects of physical activity on executive functions, attention and academic performance in preadolescent children: A meta-analysis. J. Sci. Med. Sport 21, 501–507. doi: 10.1016/j.jsams.2017.09.595
Donnelly, J. E., Hillman, C. H., Castelli, D., Etnier, J. L., Lee, S., Tomporowski, P., et al. (2016). Physical activity. fitness, cognitive function, and academic achievement in children: A systematic review. Med. Sci. Sports Exerc. 48, 1197–1222. doi: 10.1249/MSS.0000000000000901
Duvernoy, H. M. (2005). The human hippocampus: Functional anatomy, vascularization and serial sections with MRI. Berlin: Springer Science and Business Media.
Duzel, E., van Praag, H., and Sendtner, M. (2016). Can physical exercise in old age improve memory and hippocampal function? Brain 139, 662–673. doi: 10.1093/brain/awv407
Eichenbaum, H., Otto, T., and Cohen, N. J. (1992). The hippocampus—what does it do? Behav. Neural Biol. 57, 2–36. doi: 10.1016/0163-1047(92)90724-I
Ellamil, M., Fox, K., Dixon, M., Pritchard, S., Todd, R., Thompson, E., et al. (2016). Dynamics of neural recruitment surrounding the spontaneous arising of thoughts in experienced mindfulness practitioners. Neuroimage 136, 186–196. doi: 10.1016/j.neuroimage.2016.04.034
Erickson, K. I., Prakash, R. S., Voss, M. W., Chaddock, L., Hu, L., Morris, K. S., et al. (2009). Aerobic fitness is associated with hippocampal volume in elderly humans. Hippocampus 19, 1030–1039. doi: 10.1002/hipo.20547
Erickson, K. I., Voss, M. W., Prakash, R. S., Basak, C., Szabo, A., Chaddock, L., et al. (2011). Exercise training increases size of hippocampus and improves memory. Proc. Natl. Acad. Sci. U.S.A. 108:3017. doi: 10.1073/pnas.1015950108
Faber, M., and Mills, C. (2018). The critical role of the hippocampus in mind wandering. J. Neurosci. 38, 6439–6441. doi: 10.1523/JNEUROSCI.0995-18.2018
Fanning, J., Mackenzie, M., Roberts, S., Crato, I., Ehlers, D., and McAuley, E. (2016). Physical activity, mind wandering, affect, and sleep: An ecological momentary assessment. JMIR Mhealth Uhealth 4:e104. doi: 10.2196/mhealth.5855
Fedewa, A. L., and Ahn, S. (2011). The effects of physical activity and physical fitness on children’s achievement and cognitive outcomes. Res. Q. Exerc. Sport 82, 521–535. doi: 10.1080/02701367.2011.10599785
Gauthier, I., Tarr, M. J., Moylan, J., Skudlarski, P., Gore, J. C., and Anderson, A. W. (2000). The fusiform “face area” is part of a network that processes faces at the individual level. J. Cogn. Neurosci. 12, 495–504. doi: 10.1162/089892900562165
Hagströmer, M., Oja, P., and Sjöström, M. (2006). The international physical activity questionnaire (IPAQ): A study of concurrent and construct validity. J. Public Health Nutr. 9, 755–762. doi: 10.1079/phn2005898
Hasenkamp, W., Wilson-Mendenhall, C. D., Duncan, E., and Barsalou, L. W. (2012). Mind wandering and attention during focused meditation: A fine-grained temporal analysis of fluctuating cognitive states. Neuroimage 59, 750–760. doi: 10.1016/j.neuroimage.2011.07.008
Hillman, C. H., Erickson, K. I., and Kramer, A. F. (2008). Be smart, exercise your heart: Exercise effects on brain and cognition. J. Nat. Rev. Neurosci. 9:58. doi: 10.1038/nrn2298
Hillman, C. H., Kramer, A. F., Belopolsky, A. V., and Smith, D. P. (2006). A cross-sectional examination of age and physical activity on performance and event-related brain potentials in a task switching paradigm. Int. J. Psychophysiol. 59, 30–39. doi: 10.1016/j.ijpsycho.2005.04.009
Horowitz, A. M., Fan, X., Bieri, G., Smith, L. K., Sanchez-Diaz, C. I., Schroer, A. B., et al. (2020). Blood factors transfer beneficial effects of exercise on neurogenesis and cognition to the aged brain. Science 369:167. doi: 10.1126/science.aaw2622
Iglói, K., Doeller, C. F., Berthoz, A., Rondi-Reig, L., and Burgess, N. (2010). Lateralized human hippocampal activity predicts navigation based on sequence or place memory. Proc. Natl. Acad. Sci. U.S.A. 107, 14466–14471. doi: 10.1073/PNAS.1004243107
Ikuta, T., Frith, E., Ponce, P., and Loprinzi, P. D. (2019). Association of physical activity on the functional connectivity of the hippocampal–orbitofrontal pathway. Phys. Sportsmed. 47, 290–294. doi: 10.1080/00913847.2018.1549461
Jacques, C., Jonas, J., Maillard, L., Colnat-Coulbois, S., Koessler, L., and Rossion, B. (2019). The inferior occipital gyrus is a major cortical source of the face-evoked N170: Evidence from simultaneous scalp and intracerebral human recordings. Hum. Brain Mapp. 40, 1403–1418. doi: 10.1002/hbm.24455
Kimura, K., Yasunaga, A., and Wang, L.-Q. (2013). Correlation between moderate daily physical activity and neurocognitive variability in healthy elderly people. Arch. Gerontol. Geriatr. 56, 109–117. doi: 10.1016/j.archger.2012.10.004
Kramer, A. F., Hahn, S., Cohen, N. J., Banich, M. T., McAuley, E., Harrison, C. R., et al. (1999). Ageing. fitness and neurocognitive function. Nature 400, 418–419. doi: 10.1038/22682
Kumar, N., Wheaton, L. A., Snow, T. K., and Millard-Stafford, M. (2015). Exercise and caffeine improve sustained attention following fatigue independent of fitness status. Fatigue Biomed. Health Behav. 3, 104–121. doi: 10.1080/21641846.2015.1027553
Lavenex, P., and Lavenex, P. (2013). Building hippocampal circuits to learn and remember: Insights into the development of human memory. Behav. Brain Res. 254, 8–21. doi: 10.1016/j.bbr.2013.02.007
Liu, H., Zhao, G., Cai, K., Zhao, H., and Shi, L. (2011). Treadmill exercise prevents decline in spatial learning and memory in APP/PS1 transgenic mice through improvement of hippocampal long-term potentiation. Behav. Brain Res. 218, 308–314. doi: 10.1016/j.bbr.2010.12.030
Luo, Y., Zhu, R., Ju, E., and You, X. (2016). Validation of the Chinese version of the mind-wandering questionnaire (MWQ) and the mediating role of self-esteem in the relationship between mind-wandering and life satisfaction for adolescents. Pers. Individ. Differ. 92, 118–122. doi: 10.1016/j.paid.2015.12.028
Luque-Casado, A., Perakakis, P., Hillman, C., Kao, S.-C., Llorens, F., Guerra, P., et al. (2015). Differences in sustained attention capacity as a function of aerobic fitness. Med. Sci. Sports Exerc. 48, 887–895. doi: 10.1249/MSS.0000000000000857
Maass, A., Düzel, S., Goerke, M., Becke, A., Sobieray, U., Neumann, K., et al. (2015). Vascular hippocampal plasticity after aerobic exercise in older adults. Mol. Psychiatry 20, 585–593. doi: 10.1038/mp.2014.114
Madden, D., Blumenthal, J., Allen, P., and Emery, C. (1989). Improving aerobic capacity in healthy older adults does not necessarily lead to improved cognitive performance. Psychol. Aging 4, 307–320. doi: 10.1037/0882-7974.4.3.307
McVay, J. C., and Kane, M. J. (2010). Does mind wandering reflect executive function or executive failure? Comment on Smallwood and Schooler (2006) and Watkins (2008). Psychol. Bull. 136, 188–197. doi: 10.1037/a0018298
Mills, C., Herrera-Bennett, A., Faber, M., and Christoff, K. (2018). “Why the mind wanders: How spontaneous thought’s default variability may support episodic efficiency and semantic optimization,” in The oxford handbook of spontaneous thought: Mind-wandering, creativity, and dreaming, eds K. Christoff and K. C. R. Fox (Oxford: Oxford University Press), 11–22. doi: 10.1093/oxfordhb/9780190464745.013.42
Mittner, M., Hawkins, G. E., Boekel, W., and Forstmann, B. U. (2016). A neural model of mind wandering. Trends Cogn. Sci. 20, 570–578. doi: 10.1016/J.TICS.2016.06.004
Mrazek, M., Phillips, D., Franklin, M., Broadway, J., and Schooler, J. (2013). Young and restless: Validation of the mind-wandering questionnaire (MWQ) reveals disruptive impact of mind-wandering for youth. Front. Psychol. 4:560. doi: 10.3389/fpsyg.2013.00560
Pereira, A. C., Huddleston, D. E., Brickman, A. M., Sosunov, A. A., Hen, R., McKhann, G. M., et al. (2007). An in vivo correlate of exercise-induced neurogenesis in the adult dentate gyrus. Proc. Natl. Acad. Sci. U.S.A. 104, 5638–5643. doi: 10.1073/pnas.0611721104
Persson, J., Stening, E., Nordin, K., and Söderlund, H. (2018). Predicting episodic and spatial memory performance from hippocampal resting-state functional connectivity: Evidence for an anterior–posterior division of function. Hippocampus 28, 53–66. doi: 10.1002/HIPO.22807
Pontifex, M. B., Raine, L. B., Johnson, C. R., Chaddock, L., Voss, M. W., Cohen, N. J., et al. (2011). Cardiorespiratory fitness and the flexible modulation of cognitive control in preadolescent children. J. Cogn. Neurosci. 23, 1332–1345. doi: 10.1162/jocn.2010.21528
Prakash, R. S., Patterson, B., Janssen, A., Abduljalil, A., and Boster, A. (2011). Physical activity associated with increased resting-state functional connectivity in multiple sclerosis. J. Int. Neuropsychol. Soc. 17, 986–997. doi: 10.1017/S1355617711001093
Prakash, R. S., Voss, M. W., Erickson, K. I., and Kramer, A. F. (2015). Physical activity and cognitive vitality. Annu. Rev. Psychol. 66, 769–797. doi: 10.1146/annurev-psych-010814-015249
Randy, R., Yves, V. A., and Ilse, D. B. (2003). Addressing overreporting on the international physical activity questionnaire (IPAQ) telephone survey with a population sample. Public Health Nutr. 6, 299–305. doi: 10.1079/phn2002427
Rendeiro, C., and Rhodes, J. S. (2018). A new perspective of the hippocampus in the origin of exercise–brain interactions. Brain Struct. Funct. 223, 2527–2545. doi: 10.1007/s00429-018-1665-6
Rhodes, R. E., and Smith, N. E. I. (2006). Personality correlates of physical activity: A review and meta-analysis. Br. J. Sports Med. 40, 958–965. doi: 10.1136/bjsm.2006.028860
Robinson, J. L., Barron, D. S., Kirby, L. A. J., Bottenhorn, K. L., Hill, A. C., Murphy, J. E., et al. (2015). Neurofunctional topography of the human hippocampus. Hum. Brain Mapp. 36, 5018–5037. doi: 10.1002/HBM.22987
Ruscheweyh, R., Willemer, C., Krüger, K., Duning, T., Warnecke, T., Sommer, J., et al. (2011). Physical activity and memory functions: An interventional study. Neurobiol. Aging 32, 1304–1319. doi: 10.1016/j.neurobiolaging.2009.08.001
Sato, W., Kochiyama, T., Uono, S., Matsuda, K., Usui, K., Inoue, Y., et al. (2014). Rapid, high-frequency, and theta-coupled gamma oscillations in the inferior occipital gyrus during face processing. Cortex 60, 52–68. doi: 10.1016/j.cortex.2014.02.024
Schneider, S., Vogt, T., Frysch, J., Guardiera, P., and Strüder, H. K. (2009). School sport—A neurophysiological approach. Neurosci. Lett. 467, 131–134. doi: 10.1016/j.neulet.2009.10.022
Shipton, O. A., El-Gaby, M., Apergis-Schoute, J., Deisseroth, K., Bannerman, D. M., Paulsen, O., et al. (2014). Left-right dissociation of hippocampal memory processes in mice. Proc. Natl. Acad. Sci. U.S.A. 111, 15238–15243. doi: 10.1073/PNAS.1405648111
Singer, J. (1966). Daydreaming: An introduction to the experimental study of inner experience. New York, NY: Random House.
Smallwood, J., and Andrews-Hanna, J. (2013). Not all minds that wander are lost: The importance of a balanced perspective on the mind-wandering state. Front. Psychol. 4:441. doi: 10.3389/fpsyg.2013.00441
Smallwood, J., Baracaia, S. F., Lowe, M., and Obonsawin, M. (2003). Task unrelated thought whilst encoding information. Conscious. Cogn. 12, 452–484. doi: 10.1016/S1053-8100(03)00018-7
Smallwood, J., McSpadden, M., and Schooler, J. W. (2008). When attention matters: The curious incident of the wandering mind. Mem. Cogn. 36, 1144–1150. doi: 10.3758/MC.36.6.1144
Smallwood, J., Ruby, F. J. M., and Singer, T. (2013). Letting go of the present: Mind-wandering is associated with reduced delay discounting. Conscious. Cogn. 22, 1–7. doi: 10.1016/j.concog.2012.10.007
Smeekens, B. A., and Kane, M. J. (2016). Working memory capacity, mind wandering, and creative cognition: An individual-differences investigation into the benefits of controlled versus spontaneous thought. Psychol. Aesthet. Creat. Arts 10, 389–415. doi: 10.1037/aca0000046
Smith, J. C., Nielson, K. A., Woodard, J. L., Seidenberg, M., Durgerian, S., Antuono, P., et al. (2011). Interactive effects of physical activity and APOE-ε4 on BOLD semantic memory activation in healthy elders. Neuroimage 54, 635–644. doi: 10.1016/j.neuroimage.2010.07.070
Stillman, C. M., Uyar, F., Huang, H., Grove, G. A., Watt, J. C., Wollam, M. E., et al. (2018). Cardiorespiratory fitness is associated with enhanced hippocampal functional connectivity in healthy young adults. Hippocampus 28, 239–247. doi: 10.1002/hipo.22827
Stranahan, A., Khalil, D., and Gould, E. (2007). Running induces widespread structural alterations in the hippocampus and entorhinal cortex. Hippocampus 17, 1017–1022. doi: 10.1002/hipo.20348
Strange, B. A., Fletcher, P. C., Henson, R. N. A., Friston, K. J., and Dolan, R. J. (1999). Segregating the functions of human hippocampus. Proc. Natl. Acad. Sci. U.S.A. 96, 4034–4039. doi: 10.1073/PNAS.96.7.403
Thielen, J.-W., Kärgel, C., Müller, B. W., Rasche, I., Genius, J., Bus, B., et al. (2016). Aerobic activity in the healthy elderly is associated with larger plasticity in memory related brain structures and lower systemic inflammation. Front. Aging Neurosci. 8:319. doi: 10.3389/fnagi.2016.00319
Tomporowski, P., Lambourne, K., and Okumura, M. (2011). Physical activity interventions and children’s mental function: An introduction and overview. Prev. Med. 52(Suppl. 1) S3–S9. doi: 10.1016/j.ypmed.2011.01.028
Tulving, E., and Markowitsch, H. (1998). Episodic and declarative memory: Role of the hippocampus. Hippocampus 8, 198–204. doi: 10.1002/(SICI)1098-106319988:3<198::AID-HIPO2<3.0.CO;2-G
Unsworth, N., and McMillan, B. D. (2013). Mind wandering and reading comprehension: Examining the roles of working memory capacity, interest, motivation, and topic experience. J. Exp. Psychol. Learn. Mem. Cogn. 39, 832–842. doi: 10.1037/a0029669
Vago, D., and David, S. (2012). Self-awareness, self-regulation, and self-transcendence (S-ART): A framework for understanding the neurobiological mechanisms of mindfulness. Front. Hum. Neurosci. 6:296. doi: 10.3389/fnhum.2012.00296
van Praag, H., Christie, B. R., Sejnowski, T. J., and Gage, F. H. (1999). Running enhances neurogenesis, learning, and long-term potentiation in mice. Proc. Natl. Acad. Sci.U.S.A. 96:13427. doi: 10.1073/pnas.96.23.13427
van Praag, H., Shubert, T., Zhao, C., and Gage, F. (2005). Exercise enhances learning and hippocampal neurogenesis in aged mice. J. Neurosci. 25, 8680–8685. doi: 10.1523/JNEUROSCI.1731-05.2005
Voelcker-Rehage, C., Godde, B., and Staudinger, U. M. (2010). Physical and motor fitness are both related to cognition in old age. Eur. J. Neurosci. 31, 167–176. doi: 10.1111/j.1460-9568.2009.07014.x
Voelcker-Rehage, C., Godde, B., and Staudinger, U. M. (2011). Cardiovascular and coordination training differentially improve cognitive performance and neural processing in older adults. Front. Hum. Neurosci. 5:26. doi: 10.3389/fnhum.2011.00026
Voss, M., Prakash, R., Erickson, K., Basak, C., Chaddock, L., Kim, J., et al. (2010). Plasticity of brain networks in a randomized intervention trial of exercise training in older adults. Front. Aging Neurosci. 2:32. doi: 10.3389/fnagi.2010.00032
Keywords: physical activity, mind wandering, hippocampus, resting-state functional connectivity, cognitive function
Citation: Shi D, Geng F, Hao X, Huang K and Hu Y (2022) Relations between physical activity and hippocampal functional connectivity: Modulating role of mind wandering. Front. Hum. Neurosci. 16:950893. doi: 10.3389/fnhum.2022.950893
Received: 23 May 2022; Accepted: 13 September 2022;
Published: 03 October 2022.
Edited by:
Fenghua Sun, The Education University of Hong Kong, Hong Kong SAR, ChinaReviewed by:
Sebastiano Stramaglia, University of Bari Aldo Moro, ItalyCopyright © 2022 Shi, Geng, Hao, Huang and Hu. This is an open-access article distributed under the terms of the Creative Commons Attribution License (CC BY). The use, distribution or reproduction in other forums is permitted, provided the original author(s) and the copyright owner(s) are credited and that the original publication in this journal is cited, in accordance with accepted academic practice. No use, distribution or reproduction is permitted which does not comply with these terms.
*Correspondence: Fengji Geng, Z2VuZ2ZAemp1LmVkdS5jbg==
Disclaimer: All claims expressed in this article are solely those of the authors and do not necessarily represent those of their affiliated organizations, or those of the publisher, the editors and the reviewers. Any product that may be evaluated in this article or claim that may be made by its manufacturer is not guaranteed or endorsed by the publisher.
Research integrity at Frontiers
Learn more about the work of our research integrity team to safeguard the quality of each article we publish.