- 1Department of Physical Therapy, Faculty of Rehabilitation Medicine, University of Alberta, Edmonton, AB, Canada
- 2Neuroscience & Mental Health Institute, University of Alberta, Edmonton, AB, Canada
Balance training, defined here as training of postural equilibrium, improves postural control and reduces the rate of falls especially in older adults. This systematic review aimed to determine the neuroplasticity induced by such training in younger (18–30 years old) and older adults (≥65 years old). We focused on spinal and corticospinal pathways, as studied with electrophysiology, in people without neurological or other systemic disorders. We were specifically interested in the change in the excitability of these pathways before and after training. Searches were conducted in four databases: MEDLINE, CINAHL, Scopus, and Embase. A total of 1,172 abstracts were screened, and 14 articles were included. Quality of the studies was evaluated with the Downs and Black checklist. Twelve of the studies measured spinal reflexes, with ten measuring the soleus H-reflex. The H-reflex amplitude was consistently reduced in younger adults after balance training, while mixed results were found in older adults, with many showing an increase in the H-reflex after training. The differences in results between studies of younger vs. older adults may be related to the differences in their H-reflexes at baseline, with older adults showing much smaller H-reflexes than younger adults. Five studies measured corticospinal and intracortical excitability using transcranial magnetic stimulation. Younger adults showed reduced corticospinal excitability and enhanced intracortical inhibition after balance training. Two studies on older adults reported mixed results after training. No conclusions could be drawn for corticospinal and intracortical plasticity given the small number of studies. Overall, balance training induced measurable change in spinal excitability, with different changes seen in younger compared to older adults.
Introduction
Balance training can reduce the incidence of falls in older adults (Sherrington et al., 2008, 2020; Mansfield et al., 2015), and improve postural equilibrium in younger adults (Zech et al., 2010; Lesinski et al., 2015b). Balance training is defined here as training that focuses on improving postural equilibrium in the upright position, while bearing weight on the feet, and performing tasks that challenge the maintenance of the upright posture, i.e., have a tendency to induce falls. Presumably, neural adaptations induced by the training underlie some of the improvements. A few narrative reviews have suggested that both spinal and corticospinal excitability are reduced after balance training in young adults (Taube et al., 2008; Taube, 2012). Another narrative literature review addressing postural control in younger and older adults indicated that there were very few studies addressing neuroplasticity induced by balance training in older adults (Papegaaij et al., 2014). Further, no systematic reviews were found.
Neuroplasticity induced by balance training is not necessarily the same in younger and older adults. The central nervous system changes substantially with age. First, the size of the soleus Hoffmann (H-) reflex and Ia reciprocal inhibition from the dorsiflexors to the soleus is age-dependent (Kido et al., 2004). The H-reflex for the soleus muscle is induced by surface electrical stimulation of the posterior tibial nerve, and measured with surface EMG over the soleus muscle. It measures the excitability in the monosynaptic Ia-motoneuron pathway. The soleus H-reflex is of interest here, because it is highly modulated during various types of movement such as standing, walking and running (Capaday and Stein, 1986, 1987; Edamura et al., 1991), and has been suggested to interfere with upright balance (Llewellyn et al., 1990). The amplitude of the soleus H-reflex is usually normalized to the maximum M-wave (MMAX) to control for the natural decline in muscle size with age. Some studies reported lower HMAX/MMAX ratio in older adults compared to younger adults (Sabbahi and Sedgwick, 1982; deVeries et al., 1985; Kido et al., 2004; Baudry et al., 2014) while others have reported no changes with age (Vandervoort and Hayes, 1989; Scaglioni et al., 2003). Second, modulation of the H-reflex amplitude as a function of posture also changes with age, even when the levels of background motor neuron excitability is held constant in the tasks. In younger adults, the soleus H-reflex amplitude is largest in lying, and smaller in standing (Koceja et al., 1995; Angulo-Kinzler et al., 1998; Baudry et al., 2015). In contrast, for older adults, the change in the amplitude of the soleus H-reflex as a function of posture was inconsistent. For example, the HMAX/MMAX ratio from lying or sitting to standing postures at matched background levels of EMG in the soleus either did not change (Koceja et al., 1995), increased (Angulo-Kinzler et al., 1998) or decreased (Baudry et al., 2015) in older adults.
Motor regions of the brain are also affected by age. Excitability of corticospinal tracts (CST), studied with single-pulse transcranial magnetic stimulation (TMS) to the primary motor cortex, has mostly addressed age-related changes induced in finger muscles (Rossini et al., 1992; Pitcher et al., 2003; Sale and Semmler, 2005; Oliviero et al., 2006; Talelli et al., 2008; Houde et al., 2018; Rozand et al., 2019). In finger muscles, when the amplitude of the motor evoked potential (MEP) is normalized to the M-wave, the maximum MEP amplitude (MEPMAX) was either similar between younger and older adults (Rossini et al., 1992; Sale and Semmler, 2005; Rozand et al., 2019), or slightly higher (Pitcher et al., 2003) or lower (Talelli et al., 2008) in the older adults. A small number of studies measured MEPMAX in the leg muscles. Compared to young adults, older adults show greater (Baudry et al., 2014, 2015) or similar MEPMAX (Stevens-Lapsley et al., 2013; Hassanlouei et al., 2017). With a change in posture from sitting to standing, greater MEPs were seen in the soleus muscle at matched levels of background EMG, for both younger and older adults, but the percentages of change from sitting to standing was the same between groups (Baudry et al., 2015). Thus, there may be some differences in how muscles in the upper and lower limbs respond to aging.
Responses to postural perturbations also show age-related differences. For example, the latency of postural responses are slower in older compared to younger adults, with an increase of approximately 5 ms for the short latency response (Nardone et al., 1995), and approximately 20–30 ms for the long latency response (Nardone et al., 1995; Allum et al., 2002; Lin and Woollacott, 2002; Tokuno et al., 2010). The amplitudes of the muscle responses as seen from surface EMG also tend to be larger in older adults, especially in the later responses (Tokuno et al., 2010). Certain types of disturbances, such as lateral platform tilts, reveal interesting differences in strategies between older and younger adults, suggesting that older adults adopt a stiffer trunk posture, perhaps as a result of stiffer passive tissue properties and/or muscle co-contraction, which may predispose them to instability (Allum et al., 2002).
Given these many differences between the young and the old, this systematic review aims to summarize the spinal and corticospinal adaption induced by balance training in people without neurological or musculoskeletal problems. Specifically, we were interested in (1) the neurological changes in the spinal and corticospinal pathways after balance training, and (2) whether older and younger adults show different neural adaption after balance training.
Methods
The protocol of this systematic review was registered and published on PROSPERO in April 2021 (https://www.crd.york.ac.uk/PROSPERO/display_record.php?RecordID=250604, protocol ID: CRD42021250604).
Selection Criteria
Original research reported in a peer-reviewed journal were considered. Inclusion and exclusion criteria are outlined in Table 1. We were interested in the plasticity induced naturally by balance training, so training that specifically targeted a pathway was excluded, such as using biofeedback to modify the size of the H-reflex during the training task. The selection criteria for training dosage and frequency were based on previous meta-analyses on the behavioral effects of balance training in younger and older adults (Lesinski et al., 2015a,b). Studies of pathologies were not included to reduce heterogeneity in this initial review. Exceptions were made for studies in which there was mention in the abstract of a control group of neurologically intact individuals. Those papers went through full-text screening to determine if results from the control group were reported separately.
Search Strategy
Systematic literature searches were conducted in 4 databases: MEDLINE, CINAHL, Scopus, and Embase, under the guidance of a librarian from the University of Alberta. Articles were searched up to April 12th, 2021, for MEDLINE, CINAHL, and Scopus, and up to April 14th, 2021 for Embase, with no limitation on the start date.
Two sets of keywords were used to encompass the areas of: (1) balance training, and (2) outcome measures of interest. Based on our preliminary search, we found the training programs to be named in a variety of ways, such as sensorimotor training, Tai-Chi training, slackline training, perturbation-based training. Thus, we selected the following keywords to describe the training modality, in an attempt to capture a variety of balance training programs: “balance training” or “balance perturbation” or “functional balance” or “balance exercise” or “Tai-chi” or “agility training” or “alpine skiing” or “sensorimotor training” or “slackline training” or “postural control training” or “unicycle” or “dance” or “martial arts”. Our second set of keywords addressed the outcome measures of interest. We chose to focus on the neural plasticity in spinal and corticospinal pathways, so the keywords were: “neural plasticity” or “training-induced plasticity” or “learning-dependent plasticity” or “brain plasticity” or “neuroplasticity” or “neural adaptation” or “spinal reflexes” or “reflex modulation” or “postural reflex” or “Hoffman reflex” or “cutaneous reflexes” or “musculocutaneous reflexes” or “H-reflex” or “Hmax” or “H-max” or “corticomotor-excitability” or “cortical-excitability” or “spinal-excitability” or “short interval cortical inhibition” or “SICI” or “transcranial magnetic stimulation” or “TMS” or “motor evoked potential”.
Additional filters were used to limit the search results to those studies with human participants, and published in English. The syntax was modified based on the function and interface of each database. Our librarian consultant reviewed and confirmed that the translations of syntax between databases were correct. A complete search syntax is included in the Supplementary Material.
Screening Process
Search results were exported from the individual databases and imported to Covidence, a management software for systematic reviews (Covidence, Melbourne, Australia). Abstract screening, full-text screening, and data extraction were all completed in Covidence.
The same inclusion/exclusion criteria (Table 1) were used for both abstract and full-text screening. During the abstract screening, two reviewers (YS and one of CH, JY, or MB) screened the titles and abstracts independently to exclude studies that were clearly irrelevant. Studies that met all the criteria, or did not contain sufficient information in the abstract to allow a determination were retained for full-text screening. During the full-text screen, two reviewers (YS and one of CH, JY, or MB) screened the full-text independently. Studies that did not meet the inclusion/exclusion criteria were excluded. Differences between reviewers at each stage of screening were resolved between the two reviewers, and if this was impossible, a third author participated to reach a consensus.
Data Extraction
Data extraction was conducted by two reviewers (YS and JY, or YS and CH) independently, by reading the full-text. The following data were extracted from the eligible studies: author, title, study purpose, study design, number of participants, sample size justification, age of participants, gender or sex of participants, inclusion & exclusion criteria, the participants' previous experience with the training task, details of the balance training, whether the training was supervised and/or progressive, training frequency (i.e., sessions/week), training dose (i.e., minutes engaged in balance-related tasks), training results (i.e., measures before and after training), measures of spinal pathways, measures of corticospinal pathways, other measures, statistical analyses, conclusions. If the study compared two types of training, such as in a randomized controlled trial (RCT), the group that received the highest dose of balance-related training was used. If both groups received the same amount of balance training, then results from both groups were included.
Further, methodological factors that could influence the electrophysiological outcomes were extracted. For the spinal measurements, these included: (1) nerve and muscle tested, (2) posture during the test, (3) task performed during the test (i.e., at rest or during movement), (4) how the reflex was controlled if the test was performed during movement (i.e., matched background EMG, matched M-wave size or other, or unmatched), (5) stimulus intensity, (6) number of pulses averaged if averaging was used. For TMS measures, these included: (1) the type of coil, (2) coil position, (3) the stimulator used, (4) the number of stimuli averaged, (5) stimulus intensity, (6) conditions that were specifically controlled (such as background EMG, timing of stimulus application), (7) if paired-pulse stimulation was used, the intensity of each pulse and the inter-stimulus interval between the first and second stimulus, (8) the posture during the test, (9) the task performed during the test.
Quality Assessment
The Downs & Black checklist (Downs and Black, 1998) was used to assess the quality of each study. This method encompasses evaluation of methodological quality for both RCT and non-RCT studies. The score reflects the quality of the reporting, internal and external validity. Two evaluators (either YS and CH or YS and JY) independently scored the papers and discrepancies were resolved by discussion and consensus.
Synthesis of Evidence
Studies were grouped according to the ages of the participants, based on the mean age. Those studies reporting a mean age of 65 years old or older were considered older adults. The intervention methods differed considerably, so the interventions were grouped according to similarities in type, for example, balancing in standing on unstable surfaces, martial arts, and specific types of sports. Since most of the training sessions included some time spent in warm-up, rest, or exercises that did not focus on balance, we calculated the total time spent in balance-related exercises as follows. The aspects of the training that challenged balance were identified, and the time spent on those balance tasks were calculated. This required that the study provided description of the tasks and the time spent on each of the tasks.
The balance outcomes also differed considerably, so we determined whether a quantitative outcome demonstrated improvement, such as displacement of the center of pressure during standing on a force platform, or a standardized and validated clinical score. Outcomes of spinal and corticospinal excitability were grouped according to the type of measure and variable quantified. In cases where a comparable outcome was reported from multiple groups, the data were summarized in table or graphic form. If means and standard deviations were not reported or only reported in graphic form, then the corresponding authors were contacted to obtain the actual means and standard deviations. If authors did not respond after two attempts to contact, the means were estimated directly from the reported figures.
Results
Study Selection and Characteristics
Figure 1 shows the PRISMA flow diagram. A total of 2,103 articles were identified. After removing 931 duplicates, we screened 1,172 abstracts and 42 articles were included for full-text screening. The majority of papers were excluded because they were irrelevant, or did not meet our inclusion criteria. During full-text screening, one paper was identified (Granacher et al., 2006) to have measured balance using methods that were not independent of the electrophysiological measures. In this study, postural reflexes were induced by translating the support-surface (treadmill), and improvements in balance (reduction in induced ankle and knee velocity) as well as neuroplasticity (reflex latencies) were deduced from these same trials. We excluded this paper, because we believe the argument was circular, in that any changes in neurophysiological outcome would likely induce changes in the mechanical outcome of the same trial, and could not be assumed to be an improvement in balance without an independent measure of balance, as reported in all other papers included in our review. We did not anticipate this possibility a priori, but by consensus between the authors, this paper was excluded. Thus, full-text screening resulted in 14 eligible studies.
Among the 14 studies (Table 2), 12 measured spinal plasticity, 5 measured corticospinal or intracortical plasticity, including 3 that measured both spinal and corticospinal plasticity. Key data extracted from measures of spinal excitability and corticospinal or intracortical plasticity are shown in Tables 3, 4, respectively. One paper (Lauber et al., 2021) presented results from both soleus H-reflex and TMS measurements, but the data collection procedures for the H-reflex were not reported. Thus, this study was included among those that only measured corticospinal plasticity; the results of H-reflexes from this study were not included. Six out of 14 studies trained older adults (65 years or older); these studies are identified in blue shading in Tables 2–4. One study did not specify the ages of the participants (Keller et al., 2012), but we confirmed the age of the participants to be younger adults through communication with the corresponding author. Thus 8 studies focused on younger adults between 18 and 30 years old.
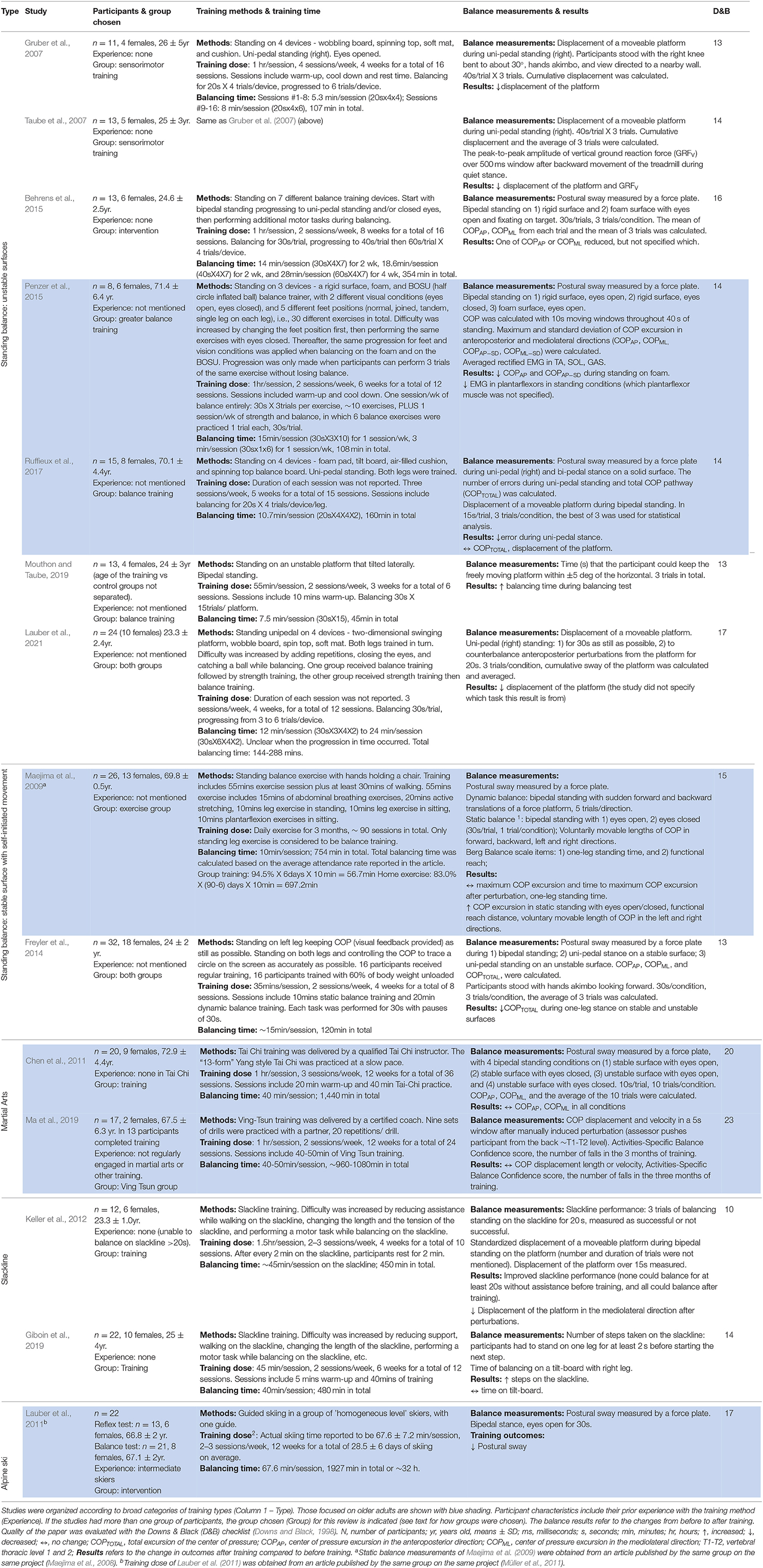
Table 2. Summary of participant characteristics, training, balance outcomes, and the quality of the papers.
Quality of the Studies
The scores obtained from the Downs & Black checklist are shown in Table 2 (last column). Higher scores indicate better quality, with the best possible score being 28. The majority of papers were of moderate quality: mean ± standard deviation (SD) = 15.2 ± 3.3, median = 14, with a range from 10 to 23. The most common unmet criterion was blinding of participants to the intervention, which was impossible by nature of the interventions. Other criteria that were most often unmet were: reporting of adverse events, using a representative sample of the population, delivering the intervention in a setting where such interventions are normally delivered, blinding of evaluators, and concealment of randomization to participants and researcher until completion of the study.
Training Modality and Dosage
The training details are summarized in Table 2. Studies were broadly grouped into 5 categories: (1) standing balance on unstable surfaces (7 studies), (2) standing balance on a stable surface (2 studies), (3) martial arts training (2 studies), (4) slackline training (2 studies), and (5) alpine skiing (1 study). Here, the term “standing balance” describes training that required participants to stand on one spot while maintaining balance during various tasks. All the training exercises were performed in an upright posture, supporting body weight on the feet.
Total training time ranged from 6 sessions over 3 weeks to daily exercise over 3 months. All studies provided sufficient information to estimate the actual time spent on balance-related tasks except the skiing time of Lauber et al. (2011), which was obtained from a different article using the same training method, referred to by the authors in their Methods (Müller et al., 2011). In this way, the total balancing time through the entire training program was estimated to range from 45 min (Mouthon and Taube, 2019) to 32 h (Lauber et al., 2011). The frequency of exercises ranged from 2 to 7 times per week, and the duration of the sessions ranged from about 5 min to 3 h per session.
Balance Outcomes
Balance measurements and results are summarized in Table 2. Most of the studies provided sufficient detail about balance outcomes. The study by Maejima and colleagues (Maejima et al., 2009) referred to an earlier publication for methods regarding the balance measure, so they were obtained from the earlier publication (Maejima et al., 2008). Balance outcomes were measured in a variety of ways. For example, balance duration when standing uni- or bipedally on different surfaces, postural sway when attempting to stay stationary, the degree of disturbance to the body from a perturbation, or the reach distance in a Functional Reach Test (Duncan et al., 1990). Regardless, the majority of studies showed improvements in balance with training. Only two of the 14 studies did not show improvements (Chen et al., 2011; Ma et al., 2019).
Spinal Plasticity After Balance Training
Spinal plasticity was reported in 12 studies with two types of spinal reflexes: (1) soleus H-reflex (10 studies), and (2) short latency postural reflexes induced by external perturbations (2 studies). Measurement methods and results from spinal reflexes are shown in Table 3.
Soleus H-reflexes were quantified in three different ways: (1) the ratio of maximal H-reflex over the maximum M-wave (HMAX/MMAX) (8 studies); (2) the H-reflex during a task normalized to another standardized testing condition or MMAX (normalized H-reflex) (2 studies); (3) the slope of the rising phase of the H-reflex recruitment (1 study). Since there were several papers that reported HMAX/MMAX under matched conditions before and after training, we summarized these pre-post changes for younger and older adults. In cases where more than one condition was reported for the HMAX/MMAX, HMAX/MMAX ratios from all the conditions were extracted. All authors contacted to obtain values responded except two, so those were estimated directly from the published figures.
The results for the change in HMAX/MMAX are shown in Figure 2, with cold colors (dots) representing younger adults and warm colors (open circles) representing older adults. Note that some studies reported the findings under more than one condition, so those different conditions are represented with the same color. As a group, older adults had smaller H-reflexes prior to training compared to younger adults (horizontal axis); this was expected based on prior literature (see Introduction). Interestingly, the way in which the reflexes changed with balance training was also different. Younger adults tended to show a reduction in the HMAX/MMAX with training, whereas older adults tended to show smaller changes in either direction, with a suggestion that the change depended on the magnitude of HMAX/MMAX prior to training.
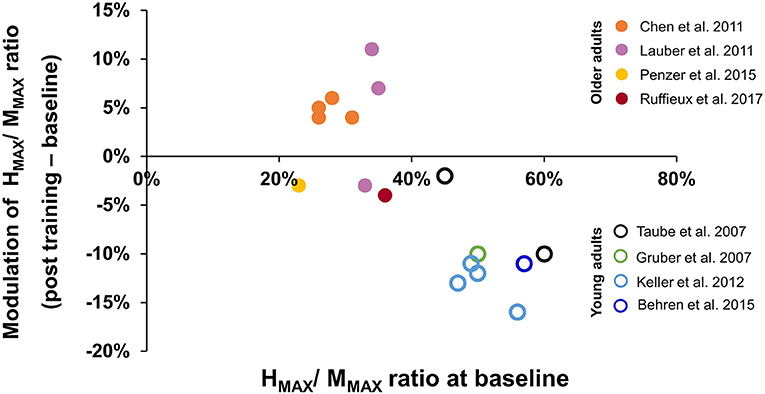
Figure 2. Modulation of HMAX/MMAX ratio after balance training. The x-axis represents averaged HMAX/MMAX ratio at baseline, the y-axis represents the modulation of HMAX/MMAX ratio after balance training, with negative values indicating reduced HMAX/MMAX ratio after training. Data were obtained from 8 studies (see Results, Section 3.5). Data from different test conditions within the same study are represented by the same color. The solid dots represent data from older adults, while the open circles represent data from young adults.
Postural reflexes after perturbations were measured in two studies with older adults. The perturbations were applied through translational movements of a platform in anterior or posterior directions or through a forward push from the experimenter. One study reported shorter response latency in the gastrocnemius muscle, and decreased integrated EMG from the TA and quadriceps muscles (Maejima et al., 2009) while the other study showed greater reflex latency in the gastrocnemius muscle (Ma et al., 2019).
Corticospinal and Intracortical Plasticity After Balance Training
Corticospinal or intracortical excitability was reported in five studies, with four of the five studies coauthored by a few of the same authors, and originating from two labs. Methods and results are summarized in Table 4.
One study reported the input-output relationship of the MEP amplitude in the soleus muscle during standing in older adults only (Penzer et al., 2015). The slope of the input-output relationship was reduced and the stimulus intensity required to elicit the MEPMAX increased after training, suggesting a reduction in the excitability of the motor cortex (Penzer et al., 2015).
Two studies measured TMS-conditioned soleus H-reflex in response to standing perturbations (Taube et al., 2007; Ruffieux et al., 2017). Perturbations were applied through a standardized, rapid backward movement of the treadmill belt at 60 m/s2, while the participant stood on the stationary treadmill belt. In these two studies, TMS conditioned H-reflexes were used to indicate the excitability of the monosynaptic input from the CST. The soleus H-reflex and the single-pulse, subthreshold, TMS-evoked MEP in the soleus were timed to arrive during the short (SLR) or long-latency response (LLR) after the perturbation. The inter-stimulus interval between the stimulation to the tibial nerve and primary motor cortex was timed so that the TMS pulse arrived at the motor neuron when the first observable H-reflex facilitation occurred. Facilitation at this time is mostly attributable to the direct monosynaptic projections from the motor cortex to spinal motor neuron (Nielsen et al., 1993; Petersen et al., 1999). The amplitude of the TMS conditioned H-reflex reduced after balance training at the LLR but not the SLR in younger participants (Taube et al., 2007). Further, a significant correlation was observed between improvements in balance performance (i.e., postural sway during uni-pedal standing) and reduction in TMS-conditioned H-reflex after perturbations. The results suggest a reduction in the corticospinal input to the soleus with training in younger participants, and that the reduction was associated with the improvements in balance. With the identical training and assessment methods, the above changes were not observed in older participants, even though there were improvements in balance, as reflected by a uni-pedal stance test (Ruffieux et al., 2017).
Two other studies (Mouthon and Taube, 2019; Lauber et al., 2021) reported changes in short latency intracortical inhibition (SICI) after training in younger participants. SICI is thought to reflect GABAA activity in the motor cortex (Ziemann, 2013). SICI was measured in the TA (Mouthon and Taube, 2019) and SOL muscles (Lauber et al., 2021), using standard protocols (Kujirai et al., 1993) that were identical in the two studies. Briefly, after the hotspot for obtaining MEPs in each muscle was determined, paired-pulse TMS was used, with the initial conditioning pulse subthreshold (0.8x active motor threshold), and the subsequent test pulse suprathreshold (1.2x active motor threshold), and an inter-stimulus interval of 2.5 ms in both studies. SICI was quantified as the amount of depression generated by the conditioning pulse as a function of the size of the unconditioned test pulse. In one study (Mouthon and Taube, 2019), SICI in the TA muscle was elicited under 3 conditions: lying, quiet standing, and standing on different movable platforms. Enhanced SICI was observed in all the test conditions after balance training. SICI in the SOL muscle was elicited under 4 conditions: quiet sitting, sitting with ballistic plantarflexion, quiet standing, and standing with perturbation from posteriorly moved treadmill belt. Increased SICI was only observed in the soleus muscle after balance training during the standing with perturbation condition (Lauber et al., 2021). Overall, greater intracortical inhibition was observed when a change was induced after training.
Discussion
Overview
In general, balance training induced different excitability changes in the spinal reflexes in younger compared to older adults. The amplitude of the soleus H-reflex, for example, was consistently reduced in younger participants after balance training, whereas the same reflex tended to change less or in the opposite direction after training in older adults. A number of possible reasons for these differences are considered below. Corticospinal adaptations were reported in five studies with only two studies focused on older adults. Because of the small number of studies, and very few in which younger and older adults were tested under matched conditions, no clear conclusions can be drawn with respect to cortical and corticospinal excitability.
Possible Explanations for Differences in Training Induced Changes in Spinal Reflexes Between Younger and Older Adults
Balance training in younger adults resulted in a reduction of the soleus H-reflex gain after training, most clearly seen in the change to the HMAX/MMAX ratio (Figure 2). A reduction in the soleus H-reflex gain with balance training is consistent with acute modulation of the reflex gain when balance is challenged. For example, comparing lying to standing (Mynark and Koceja, 1997; Mynark et al., 1997), sitting to standing (Goulart et al., 2000), or standing to walking (Capaday and Stein, 1986), decreased soleus H-reflex amplitude was observed when balance was challenged, at matched excitability levels of the motor neuron pool (i.e., matched background EMG). Studies that incorporated balancing on unstable surfaces induced further reductions in the H-reflex amplitude (Llewellyn et al., 1990; Hoffman and Koceja, 1995; Solopova et al., 2003). Some have suggested that reduced spinal reflex gain can prevent the automatic soleus stretch reflex from precipitating postural instability, thereby enhancing balance during a more challenging task (Llewellyn et al., 1990). Perhaps the reduction in reflex gain with training serves the same purpose.
Compared to younger adults, older adults showed smaller H-reflex amplitudes before training (Figure 2), again consistent with other reports of the soleus H-reflex in older adults, regardless of posture (Sabbahi and Sedgwick, 1982; Koceja et al., 1995; Angulo-Kinzler et al., 1998; Baudry et al., 2015), even when normalized to the maximum M-wave (Koceja et al., 1995; Angulo-Kinzler et al., 1998; Baudry et al., 2015). Interestingly, the changes induced by balance training in older adults were either a very small decrease, or an increase in the HMAX/MMAX ratio, opposite in direction to that seen in younger adults (Figure 2). The reasons for these differences between younger and older adults are unknown.
One explanation might be that modulating the reflex gain with training may be more difficult to achieve in older adults, analogous to “blunted” neuromuscular plasticity induced by resistance training (Bickel et al., 2011). There is limited evidence, however, that older adults have the same capacity as younger adults to reduce the gain of the soleus H-reflex when it is appropriate for balance. For example, when the H-reflex was used as a perturbation during standing on an unstable surface, older adults were able to reduce their H-reflex amplitude to the same extent as younger adults, at least in the short term (i.e., over 2 training days) (Mynark and Koceja, 2002).
Another possibility is that there is a particular range of H-reflex gain that is best suited for a particular task, and hence the direction and magnitude of the change are dependent on the baseline excitability prior to training, which could vary between individuals. This idea has been proposed by Koceja et al. (1995), when comparing the H-reflex modulation from prone to standing. In their study, young participants showed a reduction in HMAX/ MMAX from prone to standing. In contrast, older adults exhibited two types of responses: the subgroup with higher HMAX/ MMAX in prone showed a reduction in HMAX/ MMAX when standing, while the subgroup with lower HMAX/ MMAX in prone showed an increase in HMAX/ MMAX when standing (Koceja et al., 1995), such that both groups reached similar HMAX/ MMAX in the standing position. With long term training in a balancing task, as we are considering here, it is possible that the magnitude and direction of change in the soleus H-reflex is dependent on the baseline H-reflex excitability prior to training. There is considerable individual variation between participants in the excitability of the spinal circuits, as shown by Solopova et al. (2022). Further, although aging is associated with a general reduction in the H-reflex amplitude (reviewed in Introduction), it may also be modified by the level and type of activity the individual normally engages in Pérot et al. (1991), Raglin et al. (1996). This may partially explain why balance training led to a different magnitude and direction of change among older adults.
Cortical Motor Pathways After Balance Training
After balance training in younger participants, there was a reduction in the excitability of the corticospinal pathways to the soleus muscle (Taube et al., 2007), and an increase in the SICI to the tibialis anterior (Mouthon and Taube, 2019) and soleus (Lauber et al., 2021). Together, this suggests that with training, the role of the motor cortex may become smaller in balance tasks in younger adults, especially during tasks that are similar to the trained tasks. The results are less clear in older adults, since the slope of the input-output curve for MEP was reduced during stationary standing (Penzer et al., 2015), and the TMS conditioned H-reflex was unchanged during balancing tasks (Ruffieux et al., 2017).
One caveat is that the methods used to obtain some of these findings were extremely complex. For example, the paradigm to measure TMS-conditioned H-reflexes during the LLR induced by a perturbation required that the perturbation, the occurrence of the LLR, the peripheral nerve stimulation and the TMS pulse had to be perfectly timed (Taube et al., 2007; Ruffieux et al., 2017). Further, the background excitability of the spinal motor neurons at the time of occurrence of these stimuli would have to be the same before and after training to truly reflect a change at the cortical level. Their measure of the excitability of the motor neuron pool was based on the background EMG obtained in separate trials with the mechanical perturbation alone without the stimulation, and from the background EMG 200 ms before the onset of the perturbation. These measures do not necessarily guarantee that the excitability of the motor neuron pool was the same for either the separate trials, or for measures taken at different times after the onset of the perturbation.
Brain activity as seen from functional MRI during imagined movements of the lower extremity showed increased brain activation in older compared to younger adults, especially during imagined standing (Zwergal et al., 2012) and isolated ankle movements (Sacheli et al., 2020). This suggests that the brain regions involved during lower extremity movements change with age. Further, neuroplasticity in the motor regions of the brain may decline with age, as seen from an age-dependent reduction in the transcranial theta-burst TMS induced long-term depression (LTD) in the primary motor cortex (Freitas et al., 2011); see also a broader review of age-related changes in brain plasticity in Pascual-Leone et al. (2011). Thus, it is very likely that there are differences between younger and older adults in how they respond to balance training and how well the learned behaviors are retained. These gaps in our knowledge remain to be explored.
Limitations
This review included only English publications, and four databases were searched. Thus, it is possible that relevant papers were missed. Further, some papers reporting balance training in individuals with pathology may have contained control participants, but were screened out because the inclusion of control participants was not mentioned in the abstract. This review was focused on electrophysiological measures of plasticity, so brain plasticity measured with brain imaging was not included.
The training methods were diverse among the included papers. We attempted to reduce the variability by focusing on training methods that were carried out during weight-bearing on the feet with a purpose to challenge balance, and had a minimum dosage (6 sessions) and frequency (once per week). Nevertheless, they ranged from exercises during standing, martial arts, skiing, and slackline walking. With this broad range of exercises included, only 14 studies fit our inclusion/exclusion criteria. Thus, it was impossible to focus more narrowly on specific training methods. It is likely that the different forms of exercise induced different neuroplastic changes, many of which may not have been possible to extract from these small numbers of diverse papers. Further, there was no measure of training intensity, a gap that remains to be filled by future work.
The diversity of outcome measures used both for balance and neurophysiological measures were considerable, making it difficult to conduct meta-analyses. We confirm that the conditions of measurement, such as the task performed during the electrophysiological measurements, the stimulus intensity used, among others, were matched in each study before and after training. Nevertheless, it is likely that the measurement conditions contributed to some of the variability in the findings. Finally, the moderate quality and the small number of papers mean that the conclusions remain preliminary.
Data Availability Statement
The original contributions presented in the study are included in the article/Supplementary Material, further inquiries can be directed to the corresponding author.
Author Contributions
YS and JY conceived of the study and designed the search approach. YS searched articles. YS, CH, MB, and JY screened abstracts and full texts. YS, CH, and JY extracted data. The draft of the manuscript was written by YS and JY, and revised by YS, CH, MB, and JY. All authors read and approved the final manuscript.
Funding
YS is funded by a Postdoctoral Recruitment Award from the Faculty of Rehabilitation Medicine at the University of Alberta.
Conflict of Interest
The authors declare that the research was conducted in the absence of any commercial or financial relationships that could be construed as a potential conflict of interest.
Publisher's Note
All claims expressed in this article are solely those of the authors and do not necessarily represent those of their affiliated organizations, or those of the publisher, the editors and the reviewers. Any product that may be evaluated in this article, or claim that may be made by its manufacturer, is not guaranteed or endorsed by the publisher.
Acknowledgments
We would like to thank Elizabeth Dennett, librarian, who helped us create and revise search syntaxes for the databases, and to Dr. Tania Lam and Dr. E.Paul Zehr for providing valuable feedback on earlier versions of this manuscript.
Supplementary Material
The Supplementary Material for this article can be found online at: https://www.frontiersin.org/articles/10.3389/fnhum.2022.921490/full#supplementary-material
References
Allum, J. H. J., Carpenter, M. G., Honegger, F., Adkin, A. L., and Bloem, B. R. (2002). Age-dependent variations in the directional sensitivity of balance corrections and compensatory arm movements in man. J. Physiol. 542, 643–663. doi: 10.1113/jphysiol.2001.015644
Angulo-Kinzler, R. M., Mynark, R. G., and Koceja, D. M. (1998). Soleus H-reflex gain in elderly and young adults: modulation due to body position. J. Gerontol. Ser. Biol. Sci. Med. Sci. 53, 120–125. doi: 10.1093/gerona/53A.2.M120
Baudry, S., Collignon, S., and Duchateau, J. (2015). Influence of age and posture on spinal and corticospinal excitability. Exp. Gerontol. 69, 62–69. doi: 10.1016/j.exger.2015.06.006
Baudry, S., Penzer, F., and Duchateau, J. (2014). Input-output characteristics of soleus homonymous Ia afferents and corticospinal pathways during upright standing differ between young and elderly adults. Acta Physiol. 210, 667–677. doi: 10.1111/apha.12233
Behrens, M., Mau-Moeller, A., Wassermann, F., Bader, R., and Bruhn, S. (2015). Effect of balance training on neuromuscular function at rest and during isometric maximum voluntary contraction. European J. Appl. Physiol. 115, 1075–1085. doi: 10.1007/s00421-014-3089-1
Bickel, C. S., Cross, J. M., and Bamman, M. M. (2011). Exercise dosing to retain resistance training adaptations in young and older adults. Med. Sci. Sports Exerc. 43, 1177–1187. doi: 10.1249/MSS.0b013e318207c15d
Capaday, C., Stein, R. B. (1986). Amplitude modulation of the soleus H-reflex in the human during walking and standing. J. Neurosci. 6, 1308–1313. doi: 10.1523/JNEUROSCI.06-05-01308.1986
Capaday, C., and Stein, R. B. (1987). Difference in the amplitude of the human soleus H reflex during walking and running. J. Physiol. 392, 513–522. doi: 10.1113/jphysiol.1987.sp016794
Chen, Y. S., Zhou, S., and Cartwright, C. (2011). Effect of 12 weeks of Tai Chi training on Soleus Hoffmann reflex and control of static posture in older adults. Arch. Phys. Med. Rehabilit. 92, 886–891. doi: 10.1016/j.apmr.2010.12.043
deVeries, H. A., Wiswell, R. A., Romero, G. T., and Heckathorne, E. (1985). Changes with age in monosynaptic reflexes elicited by mechanical and eletrical stimulation. Am. J. Phys. Med. 64, 71–81.
Downs, S. H., and Black, N. (1998). The feasibility of creating a checklist for the assessment of the methodological quality both of randomised and non-randomised studies of health care interventions. J. Epidemiol. Commun. Health 52, 377–384. doi: 10.1136/jech.52.6.377
Duncan, P. W., Weiner, D. K., Chandler, J., and Studenski, S. (1990). Functional reach: a new clinical measure of balance. J. Gerontol. 45, 1–2. doi: 10.1093/geronj/45.6.M192
Edamura, M., Yang, J. F., and Stein, R. B. (1991). Factors that determine the magnitude and time course of human H-reflexes in locomotion. J. Neurosci. 11, 420–427. doi: 10.1523/JNEUROSCI.11-02-00420.1991
Freitas, C., Perez, J., Knobel, M., Tormos, J. M., Oberman, L., Eldaief, M., et al. (2011). Changes in cortical plasticity across the lifespan. Front. Aging Neurosci. 3, 1–8. doi: 10.3389/fnagi.2011.00005
Freyler, K., Weltin, E., Gollhofer, A., and Ritzmann, R. (2014). Improved postural control in response to a 4-week balance training with partially unloaded bodyweight. Gait Posture. 40, 291–296. doi: 10.1016/j.gaitpost.2014.04.186
Giboin, L. S., Loewe, K., Hassa, T., Kramer, A., Dettmers, C., Spiteri, S., et al. (2019). Cortical, subcortical and spinal neural correlates of slackline training-induced balance performance improvements. NeuroImage. 202, 116061. doi: 10.1016/j.neuroimage.2019.116061
Goulart, F., Valls-Solé, J., and Alvarez, R. (2000). Posture-related changes of soleus H-reflex excitability. Muscle Nerve. 23, 925–932. doi: 10.1002/(sici)1097-4598(200006)23:6<925::aid-mus13>3.0.co;2-k
Granacher, U., Gollhofer, A., and Strass, D. (2006). Training induced adaptations in characteristics of postural reflexes in elderly men. Gait Posture 24, 459–466. doi: 10.1016/j.gaitpost.2005.12.007
Gruber, M., Taube, W., Gollhofer, A., Beck, S., Amtage, F., and Schubert, M. (2007). Training-specific adaptations of H- and stretch reflexes in human soleus muscle. J. Mot. Behav. 39, 68–78. doi: 10.3200/JMBR.39.1.68-78
Hassanlouei, H., Sundberg, C. W., Smith, A. E., Kuplic, A., and Hunter, S. K. (2017). Physical activity modulates corticospinal excitability of the lower limb in young and old adults. J. Appl. Physiol. 123, 364–374. doi: 10.1152/japplphysiol.01078.2016
Hoffman, M. A., and Koceja, D. M. (1995). The effects of vision and task complexity on Hoffmann reflex gain. Brain Res. 700, 303–307. doi: 10.1016/0006-8993(95)01082-7
Houde, F., Laroche, S., Thivierge, V., Martel, M., Harvey, M. P., Daigle, F., et al. (2018). Transcranial magnetic stimulation measures in the elderly: reliability, smallest detectable change and the potential influence of lifestyle habits. Front. Aging Neurosci. 10, 1–12. doi: 10.3389/fnagi.2018.00379
Keller, M., Pfusterschmied, J., Buchecker, M., Müller, E., and Taube, W. (2012). Improved postural control after slackline training is accompanied by reduced H-reflexes. Scand. J. Medi. Sci. Sports 22, 471–477. doi: 10.1111/j.1600-0838.2010.01268.x
Kido, A., Tanaka, N., and Stein, R. B. (2004). Spinal excitation and inhibition decrease as humans age. Canad. J. Physiol. Pharmacol. 82, 238–248. doi: 10.1139/y04-017
Koceja, D. M., Markus, C. A., and Trimble, M. H. (1995). Postural modulation of the soleus H reflex in young and old subjects. Electroencephalogr. Clin. Neurophysiol. Electromyogr. 97, 387–393. doi: 10.1016/0924-980X(95)00163-F
Kujirai, T., Caramia, M. D., Rothwell, J. C., Day, B. L., Thompson, P. D. A., et al. (1993). Corticocortical inhibition in human motor cortex. J. Physiol. 1993, 501–519. doi: 10.1113/jphysiol.1993.sp019912
Lauber, B., Gollhofer, A., and Taube, W. (2021). What to train first: balance or explosive strength? Impact on performance and intracortical inhibition. Scand. J. Med. Sci. Sports 31, 1301–1312. doi: 10.1111/sms.13939
Lauber, B., Keller, M., Gollhofer, A., Müller, E., and Taube, W. (2011). Spinal reflex plasticity in response to alpine skiing in the elderly. Scand. J. Med. Sci. Sports 21, 62–68. doi: 10.1111/j.1600-0838.2011.01343.x
Lesinski, M., Hortobágyi, T., Muehlbauer, T., Gollhofer, A., and Granacher, U. (2015a). Dose-response relationships of balance training in healthy young adults: a systematic review and meta-analysis. Sports Med. 45, 557–576. doi: 10.1007/s40279-014-0284-5
Lesinski, M., Hortobágyi, T., Muehlbauer, T., Gollhofer, A., and Granacher, U. (2015b). Effects of balance training on balance performance in healthy older adults: a systematic review and meta-analysis. Sports Med. 45, 1721–1738. doi: 10.1007/s40279-015-0375-y
Lin, S., and Woollacott, M. H. (2002). Postural muscle responses following changing balance threats in young, stable older, and unstable older adults. J. Motor Behav. 34, 37–44. doi: 10.1080/00222890209601929
Llewellyn, M., Yang, J. F., and Prochazka, A. (1990). Human H-reflexes are smaller in difficult beam walking than in normal treadmill walking. Exp. Brain Res. 83, 22–28. doi: 10.1007/BF00232189
Ma, A. W. W., Wang, H. K., Chen, D. R., Chen, Y. M., Chak, Y. T. C., Chan, J. W. Y., et al. (2019). Chinese martial art training failed to improve balance or inhibit falls in older adults. Perceptual Motor Skills 126, 389–409. doi: 10.1177/0031512518824945
Maejima, H., Kanetada, Y., Sunahori, H., Murase, A., Otani, T., Sakamoto, N., et al. (2008). The effects of comprehensive exercise program on the adjustments of standing balance in community-dwelling elderly persons. J. Jpn. Phys. Therapy Assoc. 11, 7–13. doi: 10.1298/jjpta.11.7
Maejima, H., Sunahori, H., Kanetada, Y., Murase, A., Tobimatsu, Y., Otani, T., et al. (2009). The neural adjustment of postural response through community-based daily exercises in elderly persons. Clin. Biomech. 24, 499–503. doi: 10.1016/j.clinbiomech.2009.03.007
Mansfield, A., Wong, J. S., Bryce, J., Knorr, S., and Patterson, K. K. (2015). Does perturbation-based balance trianing prevent falls? Systematic review and meta-analysis of preliminary randomized controlled trials. Phys. Therapy 95, 700–709. doi: 10.2522/ptj.20140090
Mouthon, A., and Taube, W. (2019). Intracortical inhibition increases during postural task execution in response to balance training. Neuroscience 401, 35–42. doi: 10.1016/j.neuroscience.2019.01.007
Müller, E., Gimpl, M., Poetzelsberger, B., Finkenzeller, T., and Scheiber, P. (2011). Salzburg skiing for the elderly study: study design and intervention - health benefit of alpine skiing for elderly. Scand. J. Med. Sci. Sports 21, 1–8. doi: 10.1111/j.1600-0838.2011.01336.x
Mynark, R. G., and Koceja, D. M. (1997). Comparison of soleus H-reflex gain from prone to standing in dancers and controls. Electroencephalogr. Clin. Neurophysiol. Electromyogr. Motor Control 105, 135–140. doi: 10.1016/S0924-980X(96)96096-8
Mynark, R. G., and Koceja, D. M. (2002). Down training of the elderly soleus H reflex with the use of a spinally induced balance perturbation. J. Appl. Physiol. 93, 127–133. doi: 10.1152/japplphysiol.00007.2001
Mynark, R. G., Koceja, D. M., and Lewis, C. A. (1997). Heteronymous monosynaptic Ia facilitation from supine to standing and its relationship to the soleus H-reflex. Int. J. Neurosci. 92, 171–186. doi: 10.3109/00207459708986400
Nardone, A., Siliotto, R., Grasso, M., and Schieppati, M. (1995). Influence of aging on leg muscle reflex responses to stance perturbation. Arch. Phys. Med. Rehabilitat. 76, 158–165. doi: 10.1016/S0003-9993(95)80025-5
Nielsen, J., Petersen, N., Deuschl, G., and Ballegaard, M. (1993). Task-related changes in the effect of magnetic brain stimulation on spinal neurones in man. Physiology 1993, 223–243. doi: 10.1113/jphysiol.1993.sp019899
Oliviero, A., Profice, P., Tonali, P. A., Pilato, F., Saturno, E., Dileone, M., et al. (2006). Effects of aging on motor cortex excitability. Neurosci. Res. 55, 74–77. doi: 10.1016/j.neures.2006.02.002
Papegaaij, S., Taube, W., Baudry, S., Otten, E., and Hortobágyi, T. (2014). Aging causes a reorganization of cortical and spinal control of posture. Front. Aging Neurosci. 6, 1–15. doi: 10.3389/fnagi.2014.00028
Pascual-Leone, A., Freitas, C., Oberman, L., Horvath, J. C., Halko, M., Eldaief, M., et al. (2011). Characterizing brain cortical plasticity and network dynamics across the age-span in health and disease with TMS-EEG and TMS-fMRI. Brain Topogr. 24, 302–315. doi: 10.1007/s10548-011-0196-8
Penzer, F., Duchateau, J., and Baudry, S. (2015). Effects of short-term training combining strength and balance exercises on maximal strength and upright standing steadiness in elderly adults. Exp. Gerontol. 61, 38–46. doi: 10.1016/j.exger.2014.11.013
Pérot, C., Goubel, F., and Mora, I. (1991). Quantification of T- and H-responses before and after a period of endurance training. Eur. J. Appl. Physiol. Occup. Physiol. 63, 368–375. doi: 10.1007/BF00364464
Petersen, N., Christensen, L. O. D., Morita, H., Sinkjaer, T., and Nielsen, J. (1999). Evidence suggesting that a transcortical reflex pathway contributes to cutaneous reflexes in the tibialis anterior muscle during walking in man. Exp. Brain Res. 124, 59–68. doi: 10.1007/s002210050600
Pitcher, J. B., Ogston, K. M., and Miles, T. S. (2003). Age and sex differences in human motor cortex input-output characteristics. J. Physiol. 546, 605–613. doi: 10.1113/jphysiol.2002.029454
Raglin, J. S., Koceja, D. M., Stager, J. M., and Harms, C. A. (1996). Mood, neuromuscular function, and performance during training in female swimmers. Med. Sci. Sports Exercise 28, 372–377. doi: 10.1097/00005768-199603000-00013
Rossini, P. M., Desiato, M. T., and Caramia, M. D. (1992). Age-related changes of motor evoked potentials in healthy humans: non-invasive evaluation of central and peripheral motor tracts excitability and conductivity. Brain Res. 593, 14–19. doi: 10.1016/0006-8993(92)91256-E
Rozand, V., Senefeld, J. W., Sundberg, C. W., Smith, A. E., and Hunter, S. K. (2019). Differential effects of aging and physical activity on corticospinal excitability of upper and lower limb muscles. J. Neurophysiol. 122, 241–250. doi: 10.1152/jn.00077.2019
Ruffieux, J., Mouthon, A., Keller, M., Wälchli, M., and Taube, W. (2017). Behavioral and neural adaptations in response to five weeks of balance training in older adults: a randomized controlled trial. J. Negative Results BioMed. 16, 1–9. doi: 10.1186/s12952-017-0076-1
Sabbahi, M. A., and Sedgwick, E. M. (1982). Age-related changes in monosynaptic reflex excitability. J. Gerontol. 37, 24–32. doi: 10.1093/geronj/37.1.24
Sacheli, L. M., Zapparoli, L., Bonandrini, R., Preti, M., Pelosi, C., Sconfienza, L. M., et al. (2020). How aging affects the premotor control of lower limb movements in simulated gait. Human Brain Mapping 41, 1889–1903. doi: 10.1002/hbm.24919
Sale, M. Y., and Semmler, J. G. (2005). Age-related differences in corticospinal control during functional isometric contractions in left and right hands. J. Appl. Physiol. 99, 1483–1493. doi: 10.1152/japplphysiol.00371.2005
Scaglioni, G., Narici, M. V., Maffiuletti, N. A., Pensini, M., and Martin, A. (2003). Effect of ageing on the electrical and mechanical properties of human soleus motor units activated by the H reflex and M wave. J. Physiol. 548, 649–661. doi: 10.1113/jphysiol.2002.032763
Sherrington, C., Fairhall, N., Wallbank, G., Tiedemann, A., Michaleff, Z. A., Howard, K., et al. (2020). Exercise for preventing falls in older people living in the community: an abridged Cochrane systematic review. Br. J. Sports Med. 54, 885–891. doi: 10.1136/bjsports-2019-101512
Sherrington, C., Whitney, J. C., Lord, S. R., Herbert, R. D., Cumming, R. G., and Close, J. C. T. (2008). Effective exercise for the prevention of falls: a systematic review and meta-analysis. J. Am. Geriatrics Soc. 56, 2234–2243. doi: 10.1111/j.1532-5415.2008.02014.x
Solopova, I. A., Kazennikov, O. V., Deniskina, N. B., Levik, Y. S., and Ivanenko, Y. P. (2003). Postural instability enhances motor responses to transcranial magnetic stimulation in humans. Neurosci. Lett. 337, 25–28. doi: 10.1016/S0304-3940(02)01297-1
Solopova, I. A., Selinov, V. A., O.Blinov, E., Dolinskaya, I. Y., Zhvansky, D. S., Lacquaniti, F., et al. (2022). Higher responsiveness of pattern generation circuitry to sensory stimulation in healthy human is associated with a larger Hoffmann reflex. Biology. 11, 707. doi: 10.3390/biology11050707
Stevens-Lapsley, J. E., Thomas, A. C., Hedgecock, J. B., and Kluger, B.M. (2013). Corticospinal and intracortical excitability of the quadriceps in active older and younger healthy adults. Arch. Gerontol. Geriatrics 56, 279–284. doi: 10.1016/j.archger.2012.06.017
Talelli, P., Waddingham, W., Ewas, A., Rothwell, J. C., and Ward, N. S. (2008). The effect of age on task-related modulation of interhemispheric balance. Exp. Brain Res. 186, 59–66. doi: 10.1007/s00221-007-1205-8
Taube, W. (2012). Neurophysiologiscal adaptations in response to balance training. Deutsche Zeitschrift fur Sportmedizin 63, 273–277. doi: 10.5960/dzsm.2012.030
Taube, W., Gruber, M., Beck, S., Faist, M., Gollhofer, A., and Schubert, M. (2007). Cortical and spinal adaptations induced by balance training: correlation between stance stability and corticospinal activation. Acta Physiol. 189, 347–358. doi: 10.1111/j.1748-1716.2007.01665.x
Taube, W., Gruber, M., and Gollhofer, A. (2008). Spinal and supraspinal adaptations associated with balance training and their functional relevance. Acta Physiol. 193, 101–116. doi: 10.1111/j.1748-1716.2008.01850.x
Tokuno, C. D., Cresswell, A. G., Thorstensson, A., and Carpenter, M. G. (2010). Age-related changes in postural responses revealed by support-surface translations with a long acceleration-deceleration interval. Clin. Neurophysiol. 121, 109–117. doi: 10.1016/j.clinph.2009.09.025
Vandervoort, A. A., and Hayes, K. C. (1989). Plantarflexor muscle function in young and elderly women. Eur. J. Appl. Physiol. Occup. Physiol. 58, 389–394. doi: 10.1007/BF00643514
Zech, A., Hübscher, M., Vogt, L., Banzer, W., Hänsel, F., and Pfeifer, K. (2010). Balance training for neuromuscular control and performance enhancement: a systematic review. J. Athletic Training 45, 392–403. doi: 10.4085/1062-6050-45.4.392
Ziemann, U. (2013). Pharmaco-transcranial magnetic stimulation studies of motor excitability. Handbook Clin. Neurol. 116, 387–397. doi: 10.1016/B978-0-444-53497-2.00032-2
Keywords: postural control, postural equilibrium, exercise, spinal reflexes, corticospinal excitability
Citation: Sun Y, Hurd CL, Barnes MM and Yang JF (2022) Neural Plasticity in Spinal and Corticospinal Pathways Induced by Balance Training in Neurologically Intact Adults: A Systematic Review. Front. Hum. Neurosci. 16:921490. doi: 10.3389/fnhum.2022.921490
Received: 15 April 2022; Accepted: 20 June 2022;
Published: 17 August 2022.
Edited by:
Nadia Dominici, VU Amsterdam, NetherlandsReviewed by:
Yury Ivanenko, Santa Lucia Foundation (IRCCS), ItalyBenedikt Lauber, University of Fribourg, Switzerland
Alejandro Jose Lopez, Emory University, United States
Copyright © 2022 Sun, Hurd, Barnes and Yang. This is an open-access article distributed under the terms of the Creative Commons Attribution License (CC BY). The use, distribution or reproduction in other forums is permitted, provided the original author(s) and the copyright owner(s) are credited and that the original publication in this journal is cited, in accordance with accepted academic practice. No use, distribution or reproduction is permitted which does not comply with these terms.
*Correspondence: Jaynie F. Yang, jaynie@ualberta.ca
†ORCID: Yao Sun orcid.org/0000-0002-0178-3612
Caitlin L. Hurd orcid.org/0000-0003-3719-864X
Michelle M. Barnes orcid.org/0000-0001-5298-6025
Jaynie F. Yang orcid.org/0000-0002-7507-8924