- 1Department of Clinical Neuroscience, Institute of Neuroscience and Physiology, Sahlgrenska Academy, University of Gothenburg, Gothenburg, Sweden
- 2Center for Bionics and Pain Research, Mölndal, Sweden
- 3Department of Orthopaedics, Institute of Clinical Sciences, Sahlgrenska Academy, University of Gothenburg, Mölndal, Sweden
- 4Section of Neurocare, Sahlgrenska University Hospital, Gothenburg, Sweden
- 5Department of Occupational Therapy and Physiotherapy, Sahlgrenska University Hospital, Gothenburg, Sweden
- 6Operational Area 3, Sahlgrenska University Hospital, Mölndal, Sweden
- 7Department of Electrical Engineering, Chalmers University of Technology, Gothenburg, Sweden
Background: Upper limb impairment is common after stroke, and many will not regain full upper limb function. Different technologies based on surface electromyography (sEMG) have been used in stroke rehabilitation, but there is no collated evidence on the different sEMG-driven interventions and their effect on upper limb function in people with stroke.
Aim: Synthesize existing evidence and perform a meta-analysis on the effect of different types of sEMG-driven interventions on upper limb function in people with stroke.
Methods: PubMed, SCOPUS, and PEDro databases were systematically searched for eligible randomized clinical trials that utilize sEMG-driven interventions to improve upper limb function assessed by Fugl-Meyer Assessment (FMA-UE) in stroke. The PEDro scale was used to evaluate the methodological quality and the risk of bias of the included studies. In addition, a meta-analysis utilizing a random effect model was performed for studies comparing sEMG interventions to non-sEMG interventions and for studies comparing different sEMG interventions protocols.
Results: Twenty-four studies comprising 808 participants were included in this review. The methodological quality was good to fair. The meta-analysis showed no differences in the total effect, assessed by total FMA-UE score, comparing sEMG interventions to non-sEMG interventions (14 studies, 509 participants, SMD 0.14, P 0.37, 95% CI –0.18 to 0.46, I2 55%). Similarly, no difference in the overall effect was found for the meta-analysis comparing different types of sEMG interventions (7 studies, 213 participants, SMD 0.42, P 0.23, 95% CI –0.34 to 1.18, I2 73%). Twenty out of the twenty-four studies, including participants with varying impairment levels at all stages of stroke recovery, reported statistically significant improvements in upper limb function at post-sEMG intervention compared to baseline.
Conclusion: This review and meta-analysis could not discern the effect of sEMG in comparison to a non-sEMG intervention or the most effective type of sEMG intervention for improving upper limb function in stroke populations. Current evidence suggests that sEMG is a promising tool to further improve functional recovery, but randomized clinical trials with larger sample sizes are needed to verify whether the effect on upper extremity function of a specific sEMG intervention is superior compared to other non-sEMG or other type of sEMG interventions.
Introduction
Stroke is one of the leading causes of disability in adults (Feigin et al., 2017). Approximately 50 to 70% of individuals with stroke demonstrate upper limb impairment in the acute phase (Persson et al., 2012; Raffin and Hummel, 2018), and only 5 to 20% regain full upper limb dexterity 6 months after the onset of stroke (Nijland et al., 2010). Upper limb impairment limits activities of daily living and participation in different social contexts and physical environments (Foley et al., 2019).
Upper limb rehabilitation is crucial to maximize outcomes and decrease disability (Pollock et al., 2013; Hatem et al., 2016). Along with traditional clinical interventions, several technologies such as neuromuscular stimulation, invasive and non-invasive brain stimulation, robotic devices, virtual reality gaming, and electromyography (EMG) exist to enhance recovery after stroke (Pollock et al., 2013; Hatem et al., 2016). Each technology has advantages and disadvantages and relies on different rehabilitation approaches to improve upper limb function.
Surface EMG (sEMG), in which electrodes placed over the skin record the electrical activity of a muscle or group of muscles, has been used for neurorehabilitation for more than five decades (Criswell, 2010; Merletti and Farina, 2016; Campanini et al., 2020). sEMG can be applied as an assessment to evaluate muscle activation patterns or as a tool to complement and enhance different neuromuscular rehabilitation interventions (Criswell, 2010; Merletti and Farina, 2016; Campanini et al., 2020; Mcmanus et al., 2020). sEMG is an objective method that provides real-time information on muscle activity in terms of timing, location, and contraction intensity (Criswell, 2010; Merletti and Farina, 2016; Mcmanus et al., 2020). One advantage of the sEMG is that it can record muscle activity even when no visible movement or palpable muscle activity is present (Campanini et al., 2020; Cappellini et al., 2020), which widens the possible application areas (Criswell, 2010; Merletti and Farina, 2016; Campanini et al., 2020; Mcmanus et al., 2020).
In stroke, sEMG has been used for upper limb rehabilitation since the beginning of the 90s (Merletti and Farina, 2016). The visual and/or auditory biofeedback on muscle activity provided by an sEMG system has been used to enhance motor function and facilitate learning towards a more effective use of the affected limb (Schleenbaker and Mainous, 1993; Woodford and Price, 2007; Giggins et al., 2013). In addition, sEMG has been applied to trigger neuromuscular electrical stimulation (NMS) for specific target muscle groups to promote upper limb function in stroke (Takeda et al., 2017; Monte-Silva et al., 2019). Furthermore, electromechanical and robot-assisted arm training using sEMG signals to drive robotic devices, such as an exoskeleton or active orthosis, has shown to improve upper limb function and performance in activities of daily living (Norouzi-Gheidari et al., 2012; Mehrholz et al., 2015; Hameed et al., 2020). The feedback provided by sEMG can increase awareness, self-regulation, precision, and control of muscle contraction in real-time, which might improve compliance and motivation (Criswell, 2010; Merletti and Farina, 2016; Mcmanus et al., 2020).
Taking the large variety of clinical application areas of sEMG, more knowledge is needed to better understand the benefits, possibilities, and potential clinical effects of the different types of sEMG interventions on motor recovery after stroke (Campanini et al., 2020; Mcmanus et al., 2020). Previous reviews and meta-analyses have exclusively been dedicated to one specific type of sEMG intervention (Schleenbaker and Mainous, 1993; Bolton et al., 2004; Woodford and Price, 2007; Meilink et al., 2008; Norouzi-Gheidari et al., 2012; Basteris et al., 2014; Mehrholz et al., 2015; Eraifej et al., 2017; Takeda et al., 2017; Monte-Silva et al., 2019; Yang et al., 2019; Hameed et al., 2020), and summarized knowledge on the effect of different types of sEMG-driven interventions on upper limb function in stroke is limited. Thus, the aim of this systematic review and meta-analysis is to synthesize existing evidence on the effect of different sEMG-driven interventions on upper limb impairment in people with stroke and identify which type of sEMG intervention could be beneficial for this purpose.
Materials and Methods
The present systematic review and meta-analysis was registered in the PROSPERO database prior analysis (CRD42021243372), and the PRISMA 2020 statement was followed (Page et al., 2021).
Search Strategy
One of the authors (MM-N) conducted an online search in PubMed, Scopus, and PEDro databases, including relevant articles in English published between January 2000 and May 2021. The search terms used are shown in Table 1. In addition, the references of identified relevant papers and existing systematic reviews and meta-analyses in the field were screened (Schleenbaker and Mainous, 1993; Bolton et al., 2004; Woodford and Price, 2007; Meilink et al., 2008; Norouzi-Gheidari et al., 2012; Basteris et al., 2014; Mehrholz et al., 2015; Eraifej et al., 2017; Takeda et al., 2017; Monte-Silva et al., 2019; Yang et al., 2019; Hameed et al., 2020; Doumas et al., 2021).
Selection Criteria
Studies were eligible for inclusion if participants were older than 18 years and had an upper limb motor impairment due to stroke. Only randomized control trials (RCT) using sEMG interventions to improve upper limb function were included. The sEMG signal needed to be obtained from the upper limb, and the protocol was required to explicitly state the number of sessions, time, and frequency of treatment. Fugl-Meyer Assessment of Upper Extremity (FMA-UE) (Fugl-Meyer et al., 1975) needed to be one of the included outcomes. The FMA-UE is a recommended assessment for upper limb sensorimotor function in stroke trials and is considered as a gold standard (Fugl-Meyer et al., 1975; Gladstone et al., 2002; Hernández et al., 2019). Studies were excluded if sEMG signal recording was only used as an assessment instead of an intervention tool or if the sEMG intervention was not the study primary focus. For the studies where the FMA-UE total score was not specified, the corresponding author was contacted. Studies were excluded from the meta-analysis if it was not possible to obtain the total FMA-UE score.
One reviewer performed the screening for title and abstract for relevance (MM-N). Following, full texts of all potentially pertinent articles were reviewed by two authors (MM-N, MBK). Then, the two reviewers’ opinions were compared, and any disagreement was resolved by a third reviewer (MAM).
Data Extraction
The data from the included studies were extracted by one reviewer (MM-N) and independently checked by a second reviewer (MBK). The extracted data included: study characteristics (study design, year published, inclusion/exclusion criteria, and sample size), participant characteristics (stroke onset, upper limb impairment), interventions (type of sEMG intervention, sEMG electrode site, and training description), treatment protocol (number of sessions, frequency, session duration and follow-up times), setting (hospital, laboratory, or home), outcomes (FMA-UE scores on control and experimental group at all time points), main results and conclusions.
Time since stroke was classified as acute (< 7 days), subacute (7 days to 6 months), and chronic (≥ 6 months) (Hatem et al., 2016). The upper limb impairment was categorized according to the baseline mean value of the total FMA-UE scores reported in the articles. FMA-UE score < 31 was defined as “severe”, 32-57 as “moderate” and 58-66 as “mild” impairment (Pang et al., 2006; Persson et al., 2015; Bustrén et al., 2017).
The sEMG interventions used in different studies were categorized into subgroups according to their type of application: sEMG-triggered neuromuscular stimulation (sEMG-NMS), sEMG providing visual and/or auditory biofeedback (sEMG-BFB), sEMG-driven robotic device (sEMG-RT), or a combination of those. The control or comparison interventions that did not include sEMG were categorized as non-sEMG interventions (e.g., conventional care, cyclic NMS, passive motion robot).
For studies comparing different sEMG interventions protocols with each other, the interventions were categorized as Intervention 1 when the sEMG interventions was utilized alone, and as Intervention 2 when the sEMG intervention was combined with another training modality (e.g., mirror therapy, task-oriented training, mental imagery).
Methodological Quality Assessment
The methodological quality of the randomized controlled trials was assessed independently by two reviewers (MBK, MM-N) using the Physiotherapy Evidence Database (PEDro) scale. This scale is used to evaluate the quality and potential risk of bias of randomized controlled trials (Maher et al., 2003; Moseley et al., 2019). A third reviewer (MAM) was consulted in case of any discrepancy. PEDro scores of 9 to 10 were considered as “excellent,” 6 to 8 as “good”, 4 to 5 as “fair”, and below 4 as “poor” quality (Maher et al., 2003; Moseley et al., 2019). Only studies with a PEDro score 4 and more were included for the meta-analysis.
Data Analysis
The included studies comparing sEMG interventions with non-sEMG interventions and studies comparing sEMG interventions with another sEMG intervention protocol were summarized and analyzed separately. For studies comparing more than two intervention protocols, each comparison was included independently under the respective type of sEMG intervention.
In the meta-analysis, the effect size of each study was determined using the Hedges’ g measure (Harrer et al., 2021). In case a study did not provide the standard deviation (SD) of the change from baseline, the data were imputed using the Cochrane correlation method (Higgins et al., 2019). The standardized mean difference (SMD) with 95% confidence intervals was used for the pooled effect using the FMA-UE total score. Statistical heterogeneity was calculated using the I2 test (Higgins et al., 2019; Deeks et al., 2021; Harrer et al., 2021). Heterogeneity values between 30 and 60% suggest moderate heterogeneity, 50%-90% might represent substantial heterogeneity, and 75-100% represent considerable heterogeneity (Higgins et al., 2019). A random-effect model was used (Higgins et al., 2019; Deeks et al., 2021; Harrer et al., 2021). Sub-group analyses among the different types of sEMG intervention were also performed. The meta-analysis and forest plots were produced with R software and R studio statistical program (version R-4.0.5) (Harrer et al., 2021).
Results
Included Studies and Methodological Quality Assessment
In total, 2021 articles were identified using the search strategy (Figure 1). After the screening for eligibility, 24 studies from 10 countries were included in the systematic review. Of those, 14 studies comparing sEMG with non-sEMG interventions and 7 studies comparing sEMG interventions with another sEMG intervention protocol were included in the meta-analysis. One study with three intervention groups (Page et al., 2020) was included in the analysis of sEMG compared with non-sEMG as well as in the analysis of sEMG intervention compared to another sEMG intervention. Four studies were not included in the meta-analysis, of which two did not provide the total FMA-UE score (Hu et al., 2009, 2015), and two used the same type of sEMG intervention in both groups and compared other aspects of the intervention (immediate versus delayed protocol and robotic assistance applied at upper arm versus assistance applied at wrist level, respectively) (Kojima et al., 2014; Qian et al., 2019). According to the PEDro scale 18 RCTs showed good and 6 RCTs fair methodological quality (Table 2).
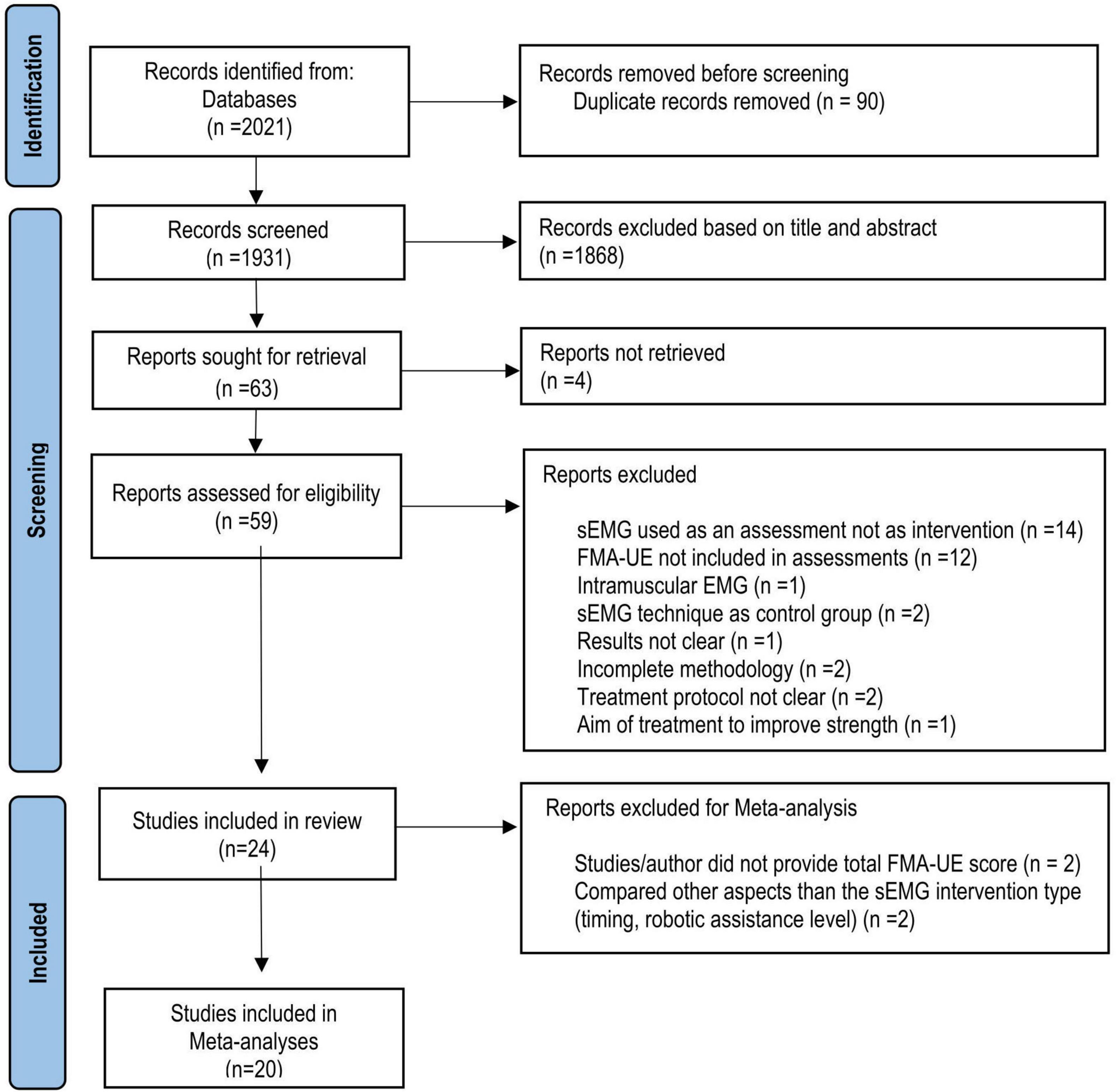
Figure 1. Flow chart (PRISMA) of study selection. RCT, Randomized clinical trial; sEMG; surface electromyography, FMA-UE, Fugl-Meyer Assessment for the upper extremity.
Characteristics of the Included Studies
Table 3 summarizes the studies that compared sEMG interventions with non-sEMG interventions, and Table 4 shows the studies that compared the sEMG interventions alone with the sEMG interventions combined with another training modality. One study (Page et al., 2020), reporting comparisons both with a non-sEMG intervention and with another sEMG intervention, was included in both tables.
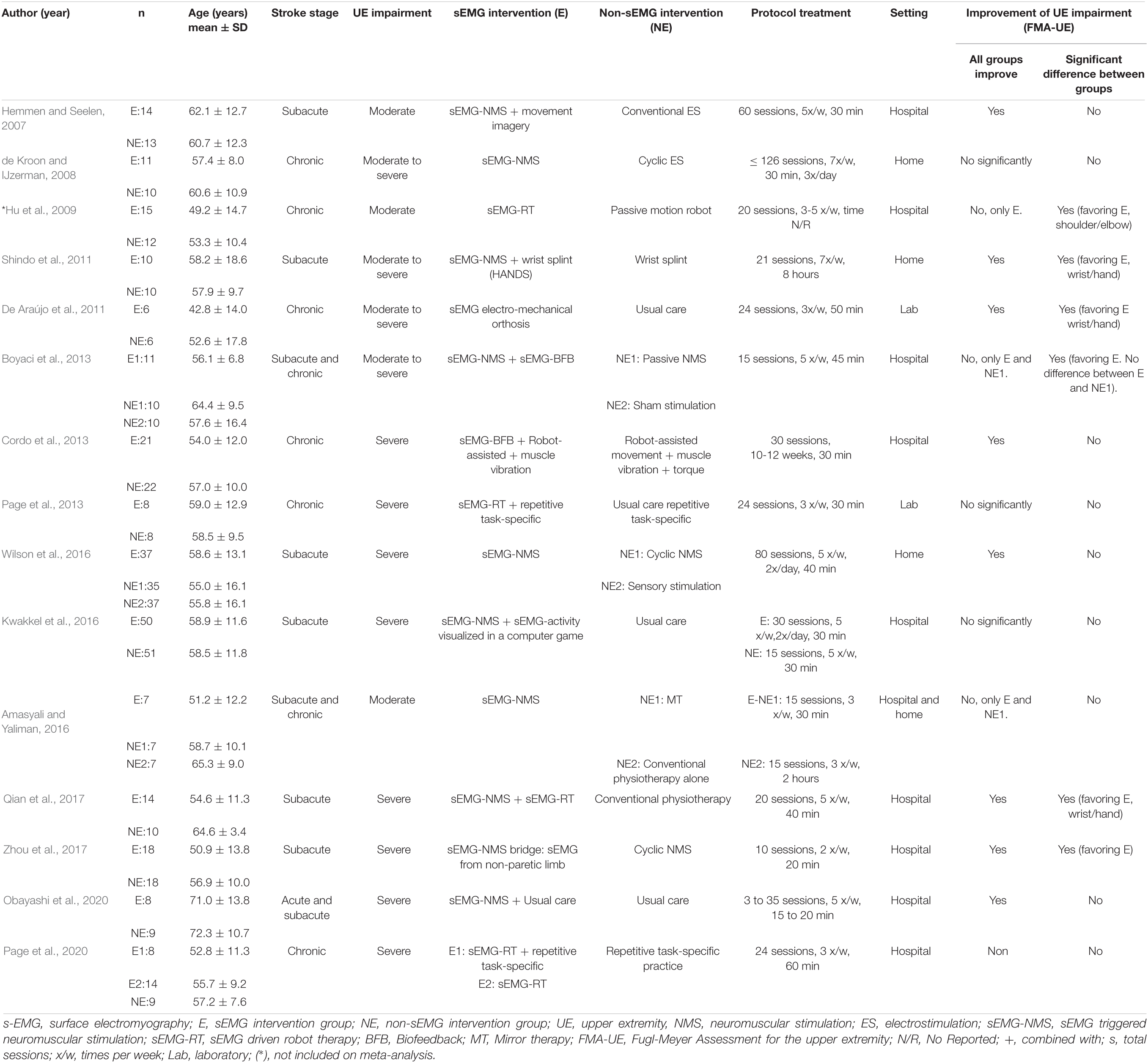
Table 3. Characteristics of the included studies comparing sEMG intervention with non-sEMG intervention.
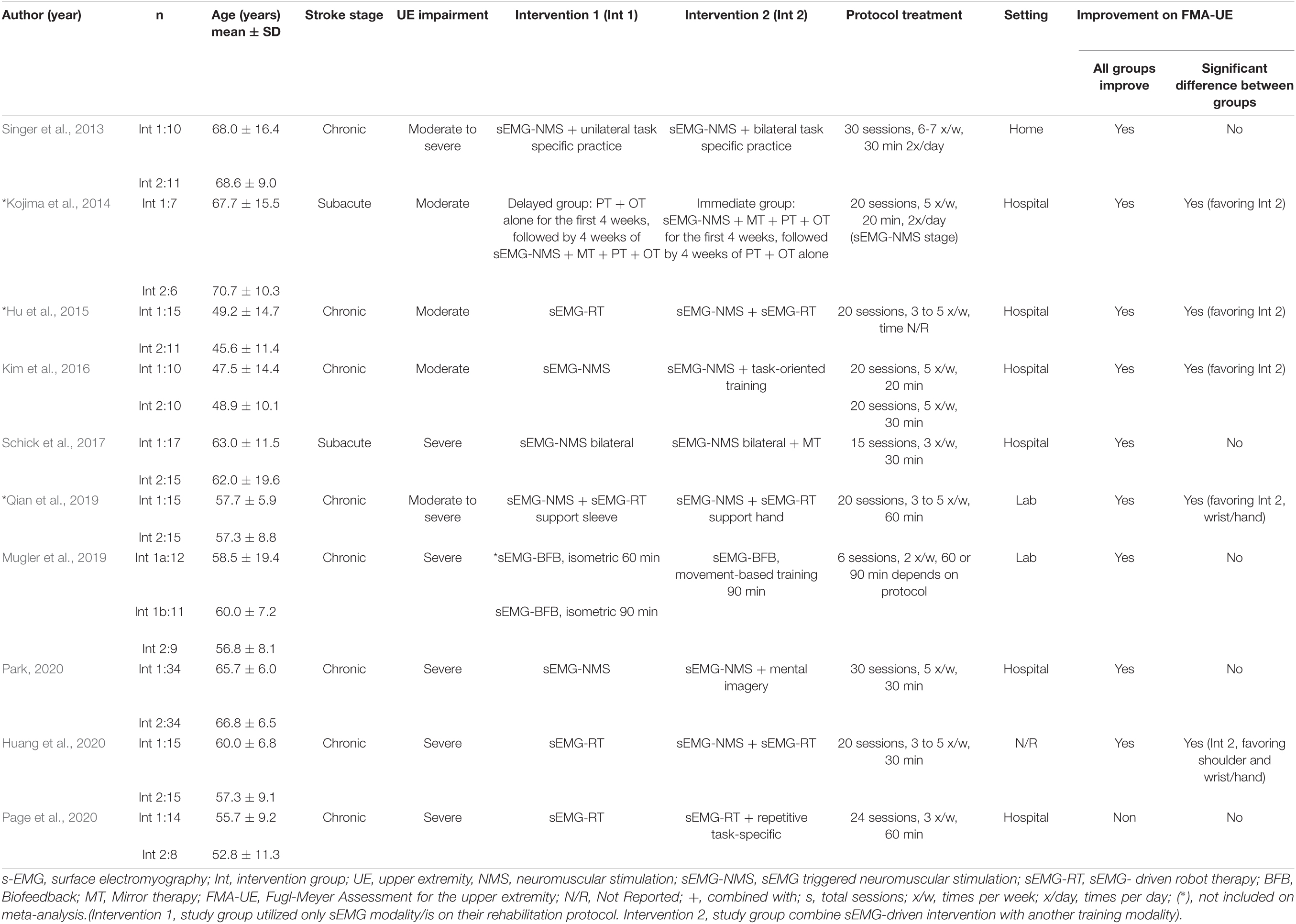
Table 4. Characteristics of the included studies comparing sEMG intervention with another sEMG intervention.
In total, 808 participants from 24 studies were included. The mean age of the included study groups, as reported by RCTs, varied between 42 and 72 years. The sample sizes varied from 6 to 51 participants for each intervention group. The participants were included in the chronic (n = 13), subacute (n = 8), mixed subacute/chronic (n = 2), or acute/subacute (n = 1) stage of stroke recovery (Figure 2). According to the total FMA-UE score, 12 studies included participants with severe, 6 with severe to moderate, and 6 with moderate upper extremity impairment (Figure 3). More than half of the studies (n = 14) were conducted in hospital settings, followed by research laboratory (n = 4), home (n = 4), and mixed home and hospital setting (n = 1). One study did not report their treatment location (Huang et al., 2020). Half of the studies (n = 12) used sEMG to trigger neuromuscular stimulation, 6 used sEMG to drive robotic devices, 2 to provide visual and/or auditory biofeedback, and 6 studies combined different types of sEMG interventions (Figure 4). As illustrated in Figure 4, the number of publications evaluating the single or combined effect of sEMG interventions on upper extremity function has increased over the past years.
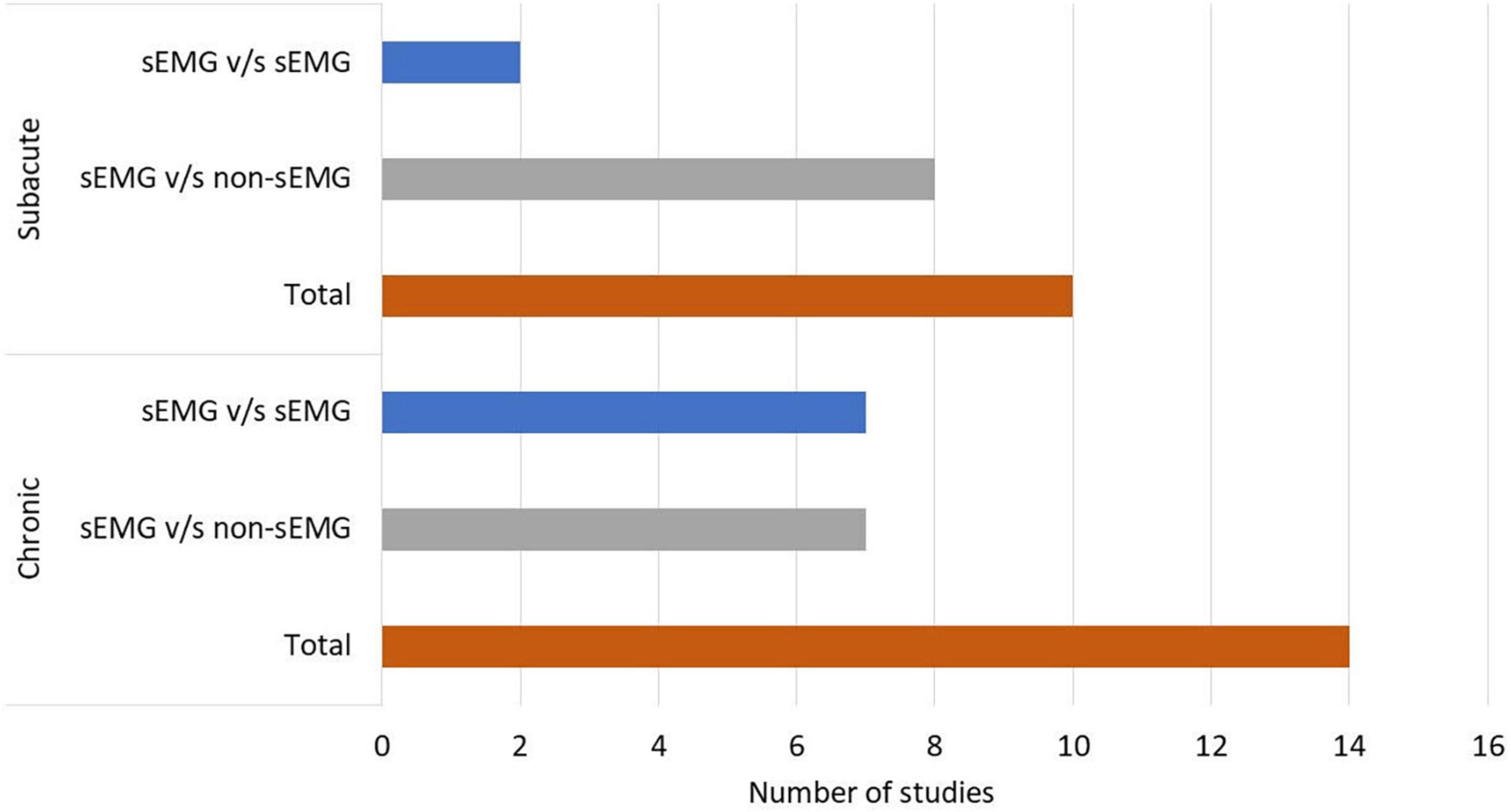
Figure 2. Number of studies performed in patients with chronic and subacute stroke. s-EMG v/s sEMG, studies comparing different surface electromyography driven interventions; s-EMG v/s non-sEMG, studies comparing surface electromyography driven interventions with non-surface electromyography driven groups.
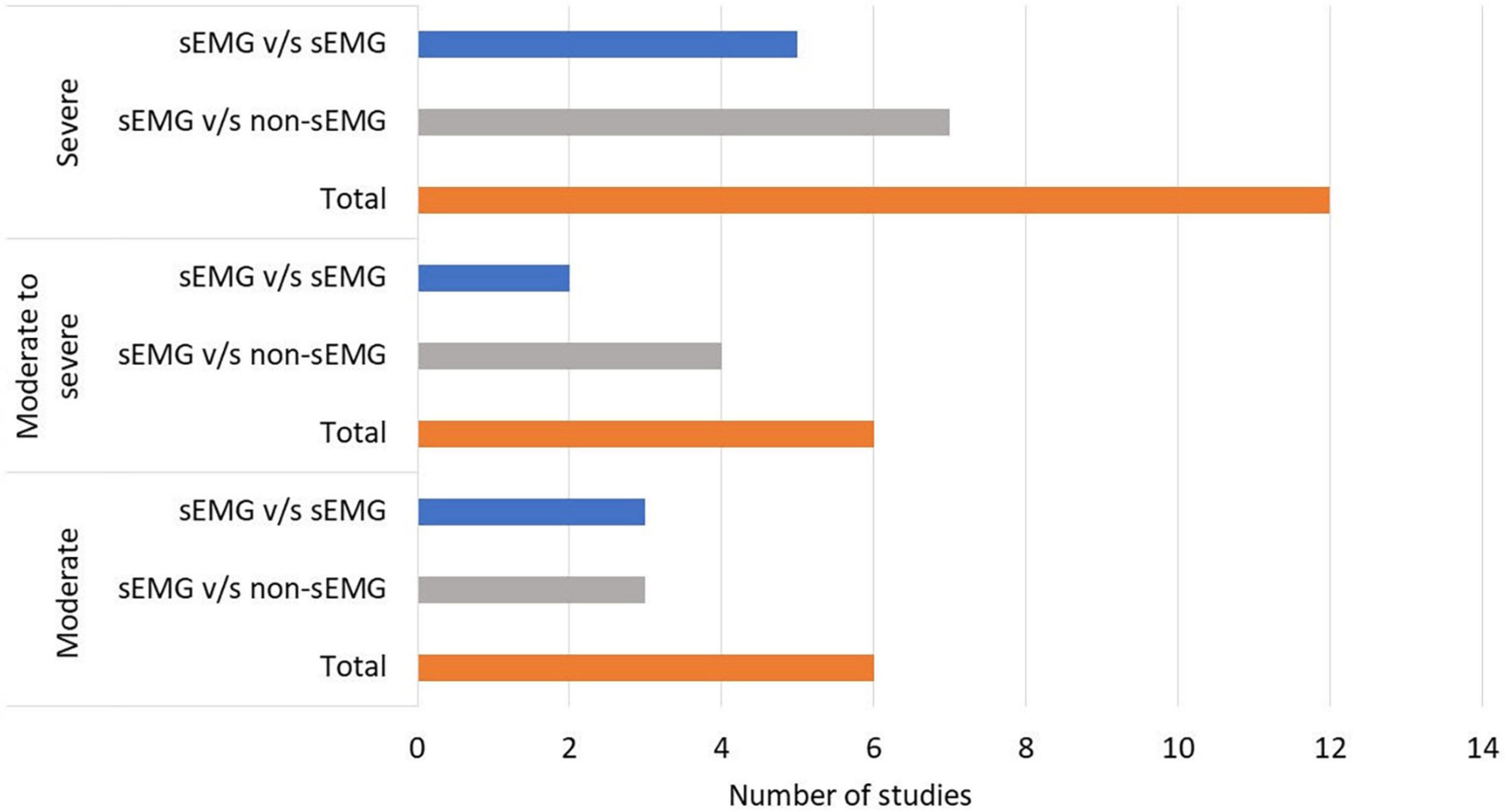
Figure 3. Number of studies performed in patients with moderate to severe upper limb impairment. s-EMG v/s sEMG, studies comparing different surface electromyography driven interventions; s-EMG v/s non-sEMG, studies comparing surface electromyography driven interventions with non-surface electromyography driven groups.
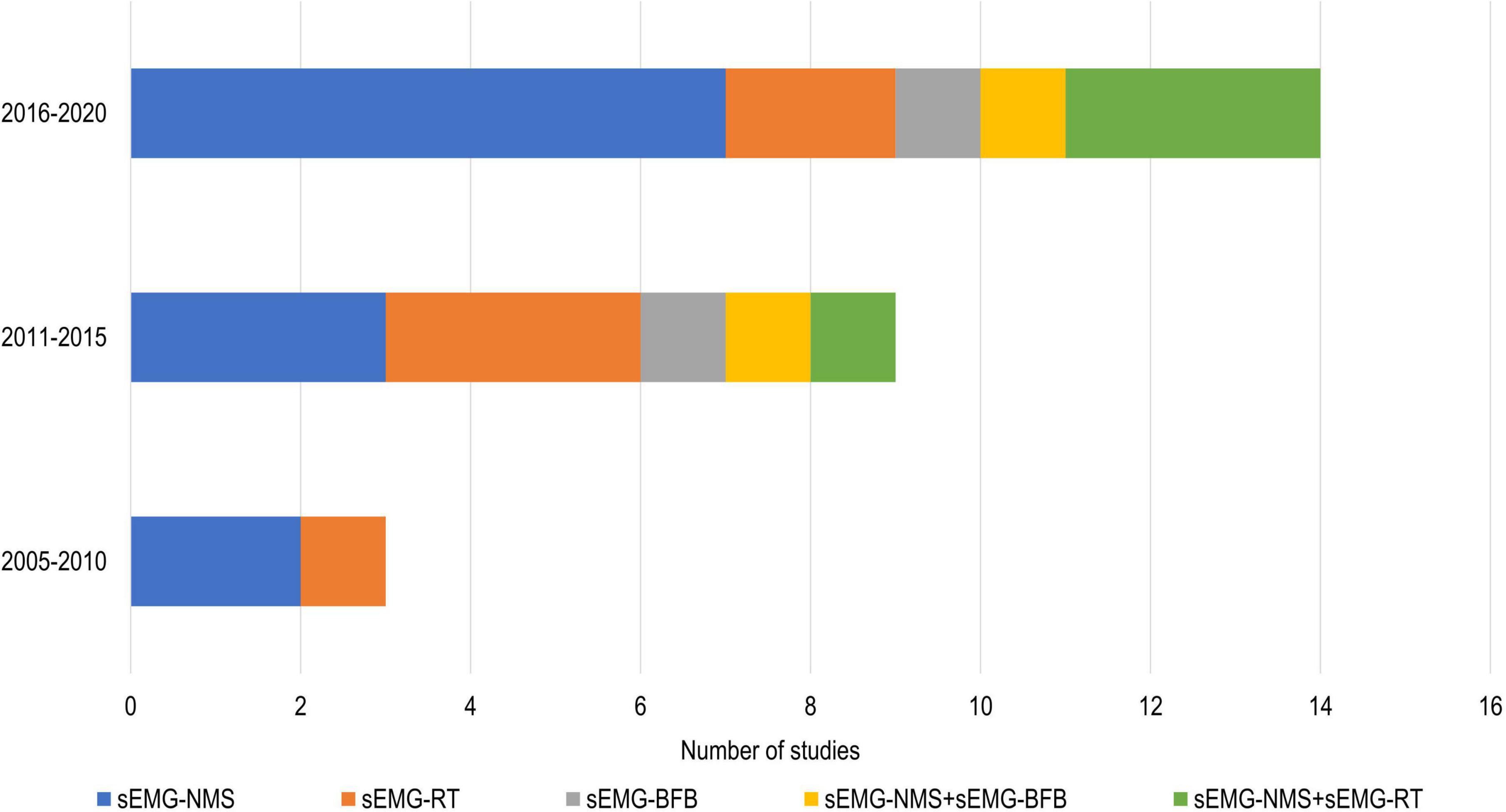
Figure 4. Number of studies using different sEMG interventions shown over time. s-EMG, surface electromyography; sEMG-NMS, sEMG triggered neuromuscular stimulation; sEMG-RT, s-EMG driven robot therapy; sEMG-BFB, sEMG providing visual and/or auditory biofeedback; +, combine with.
The average dose of the interventions, as reported in the included studies, was 27 sessions provided 4 times per week for about 54 min per session (Tables 3, 4). However, one study provided a high-intensity training 7 times a week, 3 times a day for 30 min each time (de Kroon and IJzerman, 2008), and another study (Obayashi et al., 2020) had an individualized dose protocol varying from 3 to 35 sessions depending on the participant.
Half of the studies (n = 12) (Hemmen and Seelen, 2007; de Kroon and IJzerman, 2008; Hu et al., 2009, 2015; Singer et al., 2013; Amasyali and Yaliman, 2016; Kwakkel et al., 2016; Wilson et al., 2016; Qian et al., 2017, 2019; Mugler et al., 2019; Huang et al., 2020) reported follow-up data, and only two trials (Kwakkel et al., 2016; Wilson et al., 2016) reported more than one follow-up session. A follow-up at 3 months after the intervention was the most common. Only two studies (Amasyali and Yaliman, 2016; Kwakkel et al., 2016) reported a decline of the main outcome at follow-up. The sEMG signal source was commonly obtained from muscles at the wrist (n = 15), followed by both elbow and wrist (n = 5) (Hemmen and Seelen, 2007; de Kroon and IJzerman, 2008; Hu et al., 2009; De Araújo et al., 2011; Huang et al., 2020), elbow (n = 2) (Page et al., 2013, 2020), and all parts of the upper limb (n = 2) (Mugler et al., 2019; Obayashi et al., 2020). Half of the studies (n = 12) (De Araújo et al., 2011; Shindo et al., 2011; Cordo et al., 2013; Page et al., 2013, 2020; Singer et al., 2013; Kim et al., 2016; Kwakkel et al., 2016; Qian et al., 2017, 2019; Schick et al., 2017; Mugler et al., 2019) incorporated functional task to their sEMG training protocol, such as, grasp and release objects (Singer et al., 2013; Qian et al., 2017), pushing objects (Kim et al., 2016), virtual reality training (Kwakkel et al., 2016; Mugler et al., 2019), daily life activities (Shindo et al., 2011), and others (Page et al., 2013; Qian et al., 2019). The majority of the studies (n = 20) reported statistically significant improvements in FMA-UE scores compared to the baseline scores (Tables 3, 4).
Meta-Analysis
Surface Electromyography Interventions in Comparison With Non-sEMG Interventions
The meta-analysis revealed no differences for the total effect of sEMG interventions compared to non-sEMG interventions on the total FMA-UE score (14 studies, 509 participants, SMD 0.14, P 0.37, 95% CI –0.18 to 0.46, I2 55%, Figure 5). Moderate heterogeneity was present in the total pooled analysis (Higgins et al., 2019). No difference was found at the subgroup level when comparing the different types of sEMG interventions with non-sEMG interventions. Two studies, one that utilized sEMG-NMS (Shindo et al., 2011) and one that combined sEMG-NMS with sEMG-driven robotic device (Qian et al., 2017), showed a statistically significant effect on improving upper limb function compared to a non-sEMG intervention.
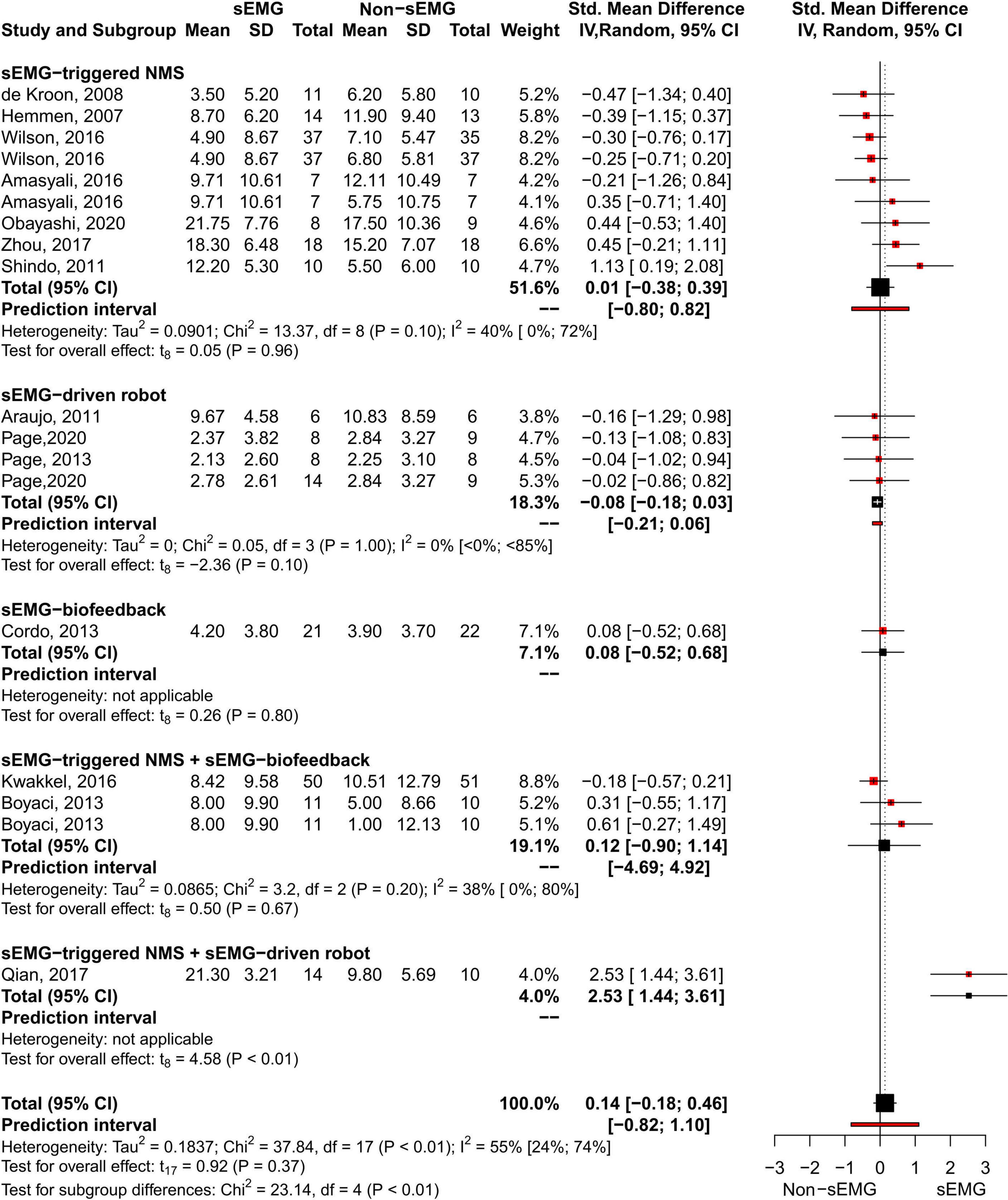
Figure 5. Meta-analyses of the effect of sEMG intervention versus non-sEMG intervention on total FMA-UE score. s-EMG, surface electromyography; NMS, neuromuscular stimulation; +, combine with.
sEMG Intervention in Comparison With Another sEMG Interventions
No difference in the overall and subgroup effect according to the total FMA-UE score was found in the meta-analysis comparing training protocols including sEMG interventions alone (Intervention 1) to protocols combining sEMG interventions with another training modality (Intervention 2) (7 studies, 213 participants, SMD 0.42, P 0.23, 95% CI –0.34 to 1.18, I2 73%, Figure 6). Substantial heterogeneity was found for the overall result (Higgins et al., 2019). Two studies (Kim et al., 2016; Huang et al., 2020) showed an effect favoring Intervention 2. One of the studies (Huang et al., 2020) compared sEMG-driven robotic intervention alone to sEMG-driven robotic combined with sEMG-NMS, and the other study (Kim et al., 2016) compared sEMG-NMS to sEMG-NMS combined with task-oriented training. Moreover, the most common type of sEMG intervention in both meta-analyses was sEMG-NMS intervention.
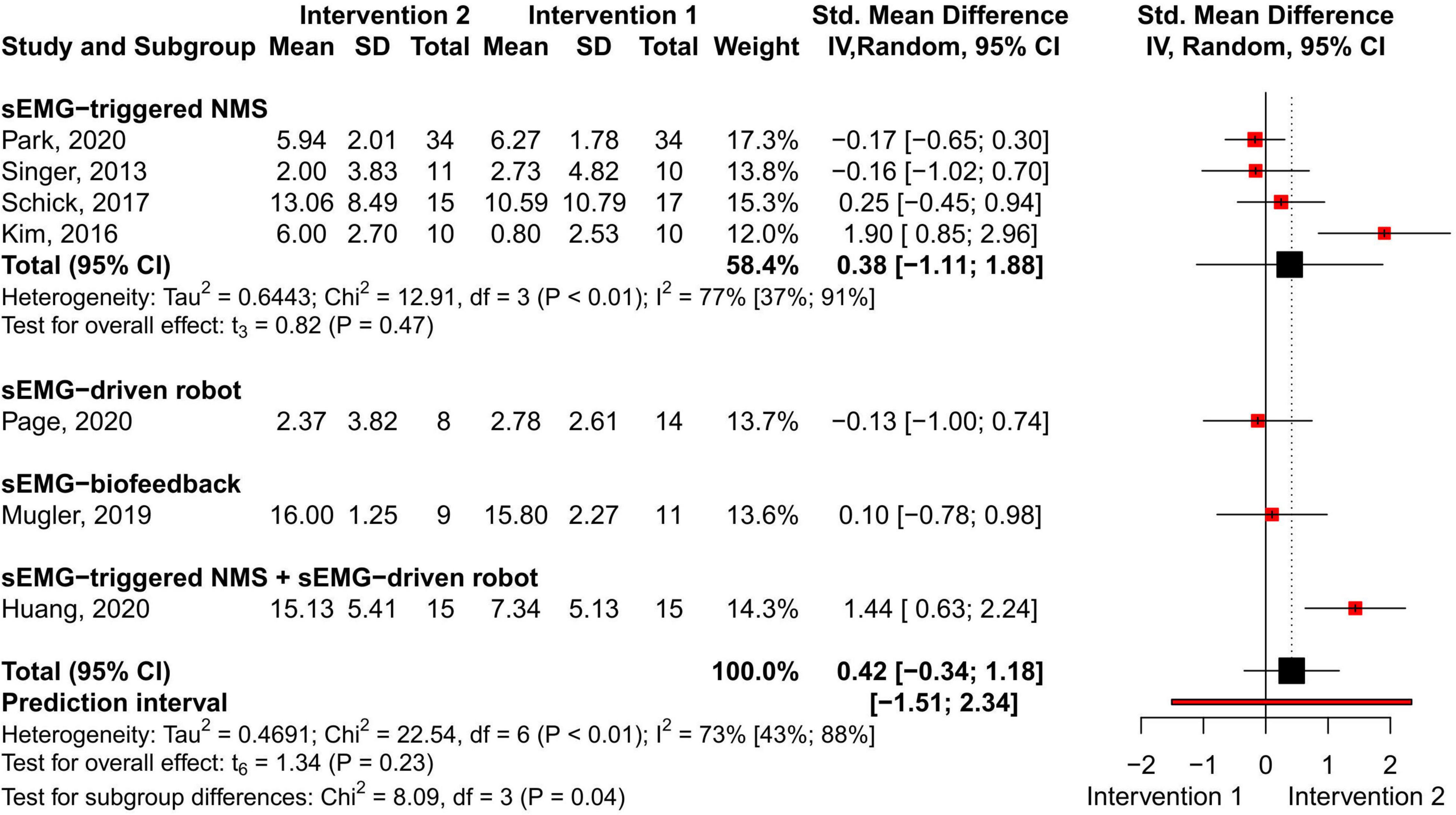
Figure 6. Meta-analyses of the effect of sEMG intervention versus another sEMG intervention on total FMA-UE score. s-EMG, surface electromyography; NMS, neuromuscular stimulation; +, combine with.
Discussion
The present systematic review and meta-analysis synthesizes the effects of different surface electromyography-driven interventions on upper limb function in people with stroke. A total of 24 studies (n = 808) were included in the systematic review, and 20 studies were included in the meta-analyses. The results of the meta-analyses revealed no differences for the total effect of sEMG interventions compared to non-sEMG interventions nor for studies comparing sEMG interventions with another sEMG intervention protocol when assessed by FMA-UE total score. Moreover, it is worth to notice that sEMG is not an intervention used in solitary but a tool to complement and enhance treatment effects of different neuromuscular rehabilitation interventions.
To the best of our knowledge, this is the first systematic review and meta-analysis summarizing the different sEMG-driven interventions utilized for upper limb rehabilitation in people with stroke. Earlier systematic reviews and meta-analyses have evaluated a single type of sEMG intervention, most commonly sEMG-NMS (Bolton et al., 2004; Meilink et al., 2008; Eraifej et al., 2017; Monte-Silva et al., 2019). Even though we included several types and protocols of sEMG interventions in the current review, the results could not verify which single or combined sEMG-intervention (combined with other sEMG modalities or with another training modality) was most beneficial for upper limb rehabilitation. In one of the meta-analyses, two studies (Shindo et al., 2011; Qian et al., 2017) showed an effect favoring the sEMG intervention over the non-sEMG intervention, and in the other meta-analysis, two studies (Kim et al., 2016; Huang et al., 2020) showed an effect favoring the sEMG intervention combined with another training modality compared to sEMG intervention alone. Furthermore, all included studies had a relatively small sample sizes (6 to 51 participants per group), the number of comparisons available for subgroup analysis was low (1 to 9 studies), and moderate to substantial heterogeneity was observed. All these factors need to be considered when interpreting the results.
A recent meta-analysis by Monte-Silva et al. (2019), evaluating the effect of sEMG-NMS interventions, showed an effect favoring sEMG-NMS compared to a control group (non-sEMG interventions) for improving upper limb function in people with stroke. In contrast to our results, showing no overall effect assessed by FMA-UE, their meta-analysis included studies using different outcome measures on upper limb function. Another significant difference from our analysis was that the mean difference for the overall effect was calculated between the post-intervention scores and not as a change from the baseline. This means that existing differences between groups at baseline [e.g., 40, 44, 53] were ignored in their analysis. Furthermore, Monte-Silva et al. (2019) used a fixed-effect model, which assumes that all included studies are similar enough and share a true effect size, which is highly unlikely taking the differences in the populations. In our analysis, the change from baseline and a random-effect model was used to estimate the overall effect, which might be the reason for contrasting results. A meta-analysis by Meilink et al. (2008) that included three studies, using FMA-UE as an outcome measure in their subgroup analysis, found no statistically significant differences in upper limb function between sEMG-NMS stimulation and conventional care. Moreover, an earlier meta-analysis by Bolton et al. from 2004 (Bolton et al., 2004), including three RCT and two non-RCT studies with small sample sizes, showed an overall beneficial effect of sEMG-NMS on arm and hand function measured with different clinical scales. Taken the varying results, it can be concluded that sEMG-NMS interventions might have a beneficial effect on improving arm and hand function as measured by FMA-UE, but this effect is not possible to verify when the sEMG interventions are compared to a non-sEMG interventions.
In the subgroup analysis of sEMG-driven robotic device interventions, no difference in the overall effect could be found. The sEMG-driven robotic device intervention has not previously been included in a meta-analysis, even though it has been considered as a potential rehabilitation option for stroke (Basteris et al., 2014). The number of studies using sEMG-driven robotic device has been increasing in the past years, which is promising, especially considering its potential to provide unsupervised intensive and repetitive training (Norouzi-Gheidari et al., 2012). However, the costs and complexity of robot devices might be a barrier for implementation in clinical and home settings.
Two studies that investigated sEMG-visual and/or auditory biofeedback were included in the meta-analysis (Cordo et al., 2013; Mugler et al., 2019). The results showed no difference in effect when compared to a non-sEMG intervention or to a different sEMG intervention protocol. This is in contrast to a small meta-analysis employing older methodology by Schleenbaker et al. from 1993 (Schleenbaker and Mainous, 1993), in which the authors concluded that the sEMG-visual and/or auditory biofeedback can be an effective tool for neuromuscular relearning in stroke (Schleenbaker and Mainous, 1993). Thus, more studies utilizing sEMG-visual and/or auditory biofeedback are needed to determine its effectiveness compared to other interventions.
Among the studies that combined different types of sEMG interventions, two studies (Qian et al., 2017; Huang et al., 2020) combining sEMG-NMS with sEMG-driven robotic devices, showed a favorable effect compared to non-sEMG intervention or sEMG-driven robotic device used alone, respectively. Even when these results are promising, more RCTs using sEMG interventions in combination with other interventions are needed to obtain its effect.
About half of the included studies in this systematic review incorporated functional tasks in their sEMG training protocol. This is in line with current literature which indicates that task-oriented training facilitates motor control, motor learning and brain activation patterns (Hubbard et al., 2009) and, therefore, might have positive impact on upper limb rehabilitation. The results also show that improvements in upper limb function can still be achieved in the chronic phase of stroke (De Araújo et al., 2011; Hu et al., 2015; Kim et al., 2016; Qian et al., 2019; Huang et al., 2020) and that sEMG-driven interventions might be feasible for people with severe upper limb impairment (Qian et al., 2017; Zhou et al., 2017; Huang et al., 2020). This is promising when considering the limited rehabilitation options available for people with chronic and severe upper limb impairments.
Most commonly, the training sessions lasted for 30 min and were applied 3 to 5 times a week, which could be considered as clinically feasible. Only a few studies included a follow-up assessment on their rehabilitation protocol, and therefore an evaluation of long-term effects are not feasible. Furthermore, most of the studies were conducted in the hospital or laboratory settings, and only a few were performed in a home environment. Since the home-based treatments can have several benefits, such as improved adherence, higher dose, and lower cost (Chaiyawat et al., 2009), future studies are needed to evaluate their effect and usefulness for stroke rehabilitation.
Strengths and Limitations
The present systematic review and meta-analysis is, to our knowledge, the first to summarize and analyze the effects of different sEMG-driven interventions utilized for stroke upper limb rehabilitation. Even when the number of identified randomized clinical trials using sEMG-NMS can be considered sufficient in this meta-analysis, the number of studies included in the subgroup analysis was small. Another limitation is the relatively small sample sizes of the included studies. Only one study included 100 participants (Kwakkel et al., 2016), while the majority ranged between 12 to 40 participants. Moreover, substantial to moderate heterogeneity was found on the meta-analyses due to the high methodological variance occurring in the included studies. Also, it needs to be noticed that a variety of non-sEMG interventions were used as a comparison group in the included studies. Finally, it needs to be taken into account that six studies, with “fair” methodological quality according to the PEDro scale, were also included in the meta-analyses.
Clinical Implications
This systematic review and meta-analysis found insufficient evidence to identify which types of sEMG-driven interventions have a beneficial effect on upper limb function compared to non-sEMG interventions. However, most of the sEMG interventions showed a positive effect on upper limb function post-intervention compared to baseline. From a clinical perspective, the positive effect observed in individuals with chronic and severe stroke impairment is promising and calls for further studies in these clinical subgroups.
Conclusion
This review and meta-analysis synthesized the current evidence of different types of sEMG-driven interventions for upper limb rehabilitation in people with stroke. No differences in the total effect (measured by total FMA-UE score) of sEMG interventions compared to non-sEMG interventions, nor sEMG interventions compared to sEMG interventions combined with other therapies, were found in our meta-analyses. Although we included several types of sEMG-driven interventions, the results could not verify which single or combined sEMG-intervention was most beneficial for upper limb function. Notably, most of the included studies reported statistically significant improvements on upper limb function compared to baseline FMA-UE scores, and these effects were shown even on participants with severe stroke in the chronic stage of stroke recovery.
Even though, surface EMG-driven interventions are promising for promoting upper limb function in people with stroke, more randomized controlled trials with larger sample sizes, unified methodology and outcome measures are required to establish the effectiveness of the different types of sEMG interventions in combination with other therapies. Considering the high-intensity training that sEMG interventions can offer, future studies should further explore its effectiveness in home settings, especially in chronic stroke cases.
Data Availability Statement
The original contributions presented in the study are included in the article/supplementary material, further inquiries can be directed to the corresponding author/s.
Author Contributions
MAM, MM-N, MBK, and AN organized the database. MAM, MM-N, and MBK screened the included studies. MM-N performed the meta-analysis and wrote the first draft of the manuscript. All authors contributed to conception and design of the study, manuscript revision, read, and approved the submitted version.
Funding
Support for this study was provided by the Swedish Society for Medical Research (S19-0074), the Swedish state under an agreement between the Swedish government and the county councils, the ALF agreement (ALFGBG-932697 and ALFGBG-879111), Promobilia Foundation (19500), the IngaBritt and Arne Lundbergs Foundation (2020-0), and the Swedish Research Council (Vetenskapsrådet - 2020-04817). The funders had no role in the research.
Conflict of Interest
The authors declare that the research was conducted in the absence of any commercial or financial relationships that could be construed as a potential conflict of interest.
Publisher’s Note
All claims expressed in this article are solely those of the authors and do not necessarily represent those of their affiliated organizations, or those of the publisher, the editors and the reviewers. Any product that may be evaluated in this article, or claim that may be made by its manufacturer, is not guaranteed or endorsed by the publisher.
References
Amasyali, S. Y., and Yaliman, A. (2016). Comparison of the effects of mirror therapy and electromyography-triggered neuromuscular stimulation on hand functions in stroke patients: a pilot study. Int. J. Rehabil. Res. 39, 302–307. doi: 10.1097/MRR.0000000000000186
Basteris, A., Amirabdollahian, F., Nijenhuis, S., Buurke, J., and Prange, G. (2014). Training modalities in robot-mediated upper limb rehabilitation in stroke: a framework for classification based on a systematic review. J. Neuroeng. Rehabil. 11, 1–15. doi: 10.1186/1743-0003-11-111
Bolton, D. A. E., Cauraugh, J. H., and Hausenblas, H. A. (2004). Electromyogram-triggered neuromuscular stimulation and stroke motor recovery of arm/hand functions: a meta-analysis. J. Neurol. Sci. 223, 121–127. doi: 10.1016/j.jns.2004.05.005
Boyaci, A., Topuz, O., Alkan, H., Ozgen, M., Sarsan, A., Yildiz, N., et al. (2013). Comparison of the effectiveness of active and passive neuromuscular electrical stimulation of hemiplegic upper extremities: a randomized, controlled trial. Int. J. Rehabil. Res. 36, 315–322. doi: 10.1097/MRR.0b013e328360e541
Bustrén, E. L., Sunnerhagen, K. S., and Alt Murphy, M. (2017). Movement kinematics of the ipsilesional upper extremity in persons with moderate or mild stroke. Neurorehabil. Neural Repair 31, 376–386. doi: 10.1177/1545968316688798
Campanini, I., Disselhorst-Klug, C., Rymer, W. Z., and Merletti, R. (2020). Surface EMG in clinical assessment and neurorehabilitation: barriers limiting its use. Front. Neurol. 11:934. doi: 10.3389/fneur.2020.00934
Cappellini, G., Sylos-Labini, F., Assenza, C., Libernini, L., Morelli, D., Lacquaniti, F., et al. (2020). Clinical relevance of state-of-the-art analysis of surface electromyography in cerebral palsy. Front. Neurol. 11:583296. doi: 10.3389/fneur.2020.583296
Chaiyawat, P., Kulkantrakorn, K., and Sritipsukho, P. (2009). Effectiveness of home rehabilitation for ischemic stroke. Neurol. Int. 1:e10. doi: 10.4081/ni.2009.e10
Cordo, P., Wolf, S., Lou, J. S., Bogey, R., Stevenson, M., Hayes, J., et al. (2013). Treatment of severe hand impairment following stroke by combining assisted movement, muscle vibration, and biofeedback. J. Neurol. Phys. Ther. 37, 194–203. doi: 10.1097/NPT.0000000000000023
Criswell, E. (2010). Crams Introduction to Surface Electromyography, 2 Edn. Burlington, MA: Jones and Bartlett Publishers.
De Araújo, R. C., Junior, F. L., Rocha, D. N., Sono, T. S., and Pinotti, M. (2011). Effects of intensive arm training with an electromechanical orthosis in chronic stroke patients: a preliminary study. Arch. Phys. Med. Rehabil. 92, 1746–1753. doi: 10.1016/j.apmr.2011.05.021
de Kroon, J. R., and IJzerman, M. J. (2008). Electrical stimulation of the upper extremity in stroke: cyclic versus EMG-triggered stimulation. Clin. Rehabil. 22, 690–697. doi: 10.1177/0269215508088984
Deeks, J. J., Higgins, J. P. T., and Altman, D. G. (2021). “Analyzing data and undertaking meta-analyses,” in Cochrane Handbook for Systematic Reviews of Interventions, Vol. 1, eds J. P. T. Higgins and S. Green (Hoboken, NJ: John Wiley & Sons).
Doumas, I., Everard, G., Dehem, S., and Lejeune, T. (2021). Serious games for upper limb rehabilitation after stroke: a meta-analysis. J. Neuroeng. Rehabil. 18, 1–16. doi: 10.1186/s12984-021-00889-1
Eraifej, J., Clark, W., France, B., Desando, S., and Moore, D. (2017). Effectiveness of upper limb functional electrical stimulation after stroke for the improvement of activities of daily living and motor function: a systematic review and meta-analysis. Syst. Rev. 6, 1–21. doi: 10.1186/s13643-017-0435-5
Feigin, V. L., Krishnamurthi, R. V., Theadom, A. M., Abajobir, A. A., Mishra, S. R., Ahmed, M. B., et al. (2017). Global, regional, and national burden of neurological disorders during 1990–2015: a systematic analysis for the Global Burden of Disease Study 2015. Lancet Neurol. 16, 877–897. doi: 10.1016/S1474-4422(17)30299-5
Foley, E. L., Nicholas, M. L., Baum, C. M., and Connor, L. T. (2019). Influence of environmental factors on social participation post-stroke. Behav. Neurol. 2019:2606039. doi: 10.1155/2019/2606039
Fugl-Meyer, A. R., Jääskö, L., Leyman, I., and Olsson, S. (1975). The post-stroke hemiplegic patient. A method for evaluation of physical performance. Scand. J. Rehabil. Med. 7, 13–31.
Giggins, O. M., Persson, U. M. C., and Caulfield, B. (2013). Biofeedback in rehabilitation. J. Neuroeng. Rehabil. 10:1. doi: 10.1186/1743-0003-10-60
Gladstone, D. J., Danells, C. J., and Black, S. E. (2002). The fugl-meyer assessment of motor recovery after stroke: a critical review of its measurement properties. Neurorehabil. Neural Repair 16, 232–240. doi: 10.1177/154596802401105171
Hameed, H. K., Hassan, W. Z. W., Shafie, S., Ahmad, S. A., and Jaafar, H. A. (2020). Review on surface electromyography-controlled hand robotic devices used for rehabilitation and assistance in activities of daily living. J. Prosthetics Orthot. 32, 3–13. doi: 10.1097/JPO.0000000000000277
Harrer, M., Cuijpers, P., Furukawa, T. A., and Ebert, D. D. (2021). “Doing meta-analysis with R,” in A Hands-On Guide, 1st Edn, ed. M. Stephenson (Abingdon: Taylor & Francis Group). doi: 10.1201/9781003107347-2
Hatem, S. M., Saussez, G., della Faille, M., Prist, V., Zhang, X., Dispa, D., et al. (2016). Rehabilitation of motor function after stroke: a multiple systematic review focused on techniques to stimulate upper extremity recovery. Front. Hum. Neurosci. 10:442. doi: 10.3389/fnhum.2016.00442
Hemmen, B., and Seelen, H. (2007). Effects of movement imagery and electromyography-triggered feedback on arm-hand function in stroke patients in the subacute phase. Clin. Rehabil. 21, 587–594. doi: 10.1177/0269215507075502
Hernández, E. D., Galeano, C. P., Barbosa, N. E., Forero, S. M., Nordin, Å, Sunnerhagen, K. S. M. P. D., et al. (2019). Intra- And inter-rater reliability of Fugl-Meyer assessment of upper extremity in stroke. J. Rehabil. Med. 51, 652–659. doi: 10.2340/16501977-2590
Higgins, J. P. T., Li, T., and Deeks, J. J. (2019). “Choosing effect measures and computing estimates of effect,” in Cochrane Handbook for Systematic Reviews of Interventions, eds J. P. T. Higgins and S. Green (Hoboken, NJ: John Wiley & Sons), 143–176. doi: 10.1002/9781119536604.ch6
Hu, X. L., Tong, K. Y., Song, R., Zheng, X. J., and Leung, W. W. F. (2009). A comparison between electromyography-driven robot and passive motion device on wrist rehabilitation for chronic stroke. Neurorehabil. Neural Repair 23, 837–846. doi: 10.1177/1545968309338191
Hu, X. L., Tong, R. K. Y., Ho, N. S. K., Xue, J. J., Rong, W., and Li, L. S. W. (2015). Wrist rehabilitation assisted by an electromyography-driven neuromuscular electrical stimulation robot after stroke. Neurorehabil. Neural Repair 29, 767–776. doi: 10.1177/1545968314565510
Huang, Y., Nam, C., Li, W., Rong, W., Xie, Y., Liu, Y., et al. (2020). A comparison of the rehabilitation effectiveness of neuromuscular electrical stimulation robotic hand training and pure robotic hand training after stroke: a randomized controlled trial. Biomed. Signal. Process. Control 56:101723. doi: 10.1016/j.bspc.2019.101723
Hubbard, I. J., Parsons, M. W., Neilson, C., and Carey, L. M. (2009). Task-specific training: evidence for and translation to clinical practice. Occup. Ther. Int. 16, 175–189. doi: 10.1002/oti.275
Kim, S. H., Park, J. H., Jung, M. Y., and Yoo, E. Y. (2016). Effects of task-oriented training as an added treatment to electromyogram-triggered neuromuscular stimulation on upper extremity function in chronic stroke patients. Occup. Ther. Int. 23, 165–174. doi: 10.1002/oti.1421
Kojima, K., Ikuno, K., Morii, Y., Tokuhisa, K., Morimoto, S., and Shomoto, K. (2014). Feasibility study of a combined treatment of electromyography-triggered neuromuscular stimulation and mirror therapy in stroke patients: a randomized crossover trial. NeuroRehabilitation. 34, 235–244. doi: 10.3233/NRE-131038
Kwakkel, G., Winters, C., Van Wegen, E. E. H., Nijland, R. H. M., Van Kuijk, A. A. A., Visser-Meily, A., et al. (2016). Effects of unilateral upper limb training in two distinct prognostic groups early after stroke: the EXPLICIT-stroke randomized clinical trial. Neurorehabil. Neural Repair 30, 804–816. doi: 10.1177/1545968315624784
Maher, C. G., Sherrington, C., Herbert, R. D., Moseley, A. M., and Elkins, M. (2003). Reliability of the PEDro scale for rating quality of randomized controlled trials. Phys. Ther. 83, 713–721. doi: 10.1093/ptj/83.8.713
Mcmanus, L., De Vito, G., Lowery, M. M., and Mcmanus, L. (2020). Analysis and biophysics of surface emg for physiotherapists and kinesiologists : toward a common language with rehabilitation engineers. Front. Neurol. 11:576729. doi: 10.3389/fneur.2020.576729
Mehrholz, J., Pohl, M., Platz, T., Kugler, J., and Elsner, B. (2015). Electromechanical and robot-assisted arm training for improving activities of daily living, arm function, and arm muscle strength after stroke. Cochrane Database Syst. Rev. 2015:CD006876. doi: 10.1002/14651858
Meilink, A., Hemmen, B., Seelen, H. A. M., and Kwakkel, G. (2008). Impact of EMG-triggered neuromuscular stimulation of the wrist and finger extensors of the paretic hand after stroke: a systematic review of the literature. Clin. Rehabil. 22, 291–305. doi: 10.1177/0269215507083368
Merletti, R., and Farina, D. (2016). “Surface electromyography: physiology, engineering, and applications,” in Biomechanical Evaluation of Movement in Sport and Exercise, 1st Edn, eds C. J. Payton and A. Burden (Hoboken, NJ: John Wiley & Sons).
Monte-Silva, K., Piscitelli, D., Norouzi-Gheidari, N., Batalla, M. A. P., Archambault, P., and Levin, M. F. (2019). Electromyogram-related neuromuscular electrical stimulation for restoring wrist and hand movement in poststroke hemiplegia: a systematic review and meta-analysis. Neurorehabil. Neural Repair 33, 96–111. doi: 10.1177/1545968319826053
Moseley, A. M., Rahman, P., Wells, G. A., Zadro, J. R., Sherrington, C., Toupin-April, K., et al. (2019). Agreement between the cochrane risk of bias tool and physiotherapy evidence database (PEDro) scale: a meta-epidemiological study of randomized controlled trials of physical therapy interventions. PLoS One 14:e0222770. doi: 10.1371/journal.pone.0222770
Mugler, E. M., Tomic, G., Singh, A., Hameed, S., Lindberg, E. W., Gaide, J., et al. (2019). Myoelectric computer interface training for reducing co-activation and enhancing arm movement in chronic stroke survivors: a randomized trial. Neurorehabil. Neural Repair 33, 284–295. doi: 10.1177/1545968319834903
Nijland, R. H. M., Van Wegen, E. E. H., Harmeling-Van Der Wel, B. C., and Kwakkel, G. (2010). Presence of finger extension and shoulder abduction within 72 hours after stroke predicts functional recovery. Stroke 41, 745–750. doi: 10.1161/STROKEAHA.109.572065
Norouzi-Gheidari, N., Archambault, P. S., and Fung, J. (2012). Effects of robot-assisted therapy on stroke rehabilitation in upper limbs: systematic review and meta-analysis of the literature. J. Rehabil. Res. Dev. 49, 479–496. doi: 10.1682/jrrd.2010.10.0210
Obayashi, S., Takahashi, R., and Onuki, M. (2020). Upper limb recovery in early acute phase stroke survivors by coupled EMG-triggered and cyclic neuromuscular electrical stimulation. NeuroRehabilitation 46, 417–422. doi: 10.3233/NRE-203024
Page, M. J., McKenzie, J. E., Bossuyt, P. M., Boutron, I., Hoffmann, T. C., Mulrow, C. D., et al. (2021). The PRISMA 2020 statement: an updated guideline for reporting systematic reviews. BMJ 372:n71. doi: 10.1136/bmj.n71
Page, S. J., Griffin, C., and White, S. E. (2020). Efficacy of myoelectric bracing in moderately impaired stroke survivors: a randomized, controlled trial. J. Rehabil. Med. 52:jrm00017. doi: 10.2340/16501977-2644
Page, S. J., Hill, V., and White, S. (2013). Portable upper extremity robotics is as efficacious as upper extremity rehabilitative therapy: a randomized controlled pilot trial. Clin. Rehabil. 27, 494–503. doi: 10.1177/0269215512464795
Pang, M. Y., Harris, J. E., and Eng, J. J. (2006). A community-based upper-extremity group exercise program improves motor function and performance of functional activities in chronic stroke: a randomized controlled trial. Arch. Phys. Med. Rehabil. 87, 1–9. doi: 10.1016/j.apmr.2005.08.113
Park, J. H. (2020). Effects of mental imagery training combined electromyogram-triggered neuromuscular electrical stimulation on upper limb function and activities of daily living in patients with chronic stroke: a randomized controlled trial. Disabil. Rehabil. 42, 2876–2881. doi: 10.1080/09638288.2019.1577502
Persson, H. C., Alt Murphy, M., Danielsson, A., and Lundgren-Nilsson, Å, and Sunnerhagen, K. S. (2015). A cohort study investigating a simple, early assessment to predict upper extremity function after stroke - a part of the SALGOT study. BMC Neurol. 15:349. doi: 10.1186/s12883-015-0349-6
Persson, H. C., Parziali, M., Danielsson, A., and Sunnerhagen, K. S. (2012). Outcome and upper extremity function within 72 hours after first occasion of stroke in an unselected population at a stroke unit. A part of the SALGOT study. BMC Neurol. 12:1. doi: 10.1186/1471-2377-12-162
Pollock, A., Farmer, S. E., Brady, M. C., Langhorne, P., Mead, G. E., Mehrholz, J., et al. (2013). Interventions for improving upper limb function after stroke. Cochrane Database Syst. Rev. 2013:CD010820. doi: 10.1002/14651858.CD010820
Qian, Q., Hu, X., Lai, Q., Ng, S. C., Zheng, Y., and Poon, W. (2017). Early stroke rehabilitation of the upper limb assisted with an electromyography-driven neuromuscular electrical stimulation-robotic arm. Front. Neurol. 8:447. doi: 10.3389/fneur.2017.00447
Qian, Q., Nam, C., Guo, Z., Huang, Y., Hu, X., Ng, S. C., et al. (2019). Distal versus proximal - An investigation on different supportive strategies by robots for upper limb rehabilitation after stroke: a randomized controlled trial. J. Neuroeng. Rehabil. 16, 1–16. doi: 10.1186/s12984-019-0537-5
Raffin, E., and Hummel, F. C. (2018). Restoring motor functions after stroke: multiple approaches and opportunities. Neuroscientist 24, 400–416. doi: 10.1177/1073858417737486
Schick, T., Schlake, H. P., Kallusky, J., Hohlfeld, G., Steinmetz, M., Tripp, F., et al. (2017). Synergy effects of combined multichannel EMG-triggered electrical stimulation and mirror therapy in subacute stroke patients with severe or very severe arm/hand paresis. Restor. Neurol. Neurosci. 35, 319–332. doi: 10.3233/RNN-160710
Schleenbaker, R. E., and Mainous, A. G. (1993). Electromyographic biofeedback for neuromuscular reeducation in the hemiplegic stroke patient: a meta-analysis. Arch. Phys. Med. Rehabil. 74, 1301–1304. doi: 10.1016/0003-9993(93)90083-M
Shindo, K., Fujiwara, T., Hara, J., Oba, H., Hotta, F., Tsuji, T., et al. (2011). Effectiveness of hybrid assistive neuromuscular dynamic stimulation therapy in patients with subacute stroke: a randomized controlled pilot trial. Neurorehabil. Neural Repair 25, 830–837. doi: 10.1177/1545968311408917
Singer, B. J., Vallenceb, A. M., Cleary, S., Cooper, I., and Loftusb, A. M. (2013). The effect of EMG triggered electrical stimulation plus task practice on arm function in chronic stroke patients with moderate-severe arm deficits. Restor. Neurol. Neurosci. 31, 681–691. doi: 10.3233/RNN-130319
Takeda, K., Tanino, G., and Miyasaka, H. (2017). Review of devices used in neuromuscular electrical stimulation for stroke rehabilitation. Med. Devices Evid. Res. 10, 207–213. doi: 10.2147/MDER.S123464
Wilson, R. D., Page, S. J., Delahanty, M., Knutson, J. S., Gunzler, D. D., Sheffler, L. R., et al. (2016). Upper-limb recovery after stroke: a randomized controlled trial comparing emg-triggered, cyclic, and sensory electrical stimulation. Neurorehabil. Neural Repair 30, 978–987. doi: 10.1177/1545968316650278
Woodford, H., and Price, C. (2007). EMG biofeedback for the recovery of motor function after stroke. Cochrane Database Syst. Rev. 2007:CD004585. doi: 10.1002/14651858.CD004585.pub2
Yang, J. D., de Liao, C., Huang, S. W., Tam, K. W., Liou, T. H., Lee, Y. H., et al. (2019). Effectiveness of electrical stimulation therapy in improving arm function after stroke: a systematic review and a meta-analysis of randomised controlled trials. Clin. Rehabil. 33, 1286–1297. doi: 10.1177/0269215519839165
Keywords: electromyography, stroke, upper limb function, Fugl-Meyer Assessment, biofeedback, paresis
Citation: Munoz-Novoa M, Kristoffersen MB, Sunnerhagen KS, Naber A, Alt Murphy M and Ortiz-Catalan M (2022) Upper Limb Stroke Rehabilitation Using Surface Electromyography: A Systematic Review and Meta-Analysis. Front. Hum. Neurosci. 16:897870. doi: 10.3389/fnhum.2022.897870
Received: 16 March 2022; Accepted: 25 April 2022;
Published: 20 May 2022.
Edited by:
Jason Friedman, Tel Aviv University, IsraelReviewed by:
Laurence Kenney, University of Salford, United KingdomNoureddin Nakhostin Ansari, Tehran University of Medical Sciences, Iran
Copyright © 2022 Munoz-Novoa, Kristoffersen, Sunnerhagen, Naber, Alt Murphy and Ortiz-Catalan. This is an open-access article distributed under the terms of the Creative Commons Attribution License (CC BY). The use, distribution or reproduction in other forums is permitted, provided the original author(s) and the copyright owner(s) are credited and that the original publication in this journal is cited, in accordance with accepted academic practice. No use, distribution or reproduction is permitted which does not comply with these terms.
*Correspondence: Margit Alt Murphy, bWFyZ2l0LmFsdC1tdXJwaHlAbmV1cm8uZ3Uuc2U=; Max Ortiz-Catalan, bWF4b0BjaGFsbWVycy5zZQ==
†These authors have contributed equally to this work and share senior and last authorship