- 1School of Physical Education, Shenzhen University, Shenzhen, China
- 2Institute of Textiles and Clothing, The Hong Kong Polytechnic University, Hong Kong, China
- 3College of Physical Education, Southwest University, Chongqing, China
- 4Department of Sport Rehabilitation, Shanghai University of Sport, Shanghai, China
- 5Civil and Environmental Engineering Department, The Hong Kong Polytechnic University, Kowloon, Hong Kong SAR, China
It is widely known that exercise improves inhibitory control; however, the mechanisms behind the cognitive improvement remain unclear. This study analyzes the extant literature on the neuronal effects of exercise on inhibitory control functions. We searched four online databases (Pubmed, Scopus, PsycINFO, and Web of Science) for relevant peer-reviewed studies to identify eligible studies published before September 1, 2021. Among the 4,090 candidate studies identified, 14 meet the inclusion criteria, and the results of 397 participants in these 14 studies are subsequently analyzed. We quantify the neural effects on the entire brain by using GingerALE software and identify 10 clusters of exercise-induced neuronal with either increases/decreases in the superior temporal gyrus (BA 22), precuneus (BA 7), superior frontal gyrus (BA 10), cuneus (BA 19), precuneus (BA 19), caudate, posterior cingulate (BA 19), middle temporal gyrus (B 37), parahippocampal gyrus (BA 30), precentral gyrus (BA 6). Meta-analytic coactivation map (MACM) showed that multiple functional networks overlap with brain regions with activation likelihood estimation (ALE) results. We propose the effect of exercise on neural activity is related to inhibitory control in the extended frontoparietal, default mode network (DMN), visual network, and other pathways. These results provide preliminary evidence of the neural effects of exercise on inhibitory control.
Introduction
Inhibitory control or inhibition is defined as suppressing prepotent responses to goal-irrelevant stimuli and contributes to anticipation, planning, and goal setting. Inhibitory control is one of the three core executive functions of the brain (Aron, 2007; Liang et al., 2021b). People who suffer from impaired inhibitory control have a lower quality of life and develop health problems and diseases which applies to healthy and sick people (Liang et al., 2021a). Meanwhile, impaired inhibitory control could be a hallmark feature of several neuropsychological DSM-5 disorders including attention-deficit/hyperactivity disorder (ADHD; Crosbie et al., 2008; Bari and Robbins, 2013), bipolar disorder (Hidiroglu et al., 2015), schizophrenia (Enticott et al., 2008; Hughes et al., 2012), and substance use disorders (Liao et al., 2014; Lee et al., 2015). Although the disorders listed here are primarily characterized by difficulties in controlling behavior (Brady et al., 2011; Sofuoglu et al., 2013; Baumeister et al., 2018), they are also found in normal cognitive aging (Coxon et al., 2012; Smittenaar et al., 2015). Thus, to understand the risk of developing these disorders and contribute to current prevention and treatment measures, an analysis of the neural underpinnings of inhibitory control is essential and timely.
Physical activity has garnered significant attention as a potentially effective method for elevating cognitive function and improving brain health throughout life (Loprinzi et al., 2013; Ji et al., 2021). Meta-analyses of healthy participants (Li et al., 2020; Amatriain-Fernandez et al., 2021; Chen et al., 2021), autism spectrum disorder (ASD; Liang et al., 2021b), mild cognitive impairment (MCI; Biazus-Sehn et al., 2020) as well as ADHD patients (Liang et al., 2021a) have shown that exercise has a positive effect on inhibitory control in individuals. Neuroimaging studies have also demonstrated the inhibitory control mechanisms of the brain by exploring the relationship between exercise and brain region activation during specific tasks (e.g., flanker, go/no-go, and Stroop tasks). Previous studies reported that the “cognitive control network” actively coordinates multiple brain regions, such as the frontal cortex (including the anterior cingulate cortex), parietal cortex, motor regions, and cerebellum (Niendam et al., 2012; Akatsuka et al., 2015; Chu et al., 2015). The effects of exercise on functional changes in inhibitory control are not apparent. Indeed, some studies report an increase in the activation of the prefrontal and parietal lobes following exercise intervention (Mehren et al., 2019c), whereas others reported less activation in the frontal and temporal lobes during similar inhibitory-based tasks (Krafft et al., 2014; Hsu et al., 2018).
The last few years have seen the introduction of several tools for performing a meta-analysis of data obtained from brain imaging research, allowing for the quantitative integration of findings from different studies. Activation likelihood estimation (ALE) is a relatively way to estimate the probability that at least one activation focus from a set of experiments lies at the location of a specific voxel, using Gaussian assumptions of spatial uncertainty (Turkeltaub et al., 2002) This study, using the ALE method, aimed to explore the overall neural changes in inhibitory control associated with exercise. We hypothesized the critical regions of exercise-induced inhibitory control are related to several frontal and parietal cortex brain areas. To validate ALE results whether overlap the brain networks to related frontoparietal or other brain networks, we applied a meta-analytic co-activation model (MACM) approach using activation clusters as regions of interest (ROIs) from our ALE results.
Method
The meta-analysis in this study is completed and reported by the Preferred Reporting Items for Systematic Reviews and Meta-Analyses Statement (PRISMA). The protocol was registered as trial registration number CRD42021285736 under the International Prospective Register of Systematic Reviews (PROSPERO).
Search Strategy
Four electronic databases (Pubmed, Scopus, PsycINFO, and Web of Science) were searched from their inception to September 1, 2021, to identify all published studies on functional magnetic resonance imaging (fMRI) that investigate the impact of exercise on the activation of different brain areas during inhibitory control tasks. The initial search used three key terms: physical activity or exercise, inhibitory control, and fMRI. We also hand-searched recent systematic reviews and meta-analyses to identify potential studies (Liang et al., 2021a; Yu et al., 2021). The search was limited to English-language results and human subjects. The reference lists of included studies were manually reviewed for relevant articles that were captured through the database searches. The detailed keyword search strategy is presented in the Appendix Table.
Study Selection
The screening for relevant studies was conducted in accordance with the PICOS (participants, intervention, comparisons, outcomes, and study design) principles. The participants included individuals of all ages and pathologies. The studies must have investigated the effect of exercise on inhibitory control and examined pre/post-intervention with at least one group assigned to physical activity/exercise intervention. During fMRI scanning, studies must have assessed brain activation patterns via completed inhibitory control tasks (e.g., go/no-go, stroop, flanker, or Simon tasks). For inclusion, retrievable data in standard Talairach or Montreal Neurologic Institute (MNI) space was also required. Finally, the chosen studies included randomized controlled trials (RCTs) and non-randomized controlled trial studies (NRCTs) published in peer-reviewed journals.
Two reviewers independently conducted the multi-step search process based on these selection criteria and screened the studies based on their title and abstract. Full-length texts were eventually used to identify eligible articles. If consensus could not be reached, a third reviewer made the final decision after discussion with the two reviewers.
Data Extraction
A standardized data extraction form was developed to extract relevant data from each study, including the bibliographic details (author and year), participant characteristics (sample size, sex, and age range), intervention components (intervention design and duration), fMRI task, software used, and active results/foci.
Quality Assessment
The Physiotherapy Evidence Database (PEDro) scale, a reliable and valid instrument for assessing the methodological quality of studies that focus on the effects of physical activity on cognitive functions, was used to determine the methodological quality (Sherrington et al., 2000). This scale includes 11 rating criteria including eligibility, randomization, allocation, blinding (subjects and experimenter), intention-to-treat, between-group comparison, and point measures. The methodological criteria were scored as Yes (one point), No (zero points) or Do not know (zero points). The PEDro score of each selected study served as an indicator of the methodological quality (<4 = poor; 4–5 = fair; 6–8 = good; and 9–10 = excellent).
The fMRI quality was determined from a set of guidelines for the standardized reporting of the fMRI studies and used to assess the fMRI study design/reporting quality and quality of the fMRI data of each included study (Poldrack et al., 2008). The fMRI form has 8-item rating criteria for eligibility, the experimental design, handedness and gender of participants, explanation for rejected data, details of the imaging parameters, software analysis method and package used, motion correction method during pre-processing, multiple comparison correction, and detailed description of the first and second level analyses.
Data Analysis
The ALE is a reliable quantitative method for coordinate-based meta-analyses to identify brain activation during cognitive functions after exercise (Laird et al., 2005). In this study, GingerALE v2.3.6 (http://www.brainmap.org) software is used to analyze the data. A statistical threshold of uncorrected p < 0.001 and a minimum cluster size of 100 mm3 were used (Meng et al., 2020). The ALE maps were imported into Mango Version 4.1 (http://ric.uthscsa.edu/mango/mango.html) software and overlaid on an anatomical template in MNI space for visualization. The effect of exercise on behavioral performance is not examined due to missing data and changes in an inhibitory control task that resulted in the effect magnitude being inestimable.
Additionally, to obtain the MACM based on the ALE results, we followed the procedure proposed by Robinson et al. (2010) as implemented in NeuroSynth (http://neurosynth.org/; Yarkoni et al., 2011). The activation clusters as seed ROIs were separately entered into NeuroSynth to evaluate the MACM. In brief, the software searches among more than 11,000 fMRI studies (totaling 413,429 activations in the MNI152 coordinate space) those reporting activation in a spherical seed (6 mm) around the searched coordinates. The identified co-activations are then pooled together to form the MACM output that is corrected for multiple comparisons (p < 0.01 false discovery rate; FDR as provided in NeuroSynth). Namely, a z-score is assigned to each voxel, representing the strength of the association between a given voxel and the seed coordinates.
Results
Study Selection
Four thousand and eighty-eight studies were identified from the four databases, and two studies were identified from other systemic reviews. Included studies were chosen after thoroughly screening the titles, abstracts, and full text. Fourteen studies were identified for the meta-analysis (Figure 1).
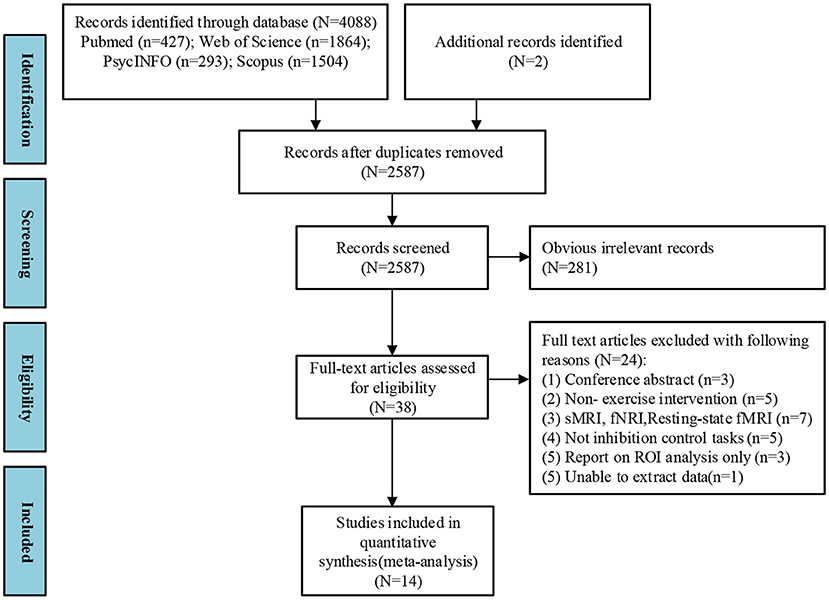
Figure 1. PRISMA flow diagram of selected studies. Multi-step search process shows the number of studies screened and those that meet the inclusion criteria.
Study Characteristics
Table 1 lists the characteristics of the 14 studies that involved 397 participants. Six studies (Mehren et al., 2019a,b,c; Won et al., 2019; Cui et al., 2020; Meng et al., 2020) utilized acute exercise paradigms and eight studies (Colcombe et al., 2004; Liu-Ambrose et al., 2012; Krafft et al., 2014; Sachs et al., 2017; Hsu et al., 2018; Martinsen et al., 2018; Pensel et al., 2018; Wu et al., 2018) utilized chronic exercise routines. Three neurocognitive tasks (including flanker, stroop, and go/no-go tasks) were used to assess inhibitory control in participants across the studies.
Quality Assessment
Table 2 shows the methodological quality of the studies using the PEDro scale. The total scores range from 6 to 9 (M = 6.71). Notably, none of the studies reported blinded data due to the difficulties of using blinded subjects, therapists, and assessors in an exercise intervention. The studies that failed to obtain points in other criteria due to their study design include lack of eligibility (n = 1), random allocation (n = 9), and concealed allocation (n = 10).
Table 3 shows the fMRI quality assessment for all of the studies from a set of guidelines for the standardized reporting of fMRI studies. Other criteria were lacking in at least one reporting guideline, particularly in the scan rejection mentioned (n = 13), scan rejection reason (n = 14), the method for motion correction (n = 7), volume acquired per session (n = 14), and clear descriptions of the first (n = 8) and second level contrasts (n = 7).
The Overall Analysis of Activity Results
Among the 14 studies that assessed brain activation during inhibitory control tasks after exercise, the exercise groups (EGs) show increased brain activation compared to the control groups (CGs) in six clusters: (1) superior temporal gyrus (BA 22), (2) precuneus (BA 7 and BA 19), (3) superior frontal gyrus (BA 10), and (4) cuneus (BA 19). Five regions showed reduced brain activation: (1) caudate gray matter, (2) posterior cingulate cortex (BA 31), (3) middle temporal gyrus (BA 37), (4) parahippocampal gyrus, and (5) precentral gyrus (BA 6), as shown in Table 4 and Figure 2.
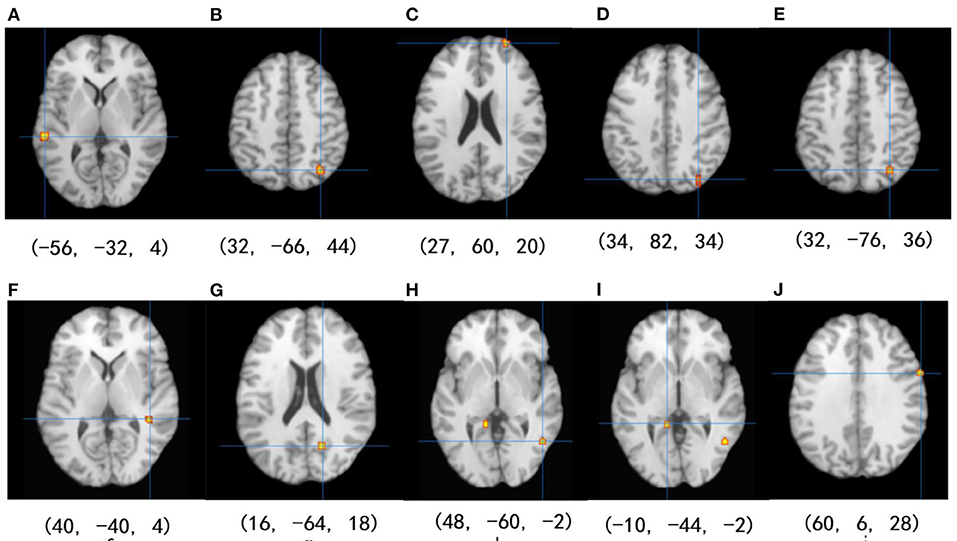
Figure 2. Results of the ALE meta-analysis show increased and reduced activation for inhibitory control in the EGs compared with CGs. Uncorrected p < 0.001 and cluster size >100 mm3 (from A–D: activation is increased, and from E–J: activation is decreased).
Coactivation Maps
Coactivation maps were plotted after the MACM analysis, as shown the Table 5. To understand the correspondence between the obtained coactivation maps and the brain functional connectivity networks, we compared it with the cortical parcellation atlas built by Yeo et al. (2011) on 1,000 healthy young subjects. We found that multiple functional networks, including the frontoparietal network, visual network, default mode network, and attention network, overlap with brain regions with ALE results, although this is a subjective judgment.
Discussion
The primary aim of the ALE meta-analysis in this study is to offer the first quantitative summary of the effect of exercise on increased and decreased neural activity of different brain areas during inhibitory control tasks. We identified five clusters of increased neural activity [left superior temporal gyrus, right precuneus (BA 7 and BA 19), right superior frontal gyrus, and right cuneus] and five clusters of diminished neural activity (left parahippocampal, right posterior cingulate, right middle temporal and right precentral gyrus, and right caudate) following exercise. MACM analysis suggested that inhibitory control involved multiple functional networks. These findings may help identify the underlying mechanisms of increased inhibitory control that are exercise-induced.
Previous work found that the neurophysiological mechanism that controls movement is located in the prefrontal cortex (Niendam et al., 2012; Ardila et al., 2018). Our finding shows that there are changes in brain activity in the superior frontal and precentral gyrus of the frontal lobe. It should be noted that both of these regions are responsible for motor control (Niendam et al., 2012; Ardila et al., 2018).
The superior frontal gyrus is also part of the prefrontal cortex, which is responsible for cognitive control functions. This is closely connected with high-level cognitive functions such as interference inhibition, conflict solving, and selective attention (Cabeza and Nyberg, 2000; Baym et al., 2008). The precentral gyrus is part of the supplementary motor area (SMA), which is essential for cognitive control as found in previous studies of concern, especially when motor movements need to be inhibited (Nachev et al., 2008; Aron, 2010).
Our findings showed one change in brain activity in the parietal lobe is the precuneus. The precuneus region is an essential area for attention selection and response to conflict (Indovina and Macaluso, 2004). Previous research found that it plays a pivotal role in the prefrontal-parietal circuit when performing inhibitory tasks (Garavan et al., 2002; Mehren et al., 2019c). Thus, this study concludes that exercise is closely related to connectivity in the frontoparietal network. However, more studies are needed to verify this conclusion.
Besides, the finding showed that three regions' activity changed, including the superior temporal gyrus, middle temporal gyrus, and caudate in the temporal lobe during inhibitory control tasks. It is worthy to note that the superior temporal sulcus typically provides the amygdala with visual information that contributes to identifying the affective or motivational significance of visually perceived objects (Arzimanoglou et al., 2005). An aerobic intervention experiment by Hsu et al. (2018) showed that aerobic intervention alters the activation of the superior temporal gyrus in patients with cognitive impairment and pointed out that there is an apparent correlation between the activation of the superior temporal gyrus and the reaction speed in the go/no-go task. Liu-Ambrose et al. found that after 52 elderly subjects received resistance training twice a week for 12 months, the pathway extending from the left anterior middle temporal gyrus and the left anterior insula to the lateral orbitofrontal cortex was activated during the flanker task (Liu-Ambrose et al., 2012).
It has been proven that the caudate is one of the primary input nuclei receiving inputs from the prefrontal cortex and transferring information to the basal ganglia (Kunishio and Haber, 1994; Haber et al., 2000; Nakahara et al., 2002). The dorsolateral prefrontal cortex-caudate circuit was also shown to be involved in proactive inhibition via the indirect pathway (Jahfari et al., 2011). We speculate that exercise effectively activates these pathways in response to inhibitory tasks. However, additional experiments are required for verification.
Finally, we found posterior cingulate gyrus and parahippocampal gyrus have activation changes, and these two regions both belong to the limbic structures. The posterior cingulate gyrus is close to the limbic system and belongs to the cortical region functioning as a gateway between the limbic system and the midbrain and diencephalon (Li et al., 2017). Since the posterior cingulate gyrus and precuneus are identified here, we hypothesize that exhibited hyper-connectivity (i.e., stronger positive connectivity) is shown in the default mode network (DMN) regions during inhibitory control tasks which is consistent with findings in previous studies (Yu et al., 2021). Furthermore, the degree of coupling between the DMN and the frontal-parietal network positively correlates with overall cognitive function. Therefore, the DMN, which is the main component for the stop signal in inhibitory tasks is particularly significant for the impact of physical exercise on inhibitory control ability.
The parahippocampal gyrus is an active region in the limbic system, and exercise intervention appears to strengthen their neuronal excitability, increase the volume of white matter/gray matter, and positively change the production of brain-derived neural factors (Li et al., 2017; Loprinzi, 2017; Muller et al., 2017; Ji et al., 2021). Previous research has validated the effect of exercise on the plasticity of the parahippocampal gyrus, which plays a crucial role in maintaining memory function and aids in spatial information processing and object recognition (Brown and Aggleton, 2001; Raslau et al., 2015). We guess that the parahippocampal gyrus of spatial information processing and object recognition functions are also likely the underlying reason(s) that exercise improves cognitive control function.
There are some limitations to this study. First, only 14 studies are included, which is a smaller sample size. As a result, a subgroup analysis of the exercise intensity and type of exercise is challenging. Second, this study contains different inhibitory control tasks; although the flanker, go-no-go, and stroop tasks are all standard tasks for measuring inhibitory control, the components are slightly different.
Conclusions
Neural mechanisms increase exercise-induced inhibitory control by changing the activation of single and multiple brain regions. We guess that critical areas mediate inhibitory control and are associated with the frontoparietal, visual network, DMN, and other pathways. However, due to the lenient threshold used in the ALE analysis, further research with more rigorous methods is required to link exercise to neuroplasticity changes with respect to inhibitory control.
Data Availability Statement
The raw data supporting the conclusions of this article will be made available by the authors, without undue reservation.
Author Contributions
JW and ZR were responsible for the conceptualization, investigation, and hypothesis of the research. JW and WX conducted systematic search, data extraction, quality assessment, and data analyses. JY, KZ, LP, OT, and ZR reviewed and edited the initial draft and revisions. All authors contributed to the article and approved the submitted version.
Funding
The article was supported by Research Foundation for Young Teacher of Shenzhen University [grant number QNJS0274]; High-level Scientific Research Foundation for the Introduction of Talent of Shenzhen University [grant number RC00228]; and Natural Science Featured Innovation Projects in Ordinary Universities in Guangdong Province [grant number 2021KTSCX297].
Conflict of Interest
The authors declare that the research was conducted in the absence of any commercial or financial relationships that could be construed as a potential conflict of interest.
Publisher's Note
All claims expressed in this article are solely those of the authors and do not necessarily represent those of their affiliated organizations, or those of the publisher, the editors and the reviewers. Any product that may be evaluated in this article, or claim that may be made by its manufacturer, is not guaranteed or endorsed by the publisher.
Supplementary Material
The Supplementary Material for this article can be found online at: https://www.frontiersin.org/articles/10.3389/fnhum.2022.891095/full#supplementary-material
References
Akatsuka, K., Yamashiro, K., Nakazawa, S., Mitsuzono, R., and Maruyama, A. (2015). Acute aerobic exercise influences the inhibitory process in the go/no-go task in humans. Neurosci. Lett. 600, 80–84. doi: 10.1016/j.neulet.2015.06.004
Amatriain-Fernandez, S., Garcia-Noblejas, M. E., and Budde, H. (2021). Effects of chronic exercise on the inhibitory control of children and adolescents: a systematic review and meta-analysis. Scand. J. Med. Sci. Sports 31, 1196–1208. doi: 10.1111/sms.13934
Ardila, A., Bernal, B., and Rosselli, M. (2018). Executive functions brain system: an activation likelihood estimation meta-analytic study. Arch. Clin. Neuropsychol. 33, 379–405. doi: 10.1093/arclin/acx066
Aron, A. R.. (2007). The neural basis of inhibition in cognitive control. Neuroscientist 13, 214–228. doi: 10.1177/1073858407299288
Aron, A. R.. (2010). From reactive to proactive and selective control: developing a richer model for stopping inappropriate responses. Biol. Psychiatry 69, e55–e68. doi: 10.1016/j.biopsych.2010.07.024
Arzimanoglou, A., Aldenkamp, A., Cross, H., and Lassonde, M. (2005). Cognitive Dysfunction in Children with Temporal Lobe Epilepsy (Vol. 1). John Libbey Eurotext.
Bari, A., and Robbins, T. W. W. (2013). Noradrenergic versus dopaminergic modulation of impulsivity, attention and monitoring behaviour in rats performing the stop-signal task. Psychopharmacology 230, 89–111. doi: 10.1007/s00213-013-3141-6
Baumeister, S., Wolf, I., Holz, N., Boecker-Schlier, R., Adamo, N., Holtmann, M., et al. (2018). Neurofeedback training effects on inhibitory brain activation in ADHD: a matter of learning? Neuroscience 378, 89–99. doi: 10.1016/j.neuroscience.2016.09.025
Baym, C. L., Corbett, B. A., Wright, S. B., and Bunge, S. A. (2008). Neural correlates of tic severity and cognitive control in children with Tourette syndrome. Brain 131, 165–179. doi: 10.1093/brain/awm278
Biazus-Sehn, L. F., Schuch, F. B., Firth, J., and Stugger, F. S. (2020). Effects of physical exercise on cognitive function of older adults with mild cognitive impairment: a systematic review and meta -analysis. Arch. Gerontol. Geriatr. 89:104048. doi: 10.1016/j.archger.2020.104048
Brady, K. T., Gray, K. M., and Tolliver, B. K. (2011). Cognitive enhancers in the treatment of substance use disorders: clinical evidence. Pharmacol. Biochem. Behav. 99, 285–294. doi: 10.1016/j.pbb.2011.04.017
Brown, M. W., and Aggleton, J. P. (2001). Recognition memory: what are the roles of the perirhinal cortex and hippocampus? Nat. Rev. Neurosci. 2, 51–61. doi: 10.1038/35049064
Cabeza, R., and Nyberg, L. (2000). Imaging cognition II: an empirical review of 275 PET and fMRI studies. J. Cogn. Neurosci. 12, 1–47. doi: 10.1162/08989290051137585
Chen, F., Hung, T. M., and Chang, Y. (2021). Reply to: comment on: “effects of exercise training interventions on executive function in older adults: a systematic review and meta-analysis.” Sports Med. 51, 597–598. doi: 10.1007/s40279-020-01370-0
Chu, C., Alderman, B. L., Wei, G., and Wei, G. (2015). Effects of acute aerobic exercise on motor response inhibition: an ERP study using the stop-signal task. J. Sport Health Sci. 4, 73–81. doi: 10.1016/j.jshs.2014.12.002
Colcombe, S. J., Kramer, A. F., Erickson, K. I., Scalf, P., McAuley, E., Cohen, N. J., et al. (2004). Cardiovascular fitness, cortical plasticity, and aging. Proc. Natl. Acad. Sci. U.S.A. 101, 3316–3321. doi: 10.1073/pnas.0400266101
Coxon, J. P., Van Impe, A., Wenderoth, N., and Swinnen, S. P. (2012). Aging and inhibitory control of action: cortico-subthalamic connection strength predicts stopping performance. J. Neurosci. 32, 8401–8412. doi: 10.1523/JNEUROSCI.6360-11.2012
Crosbie, J., Perusse, D., Barr, C. L., and Schachar, R. J. (2008). Validating psychiatric endophenotypes: inhibitory control and attention deficit hyperactivity disorder. Neurosci. Biobehav. Rev. 32, 40–55. doi: 10.1016/j.neubiorev.2007.05.002
Cui, J., Zou, L., Herold, F., Yu, Q., Jiao, C., Zhang, Y., et al. (2020). Does cardiorespiratory fitness influence the effect of acute aerobic exercise on executive function? Front. Hum. Neurosci. 14:569010. doi: 10.3389/fnhum.2020.569010
Enticott, P. G., Ogloff, J. R., and Bradshaw, J. L. (2008). Response inhibition and impulsivity in schizophrenia. Psychiatry Res. 157, 251–254. doi: 10.1016/j.psychres.2007.04.007
Garavan, H., Ross, T. J., Murphy, K., Roche, R. A., and Stein, E. A. (2002). Dissociable executive functions in the dynamic control of behavior: inhibition, error detection, and correction. Neuroimage 17, 1820–1829. doi: 10.1006/nimg.2002.1326
Haber, S. N., Fudge, J. L., and McFarland, N. R. (2000). Striatonigrostriatal pathways in primates form an ascending spiral from the shell to the dorsolateral striatum. J. Neurosci. 20, 2369–2382. doi: 10.1523/JNEUROSCI.20-06-02369.2000
Hidiroglu, C., Torres, I. J., Er, A., Isik, G., Yaln, N., Yatham, L. N., et al. (2015). Response inhibition and interference control in patients with bipolar I disorder and first-degree relatives. Bipolar Disord. 17, 781–794. doi: 10.1111/bdi.12335
Hsu, C. L., Best, J. R., Davis, J. C., Nagamatsu, L. S., Wang, S., Boyd, L. A., et al. (2018). Aerobic exercise promotes executive functions and impacts functional neural activity among older adults with vascular cognitive impairment. Br. J. Sports Med. 52:184. doi: 10.1136/bjsports-2016-096846
Hughes, M. E., Fulham, W. R., Johnston, P. J., and Michie, P. T. (2012). Stop-signal response inhibition in schizophrenia: behavioural, event-related potential and functional neuroimaging data. Biol. Psychol. 89, 220–231. doi: 10.1016/j.biopsycho.2011.10.013
Indovina, I., and Macaluso, E. (2004). Occipital-parietal interactions during shifts of exogenous visuospatial attention: trial-dependent changes of effective connectivity. Magn. Reson. Imaging 22, 1477–1486. doi: 10.1016/j.mri.2004.10.016
Jahfari, S., Waldorp, L., van den Wildenberg, W. P., Scholte, H. S., Ridderinkhof, K. R., and Forstmann, B. U. (2011). Effective connectivity reveals important roles for both the hyperdirect (fronto-subthalamic) and the indirect (fronto-striatal-pallidal) fronto-basal ganglia pathways during response inhibition. J. Neurosci. 31, 6891–6899. doi: 10.1523/JNEUROSCI.5253-10.2011
Ji, L., Steffens, D. C., and Wang, L. (2021). Effects of physical exercise on the aging brain across imaging modalities: a meta-analysis of neuroimaging studies in randomized controlled trials. Int. J. Geriatr. Psychiatry 36, 1148–1157. doi: 10.1002/gps.5510
Krafft, C. E., Schwarz, N. F., Chi, L., Weinberger, A. L., Schaeffer, D. J., Pierce, J. E., et al. (2014). An 8-month randomized controlled exercise trial alters brain activation during cognitive tasks in overweight children. Obesity 22, 232–242. doi: 10.1002/oby.20518
Kunishio, K., and Haber, S. N. (1994). Primate cingulostriatal projection: limbic striatal versus sensorimotor striatal input. J. Comp. Neurol. 350, 337–356.doi: 10.1002/cne.903500302
Laird, A. R., Fox, P. M., Price, C. J., Glahn, D. C., Uecker, A. M., Lancaster, J. L., et al. (2005). ALE meta-analysis: controlling the false discovery rate and performing statistical contrasts. Hum. Brain Mapp. 25, 155–164. doi: 10.1002/hbm.20136
Lee, R. S., Hoppenbrouwers, S., and Franken, I. (2015). A systematic meta-review of impulsivity and compulsivity in addictive behaviors. Neuropsychol. Rev. 29, 14–26. doi: 10.1007/s11065-019-09402-x
Li, L., Zhang, J., Cao, M., Hu, W., Zhou, T., Huang, T., et al. (2020). The effects of chronic physical activity interventions on executive functions in children aged 3-7 years: a meta-analysis. J. Sci. Med. Sport 23, 949–954. doi: 10.1016/j.jsams.2020.03.007
Li, M. Y., Huang, M. M., Li, S. Z., Tao, J., Zheng, G., and Chen, L. (2017). The effects of aerobic exercise on the structure and function of DMN-related brain regions: a systematic review. Int. J. Neurosci. 127, 634–649. doi: 10.1080/00207454.2016.1212855
Liang, X., Li, R., Wong, S. H., Sum, R. K., and Sit, C. H. P. (2021a). The impact of exercise interventions concerning executive functions of children and adolescents with attention-deficit/hyperactive disorder: a systematic review and meta-analysis. Int. J. Behav. Nutr. Phys. Act 18:68. doi: 10.1186/s12966-021-01135-6
Liang, X., Li, R., Wong, S. H., Sum, R. K., Wang, P., Yang, B., et al. (2021b). The effects of exercise interventions on executive functions in children and adolescents with autism spectrum disorder: a systematic review and meta-analysis. Sports Med. 52, 75–88. doi: 10.1007/s40279-021-01545-3
Liao, D., Huang, C., Hu, S., Fang, S., Wu, C., Chen, W., et al. (2014). Cognitive control in opioid dependence and methadone maintenance treatment. PLoS ONE 9:e94589. doi: 10.1371/journal.pone.0094589
Liu-Ambrose, T., Nagamatsu, L. S., Voss, M. W., Khan, K. M., and Handy, T. C. (2012). Resistance training and functional plasticity of the aging brain: a 12-month randomized controlled trial. Neurobiol. Aging 33, 1690–1698. doi: 10.1016/j.neurobiolaging.2011.05.010
Loprinzi, P. D.. (2017). The effects of physical exercise on parahippocampal function. Physiol. Int. 106, 114–127. doi: 10.1556/2060.106.2019.10
Loprinzi, P. D., Herod, S. M., Cardinal, B. J., and Noakes, T. D. (2013). Physical activity and the brain: a review of this dynamic, bi-directional relationship. Brain Res. 1539, 95–104. doi: 10.1016/j.brainres.2013.10.004
Martinsen, S., Flodin, P., Berrebi, J., Löfgren, M., Bileviciute-Ljungar, I., Mannerkorpi, K., et al. (2018). The role of long-term physical exercise on performance and brain activation during the Stroop colour word task in fibromyalgia patients. Clin. Physiol. Funct. Imaging 38, 508–516. doi: 10.1111/cpf.12449
Mehren, A., Luque, C. D., Brandes, M., Lam, A. P., Thiel, C. M., Philipsen, A., et al. (2019a). Intensity-dependent effects of acute exercise on executive function. Neural Plast 2019:8608317. doi: 10.1155/2019/8608317
Mehren, A., Oezyurt, J., Lam, A. P., Brandes, M., Müller, H. H., Thiel, C. M., et al. (2019b). Acute effects of aerobic exercise on executive function and attention in adult patients with ADHD. Front. Psychiatry 10:132. doi: 10.3389/fpsyt.2019.00132
Mehren, A., Ozyurt, J., Thiel, C. M., Brandes, M., Lam, A. P., and Philipsen, A. (2019c). Effects of acute aerobic exercise on response inhibition in adult patients with ADHD. Sci. Rep. 9:19884. doi: 10.1038/s41598-019-56332-y
Meng, X., Huang, D., Ao, H., Wang, X., and Gao, X. (2020). Food cue recruits increased reward processing and decreased inhibitory control processing in the obese/overweight: an activation C likelihood estimation meta-analysis of fMRI studies. Obes. Res. Clin. Pract. 14, 127–135. doi: 10.1016/j.orcp.2020.02.004
Metcalfe, A. W., MacIntosh, B. J., Scavone, A., Ou, X., Korczak, D., and Goldstein, B. I. (2016). Effects of acute aerobic exercise on neural correlates of attention and inhibition in adolescents with bipolar disorder. Transl. Psychiatry 6:e814. doi: 10.1038/tp.2016.85
Muller, P., Rehfeld, K., Schmicker, M., Hökelmann, A., Dordevic, M., Lessmann, V., et al. (2017). Evolution of neuroplasticity in response to physical activity in old age: the case for dancing. Front. Aging Neurosci. 9:56. doi: 10.3389/fnagi.2017.00056
Nachev, P., Kennard, C., and Husain, M. (2008). Functional role of the supplementary and pre-supplementary motor areas. Nat. Rev. Neurosci. 9, 856–869. doi: 10.1038/nrn2478
Nakahara, K., Hayashi, T., Konishi, S., and Miyashita, Y. (2002). Functional MRI of macaque monkeys performing a cognitive set-shifting task. Science. 295, 1532–1536. doi: 10.1126/science.1067653
Niendam, T. A., Laird, A. R., Ray, K. L., Dean, Y. M., Glahn, D. C., and Carter, C. S. (2012). Meta-analytic evidence for a superordinate cognitive control network subserving diverse executive functions. Cogn. Affect. Behav. Neurosci. 12, 241–268. doi: 10.3758/s13415-011-0083-5
Pensel, M. C., Daamen, M., Scheef, L., Knigge, H. U., Vega, S. R., Martin, J. A., et al. (2018). Executive control processes are associated with individual fitness outcomes following regular exercise training: blood lactate profile curves and neuroimaging findings. Sci. Rep. 8:4893. doi: 10.1038/s41598-018-23308-3
Poldrack, R. A., Fletcher, P. C., Henson, R. N, Worsley, K. J., Brett, M., and Nichols, T. E. (2008). Guidelines for reporting an fMRI study. Neuroimage 40, 409–414. doi: 10.1016/j.neuroimage.2007.11.048
Raslau, F. D., Mark, I. T., Klein, A. P., Ulmer, J., Mathews, V., and Mark, L. P. (2015). Memory part 2: the role of the medial temporal lobe. Am. J. Neuroradiol. 36, 846–849. doi: 10.3174/ajnr.A4169
Robinson, J. L., Laird, A. R., Glahn, D. C., Lovallo, W. R., and Fox, P. T. (2010). Metaanalytic connectivity modeling: delineating the functional connectivity of the human amygdala. Hum. Brain Mapp. 31, 173–184. doi: 10.1002/hbm.20854
Sachs, M., Kaplan, J., Sarkissian, A. D., and Habibi, A. (2017). Increased engagement of the cognitive control network associated with music training in children during an fMRI Stroop task. PLoS ONE 12:e0187254. doi: 10.1371/journal.pone.0187254
Sherrington, C., Herbert, R. D., Maher, C. G., and Moseley, A. M. (2000). PEDro. A database of randomized trials and. systematic reviews in physiotherapy. Man Ther. 5, 223–226. doi: 10.1054/math.2000.0372
Smittenaar, P., Rutledge, R. B., Zeidman, P., Adams, R. A., Brown, H., Lewis, G., et al. (2015). Proactive and reactive response inhibition across the lifespan. PLoS ONE 10:e0140383. doi: 10.1371/journal.pone.0140383
Sofuoglu, M., DeVito, E. E., Waters, A. J., and Carroll, K. M. (2013). Cognitive enhancement as a treatment for drug addictions. Neuropharmacology 64, 452–463. doi: 10.1016/j.neuropharm.2012.06.021
Turkeltaub, P. E., Eden, G. F., Jones, K. M., and Zeffiro, T. A. (2002). Meta-analysis of the functional neuroanatomy of single-word reading: method and validation. Neuroimage 16, 765–780. doi: 10.1006/nimg.2002.1131
Won, J., Alfini, A. J., Weiss, L. R., Callow, D. D., and Smith, J. C. (2019). Brain activation during executive control after acute exercise in older adults. Int. J. Psychophysiol. 146, 240–248. doi: 10.1016/j.ijpsycho.2019.10.002
Wu, M., Tang, P., Goh, J. O., Chou, T., Chang, Y., Hsu, Y., et al. (2018). Task-switching performance improvements after tai chi chuan training are associated with greater prefrontal activation in older adults. Front. Aging Neurosci. 10:280. doi: 10.3389/fnagi.2018.00280
Yarkoni, T., Poldrack, R. A., Nichols, T. E., Van Essen, D. C., and Wager, T. D. (2011). Large-scale automated synthesis of human functional neuroimaging data. Nat. Methods 8, 665–670. doi: 10.1038/nmeth.1635
Yeo, B. T., Krienen, F. M., Sepulcre, J., Sabuncu, M. R., Lashkari, D., Hollinshead, M., et al. (2011). The organization of the human cerebral cortex estimated by intrinsic functional connectivity. J. Neurophysiol. 106, 1125–1165. doi: 10.1152/jn.00338.2011
Keywords: exercise, inhibitory control, meta-analysis, activation likelihood estimation, fMRI
Citation: Wu J, Xiao W, Yip J, Peng L, Zheng K, Takyi Bentil O and Ren Z (2022) Effects of Exercise on Neural Changes in Inhibitory Control: An ALE Meta-Analysis of fMRI Studies. Front. Hum. Neurosci. 16:891095. doi: 10.3389/fnhum.2022.891095
Received: 07 March 2022; Accepted: 08 June 2022;
Published: 24 June 2022.
Edited by:
Junhao Huang, Guangzhou Sport University, ChinaReviewed by:
Ruiwang Huang, South China Normal University, ChinaMeng Zhang, Xinxiang Medical University, China
Copyright © 2022 Wu, Xiao, Yip, Peng, Zheng, Takyi Bentil and Ren. This is an open-access article distributed under the terms of the Creative Commons Attribution License (CC BY). The use, distribution or reproduction in other forums is permitted, provided the original author(s) and the copyright owner(s) are credited and that the original publication in this journal is cited, in accordance with accepted academic practice. No use, distribution or reproduction is permitted which does not comply with these terms.
*Correspondence: Zhanbing Ren, cnpiQHN6dS5lZHUuY24=
†These authors have contributed equally to this work