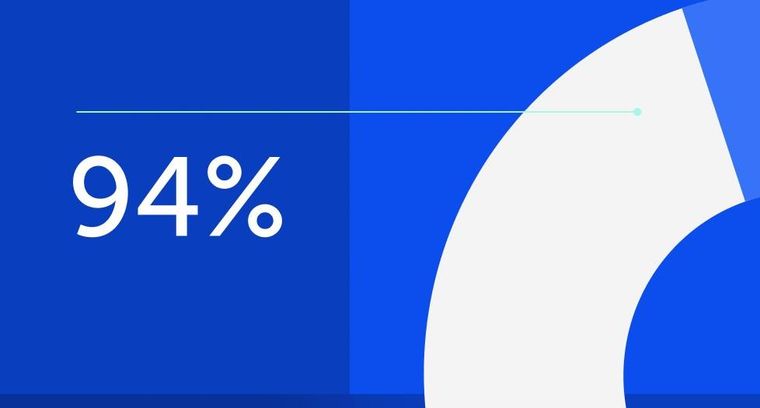
94% of researchers rate our articles as excellent or good
Learn more about the work of our research integrity team to safeguard the quality of each article we publish.
Find out more
ORIGINAL RESEARCH article
Front. Hum. Neurosci., 03 March 2022
Sec. Brain Health and Clinical Neuroscience
Volume 16 - 2022 | https://doi.org/10.3389/fnhum.2022.786853
This article is part of the Research TopicNeuroimaging and Informatics for Successful AgingView all 17 articles
This study employs fMRI to examine the neural substrates of response to cognitive training in healthy old adults. Twenty Japanese healthy elders participated in a 4-week program and practiced a verbal articulation task on a daily basis. Functional connectivity analysis revealed that in comparison to age- and education-matched controls, elders who received the cognitive training demonstrated increased connectivity in the frontotemporal regions related with language and memory functions and showed significant correlations between the behavioral change in a linguistic task and connectivity in regions for goal-oriented persistence and lexical processing. The increased hippocampal connectivity was consistent with previous research showing efficacious memory improvement and change in hippocampal functioning. Moreover, the increased intra-network connectivity following cognitive training suggested an improved neural differentiation, in contrast to the inter-network activation pattern typical in the aging brain. This research not only validates the relationship of functional change in the frontal and temporal lobes to age-associated cognitive decline but also shows promise in turning neural change toward the right direction by cognitive training.
With the increasing aging population around the globe and the lack in effective treatment in dementia, measures to intervene cognitive decline are urgently needed. Cognitive training is one of the strategies that has shown some promise in the retention of cognitive functions for healthy elders and elders at risk for mild cognitive impairments (MCI) (Ball et al., 2002; Belleville, 2008; Valenzuela and Sachdev, 2009; Mowszowski et al., 2010; Rosen et al., 2011). Additionally, studies have suggested that enriching mental activities could moderate the deterioration process as healthy elders participating in social and cognitive activities were less likely to develop MCI and eventually converted to dementia (Wilson et al., 2002; Verghese et al., 2003, 2006; Prince et al., 2013). A recent review on non-pharmaceutical interventions based on 13 reviews or meta-analyses published from 2010 to 2019 reported that cognitive interventions focusing on memory or language skills, executive functions, social interactions, etc. were effective for maintaining or improving cognitive functions in older adults regardless of their cognitive status (Sanjuan et al., 2020). This raises the question of which type of language-based training is effective on older adults who are cognitively normal.
The transmission deficit hypothesis (TDH) has pointed out that the change in linguistic competence is asymmetric in aging, with comprehension relatively preserved and production strongly affected (Burke and Shafto, 2004). Behavioral evidence has shown that word retrieval problem in healthy aging is due to a failure in the complete retrieval of the phonology of the target word, instead of generalized slowing (Shafto et al., 2007). In another study using event-related potential (ERP) and visual evoked potential (VEP), healthy young and older adults performed an implicit picture-naming task while making a segmental or syllabic decision in a Go/No-go paradigm. The older adults showed longer latencies in both behavioral judgment and ERP amplitudes in response to phonological stimuli. In contrast, there was no latency difference between the young and old groups regarding the VEP stimuli. The authors concluded that age-associated delay was only specific to the phonological system and proposed that the practice of phonological skills might improve general linguistic abilities in older adults (Neumann et al., 2009). This study hypothesizes that a linguistic task focusing on articulatory skills will improve general linguistic abilities for two reasons. First, appropriate articulation calls for awareness of linguistic constituents, which are fundamentals for proper word and sentence production. Second, articulatory practice will activate the cortices and connectivity related to phonological retrieval, which has been reported to be the cause of production difficulty and word retrieval problem commonly seen in older adults (Shafto et al., 2007).
The temporal regions, particularly the medial portions, including the hippocampus, were most commonly impacted by neurodegeneration, as studies of MCI and early Alzheimer’s disease (AD) have consistently revealed hippocampus atrophy (Braak and Braak, 1996; Kordower et al., 2001; Scheff et al., 2006). The hippocampus is related to conscious memory recollection, and the hippocampal lesions are associated with memory deficits in MCI and AD (Cohen and Squire, 1981; Tulving, 2002). Improvements in memory and change in hippocampal functioning could suggest alterations in the disease progression of MCI and AD. Therefore, interventions leading to the functional change in the hippocampus might be considered as an effective strategy for retention of cognitive reserve in successful aging. This study predicts the change in the functional connectivity in the hippocampus and sensory-motor regions after articulation training, as the training task taps into the cortices associated with movement and verbal memory. The frontal lobe is also anticipated to show improved connectivity as most previous studies have revealed frontal involvement when cognitive interventions are effective (Sanjuan et al., 2020).
Cognitive neuroscience of aging has addressed the relationship between cognitive function performance with global and local functional activations in the brain. The increased bilateral, mostly prefrontal involvement has been consistently reported in healthy old adults, while other regions either showed decreased or increased activations relative to those in young adults, as discussed in a review (Park and Reuter-Lorenz, 2009). The localized activation difference from the young adults was often interpreted as compensation or degeneration, with conflicting results by different experimental manipulations. Such inconsistency used to be attributed to the paradigm or task demand, with a weak foundation for the argument reasoning. This problem suggested that the investigation of isolated regions in the brain may not suffice to understand the neural change associated with age-induced decline. Therefore, there has been a support for the examination of network connectivity for interacting regions to elucidate the neural bases underlying altered cognitive functions in aging (Antonenko and Floel, 2014).
Functional connectivity (FC) between brain regions reveals the degree of synchronization or temporal correlations of activities in them. It reflects the quality of information transfer among brain regions, which could be achieved by direct white matter pathways or indirect connections through multiple regions (Fox and Raichle, 2007). Previous research has related hyper- and hypo-connectivity in older adults compared with young adults to decreased cognitive functions (Antonenko and Floel, 2014). Various techniques, such as electroencephalography, magnetoencephalography, and functional magnetic resonance imaging (fMRI), have been employed to study functional connectivity (Buldu et al., 2011; Ferreira and Busatto, 2013; Freitas et al., 2013). FC has been analyzed by means of correlational (Fox and Raichle, 2007), non-linear (Buldu et al., 2011), and graph-theoretical approaches (Meunier et al., 2009). This study focuses on the resting-state fMRI connectivity, similar to those in the review of FC approaches (Ferreira and Busatto, 2013), using the inter-regional correlational approach.
Twenty healthy elders (mean age = 69.7, SD = 4.2, 8 women) participated in the cognitive training group, and 20 age-matched controls (mean age = 70.3, SD = 3.6, 10 women) enrolled in this study. As shown in Table 1, there was no significant difference between the training and control groups in the education (training group mean = 12.8, SD = 2.5; control group mean = 11.9, SD = 2.1), WAIS III Vocabulary Scaled Score (training group mean = 11.8, SD = 2.7; control group mean = 12.5, SD = 2.5), Mini-Mental State Examination (MMSE) (training group mean = 29.0, SD = 1.5; control group mean = 29.4, SD = 0.8), geriatric depression scale (GDS) (training group mean = 2.1, SD = 2.3; control group mean = 0.9, SD = 1.2), and H.N. Handedness Test (training group mean = 97.0, SD = 9.5; control group mean = 99.5, SD = 2.4).
Table 1. Baseline scores for the clinical and demographic characteristics of the control and cognitive training groups.
The study procedure is illustrated in Figure 1. After healthy elders were recruited, they were randomly assigned to the training group or control group. The training group was offered explanations of behavioral measurements and scans and the training program, including the training stimuli, program duration, and practice frequency. All the 20 elders agreed to complete the program and were allocated to individual training sessions. The participants assigned to the control group were provided with explanations of behavioral measurements and scans. All of them agreed to participate in the study. Within the 28-day period of the study, research assistants made phone calls to inquire about their willingness to stay in the study and keep elders motivated by encouraging them. No elders dropped out of the study. Behavioral and scan data of all subjects collected on days 1 and 28 were analyzed.
At baseline (Day 1), all participants underwent scanning, including MPRAGE (6 min), resting-state fMRI (7 min), and DTI (8 min). Later, they completed a standardized battery of neuropsychological tests including the verbal fluency test (5 min), Stroop task (10 min), Bochumer Matrices Test (BOMAT) (15 min), WAIS-III digit span (backward only, 5 min), WAIS-III digit symbol (5 min), MMSE (10 min), GDS (3 min), H.N. Handedness (3 min), and a sentence reading task (10 min). The sentences used in the sentence reading task were categorized by sensicality and difficulty into four types: easy non-sensical sentences, difficult non-sensical sentences, easy meaningful sentences, and difficult meaningful sentences. Participants were asked to read 10 items in each type, 40 items in total. Durations of reading four types of sentences were documented for all subjects.
During the period of training, from Days 2 to 27, participants were instructed to complete the verbal articulation of 40 sentences (10 for each condition). They were required to read aloud each sentence 10 times as fast and accurately as possible, with the reading being recorded. The training time lasted 20–25 min/day, with a 1-day break after the 3-day training. Furthermore, participants were checked whether they performed the task properly by phone every 4 days. On Day 28, all participants underwent the scans, neuropsychological battery testing, and the sentence-reading task. The timing arrangement for behavioral tests, sentence reading tasks, rsfMRI, and verbal articulation training is represented in Figure 2.
There is evidence showing that the articulation rate and articulation stability are affected in aging (Tremblay et al., 2019). Particularly, aging is associated with an increase in the duration and duration variability of speech utterances in a number of tasks, such as syllable and non-word reading (Tremblay and Deschamps, 2016; Tremblay et al., 2017, 2018), and non-word repetition (Sadagopan and Smith, 2013). This study employs verbal articulation training as the intervention for two reasons. First, the training is expected to improve the articulation in elders, which they have already experienced difficulties in and might feel frustrated within daily life. Second, the practice of articulation skills is anticipated to influence the performance of other linguistic tasks, as previous studies proposed that failure in phonological retrieval led to other linguistic difficulties, such as word retrieval difficulties and delayed response (Burke and Shafto, 2004; Shafto et al., 2007; Shafto and Tyler, 2014), and suggested that elders might benefit from the training of phonological skills (Neumann et al., 2009).
The psychometric properties of the verbal articulation training included comprehension, language, memory, or articulation functions. The stimuli of the verbal articulation training contained four sets of sentences. The first set was difficult-to-articulate sentences with real words. The second set was an easy-to-articulate sentence with real words. The third set was a difficult-to-articulate sentence with pseudowords. The final set was an easy-to-articulate sentence with pseudowords. The definition of difficult or easy to articulate was determined by the consonants which were late or early acquired by Japanese children. To exclude any confounding factors, the syntactic structure, morae number, word familiarity, and word imageability were matched across the four conditions. All sentences were presented in the order of Subject-Adjective-Object-Verb. Words, such as wa, no, and wo appearing in sentences, were case markers for syntactic features. A typical example for the first set of training stimuli was シホは 私費の 施設を 保守する “Shiho protects a private facility.” An example for the second set was マキは 棚の 刀を 磨く “Maki polishes a sword on the shelf.” In the third and final sets with pseudowords, the sentences also followed an order of Subject-Adjective-Object-Verb with easy or difficult-to-articulate consonants. Table 2 illustrates the weekly training schedule with training and break days.
Images were acquired in a 3 Tesla MR scanner (TIM Trio, Siemens, Erlangen, Germany) with a 12-channel head coil in National Center for Geriatrics and Gerontology. A gradient-echo echo planar imaging (EPI) sequence was used for functional images to measure blood-oxygen level-dependent (BOLD) contrast with the following parameters: repetition time (TR) = 3,000 ms, echo time (TE) = 30 ms, flip angle = 90°, field of view (FOV) = 192 mm, voxel size = 3 × 3 mm, slice thickness = 3 mm, and 39 axial slices with 0.75 mm gaps. A T2-weighted image (TR = 5,920 ms, TE = 95 ms, flip angle = 150°, FOV = 192 mm, voxel size = 0.8 × 0.8 mm, slice thickness = 3 mm, and 39 axial slices with 0.75-mm gaps). For anatomical references, a high-resolution T1-weighted 3D MPRAGE scan covering the whole brain (TR = 2,500 ms, TE = 2.63 ms, flip angle = 7°, FOV = 256 mm, and isotropic voxels 1 × 1 × 1 mm) was obtained for all participants.
We used the CONN functional connectivity toolbox (version 16.a),1 in conjunction with SPM12 (Wellcome Department of Cognitive Neurology, London, United Kingdom),2 to perform all preprocessing steps (using default preprocessing pipeline of CONN) and subsequent statistical analyses. In this preprocessing pipeline, raw functional images were slice-time corrected, realigned (motion-corrected), unwrapped, and coregistered to the MPRAGE image of each subject in accordance with standard algorithms.
Denoising pipeline of CONN employed linear regression to estimate and remove potential confounding factors for each voxel for each subject and functional run/session and then used temporal band-pass filtering to remove temporal frequencies below 0.008 Hz or above 0.09 Hz to focus on slow frequency fluctuations. Potential confounding factors included noise components from cerebral white matter and cerebrospinal areas (Behzadi et al., 2007), estimated subject-motion parameters (Friston et al., 1996), identified outlier scans or scrubbing (Power et al., 2014), constant and first-order linear session effects, and constant task effects (Whitfield-Gabrieli and Nieto-Castanon, 2012). To minimize the BOLD variability caused by head motion, 12 potential noise components were defined from the estimated subject-motion parameters, with 3 translation parameters, 3 rotation parameters, and their associated first-order derivatives.
Images were then normalized to Montreal Neurological Institute coordinate space, spatially smoothed (8-mm full-width at half maximum), and resliced to 2 × 2 × 2 mm voxels. The regions of interest (ROIs) in this study were derived from a freely available ROI atlas defined by correlated activation patterns.3 All ROIs provided by the atlas were used for analysis.
For the calculation of FC, we used the ROI-to-ROI measure, which characterizes the connectivity between all pairs of ROIs among a predefined set of regions. The level of connectivity is represented by an ROI-to-ROI (PRC) matrix, in which each element is defined as the Fisher-transformed bivariate correlation coefficient between a pair of ROI BOLD time series:
where r is a matrix of correlation coefficients, R is the BOLD time series within each ROI, and Z is the RRC matrix of Fisher-transformed correlation coefficients.
The reading time difference of the sentence-reading task of each subject was also added as 2nd level covariates. This yielded the brain maps demonstrating the correlation between the reading time and the post-functional > pre-functional connectivity. All group-level results were corrected for multiple comparisons (false discovery rate, FDR) (p < 0.05) within the CONN toolbox.
In studying the effect of training on the performance of the sentence-reading task, we conducted a three-way ANOVA test on reading speed of the verbal training group. The effects and interactions of three factors were examined, namely, training, sensicality of sentences, and difficulty of sentences. Table 3 shows the analysis of speed (duration) in reading sentences and the effects of training. In comparing the change of neuropsychological battery after 28 days in the training and control groups, we simply subtracted the 2nd measurement from the 1st measurement of these tests in two groups, respectively, and performed an F test on the subtraction difference between the two measurements.
The treatment effect was investigated with the sentence-reading task, neuropsychological battery, and questionnaire data by the F-test. As shown in Table 3, the ANOVA of reading non-sensical and meaning sentences revealed significant main effects of training (p < 0.001), sensicality (p < 0.001), and difficulty (p = 0.022) and interactions of training × sensicality (p < 0.001) and training × sensicality × difficulty (p = 0.009). The differences of the neuropsychological battery in the baseline and follow-up between the control and training groups did not reach significance between groups (cf. Supplementary Table 1).
The regions showing significant connectivity (p < 0.05, uncorrected) are represented in Figure 3 and Table 4. The color in the node circles represents the connectivity intensity, and the size of the node suggests the degree of hubness of the node, with the bigger size being the hub with a larger number of connections to other nodes. The edges are the lines connecting nodes, which represent the connectivity among ROIs. As shown in Figure 3, all the ROIs demonstrated a similar level of intensity. The ROIs with increased connectivity were mostly right-lateralized within the fronto-temporal network, e.g., the middle frontal gyrus, the inferior frontal gyrus opercularis, the hippocampus, parahippocampal gyrus, and the temporo-occipital part of the inferior temporal gyrus. These ROIs belong to the language and memory networks. The language network ROIs included the right inferior frontal gyrus opercularis and inferior temporal gyrus. The memory network ROIs consisted of the right hippocampus, right parahippocampal gyrus, and temporoparietal cortex. The temporal ROIs also exhibited connectivity with the left precentral gyrus, visual cortices, and the left inferior cingulate gyrus.
Figures 4A–D show correlations between connectivity intensity and change in reading speed of the sentences without FDR correction. In this analysis, the behavior-function correlation was calculated by using the change of reading speed as a covariate in the computation of the regional intensity of each ROI and connectivity between ROIs. The nodes in color were the ROIs whose intensities correlated with the behavioral change, and the edges were the ROI-to-ROI connectivity showing correlations with the behavioral change. The frontal pole-planum temporal connectivity significantly correlated with the post-training > pre-training reading speed difference of the difficult non-sensical and easy non-sensical sentences (p < 0.05, FDR-corrected). There was an additional frontal pole-anterior superior temporal gyrus connectivity significantly correlated with the difficult non-sensical sentences (p < 0.05, FDR-corrected). There were no significant correlations after FDR correction between FC and the reading speed difference of easy and difficult meaningful sentences.
Figure 4. (A) Correlations between connectivity difference and reading speed of difficult non-sensical sentences. (B) Correlations between connectivity difference and reading speed of easy non-sensical sentences. (C) Correlations between connectivity difference and reading speed of difficult meaningful sentences. (D) Correlations between connectivity difference and reading speed of easy meaningful sentences.
Although the reading speed of the sentence reading task has been shown to be affected by training, the scores of neuropsychological battery before and after training in the cognitive training group did not reach statistical significance. Neither did the pre- and post-change between the control and training groups reveal significant differences (Supplementary Table 1). A potential reason could be the lack of intensity in a simple task in this study. According to reviews and meta-analysis of interventions that have proven to be more effective in improving general functioning, the intervention programs have the following characteristics: (1) a minimum of 10 weeks of treatment with two sessions per week, (2) session length of 60–90 min, (3) interventions for healthy aging in a group format, (4) study follow-ups included, (5) several cognitive skills worked on at the same time, (6) inclusion of other components related to quality of life, (7) employment of personal, internal, or external strategies, and (8) inclusion of measurements of daily functioning (Sanjuan et al., 2020). The articulation task is a relatively simple task that does not include other components related to the quality of life, such as decreasing depression level and improvement of sleep quality, as suggested in the review research. An articulation task is not a challenging task that requires the assistance of internal or external strategies. Additionally, the training program in this research was in individual format and lacked group dynamics that could boost motivations and confidence to apply the skills to daily life functioning. Consequently, the linguistic improvement resulting from articulation training could not be translated to other behavior measured by the neuropsychological battery.
In contrast, the fMRI connectivity analysis revealed prominently increased connectivity in the ROIs within the language and memory networks in the frontal and temporal lobes after training. The temporal ROIs also exhibited connectivity with the left precentral gyrus, visual cortices, and the left inferior cingulate gyrus. The pathological change in the medial temporal lobe (MTL) has been found to be strongly associated with neurodegeneration in elders (Braak and Braak, 1996; Kordower et al., 2001; Scheff et al., 2006). Research has also reported an association of higher life-span cognitive activity with a reduced rate of hippocampal atrophy in elders at risk for dementia (Valenzuela et al., 2008). Studies that employed active training for healthy elders and effectively slowed down the progression to MCI have shown that training was most efficacious in improving memory (Verghese et al., 2003, 2006; Norrie et al., 2011; Rosen et al., 2011; Prince et al., 2013; Diamond et al., 2015). The enhanced hippocampal connectivity in this study is in accordance with previous research that revealed increased hippocampal activations in elders with MCI after cognitive training (Rosen et al., 2011).
The increased connections of the hippocampus to the cerebellum also suggested enhanced communications to achieve cognitive functions, as previous research has demonstrated a functional link between the cerebellum and hippocampus in humans to facilitate the cognitive aspects of navigation (Igloi et al., 2015). In a previous study, the task-based analyses using 787 subjects from the human connectome project showed that the cerebellar cortex was engaged in language, working memory, movement, social, and emotional task processing (Guell et al., 2018a). In this research, the cerebellum lobule showed increased connectivity with the temporal lobe, which is consistent with the observation that the non-motor processing lobule in the cerebellum supports a cognitive function that is left-lateralized, i.e., language (Guell et al., 2018b; Schmahmann et al., 2019).
Notably, in this study, there was right-lateralized increased connectivity within the frontotemporal network (Figure 3). This suggested that the effectiveness of the training was manifested in enhanced communications among the frontotemporal language regions (i.e., the inferior frontal gyrus opercularis and inferior temporal gyrus) and temporal memory regions (i.e., the hippocampus and parahippocampal gyrus) in the right hemisphere. Prior fMRI studies on healthy aging have generally reported spread instead of localized functional activations in elders relative to young controls (Salami et al., 2012; Spreng and Schacter, 2012). Inter-network connectivity or activations seem to suggest detrimental effects caused by age-related inefficiency, which is consistent with neural dedifferentiation in the aging brain (Park and Reuter-Lorenz, 2009; Antonenko and Floel, 2014; Geerligs et al., 2014). The increased intra-network connectivity following cognitive training in this research could serve as evidence for improved neural differentiation. This also provides a solid foundation for the rationale of turning neural change toward the right direction by cognitive training.
The correlation between FC in the frontal pole and improved performance of the sentence reading task suggested the important role of frontal involvement in driving the task success as a result of articulation training. Recent research has shown that the structural properties of the frontal pole cortex contain information that can differentiate participants with high goal-directed persistence from those with low persistence, regardless of task domains, such as cognitive, language, and motor learning (Hosoda et al., 2020). They also found that participants with high persistence exhibited experience-dependent neuroplastic changes in the frontal pole after completing language and motor learning tasks. The increased connectivity between the frontal pole and planum temporale, which is a region typically involved in auditory processing (Nakada et al., 2001) and lexical processing (Bookheimer, 2002), could also represent the post-training neural plasticity in the fontal pole to complete the task involving auditory and lexical processes. Additionally, the anterior superior temporal gyrus has been reported to be related to sentence processing (Mellem et al., 2016). The connectivity between the anterior superior temporal gyrus and the frontal pole might suggest the training-induced persistence in reading difficult non-sensical sentences.
Regarding maintenance of training effects, previous research has reported that sustained benefits of cognitive interventions were verified in healthy elders for periods of 2 months (Chiu et al., 2017) to 5 years (Kelly et al., 2014) after treatment. The sustainability of the effects also relied on subsequent practices of the training stimuli, similar to any kind of skills. The study follow-ups are crucial for not only maintenance of the effects but also translation of the skills to general functioning (Sanjuan et al., 2020). This study did not follow up with the participants after the study was completed. Our future study will take into consideration long-term follow-ups and measurements of sustained treatment effects.
This study utilizes a simple verbal articulation task for cognitive training in Japanese healthy adults. In comparison to age- and education-matched controls, elders who received the articulation training demonstrated significantly increased connectivity in the right frontotemporal regions, with extended connectivity from temporal regions to cerebellum and visual cortices. The increased hippocampal connectivity was consistent with previous research showing efficacy in intervening cognitive decline and change in hippocampal functioning. Moreover, the increased intra-network connectivity following cognitive training suggested an improved neural differentiation, in contrast to the dedifferentiation pattern in the aging brain. Although the training was simply relative to programs used in other cognitive training studies, the fMRI connectivity analysis showed patterns suggesting a promising functional change in the frontal and temporal regions that are associated with goal-oriented persistence, as well as language and memory functions.
The original contributions presented in the study are included in the article/Supplementary Material, further inquiries can be directed to the corresponding author/s.
The studies involving human participants were reviewed and approved by IRB of National Center for Geriatrics and Gerontology. The patients/participants provided their written informed consent to participate in this study.
F-PY analyzed the data and wrote the manuscript. T-YL and C-HL assisted in the preparation of tables and figures and the organization of references. TN helped in designing the task and organized data collection. SM provided opinions on the results of verbal training from the perspective of oral functions and worked with TN to supervise the collaborative research and review the manuscript draft. All authors contributed to the article and approved the submitted version.
This research was supported by the Japan Society for the Promotion of Science (JSPS) under Grants-in-Aid for Scientific Research (KAKENHI #15H03104 and 19H04025] and the Taiwan Ministry of Education Grant (108J0054RT).
The authors declare that the research was conducted in the absence of any commercial or financial relationships that could be construed as a potential conflict of interest.
All claims expressed in this article are solely those of the authors and do not necessarily represent those of their affiliated organizations, or those of the publisher, the editors and the reviewers. Any product that may be evaluated in this article, or claim that may be made by its manufacturer, is not guaranteed or endorsed by the publisher.
We would like to acknowledge the contribution of Sachiko Kiyama, Ph.D. for designing the verbal training task and supporting data collection.
The Supplementary Material for this article can be found online at: https://www.frontiersin.org/articles/10.3389/fnhum.2022.786853/full#supplementary-material
Antonenko, D., and Floel, A. (2014). Healthy aging by staying selectively connected: a mini-review. Gerontology 60, 3–9. doi: 10.1159/000354376
Ball, K., Berch, D. B., Helmers, K. F., Jobe, J. B., Leveck, M. D., Marsiske, M., et al. (2002). Effects of cognitive training interventions with older adults: a randomized controlled trial. JAMA 288, 2271–2281. doi: 10.1001/jama.288.18.2271
Behzadi, Y., Restom, K., Liau, J., and Liu, T. T. (2007). A component based noise correction method (CompCor) for BOLD and perfusion based fMRI. Neuroimage 37, 90–101. doi: 10.1016/j.neuroimage.2007.04.042
Belleville, S. (2008). Cognitive training for persons with mild cognitive impairment. Int. Psychogeriatr. 20, 57–66. doi: 10.1017/S104161020700631X
Bookheimer, S. (2002). Functional MRI of language: new approaches to understanding the cortical organization of semantic processing. Annu. Rev. Neurosci. 25, 151–188. doi: 10.1146/annurev.neuro.25.112701.142946
Braak, H., and Braak, E. (1996). Evolution of the neuropathology of Alzheimer’s disease. Acta Neurol. Scand. Suppl. 165, 3–12. doi: 10.1111/j.1600-0404.1996.tb05866.x
Buldu, J. M., Bajo, R., Maestu, F., Castellanos, N., Leyva, I., Gil, P., et al. (2011). Reorganization of functional networks in mild cognitive impairment. PLoS One 6:e19584. doi: 10.1371/journal.pone.0019584
Burke, D. M., and Shafto, M. A. (2004). Aging and language production. Curr. Dir. Psychol. Sci. 13, 21–24. doi: 10.1111/j.0963-7214.2004.01301006.x
Chiu, H. L., Chu, H., Tsai, J. C., Liu, D., Chen, Y. R., Yang, H. L., et al. (2017). The effect of cognitive-based training for the healthy older people: a meta-analysis of randomized controlled trials. PLoS One 12:e0176742. doi: 10.1371/journal.pone.0176742
Cohen, N. J., and Squire, L. R. (1981). Retrograde amnesia and remote memory impairment. Neuropsychologia 19, 337–356. doi: 10.1016/0028-3932(81)90064-6
Diamond, K., Mowszowski, L., Cockayne, N., Norrie, L., Paradise, M., Hermens, D. F., et al. (2015). Randomized controlled trial of a healthy brain ageing cognitive training program: effects on memory, mood, and sleep. J. Alzheimers Dis. 44, 1181–1191. doi: 10.3233/JAD-142061
Ferreira, L. K., and Busatto, G. F. (2013). Resting-state functional connectivity in normal brain aging. Neurosci. Biobehav. Rev. 37, 384–400. doi: 10.1016/j.neubiorev.2013.01.017
Fox, M. D., and Raichle, M. E. (2007). Spontaneous fluctuations in brain activity observed with functional magnetic resonance imaging. Nat. Rev. Neurosci. 8, 700–711. doi: 10.1038/nrn2201
Freitas, C., Farzan, F., and Pascual-Leone, A. (2013). Assessing brain plasticity across the lifespan with transcranial magnetic stimulation: why, how, and what is the ultimate goal? Front. Neurosci. 7:42. doi: 10.3389/fnins.2013.00042
Friston, K. J., Williams, S., Howard, R., Frackowiak, R. S., and Turner, R. (1996). Movement-related effects in fMRI time-series. Magn. Reson. Med. 35, 346–355. doi: 10.1002/mrm.1910350312
Geerligs, L., Maurits, N. M., Renken, R. J., and Lorist, M. M. (2014). Reduced specificity of functional connectivity in the aging brain during task performance. Hum. Brain Mapp. 35, 319–330. doi: 10.1002/hbm.22175
Guell, X., Gabrieli, J. D. E., and Schmahmann, J. D. (2018a). Triple representation of language, working memory, social and emotion processing in the cerebellum: convergent evidence from task and seed-based resting-state fMRI analyses in a single large cohort. Neuroimage 172, 437–449. doi: 10.1016/j.neuroimage.2018.01.082
Guell, X., Schmahmann, J. D., Gabrieli, J., and Ghosh, S. S. (2018b). Functional gradients of the cerebellum. Elife 7:e36652. doi: 10.7554/eLife.36652
Hosoda, C., Tsujimoto, S., Tatekawa, M., Honda, M., Osu, R., and Hanakawa, T. (2020). Plastic frontal pole cortex structure related to individual persistence for goal achievement. Commun. Biol. 3:194. doi: 10.1038/s42003-020-0930-4
Igloi, K., Doeller, C. F., Paradis, A. L., Benchenane, K., Berthoz, A., Burgess, N., et al. (2015). Interaction between hippocampus and cerebellum crus I in sequence-based but not place-based navigation. Cereb. Cortex 25, 4146–4154. doi: 10.1093/cercor/bhu132
Kelly, M. E., Loughrey, D., Lawlor, B. A., Robertson, I. H., Walsh, C., and Brennan, S. (2014). The impact of cognitive training and mental stimulation on cognitive and everyday functioning of healthy older adults: a systematic review and meta-analysis. Ageing Res. Rev. 15, 28–43. doi: 10.1016/j.arr.2014.02.004
Kordower, J. H., Chu, Y., Stebbins, G. T., DeKosky, S. T., Cochran, E. J., Bennett, D., et al. (2001). Loss and atrophy of layer II entorhinal cortex neurons in elderly people with mild cognitive impairment. Ann. Neurol. 49, 202–213. doi: 10.1002/1531-8249(20010201)49:2<202::aid-ana40>3.0.co;2-3
Mellem, M. S., Jasmin, K. M., Peng, C., and Martin, A. (2016). Sentence processing in anterior superior temporal cortex shows a social-emotional bias. Neuropsychologia 89, 217–224. doi: 10.1016/j.neuropsychologia.2016.06.019
Meunier, D., Achard, S., Morcom, A., and Bullmore, E. (2009). Age-related changes in modular organization of human brain functional networks. Neuroimage 44, 715–723. doi: 10.1016/j.neuroimage.2008.09.062
Mowszowski, L., Batchelor, J., and Naismith, S. L. (2010). Early intervention for cognitive decline: can cognitive training be used as a selective prevention technique? Int. Psychogeriatr. 22, 537–548. doi: 10.1017/S1041610209991748
Nakada, T., Fujii, Y., Yoneoka, Y., and Kwee, I. L. (2001). Planum temporale: where spoken and written language meet. Eur. Neurol. 46, 121–125. doi: 10.1159/000050784
Neumann, Y., Obler, L. K., Gomes, H., and Shafer, V. (2009). Phonological vs sensory contributions to age effects in naming: an electrophysiological study. Aphasiology 23, 1028–1039. doi: 10.1080/02687030802661630
Norrie, L. M., Diamond, K., Hickie, I. B., Rogers, N. L., Fearns, S., and Naismith, S. L. (2011). Can older “at risk” adults benefit from psychoeducation targeting healthy brain aging? Int. Psychogeriatr. 23, 413–424. doi: 10.1017/S1041610210001109
Park, D. C., and Reuter-Lorenz, P. (2009). The adaptive brain: aging and neurocognitive scaffolding. Annu. Rev. Psychol. 60, 173–196. doi: 10.1146/annurev.psych.59.103006.093656
Power, J. D., Mitra, A., Laumann, T. O., Snyder, A. Z., Schlaggar, B. L., and Petersen, S. E. (2014). Methods to detect, characterize, and remove motion artifact in resting state fMRI. Neuroimage 84, 320–341. doi: 10.1016/j.neuroimage.2013.08.048
Prince, M., Bryce, R., Albanese, E., Wimo, A., Ribeiro, W., and Ferri, C. P. (2013). The global prevalence of dementia: a systematic review and metaanalysis. Alzheimers Dement. 9, 63.e2–75.e2. doi: 10.1016/j.jalz.2012.11.007
Rosen, A. C., Sugiura, L., Kramer, J. H., Whitfield-Gabrieli, S., and Gabrieli, J. D. (2011). Cognitive training changes hippocampal function in mild cognitive impairment: a pilot study. J. Alzheimers Dis. 26(Suppl. 3), 349–357. doi: 10.3233/JAD-2011-0009
Sadagopan, N., and Smith, A. (2013). Age differences in speech motor performance on a novel speech task. J. Speech Lang. Hear. Res. 56, 1552–1566. doi: 10.1044/1092-4388(2013/12-0293)
Salami, A., Eriksson, J., and Nyberg, L. (2012). Opposing effects of aging on large-scale brain systems for memory encoding and cognitive control. J. Neurosci. 32, 10749–10757. doi: 10.1523/JNEUROSCI.0278-12.2012
Sanjuan, M., Navarro, E., and Calero, M. D. (2020). Effectiveness of cognitive interventions in older adults: a review. Eur. J. Investig. Health Psychol. Educ. 10, 876–898. doi: 10.3390/ejihpe10030063
Scheff, S. W., Price, D. A., Schmitt, F. A., and Mufson, E. J. (2006). Hippocampal synaptic loss in early Alzheimer’s disease and mild cognitive impairment. Neurobiol. Aging 27, 1372–1384. doi: 10.1016/j.neurobiolaging.2005.09.012
Schmahmann, J. D., Guell, X., Stoodley, C. J., and Halko, M. A. (2019). The Theory and Neuroscience of Cerebellar Cognition. Annu. Rev. Neurosci. 42, 337–364. doi: 10.1146/annurev-neuro-070918-050258
Shafto, M. A., and Tyler, L. K. (2014). Language in the aging brain: the network dynamics of cognitive decline and preservation. Science 346, 583–587. doi: 10.1126/science.1254404
Shafto, M. A., Burke, D. M., Stamatakis, E. A., Tam, P. P., and Tyler, L. K. (2007). On the tip-of-the-tongue: neural correlates of increased word-finding failures in normal aging. J. Cogn. Neurosci. 19, 2060–2070. doi: 10.1162/jocn.2007.19.12.2060
Spreng, R. N., and Schacter, D. L. (2012). Default network modulation and large-scale network interactivity in healthy young and old adults. Cereb. Cortex 22, 2610–2621. doi: 10.1093/cercor/bhr339
Tremblay, P., and Deschamps, I. (2016). Structural brain aging and speech production: a surface-based brain morphometry study. Brain Struct. Funct. 221, 3275–3299. doi: 10.1007/s00429-015-1100-1
Tremblay, P., Deschamps, I., Bedard, P., Tessier, M. H., Carrier, M., and Thibeault, M. (2018). Aging of speech production, from articulatory accuracy to motor timing. Psychol. Aging 33, 1022–1034. doi: 10.1037/pag0000306
Tremblay, P., Poulin, J., Martel-Sauvageau, V., and Denis, C. (2019). Age-related deficits in speech production: from phonological planning to motor implementation. Exp. Gerontol. 126:110695. doi: 10.1016/j.exger.2019.110695
Tremblay, P., Sato, M., and Deschamps, I. (2017). Age differences in the motor control of speech: an fMRI study of healthy aging. Hum Brain Mapp. 38, 2751–2771. doi: 10.1002/hbm.23558
Tulving, E. (2002). Episodic memory: from mind to brain. Annu. Rev. Psychol. 53, 1–25. doi: 10.1146/annurev.psych.53.100901.135114
Valenzuela, M. J., Sachdev, P., Wen, W., Chen, X., and Brodaty, H. (2008). Lifespan mental activity predicts diminished rate of hippocampal atrophy. PLoS One 3:e2598. doi: 10.1371/journal.pone.0002598
Valenzuela, M., and Sachdev, P. (2009). Can cognitive exercise prevent the onset of dementia? Systematic review of randomized clinical trials with longitudinal follow-up. Am. J. Geriatr. Psychiatry 17, 179–187. doi: 10.1097/JGP.0b013e3181953b57
Verghese, J., LeValley, A., Derby, C., Kuslansky, G., Katz, M., Hall, C., et al. (2006). Leisure activities and the risk of amnestic mild cognitive impairment in the elderly. Neurology 66, 821–827. doi: 10.1212/01.wnl.0000202520.68987.48
Verghese, J., Lipton, R. B., Katz, M. J., Hall, C. B., Derby, C. A., Kuslansky, G., et al. (2003). Leisure activities and the risk of dementia in the elderly. N. Engl. J. Med. 348, 2508–2516. doi: 10.1056/NEJMoa022252
Whitfield-Gabrieli, S., and Nieto-Castanon, A. (2012). Conn: a functional connectivity toolbox for correlated and anticorrelated brain networks. Brain Connect. 2, 125–141. doi: 10.1089/brain.2012.0073
Keywords: fMRI, aging, connectivity, plasticity, hippocampus, rsfMRI = resting state fMRI
Citation: Yang F-PG, Liu T-Y, Liu C-H, Murakami S and Nakai T (2022) Verbal Training Induces Enhanced Functional Connectivity in Japanese Healthy Elderly Population. Front. Hum. Neurosci. 16:786853. doi: 10.3389/fnhum.2022.786853
Received: 30 September 2021; Accepted: 11 January 2022;
Published: 03 March 2022.
Edited by:
Jessica A. Turner, Georgia State University, United StatesReviewed by:
Takashi Tsukiura, Kyoto University, JapanCopyright © 2022 Yang, Liu, Liu, Murakami and Nakai. This is an open-access article distributed under the terms of the Creative Commons Attribution License (CC BY). The use, distribution or reproduction in other forums is permitted, provided the original author(s) and the copyright owner(s) are credited and that the original publication in this journal is cited, in accordance with accepted academic practice. No use, distribution or reproduction is permitted which does not comply with these terms.
*Correspondence: Fan-Pei Gloria Yang, ZmJ5YW5nQGdtYWlsLmNvbQ==
†These authors have contributed equally to this work
Disclaimer: All claims expressed in this article are solely those of the authors and do not necessarily represent those of their affiliated organizations, or those of the publisher, the editors and the reviewers. Any product that may be evaluated in this article or claim that may be made by its manufacturer is not guaranteed or endorsed by the publisher.
Research integrity at Frontiers
Learn more about the work of our research integrity team to safeguard the quality of each article we publish.