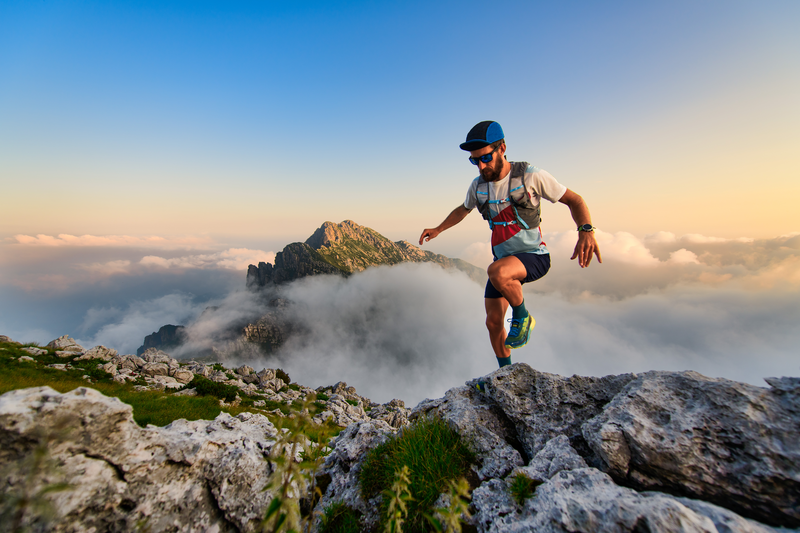
94% of researchers rate our articles as excellent or good
Learn more about the work of our research integrity team to safeguard the quality of each article we publish.
Find out more
ORIGINAL RESEARCH article
Front. Hum. Neurosci. , 04 November 2022
Sec. Motor Neuroscience
Volume 16 - 2022 | https://doi.org/10.3389/fnhum.2022.1022516
This article is part of the Research Topic From Depth (needle) to Surface: Electromyography as a Diagnostic Tool in Identifying Neuromuscular Changes Associated with Neurological Disorders View all 5 articles
Despite its importance, abnormal interactions between the proximal and distal upper extremity muscles of stroke survivors and their impact on functional task performance has not been well described, due in part to the complexity of upper extremity tasks. In this pilot study, we elucidated proximal–distal interactions and their functional impact on stroke survivors by quantitatively delineating how hand and arm movements affect each other across different phases of functional task performance, and how these interactions are influenced by stroke. Fourteen subjects, including nine chronic stroke survivors and five neurologically-intact subjects participated in an experiment involving transport and release of cylindrical objects between locations requiring distinct proximal kinematics. Distal kinematics of stroke survivors, particularly hand opening, were significantly affected by the proximal kinematics, as the hand aperture decreased and the duration of hand opening increased at the locations that requires shoulder abduction and elbow extension. Cocontraction of the extrinsic hand muscles of stroke survivors significantly increased at these locations, where an increase in the intermuscular coherence between distal and proximal muscles was observed. Proximal kinematics of stroke survivors was also affected by the finger extension, but the cocontraction of their proximal muscles did not significantly increase, suggesting the changes in the proximal kinematics were made voluntarily. Our results showed significant proximal-to-distal interactions between finger extension and elbow extension/shoulder abduction of stroke survivors exist during their functional movements. Increased cocontraction of the hand muscles due to increased neural couplings between the distal and proximal muscles appears to be the underlying mechanism.
The human upper extremity is capable of performing a myriad of functional tasks due to its biomechanical structure and its abundant motor and sensory neural innervation. Unfortunately, this dexterity is often compromised by stroke, potentially affecting self-care, employment opportunities, and societal interactions (Cerniauskaite et al., 2012).
While difficulties occurring at the joint or muscle level, such as hypertonicity (Katz and Rymer, 1989), spasticity (Opheim et al., 2014), or weakness (Ada et al., 2003; Kamper et al., 2006) certainly contribute to functional deficits, impairment of the coordination activity of multiple joints may have an even more profound effect (Ada et al., 2006). Past studies examining the arm have revealed altered interjoint kinematics in the paretic arm of stroke survivors (Cirstea et al., 2003) and reduced capacity to create the required joint interaction torques (Beer et al., 2000). Abnormal neurological coupling, as part of a flexion synergy, has also been described between muscles of the elbow and shoulder following stroke (Dewald et al., 1995; Ellis et al., 2009, 2017). These synergies have been shown to extend to finger flexor muscles as well (Miller and Dewald, 2012; McPherson and Dewald, 2019). Indeed, the number of distinct activation patterns available to stroke survivors seems to be reduced (Roh et al., 2012). Increased neural couplings between the distal and proximal muscles could affect the underlying modular structures of the spinal cord that control the arm and hand muscles during functional activities (Cheung et al., 2012).
Object manipulation, a common component of task performance, requires further interjoint coordination as the arm must position, orient, and stabilize the hand while the fingers interact with the external object. Separate neural pathways have been suggested for control of the arm and hand (Galletti et al., 2003; Fattori et al., 2010; Alstermark and Isa, 2012) and the extent of communication between these pathways has been debated (Wing et al., 1996). This coordination is likely impacted by stroke. While grasp-retrieve-release tasks have been widely studied in neurologically intact individuals (Johansson, 1998), they have not been as widely examined after stroke. In particular, the functional impact of the region of the workspace in which the grasp occurs on the hand function of stroke survivors has not been examined. In particular, few studies of this type have combined electromyography (EMG) and kinematic analyses to examine functional movements of the hand and arm following stroke. Because of the high complexity of UE functional tasks (i.e., a large number of DOFs; Valevicius et al., 2019), most kinematic assessments of stroke survivors typically focus on their shoulder and elbow motion, while excluding their finger movements (Schwarz et al., 2019).
In this pilot study, therefore, we aimed to elucidate the impact of abnormal intersegmental interactions of stroke survivors on their function. In particular, we examined in details the kinematics and muscle coordination patterns across all phases of complex functional task performance of chronic stroke survivors and control subjects. In order to overcome aforementioned difficulties, we adopted a within-subject design that varies task conditions (i.e., target locations) to induce systematic changes in proximal kinematics of stroke survivors during functional tasks, thereby allowing us to examine the effects of proximal movements on the distal mechanics of stroke survivors (proximal-to-distal impact) even with the high between-subject variability in their task mechanics. Conversely, distal-to-proximal impact was examined by comparing proximal kinematics during the phases that involved different distal movements (hand open vs. grip) within each subject. We also examined intermuscular coherence between the distal and proximal UE muscles of stroke survivors during task performance to gauge the neural coupling between these muscles, which could indicate underlying mechanisms of functional impairment following stroke (Lemon, 2008). The goal was two-fold; the primary objective was to identify the impact of distal-proximal interactions on task performance. We also aimed to verify if a detailed phase-by-phase analysis can be plausible, given a high between-subject variability of muscle activation and movement kinematics during functional activities (Wagner et al., 2007; Thies et al., 2009).
We expected to observe that the proximal movements of stroke survivors will significantly affect the kinematics and muscle activation of the distal tasks. Here, we anticipated that the abnormal distal-proximal interactions would be detected by a significant increase in the common neural inputs to the distal and proximal muscles. We also expected that the distal movements will also change the proximal kinematics, albeit to a lesser degree, as cortical projection to the arm muscles was found stronger after stroke in comparison to its connections to the hand muscles (Taga et al., 2021).
A total of 14 subjects, including nine chronic stroke survivors (S1–S9) and five neurologically-intact control subjects (C1–C5), participated in the study. Three stroke survivors had severe/severe-moderate upper-limb impairments as indicated by their Fugl-Meyer Assessment of Motor Recovery for the Upper Extremity (FMUE) scores (mean ± SD = 29.7 ± 2.9) and six stroke survivors exhibited moderate-mild UE impairments (FMUE mean ± SD = 44.2 ± 1.9) (see Table 1 for stroke subject characteristics), categorized according to a previous study that classified stroke survivors according to their movement impairments (Woytowicz et al., 2017). All five control subjects were right-handed (age: 30 ± 2.5; 2 women).
The experimental protocol was approved by the Institutional Review Boards of MedStar Health Research Institute, and written informed consent was obtained from each subject prior to participation.
An eight-camera motion capture system (Osprey Motion Analysis Corporation) captured joint kinematics with the sampling rate of 60 Hz. Reflective markers were placed on the following bony landmarks: metacarpophalangeal (MCP), proximal interphalangeal (PIP), and distal interphalangeal (DIP) joints of the index and middle fingers, interphalangeal (IP), MCP, and carpometacarpal (CMC) joints of the thumb, radial and ulnar styloid processes, lateral epicondyle of the elbow, acromion process of the shoulder, and torso (lateral ends of the right and left clavicles, and sternum).
Seven pairs of disposable, self-adhesive Ag/AgCl surface electrodes were placed on the following muscles: extensor digitorum communis (EDC), flexor digitorum superficialis (FDS), first dorsal interosseous (FDI), biceps brachii (BB), lateral head of the triceps brachii (TB), anterior deltoid (AD), and lateral deltoid (LD). During the experiment, EMG signals were amplified, band-pass filtered between 5 and 400 Hz, and sampled at 1 kHz (Myosystem 1600A, Noraxon Inc., AZ, USA).
Subjects were first asked to create maximum activations of seven muscles, which were used to normalize EMG for subsequent analyses. Subjects were first asked to perform isometric contraction of the target muscle while placing each electrode so that muscle palpation can be used to identify the muscle location, which were finger extension (EDC), proximal interphalangeal joint flexion (FDS), index finger abduction (FDI), elbow flexion (BB) and extension (TB), and shoulder flexion (AD) and abduction (LD). The same set of tasks were performed for approximately 2 s to record maximum voluntary contraction of each muscle.
Subjects then sat in front of a table on which five target locations were marked with blue circles. Subjects were strapped to the chair to prevent compensatory torso movements during reaching. The initial location (L0) was marked on the table at waist level in front of the torso and three target locations (L1–L3) were marked in three different movement directions: L1 (45° from midline contralateral to the more-impaired arm), L2 (midline), and L3 (45° from midline ipsilateral to the more-impaired arm). Two other targets were located at shoulder level by using height-adjustable platforms: L4 (30° from midline, contralateral to the more-impaired arm) and L5 (30° from midline, ipsilateral to the more-impaired arm) (Figure 1). Reaching each location requires distinct coordination of proximal DOFs. L1 mainly requires shoulder adduction and elbow extension; L2 mostly elbow extension; L3 shoulder abduction and elbow extension; L4 shoulder flexion and adduction; and L5 shoulder flexion and abduction.
Figure 1. Experimental setup. Subjects were asked to first transport a cylindrical object from the near-body location (L0) to one of the target locations (L1–L5) (“transport” task), which were determined as 50% of their voluntary range of motion (VROM) in each direction. Then they retrieved the object from the target location back to the original location (“retrieve” task). L1 and L4 were located in the contralateral to the more-impaired side, and L3 and L5 in the ipsilateral to the more-impaired side.
Subjects first performed reaching movements in each of the five movement directions to determine maximum voluntary reaching distance in each direction. Stroke survivors used their more-impaired arm while control subjects used their dominant arm. The target location in each direction was then positioned at 50% of the maximum distance each subject could reach for a given target direction in order to make the task difficulty comparable across subjects with different voluntary ranges of motion.
Once the five target locations were determined, for each of these locations, subjects transported a cylindrical object (diameter: 5 cm, height: 15 cm) from the origin (L0) to the target location (“transport” task: T1), released the object, and then returned the hand to the location L0. After a brief rest (2–3 s), the subjects repeated the movement, this time retrieving the object from the target location and returning it to L0 (“retrieve” task: T2). Subjects were instructed not to use compensatory strategies such as torso movements during reaching movements.
Three trials were recorded for each of the five target locations. Target order was randomized for each subject.
From the motion capture data, joint angles for 11 DOFs were computed. For the thumb, flexion angle at the IP, MCP, and CMC joints, denoted by IP1F, MCP1F, and CMC1F, respectively, and the abduction angle at the CMC joint (CMC1A) were computed. For the fingers, flexion angles at the MCP and PIP joints of the index and middle fingers were computed and averaged across the two fingers to yield MCP23F and PIP23F. For the arm, elbow flexion (ELF), forearm supination (FAS), shoulder flexion (SHF), and shoulder abduction (SHA) were estimated from marker data.
Electromyography signals recorded from the seven target muscles were rectified and low-pass filtered at 5 Hz to create activation profiles. These activation profiles were then normalized by the EMG at maximum contraction to compute normalized activation level of these muscles.
The intermuscular coherence was computed between the following distal and proximal muscles: distal muscles: EDC, FDS, and FDI; proximal muscles: BB, TB, AD, and LD, resulting in 12 pairs. Time-varying coherence values were computed between these muscle pairs to examine the degree of distal-proximal neural coupling throughout the entire movements. For each distal-proximal muscle pair, the time-varying EMG-EMG coherence values were estimated from the raw EMG values using a sliding window of duration 500 ms with an increment of 100 ms, within the MATLAB environment (MathWorks, Inc., Natick, MA, US) employing a script by Neurospec (Halliday et al., 1995).1
The coherence values above the 95% confidence level were then z-transformed to yield values normally distributed with a standard deviation of approximately 1 (Rosenberg et al., 1989). The z-transformed coherence was estimated for the frequency bands between 8 and 55 Hz, which include α-, β-, and γ-bands (α-band: 8–12 Hz, β-band: 13–35 Hz, low γ-band, and γ: 36–55 Hz), commonly used to represent common neural inputs associated with corticospinal pathways (Farmer et al., 1993).
Electromyography signals recorded from the seven target muscles were rectified and low-pass filtered at 5 Hz to create activation profiles. These activation profiles were then normalized by the EMG at maximum contraction to compute normalized activation level of these muscles.
From the angular trajectories of the hand and arm joints, initial and final time points (ti,tf) of the six hand submovement periods, from transport (T1) and from retrieval (T2) (Figure 2A and Table 2A), and the four arm submovement periods, from transport (T1) and from retrieval (T2) (Figure 2B and Table 2B), were identified.
Figure 2. Time points of the UE functional movements (object transport/retrieve; control subject 2; L3; trial 2). Here, finger MCP and PIP flexion (MCP23F, PIP23F) (A), shoulder flexion (SHF), elbow flexion (ELF), and hand location (in movement direction) (B) are shown (MCP, metacarpophalangeal; PIP, proximal interphalangeal). The movement periods for hand movement and arm movement periods are listed in Table 1.
Table 2. Definition of time points and periods: (A) Hand movement periods and time points. (B) Arm movement periods and time points.
The hand task kinematics (joint angles), hand muscle activation levels, and the peak intermuscular coherence values were compared between the following hand submovement periods that involved different arm mechanics, using repeated measures analyses of variance (IBM SPSS Statistics; IBM Corp., Armonk, NY, USA). The arm posture (at different time periods) was set as the within-subject variable, and the subject group (controls vs. stroke) as the between-subject variable. For the post-hoc pairwise comparisons, the p-values were corrected for multiple comparisons with a Bonferroni adjustment.
(1) Distal kinematics: hand aperture (finger extension) and grip posture were compared between the time points that involve different proximal kinematics.
• Hand aperture for object grip: at near-body (tf of ) vs. at target (tf of ).
• Hand aperture for object release: at near-body (tf of ) vs. at target (tf of ).
• Grip posture: at near-body (tiof ) vs. at target (tiof ).
(2) Distal muscle activation: mean activation level of three hand muscles (EDC, FDS, and FDI) were compared between the following periods with different proximal kinematics.
• Activation during hand open for object grip: at near-body () vs. at target ().
• Activation during hand open for object release: at near-body () vs. at target ().
(3) Intermuscular coherence: peak intermuscular coherence values of the 12 distal-proximal muscle pairs were compared between the following periods.
• Coherence during hand open for object grip: at near-body () vs. at target ().
• Coherence during hand open for object release: at near-body () vs. at target ().
(4) Movement duration: Durations of the following periods (tf-ti) were compared.
• Duration of hand open for object grip: at near-body () vs. at target ().
• Duration of hand open for object release at near-body () vs. at target ().
The arm kinematics (joint angles) and arm muscle activation levels were compared between the following arm submovement periods that involved different hand mechanics, using repeated measures analyses of variance. The distal task (hand open vs. grip) at different periods was set as the within-subject variable, and the subject group (controls vs. stroke) as the between-subject variable. Again, for the post-hoc analysis, the p-values were corrected with a Bonferroni adjustment.
(1) Proximal kinematics: arm joint angles were compared between the following conditions (two distal tasks).
• Arm posture at target (Ln): with grip (tf of ) vs. with hand open (tf of ).
(2) Proximal muscle activation: mean activation level of four arm muscles (BB, TB, AD, and LD) were compared between the following conditions.
• Mean activation during reaching: with grip () vs. with hand open ().
(3) Movement duration: Durations of the following periods (tf-ti) were compared.
• Duration of reaching: with grip () vs. with hand open ().
Inability to open their hands prevented a subset of stroke survivors (S1–S3) from voluntarily completing the task; three subjects with severe UE impairments could not voluntarily perform the task, as they failed to extend the thumb/fingers enough to grip the object at the near-body location (L0). Thus, only their proximal kinematics and EMG data were included in the analysis. Six stroke survivors with moderate impairment and five neurologically intact control subjects were able to complete the tasks, and all of their data were used for consequent analyses.
Across participants, movements of two proximal DOFs (elbow extension, shoulder abduction) significantly affected distal movements, particularly the maximum hand aperture for object grip (i.e., with no object in hand: vs. ). The responses of the two groups, however, were very different. When opening the hand to grasp the object at the target locations (during ), the hand aperture of stroke survivors became significantly smaller than their aperture at near-body location (L0) (during ), as their finger and the thumb joint flexion angles increased, and the difference was the largest at the target locations that required shoulder abduction (L5) or elbow extension (L2). Control subjects, in contrast, increased their hand aperture at these target locations with respect to the near-body location L0 (group × proximal task interaction; p = 0.04 for MCP23; p = 0.05 for PIP23, p = 0.08 for IP1; Figure 3). A similar group × proximal task interaction was observed during hand opening periods for object release (during vs. during ) but the difference was smaller, and reached statistical significance only for one thumb joint (IP1; p = 0.02).
Figure 3. Distal kinematics of two subject groups under two proximal task conditions ( vs. ). (A) Finger MCP (MCP23F); (B) finger PIP (PIP23F); (C) thumb IP (IP1F) joint flexion angles. For each box, the central mark indicates median, and the bottom and top edges of the box indicates 25th and 75th percentiles, respectively (MCP, metacarpophalangeal; PIP, proximal interphalangeal). The whiskers extend to the most extreme data points excluding outliers (which were indicated by “+” symbols).
In contrast, no significant differences in grip posture were found across locations.
Similar group × proximal task interactions were observed in the muscle activations. When two hand open movements were compared (near-body vs. at target locations L2/L5; vs. ), activation level of both extrinsic hand muscles (EDC and FDS) during hand opening prior to grip significantly increased in stroke survivors at the target locations requiring shoulder abduction/elbow extension (L2/L5) (EDC: 21.4–26.8% at L0 vs. 34.6–52.0% at L2/L5; FDS: 7.9–17.3% at L0 vs. 10.6–33.0% at L2/ L5), but not in control subjects (group × proximal task: p < 0.01 for EDC; p = 0.05 for FDS; Figure 4). Similar trends were found in the activation of the FDI and thenar muscles, but to a lesser degree (p > 0.07).
Figure 4. Muscle activation level during hand open phase under two proximal task conditions ( vs. ). (A) EDC; (B) FDS. For both muscles, the increase in the activation level was significantly greater for stroke survivors (group × proximal task: p < 0.01 for EDC and p = 0.05 for FDS) (EDC, extensor digitorum communis; FDS, flexor digitorum superficialis).
Intermuscular coherence between the hand muscles (EDC, FDS, and FDI) and the agonist/antagonist muscles of each proximal movement (i.e., BB/TB/AD for L2; LD for L5) was significantly higher at the target locations away from the body than the near body location (L0) for both subject groups (Figure 5). During the hand open periods at target locations (L2 or L5, i.e., during approach to retrieve), coherence between distal and proximal muscles increased at the early phase of the reaching movements, which was followed by the increase in the activation of the distal muscles (EDC and FDS; during , Figure 6). Such an increase in cocontraction during the hand open phase was not observed when the hand was near the body (during , Figure 6).
Figure 5. Peak intermuscular coherence value during hand open phase under two proximal task conditions ( vs. ). (A) EDC and proximal muscle pairs. (B) FDS and proximal muscle pairs (EDC, extensor digitorum communis; FDS, flexor digitorum superficialis).
Figure 6. Representative case of muscle activation and intermuscular coherence during task (Stroke subject 4, location 2, trial 1). (A–C) Activation profile of EDC, FDS, and BB. (D–E) Time-frequency plot of intermuscular coherence of EDC-BB and FDS-BB pairs. Increase in the intermuscular coherence in the β- (13–35 Hz) and low γ- (36–55 Hz) bands was mainly observed during early phase of the reaching movements toward the target (i.e., the first half of PH3), which was followed by an increase in the activation of the distal muscles (EDC and FDS). Such patterns were not observed at near-body location (i.e., during PH1) (EDC, extensor digitorum communis; FDS, flexor digitorum superficialis; BB, Biceps brachi).
The type of distal movements (hand open vs. grip) also significantly affected coordination of proximal DOFs (shoulder abduction and elbow extension) during outward movements (i.e., reaching–: transport/reaching with grip vs. : approach/reaching with hand open). The largest difference in proximal kinematics was observed in the target locations that required shoulder abduction (L3 and L5).
For some proximal DOFs, similar patterns of distal-to-proximal impact were observed in both subject groups: subjects adopted postures with greater elbow flexion (p < 0.01) (Figure 7A) and smaller shoulder flexion (p < 0.01) during approach (: distal task–hand open) than transport (: distal task: grip) (Figure 7C).
Figure 7. Proximal kinematics of two subject groups under two distal task conditions ( vs. ). (A) Elbow flexion (ELF). (B) Shoulder abduction (SHA). (C) Shoulder flexion (SHF).
The distal-to-proximal impact on other proximal DOFs, however, differed between the groups (i.e., significant group × distal task interaction). Shoulder abduction of stroke survivors was greater during approach (: hand open) than during transport (: grip), while that of control subjects was similar during these two tasks (Figure 7B; group × distal task p < 0.01). Conversely, the difference in elbow flexion between approach (: with hand open) and transport (: with grip) was greater for control subjects than for stroke survivors (Figure 7A; group × distal task p = 0.06).
When the arm muscle activation patterns were compared between the two hand movements (hand open vs. grip), however, there was no significant between-task differences in the activation level of most arm muscles, except for the elbow extensor muscle (TB), which exhibited a higher activation level when reaching with hand open (p = 0.01). No significant group differences or group × distal task interactions were found.
Movement time of stroke survivors was significantly longer than that of control subjects for both distal (hand open) and proximal (reaching) movements (both p-values ≤0.01) (Figures 8A,B).
A similar impact of proximal movements on the movement duration of distal segments was observed in both subject groups. Hand open duration was longer when subjects approach the object () than when they released it () (object: p = 0.03), and the amount of increase in movement duration was greater at certain target locations (L2 and L5) when compared to the near-body location (L0) (proximal task × object: p = 0.01). Similar trends (i.e., proximal task—object interaction) were observed in both groups, although the amount of increase in movement duration due to proximal task was greater for stroke subjects (Figure 8A) (group × proximal task × object: p = 0.08).
The impact of distal movements on the proximal movement duration, however, was different between the subject groups. Reaching movements of stroke survivors lasted longer when they approached the object (; distal task—hand open) than when they transported the object (; distal task—grip), while the movement duration of healthy subjects was similar between these two phases ( vs. ) (group × object: p = 0.05; Figure 8B).
Deficits in the ability to extend the fingers and thumb are commonly observed after stroke (Kamper and Rymer, 2001; Kamper et al., 2003, 2006; Lang et al., 2009). This reduced digit extension is thought to significantly contribute to overall functional impairment of stroke survivors. Active finger extension of stroke survivors in the early phase of recovery is predictive of eventual recovery of overall upper extremity function (Smania et al., 2007; Stinear et al., 2012) or recovery after movement therapy (Fritz et al., 2005).
Our results highlighted the importance of finger/thumb extension in functional task performance of stroke survivors. In accordance with our hypothesis, we found a significant proximal-to-distal impact (which was stronger than the distal-to-proximal impact), mainly observed during the tasks that involved hand opening. For the stroke survivors with severe impairments (Fugl-Meyer UE score <25), an inability to open their hands prevented them from performing the reach-transport-release task, as they were unable to grip the object. This is consistent with previous studies that showed successful grip of objects by stroke survivors can only be achieved when they restore their ability to extend the fingers and thumb (Lang et al., 2009).
Even for the participants with moderate impairment, although they were able to complete the task, significant changes in the task mechanics were observed, especially during movements requiring finger/thumb extension. First, the duration of the hand opening phase was found to be significantly longer than that for neurologically-intact subjects. In contrast, the difference between the two groups in movement duration while gripping (transporting) the object was much smaller. Unlike neurologically-intact subjects, the hand aperture of stroke survivors during retrieval was significantly smaller for distant targets than for the near-body locations, likely contributing to the observed delays in hand openings. Finger/thumb extension of stroke survivors also affected their proximal mechanics; the shoulder abduction of stroke survivors during object approach (with hand open) was significantly greater than that during object transport (with grip).
Proper adjustment of hand aperture during object retrieval is an important motor skill developed over time (Kuhtz-Buschbeck et al., 1998). In neurologically intact individuals, hand aperture is adjusted by visual feedback; when visual information of the object (size/shape) is unavailable, hand aperture is increased (exaggerated hand opening) to deal with the uncertainty (Jakobson and Goodale, 1991). Hand aperture during retrieval also needs to be increased to compensate for potential directional errors in reaching movements, which affect hand placement with respect to the object (Bootsma et al., 1994).
In accordance with the previous studies, in our experiments, control subjects increased their hand aperture during retrieval of the object at the target locations (farther from the body), possibly to compensate for potential directional errors in reaching movements or due to reduced visual information of the hand aperture due to the distance. Stroke survivors, however, had decreased hand aperture when retrieving objects from target locations that require shoulder abduction or elbow extension. This decrease in aperture could have significantly hampered their ability to place/orient the hand to be able to grip the object, as well as increasing the task duration.
The observed reduction in the hand aperture of stroke survivors at these target locations resulted from global increases in the activation levels of the hand muscles, particularly those of the extrinsic muscles. As the cross-sectional areas of the extrinsic flexor muscles (e.g., flexor digitorum profundus and FDS) are much greater than those of extrinsic extensor muscles (EDC or extensor indicis proprius) (Lieber et al., 1992; Valero-Cuevas et al., 1998), equal coactivation of extrinsic finger muscles would produce a net flexion. Substantial activation of extensor muscles is needed to counteract even a moderate increase in activation of flexor muscles; this increase may be challenging for stroke survivors to achieve as they often have limited capacity to activate their extrinsic finger extensor muscles (Hoffmann et al., 2016). Indeed, activation of both extrinsic hand muscles, EDC (extensor) and FDS (flexor), increased to a similar degree as stroke survivors opened their hands at these target locations (mean ± SD change in activation from L0 to L2/L5: EDC = 20.4 ± 7.8%, FDS = 19.2 ± 22.8%), thereby resulting in the fingers and thumb being more flexed when reaching the target, in a manner similar to the typical flexion synergy patterns observed in stroke survivors (Brunnstrom, 1970). In contrast, no significant changes were observed in the muscle activation pattern of control subjects (mean ± SD change in activation from L0 to L2/L5: EDC = 1.3 ± 1.2%, FDS = 0.6 ± 9.0%).
The increase in the activation levels of the hand muscles appears to be related to the increase in the intermuscular coherence at these target locations. Previous studies observed that significant increase in the intermuscular coherence of the hand muscles was accompanied by a concurrent increase in all muscle activation levels (i.e., higher cocontraction) (Danna-Dos Santos et al., 2010; Vermillion et al., 2015). Abnormal antagonist muscle cocontraction in other neurological conditions (i.e., dystonia) was also linked to the increased intermuscular coherence (Farmer et al., 1998). Thus, our results suggest that the increase in the hand muscle activation level at the target locations that require shoulder abduction/elbow extension could be explained by the increased neural coupling between the hand (EDC and FDS) and the arm (BB, TB, AD, and LD) muscles.
A significant distal-to-proximal impact on task mechanics was also observed across groups, as subjects extended their elbow further during object release (). Stroke survivors also abducted their shoulder as they approached the object (). However, unlike the proximal-to-distal impact, no significant group differences or group × task interactions were found in the activation level of the arm muscles.
Therefore, the observed group × distal task interactions in the proximal kinematics (elbow flexion and shoulder abduction) appear to be different from the proximal-to-distal effects, which resulted from a significant increase in distal muscle cocontraction. The observed changes in the proximal movement pattern of stroke survivors (e.g., increased shoulder abduction during the hand open phase) is likely due to their compensatory strategy. For instance, during approach (distal task: hand open), it was observed that some stroke survivors had to move their hands laterally to grip the object from the side due to the decreased hand aperture. This required greater shoulder abduction, which could lead to further hand closing in accordance with abnormal flexion synergy patterns (i.e., elbow flexion-shoulder abduction; Brunnstrom, 1970).
Our study emphasizes the interactions between proximal and distal limb movements in UE functional activities performed by stroke survivors. The ability to extend the fingers and thumb is a prerequisite to object transport, as stroke survivors who could not open their hands were not able to initiate the transport. Even for those participants who were able to perform the task, proximal arm postures and motions significantly affected finger/thumb extension (reduced hand aperture), while deficits in hand opening required compensatory changes in proximal kinematics. Therefore, restoration of the ability to extend the impaired fingers and thumb should be a primary focus for stroke rehabilitation, even for those who retain the ability to open the hand. More importantly, hand opening should be practiced in conjunction with proximal (reaching) movements so that stroke survivors can learn to overcome the abnormal distal-proximal coupling that affects their task performance.
The number of subjects in this pilot study was small as we aimed to test feasibility of the detailed analysis of kinematic/physiological data throughout the complex upper extremity functional task. Therefore, the results from this study need to be replicated in a future study with a larger number of subjects in order for its findings to be generalized. The small subject number could also have led to type-II errors in the changes in the kinematic (EMG) and physiological (muscle activation) measures, whose p-values were greater than 0.05. Observational studies are required to examine the impact of abnormal proximal-distal interactions in daily activities of stroke survivors.
Between-subject variability in the impairment of stroke survivors could have contributed to the variability in the data. The level of spasticity of stroke subjects, as well as the degree of their sensory deficits, was neither measured nor controlled, which could have contributed to the between-subject variability observed in the data.
The control subjects were much younger than the stroke survivors tested in this study, which could have contributed to the observed between-group differences, in particular the movement time. However, previous studies showed that, while older subjects make slower movements during reaching, their movement coordination patterns remain similar to that of younger subjects (Bennett and Castiello, 1994).
Some stroke subjects adopted compensatory strategies, involving torso movements (mainly tilting and twisting, since the torso was strapped to the chair), during reaching movements. Experimenters instructed subjects not to use such strategy, whenever detected, but it is possible that stroke survivors used torso movements to a certain degree during reaching; this could have reduced the requirements in their arm extension, or could have affected the hand orientation.
In this pilot study, we delineated how abnormal coupling between the movements of distal and proximal UE segments of chronic stroke survivors affect their functional movements. In particular, hand open (distal) and elbow extension and shoulder abduction (proximal) movements influence each other, resulting in a significant reduction in the distal range of motion (finger extension) and an abnormal proximal synergy pattern (increased elbow flexion/shoulder abduction) that can degrade task performance. Increased cocontraction of the hand muscles due to higher neural couplings between the distal and proximal muscles, indicated by the significant increase in the intermuscular coherence, appears to be the underlying mechanism of the observed functional deficits. The outcome of this study suggests that the finger/thumb extension of chronic stroke survivors should be practiced in conjunction with specific proximal movements (elbow extension/shoulder abduction) to help them overcome the abnormal distal-proximal coupling affecting task performance.
The raw data supporting the conclusions of this article will be made available by the authors, without undue reservation.
The studies involving human participants were reviewed and approved by the Institutional Review Boards of MedStar Health Research Institute. The patients/participants provided their written informed consent to participate in this study.
SL and BV designed the study. TP, HN, and BV collected the data. TP, HN, and SL analyzed the data. All authors interpreted the data, wrote the manuscript, and read and approved the final manuscript.
This work was in part supported by the National Science Foundation under Grant CBET1452763, and by the National Institute on Disability, Independent Living, and Rehabilitation Research under Grant 90REGE0004.
The authors declare that the research was conducted in the absence of any commercial or financial relationships that could be construed as a potential conflict of interest.
All claims expressed in this article are solely those of the authors and do not necessarily represent those of their affiliated organizations, or those of the publisher, the editors and the reviewers. Any product that may be evaluated in this article, or claim that may be made by its manufacturer, is not guaranteed or endorsed by the publisher.
Ada, L., Canning, C. T., and Low, S. L. (2003). Stroke patients have selective muscle weakness in shortened range. Brain 126, 724–731. doi: 10.1093/brain/awg066
Ada, L., O’Dwyer, N., and O’Neil, E. (2006). Relation between spasticity, weakness and contracture of the elbow flexors and upper limb activity after stroke: An observational study. Disabil. Rehabil. 28, 891–897. doi: 10.1080/09638280500535165
Alstermark, B., and Isa, T. (2012). Circuits for skilled reaching and grasping. Annu. Rev. Neurosci. 35, 559–578. doi: 10.1146/annurev-neuro-062111-150527
Beer, R., Dewald, J. P. A., and Rymer, W. Z. (2000). Deficits in the coordination of multijoint arm movements in patients with hemiparesis: Evidence for disturbed control of limb dynamics. Exp. Brain Res. 131, 305–319. doi: 10.1007/s002219900275
Bennett, K. M. B., and Castiello, U. (1994). Reach to grasp: Changes with age. J. Gerontol. 49, 1–7. doi: 10.1093/geronj/49.1.P1
Bootsma, R. J., Marteniuk, R. G., MacKenzie, C. L., and Zaal, F. T. (1994). The speed-accuracy trade-off in manual prehension: Effects of movement amplitude, object size and object width on kinematic characteristics. Exp. Brain Res. 98, 535–541. doi: 10.1007/BF00233990
Brunnstrom, S. (1970). Movement therapy in hemiplegia: A neurophysiological approach. New York, NY: Harper & Row.
Cerniauskaite, M., Quintas, R., Koutsogeorgou, E., Meucci, P., Sattin, D., Leonardi, M., et al. (2012). Quality-of-life and disability in patients with stroke. Am. J. Phys. Med. Rehabil. 91, 39–47. doi: 10.1097/PHM.0b013e31823d4df7
Cheung, V. C., Turolla, A., Agostini, M., Silvoni, S., Bennis, C., Kasi, P., et al. (2012). Muscle synergy patterns as physiological markers of motor cortical damage. Proc. Natl. Acad. Sci. U.S.A. 109, 14652–14656. doi: 10.1073/pnas.1212056109
Cirstea, M. C., Mitniski, A. B., Feldman, A. G., and Levin, M. F. (2003). Interjoint coordination dynamics during reaching in stroke. Exp. Brain Res. 151, 289–300. doi: 10.1007/s00221-003-1438-0
Danna-Dos Santos, A., Poston, B., Jesunathadas, M., Bobich, L. R., Hamm, T. M., and Santello, M. (2010). Influence of fatigue on hand muscle coordination and EMG-EMG coherence during three-digit grasping. J. Neurophysiol. 104, 3576–3587. doi: 10.1152/jn.00583.2010
Dewald, J. P., Pope, P. S., Given, J. D., Buchanan, T. S., and Rymer, W. Z. (1995). Abnormal muscle coactivation patterns during isometric torque generation at the elbow and shoulder in hemiparetic subjects. Brain 118, 495–510. doi: 10.1093/brain/118.2.495
Ellis, M. D., Schut, I., and Dewald, J. P. A. (2017). Flexion synergy overshadows flexor spasticity during reaching in chronic moderate to severe hemiparetic stroke. Clin. Neurophysiol. 128, 1308–1314. doi: 10.1016/j.clinph.2017.04.028
Ellis, M. D., Sukal-Moulton, T., and Dewald, J. P. A. (2009). Progressive shoulder abduction loading is a crucial element of arm rehabilitation in chronic stroke. Neurorehabil. Neural Repair 23, 862–869. doi: 10.1177/1545968309332927
Farmer, S. F., Brenner, F. D., Halliday, D. M., Rosenberg, J. R., and Stephans, J. A. (1993). The frequency content of common synaptic inputs to motoneurons studies during isometric voluntary contraction in man. J. Physiol. 470, 127–155. doi: 10.1113/jphysiol.1993.sp019851
Farmer, S. F., Sheean, G. L., Mayston, M. J., Rothwell, J. C., Marsden, C. D., Conway, B. A., et al. (1998). Abnormal motor unit synchronization of antagonist muscles underlies pathological co-contraction in upper limb dystonia. Brain 121, 801–814. doi: 10.1093/brain/121.5.801
Fattori, P., Raos, V., Breveglieri, R., Bosco, A., Marzocchi, N., and Galletti, C. (2010). The dorsomedial pathway is not just for reaching: Grasping neurons in the medial parieto-occipital cortex of the macaque monkey. J. Neurosci. 30, 342–349. doi: 10.1523/JNEUROSCI.3800-09.2010
Fritz, S. L., Light, K. E., Patterson, T. S., Behrman, A. L., and Davis, S. B. (2005). Active finger extension predicts outcomes after constraint-induced movement therapy for individuals with hemiparesis after stroke. Stroke 36, 1172–1177. doi: 10.1161/01.STR.0000165922.96430.d0
Galletti, C., Kutz, D. F., Gamberini, M., Breveglieri, R., and Fattori, P. (2003). Role of the medial parieto-occipital cortex in the control of reaching and grasping movements. Exp. Brain Res. 153, 158–170. doi: 10.1007/s00221-003-1589-z
Halliday, D. M., Rosenberg, J. R., Amjad, A. M., Breeze, P., Conway, B. A., and Farmer, S. F. (1995). A framework for the analysis of mixed time series/point process data – Theory and application to the study of physiological tremor, single motor unit discharges and electromyograms. Prog. Biophys. Mol. Biol. 64, 237–278. doi: 10.1016/S0079-6107(96)00009-0
Hoffmann, G., Conrad, M. O., Qiu, D., and Kamper, D. G. (2016). Contributions of voluntary activation deficits to hand weakness after stroke. Top. Stroke Rehabil. 23, 384–392. doi: 10.1179/1945511915Y.0000000023
Jakobson, L. S., and Goodale, M. A. (1991). Factors affecting higher-order movement planning: A kinematic analysis of human prehension. Exp. Brain Res. 86, 199–208. doi: 10.1007/BF00231054
Johansson, R. S. (1998). Sensory input and control of grip. Novartis Found. Symp. 218, 45–59. doi: 10.1002/9780470515563.ch4
Kamper, D. G., and Rymer, W. Z. (2001). Impairment of voluntary control of finger motion following stroke: Role of inappropriate muscle coactivation. Muscle Nerve 24, 673–681. doi: 10.1002/mus.1054
Kamper, D. G., Fischer, H. C., Cruz, E. G., and Rymer, W. Z. (2006). Weakness is the primary contributor to finger impairment in chronic stroke. Arch. Phys. Med. Rehabil. 87, 1262–1269. doi: 10.1016/j.apmr.2006.05.013
Kamper, D. G., Harvey, R. L., Suresh, S., and Rymer, W. Z. (2003). Relative contributions of neural mechanisms versus muscle mechanics in promoting finger extension deficits following stroke. Muscle Nerve 28, 309–318. doi: 10.1002/mus.10443
Katz, R. T., and Rymer, W. Z. (1989). Spastic hypertonia: Mechanisms and measurement. Arch. Phys. Med. Rehabil. 70, 144–155.
Kuhtz-Buschbeck, J. P., Stolze, H., Jöhnk, K., Boczek-Funcke, A., and Illert, M. (1998). Development of prehension movements in children: A kinematic study. Exp. Brain Res. 122, 424–432. doi: 10.1007/s002210050530
Lang, C. E., DeJong, S. L., and Beebe, J. A. (2009). Recovery of thumb and finger extension and its relation to grasp performance after stroke. J. Neurophysiol. 102, 451–459. doi: 10.1152/jn.91310.2008
Lemon, R. N. (2008). Descending pathways in motor control. Annu. Rev. Neurosci. 31, 195–218. doi: 10.1146/annurev.neuro.31.060407.125547
Lieber, R. L., Jacobson, M. D., Fazeli, B. M., Abrams, R. A., and Botte, M. J. (1992). Architecture of selected muscles of the forearm: Anatomy and implications for tendon transfer. J. Hand. Surg. (Am.) 17, 787–798. doi: 10.1016/0363-5023(92)90444-T
McPherson, L. M., and Dewald, J. P. A. (2019). Differences between flexion and extension synergy-driven coupling at the elbow, wrist, and fingers of individuals with chronic hemiparetic stroke. Clin. Neurophysiol. 130, 454–468. doi: 10.1016/j.clinph.2019.01.010
Miller, L. C., and Dewald, J. P. A. (2012). Involuntary paretic wrist/finger flexion forces and EMG increase with shoulder abduction load in individuals with chronic stroke. Clin. Neurophysiol. 123, 1216–1225. doi: 10.1016/j.clinph.2012.01.009
Opheim, A., Danielsson, A., Alt Murphy, M., Persson, H. C., and Sunnerhagen, K. S. (2014). Upper-limb spasticity during the first year after stroke. Am. J. Phys. Med. Rehabil. 93, 884–896. doi: 10.1097/PHM.0000000000000157
Roh, J., Rymer, W. Z., and Beer, R. F. (2012). Robustness of muscle synergies underlying three-dimensional force generation at the hand in healthy humans. J. Neurophysiol. 107, 2123–2142. doi: 10.1152/jn.00173.2011
Rosenberg, J. R., Amjad, A. M., Breeze, P., Brillinger, D. R., and Halliday, D. M. (1989). The Fourier approach to the identification of functional coupling between neuronal spike trains. Prog. Biophys. Mol. Biol. 53, 1–31. doi: 10.1016/0079-6107(89)90004-7
Schwarz, A., Kanzler, C. M., Lambercy, O., Luft, A. R., and Verbeek, J. M. (2019). Systematic review on kinematic assessments of upper limb movements after stroke. Stroke 50, 718–727. doi: 10.1161/STROKEAHA.118.023531
Smania, N., Paolucci, S., Tinazzi, M., Burgher, A., Manganotti, P., Fiaschi, A., et al. (2007). Active finger extension: A simple movement predicting recovery of arm function in patients with acute stroke. Stroke 38, 1088–1090. doi: 10.1161/01.STR.0000258077.88064.a3
Stinear, C. M., Barber, P. A., Petoe, M., Anwar, S., and Byblow, W. D. (2012). The PREP algorithm predicts potential for upper limb recovery after stroke. Brain 135, 2527–2535. doi: 10.1093/brain/aws146
Taga, M., Charalambos, C., Raju, S., Lin, J., Zhang, Y., Stern, E., et al. (2021). Corticoreticulospinal tract neurophysiology in an arm and hand muscle in healthy and stroke subjects. J. Physiol. 599, 3955–3971. doi: 10.1113/JP281681
Thies, S. B., Tresadern, P. A., Kenney, L. P., Smith, J., Howard, D., Goulermas, J. Y., et al. (2009). Movement variability in stroke patients and controls performing two upper limb functional tasks: A new assessment methodology. J. Neuroeng. Rehabil. 6:2. doi: 10.1186/1743-0003-6-2
Valero-Cuevas, F. J., Zajac, F. E., and Burgar, C. G. (1998). Large index-fingertip forces are produced by subject-independent patterns of muscle excitation. J. Biomech. 31, 693–703. doi: 10.1016/S0021-9290(98)00082-7
Valevicius, A. M., Boser, Q. A., Lavoie, E. B., Chapman, C. S., Pilarski, P. M., Hebert, J. S., et al. (2019). Characterization of normative angular joint kinematics during two functional upper limb tasks. Gait Posture 69, 176–186. doi: 10.1016/j.gaitpost.2019.01.037
Vermillion, B. C., Lum, P. S., and Lee, S. W. (2015). Proximal arm kinematics affect grip force-load force coordination. J. Neurophysiol. 114, 2265–2277. doi: 10.1152/jn.00227.2015
Wagner, J. M., Dromerick, A. W., Sahrmann, S. A., and Lang, C. E. (2007). Upper extremity muscle activation during recovery of reaching in subjects with post-stroke hemiparesis. Clin. Neurophysiol. 118, 164–176. doi: 10.1016/j.clinph.2006.09.022
Wing, A. M.,Haggard, P. and Flanagan, J. R. (1996). Hand and brain: The neurophysiology and psychology of hand movements. Cambridge, MA: Academic Press.
Woytowicz, E. J., Rietschel, J. C., Goodman, R. N., Conroy, S. S., Sorkin, J. D., Whitall, J., et al. (2017). Determining levels of upper extremity movement impairment by applying a cluster analysis to the Fugl-Meyer assessment of the upper extremity in chronic stroke. Arch. Phys. Med. Rehabil. 98, 456–462. doi: 10.1016/j.apmr.2016.06.023
Keywords: stroke, upper extremity, abnormal coupling, finger extension, abnormal synergy, intermuscular coherence
Citation: Phan T, Nguyen H, Vermillion BC, Kamper DG and Lee SW (2022) Abnormal proximal-distal interactions in upper-limb of stroke survivors during object manipulation: A pilot study. Front. Hum. Neurosci. 16:1022516. doi: 10.3389/fnhum.2022.1022516
Received: 18 August 2022; Accepted: 18 October 2022;
Published: 04 November 2022.
Edited by:
Suruliraj Karthikbabu, Kovai Medical Center Research and Educational Trust, IndiaReviewed by:
Senthil D. Kumaran, Manipal Academy of Higher Education, IndiaCopyright © 2022 Phan, Nguyen, Vermillion, Kamper and Lee. This is an open-access article distributed under the terms of the Creative Commons Attribution License (CC BY). The use, distribution or reproduction in other forums is permitted, provided the original author(s) and the copyright owner(s) are credited and that the original publication in this journal is cited, in accordance with accepted academic practice. No use, distribution or reproduction is permitted which does not comply with these terms.
*Correspondence: Sang Wook Lee, bGVlc3dAY3VhLmVkdQ==
Disclaimer: All claims expressed in this article are solely those of the authors and do not necessarily represent those of their affiliated organizations, or those of the publisher, the editors and the reviewers. Any product that may be evaluated in this article or claim that may be made by its manufacturer is not guaranteed or endorsed by the publisher.
Research integrity at Frontiers
Learn more about the work of our research integrity team to safeguard the quality of each article we publish.