- 1Department of Psychology, Hokkaido University, Sapporo, Japan
- 2INSERM - U1093 Cognition, Action, and Sensorimotor Plasticity, Dijon, France
While common semantic representations for individual words across languages have been identified, a common meaning system at sentence-level has not been determined. In this study, fMRI was used to investigate whether an across-language sentence comprehension system exists. Chinese–Japanese bilingual participants (n = 32) were asked to determine whether two consecutive stimuli were related (coherent) or not (incoherent) to the same event. Stimuli were displayed with three different modalities (Chinese written sentences, Japanese written sentences, and pictures). The behavioral results showed no significant difference in accuracy and response times among the three modalities. Multi-voxel pattern analysis (MVPA) of fMRI data was used to classify the semantic relationship (coherent or incoherent) across the stimulus modalities. The classifier was first trained to determine coherency within Chinese sentences and then tested with Japanese sentences, and vice versa. A whole-brain searchlight analysis revealed significant above-chance classification accuracy across Chinese and Japanese sentences in the supramarginal gyrus (BA 40), extending into the angular gyrus (BA 39) as well as the opercular (BA 44) and triangular (BA 45) parts of the inferior frontal gyrus in the left hemisphere (cluster-level FWE corrected p < 0.05). Significant above-chance classification accuracy was also found across Japanese sentences and pictures in the supramarginal (BA 40) and angular gyrus (BA 39). These results indicate that a common meaning system for sentence processing across languages and modalities exists, and it involves the left inferior parietal gyrus.
Introduction
Some of the languages in existence nowadays share similarities in phonological and/or orthographic properties, while others do not. However, all human beings are capable of acquiring another language besides their native language. Language is a symbolic representation of the knowledge of the world, the meaning which is also known as semantics in the domain of linguistics. It is possible to assume that the neurobiological infrastructure that is largely shared among humans is likely to be the neural system that underlines semantic processing (Binder et al., 2009; Hagoort, 2014).
The comprehension of semantics requires the automatic parallel processing of sound, word, and sentence patterns (Fromkin et al., 2014). Nevertheless, the semantic properties of words/sentences are readily distinguished from their structural properties (Binder et al., 2009). Also, the neural processing of different language structures is distinguishable (e.g., Tan et al., 2005; Grodzinsky and Friederici, 2006; Buchweitz et al., 2009), even as the brain regions overlap to some degree (e.g., Keller et al., 2001; Hickok and Poeppel, 2004).
Binder et al. (2009) reviewed neuroimaging studies (i.e., fMRI and PET studies) to identify brain regions that contribute to the semantic component of words and found that the left posterior parietal lobe, the lateral temporal cortex, and the inferior frontal gyrus demonstrated a high likelihood of activation across studies. Recent fMRI studies applied multi-voxel pattern analysis (MVPA) to investigate neural representations associated with semantics by analyzing patterns of neural activation (Mitchell et al., 2008; Shinkareva et al., 2011). This approach also enabled a comparison through which to investigate the common neural representations across people and languages (Zinszer et al., 2016; Yang et al., 2017a), especially in bilingual semantic processing. For example, the common neural representation of equivalent Portuguese and English nouns was found to be situated in the left post-central and supramarginal gyri (SMG), the left inferior and superior parietal lobes (I/SPL), the left inferior frontal gyrus (IFG), and the posterior superior temporal lobe (Buchweitz et al., 2012). Meanwhile Correia et al. (2014) argued that the shared representation across Dutch and English was located in the left anterior temporal lobe (ATL), the left angular gyrus (AG), the posterior bank of the left postcentral gyrus, the right posterior superior temporal sulcus/gyrus (STS/STG), the right anterior insula, the medial part of right ATL, and the bilateral occipital cortices. Van de Putte et al. (2017) investigated the common neural representation across French and Dutch and proved that the shared semantic representations are located in the bilateral occipito-temporal cortex and in the inferior and the middle temporal gyrus (ITG/MTG). Overall, the previous neuroimaging studies investigating the common neural representation of the semantic processing of words have yielded a consistent result, regardless of whether the participants were asked to read (Buchweitz et al., 2012), listen (Correia et al., 2014), or speak (Van de Putte et al., 2017) the words. Those studies suggested that a common neural representation might comprise a number of brain regions, including the left inferior parietal lobe (AG and portions of SMG) and the superior/middle temporal lobe. However, inconsistent results still existed, and this inconsistency might be resolved via using more natural processing, i.e., sentence processing.
In real life we communicate in written or spoken sentences formed of words that are arranged according to complicated syntactic rules (Ingram, 2007). Accordingly, the meanings conveyed by sentences transcend the individual words. However, the neural system underlying the semantic processing of sentences is still controversial. Price (2010) reviewed studies that have investigated the brain regions involved in the semantic processing of spoken sentences and argued that the neural system at the sentence level was situated in the anterior and posterior parts of the left middle temporal gyrus, the bilateral anterior temporal poles, the left AG, and the posterior cingulate/precuneus. These areas were associated with the semantic processing of words as reported by Binder et al. (2009). Interestingly, Jouen et al. (2015) identified a common neural system from processing whole sentences and images that describe human events that also includes the left AG. To our knowledge, however, only one MVPA study has argued the existence of commonalities in the neural system of bilinguals in the semantic processing of sentences across languages: Yang et al. (2017b) mapped the semantic properties of English words and their neural representations and subsequently developed a predictive model containing the neural system of sentences that were composed from these words. Although they demonstrated the above chance accuracy of predicting the activation pattern of Portuguese sentences from equivalent English sentences, the Portuguese–English bilinguals were presented with only their native Portuguese sentences. The direct prediction of simultaneous semantic processing between the two languages known by the bilinguals was not conducted. Also, as Yang et al. (2017b) have suggested, all the across-language neural decoding and prediction studies used stimuli that only encompassed a small semantic space. In other words, they used a limited number of concrete nouns to represent dwellings, tools, animals, or other objects. It is hence unknown whether the neural system of the vast semantic spaces across languages can be similarly predicted.
In order to achieve the understanding of the semantics of sentences precisely, the syntactic processing is necessary for dealing with fitnesses of different arguments of words and phrases (Bookheimer, 2002; Humphries et al., 2007). This syntactic processing is considered to be subserved by the pars opercularis (BA 44), a subpart of the left inferior frontal gyrus (IFG) (Newman et al., 2010; Friederici, 2011; Makuuchi and Friederici, 2013). Further, during the sentence comprehension, the syntactic information needs to be integrated with the semantic information (Marslen-Wilson and Tyler, 1980) that is subserved by the pars trianularis (BA 45) which is another subpart of the left IFG (Friederici, 2011). Thus, the integration processing of the syntactic and semantic information is assumed to be supported in the left IFG including BA 44 and BA 45 (Hagoort, 2005, 2014). However, some findings suggested that the region which supports the processing of the syntactic and semantic integration is located in the posterior temporal cortex (Friederici, 2011, 2012). Despite these controversies, we hypothesized that the common neural system of the semantic processing of sentences might comprise regions associated with the syntactic processing, which are located in the left IFG.
This study aimed to investigate the common neural system of the semantic processing of sentences across languages. Bilingual participants were asked to read both the Chinese and the equivalent Japanese sentences and to understand them. The cross-language classification was implemented. This application comprised training the support vector machine (SVM) classifier (Cortes and Vapnik, 1995) with sentences in one language and testing it with sentences in the other language and vice versa. Our analysis involved the training and testing of the SVM with sentences in one language. In addition, participants were presented with pictures that depicted the same kinds of human events as in the sentences. The participants thus performed the semantic processing of three different modalities: Chinese written sentences, Japanese written sentences, and pictures.
Materials and Methods
Participants
Thirty-two right-handed speakers of Chinese as their first language participated in the study. Behavioral results showed that the accuracy of three participants on either task condition was lower than the chance level (50%). Thus, these three participants were removed from both the behavioral and the fMRI analyses. The remaining 29 participants (6 males; mean age = 27.93, SD = 3.65) with normal or corrected to normal vision reported that they did not suffer from any neurological or psychiatric disorder. Each participant signed the informed consent format approved by the Ethical Committee of the Graduate School of Letters at Hokkaido University.
All the participants were late sequential bilinguals who began learning Japanese at the average age of 18.03 (SD = 3.26). They had been learning Japanese for 9.71 years (SD = 4.23) on average, and had been living in Japan for an average of 4.85 years (SD = 2.59). Except for one participant who had studied and lived in Japan for more than 10 years, all the other participants had passed the highest level of Japanese Language Proficiency Test. Twenty-two of the participants were enrolled in a graduate-level course, and one was registered in an undergraduate program at Hokkaido University. Seven of the participants were employed in occupations: of these, four were master’s degree holders, two had earned doctoral degree, and one passed the requirements for the bachelor’s degree.
The participants filled out a language self-rating questionnaire to help researchers ascertain their Japanese language proficiency. The questionnaire asked participants to award self-rating points on a scale of 1.0 (poor) to 7.0 (excellent). The questionnaire contained five questions each on listening, speaking, reading, and writing skills which were collected from the JLPT Can-do Self-Evaluation List created by the Japan Foundation and the Japan Educational Exchanges and Services1. Despite being late bilinguals, the participants rated themselves as being highly proficient in Japanese (M = 6.24, SD = 0.58).
Stimuli
The stimuli comprised 48 pairs of pictures, and Chinese and Japanese written sentences totaling 144 pairs. The pictures (adapted from Jouen et al., 2015) depicted events representing one or two persons (no negative emotional valence) performing a common daily activity (e.g., playing the piano, cooking, reading a book to a child, etc.) and were collected from the Getty photo database2. A pair of pictures either symbolized a sequence of coherent events (for example, the first picture showed a girl throwing a piece of rock and the second picture portrayed the girl playing hopscotch as shown in Figure 1) or incoherent events (e.g., the first displayed a girl and a woman mounting wallpaper, and the second portrayed the same two characters jumping up and down on a bed), and the paired pictures represented only either coherent or incoherent events. Both the Chinese and Japanese sentences were generated on the basis of the pictures: the sentences described the activities being performed by the people in the pictures. The Chinese sentences were first generated and subsequently translated into equivalent Japanese sentences. The validity of the Japanese translation was confirmed by consulting with a native Japanese speaking expert. As a result, each event pair conveying the same meanings was represented by three different modalities: the picture, the Chinese sentence, and the Japanese sentence.
Three stimuli sets (A, B, and C) were generated, each comprising 16 picture pairs, 16 Chinese sentence pairs, and 16 Japanese sentence pairs. To avoid the participants seeing a picture pair and receiving its corresponding Chinese or Japanese sentence pair in a single session, stimuli pairs were crossed. For example, the 16 picture pairs were classified into set A, and their corresponding 16 Chinese and Japanese sentence pairs were then respectively classified into sets B and C.
The stimuli were balanced for coherency (coherent or incoherent) and the number of individuals performing the activity (one or two), and both were counterbalanced across the three stimuli sets. The Chinese sentences obeyed the subject–verb–object order and the Japanese sentences obeyed the subject–object–verb order according to the grammar rules of the respective languages. The Chinese sentences had a mean length of 10.08 words (SD = 2.62) and the Japanese sentences had an average of 16.33 words (SD = 3.38).
Stimuli Evaluation
To assess the degree to which the sentences matched with the pictures, 20 pilot participants (10 Chinese natives and 10 Japanese natives) who did not participate in the fMRI experiment were recruited. These participants were presented with sentences in their respective native language and were asked to rate how appropriately the sentences were able to describe the activities being performed by the persons in the pictures on a scale of 1.0 (very poorly) to 7.0 (very well). The mean rating score was 6.51 (SD = 0.40) for the Chinese sentences and 6.69 (SD = 0.30) for the Japanese sentences. Both the Chinese and the Japanese sentences were thus rated as being excellently able to describe the activities in the pictures. In addition, the variety of activities in the pictures ensured that the Japanese used in the sentences would incorporate a vast semantic space. Insufficient knowledge of the used Japanese sentences would probably affect the semantic processing; therefore, the frequency of the use of the words of the Japanese sentences was evaluated. All the Japanese sentences were segmented into words (totally 179 words), and the incidence of the use of the particular words was investigated using the long-unit-word and the short-unit-word aspect of The Balanced Corpus of Contemporary Written Japanese (BCCWJ) (National Institute for Japanese Language and Linguistics, 2018). Except for the newly coined word “selfie,” the frequencies of the words used for the sentences ranked between extremely low and high levels of usage, extending from 0.16 to 48383.91 per million words.
Procedures
Before the fMRI scanning session, participants completed the vocabulary checking list that was generated according to word frequency. The list contained 30 words collected in the order of decreasing word frequency. The participants were asked to remember the meanings of the words that they did not know to avoid interference with sentence comprehension caused by not understanding the words that were used.
During the scanning, participants underwent three sessions (i.e., stimuli sets A, B, and C), and the order of the sessions was counterbalanced across participants. Each session included 48 trials, and all the trials were randomly presented. During a trial, a yellow fixation cross appeared on a black background for 1 s, then the first stimulus of the event pair appeared on a black background for 3 s, followed by another yellow fixation cross for 1 s before the second stimulus of the event pair was presented for 3 s. At the end of this sequence, an evaluation screen with a white fixation cross containing options on a black background was presented for 2 s plus jitter time (0, 2, 4, or 6 s) before the next trial (Figure 1). In the trials in which event pairs were presented either in Chinese or Japanese, participants were instructed to silently and consistently read the sentences until they disappeared. In the trials in which the event pairs were presented in pictures, participants were instructed to continue thinking about the activities that the persons in the pictures were performing. In each trial, participants were also asked to judge whether or not the event pair was coherent by pressing a response button with their right index or middle finger as soon as they could after the evaluation screen appeared. The evaluation screen did not disappear after they had pressed this button. The judging options were arranged in accordance with the participants’ fingers: the alternative presented in the left bottom corner corresponded to the index finger and the selection appearing in the right bottom corner corresponded to the middle finger. The locations of the options were counterbalanced across the stimuli.
fMRI Acquisition
Functional and structural image acquisition was performed on a Siemens Prisma 3.0 T scanner using a 64-channel head coil at the Research and Education Center for Brain Science of Hokkaido University. The whole brain functional images were collected using a T2∗-weighted gradient EPI sequence (TR = 2000 ms, TE = 30 ms, voxel size = 2 × 2 × 3.5 mm, and a 90° flip angle). A session consisted of 318 volumes. The high-resolution structural images covering the whole brain were acquired after the functional image acquisition using a T1 MPRAGE sequence (TR = 2300 ms, TE = 2.41 ms, TI = 900 ms, FOV = 256 × 256 mm, and an 8° flip angle).
fMRI Data Preprocessing
fMRI data processing and analysis were performed with SPM 12 (Wellcome Department of Cognitive Neurology, London, United Kingdom) in the Matlab environment. The first three scans of all the sessions were removed from the analysis to minimize T1 artifacts. The functional images were corrected for slice timing and were spatially realigned to normalize to the Montreal Neurological Institute (MNI) space without changing the voxel size. Spatial smoothing was applied using a Gaussian kernel of 6 × 6 × 6 mm full width at half-maximum for univariate analysis. To prevent the possibility of less predictive individual voxels, spatially normalized but unsmoothed images were used to perform MVPA.
fMRI Data Analysis: Univariate Analysis
To reveal neural regions generally involved in the semantic processing of Chinese sentences, Japanese sentences, and pictures, the general linear model (GLM) (Friston et al., 1995) was used to obtain contrasts between each modality and the baseline. The three different modalities were modeled as three separate regressors and were convolved with a canonical hemodynamic response function for each participant. The group analysis was then processed through a second-level random effects model by using a one-sample t-test in a group analysis of all the participants. The activated regions were extracted with a cluster-level (k ≥ 15) threshold of p < 0.05 corrected for family-wise error (FWE).
fMRI Data Analysis: Searchlight MVPA
A searchlight method (Kriegeskorte et al., 2006) with a linear SVM classifier, as implemented by LIBSVM (Chang and Lin, 2011), was performed to investigate the common semantic neural system across languages and modalities in the processing of sentence comprehension. A spherical searchlight with a radius of 9 mm was used to reveal multiple patterns that carried featured neural representations of the sentence semantics.
The classifier for the classification analysis was trained to discriminate between the neural patterns associated with the coherent and incoherent events. Two types of classification analysis were performed. First, a within-language/modality classification was accomplished with (1) only Chinese sentence trials, (2) only Japanese sentence trials, and (3) only picture trials. In all the within-language/modality classifications, a leave-one-out procedure was used: the classifier was trained on the data from any two of the three sessions and was tested on the data from the one session left. The classification was repeated thrice by interchanging the training and testing data. The accuracies were averaged across the three iterations.
The second cross-classification was accomplished across languages/modalities classification with (4) Chinese vs. Japanese sentences, (5) Chinese sentence vs. picture, and (6) Japanese sentence vs. picture. The classifier was trained with data from one language/modality condition belonging to all the three sessions. It was tested on the respective data obtained from the remaining language/modality condition belonging to all the three sessions. Each classification was repeated twice in a manner ensuring that all of the specific language/modality data were used once for the test. The resulting accuracies were averaged across classification directions.
To construct accuracy group maps for across-language/modality, the accuracies were averaged across all participants and contrasted with the average accuracy of the coherency (accuracy at chance = 50%) using a one-sample t-test to reveal the cluster level (k ≥ 10) significant classification of sentence semantics across languages/modalities (p < 0.05, FWE-corrected). Group maps were also produced for the within-language/modality (p < 0.05, FWE-corrected, k ≥ 10).
To reveal a more robust result for the searchlight MVPA analysis, statistical maps were corrected using threshold free cluster enhancement (TFCE; Smith and Nichols, 2009) as implemented in a free MatlabTFCE package3 which combined a maximal permuted statistic correction technique (Nichols and Holmes, 2001). Ten thousand permutations and a one-tailed corrected cluster threshold of p = 0.05 were used (Wurm and Caramazza, 2019).
fMRI Data Analysis: Region of Interest (ROI) Analysis
An ROI analysis was further performed to specifically investigate the effects of regions that are commonly activated during sentence processing. ROIs were selected based on Jouen et al. (2015) study, which investigated the common neural representations for the semantic processing of sentences in monolinguals by performing conjunction analysis between written sentences and pictures. Regions such as BA 22 (superior temporal gyrus), BA 39 (AG, inferior parietal lobe), and BA 45 (triangular part of inferior frontal gyrus) were selected on the basis of their reporting of these areas being involved in the semantic processing of sentences. Two further regions were selected: BA 40 (supramarginal gyrus), which is adjacent to BA 39, and BA 44 (the opercular part of the inferior frontal gyrus), which is adjacent to BA 45. These regions are considered to be part of the classical language region and are known as Wernicke’s area and Broca’s area, respectively (Catani et al., 2005; Hagoort, 2017). To assure that the sentences were processed as semantic stimuli rather than visual stimuli, BA 17 (primary visual cortex) was also selected, and it was proved to be involved in visual information processing as the control region.
Results
Behavioral Results
During the fMRI scanning, the participants performed a coherence judging task. In all the conditions involving the Chinese sentence, the Japanese sentence and the picture, they evaluated the coherence with a high accuracy (Chinese sentence: M = 92%, SEM = 0.01; Japanese sentence: M = 92%, SEM = 0.01; picture: M = 93%, SEM = 0.01; Figure 2A). No significant differences were found on the accuracy [F(2,56) = 0.56, p = 0.58, = 0.02].
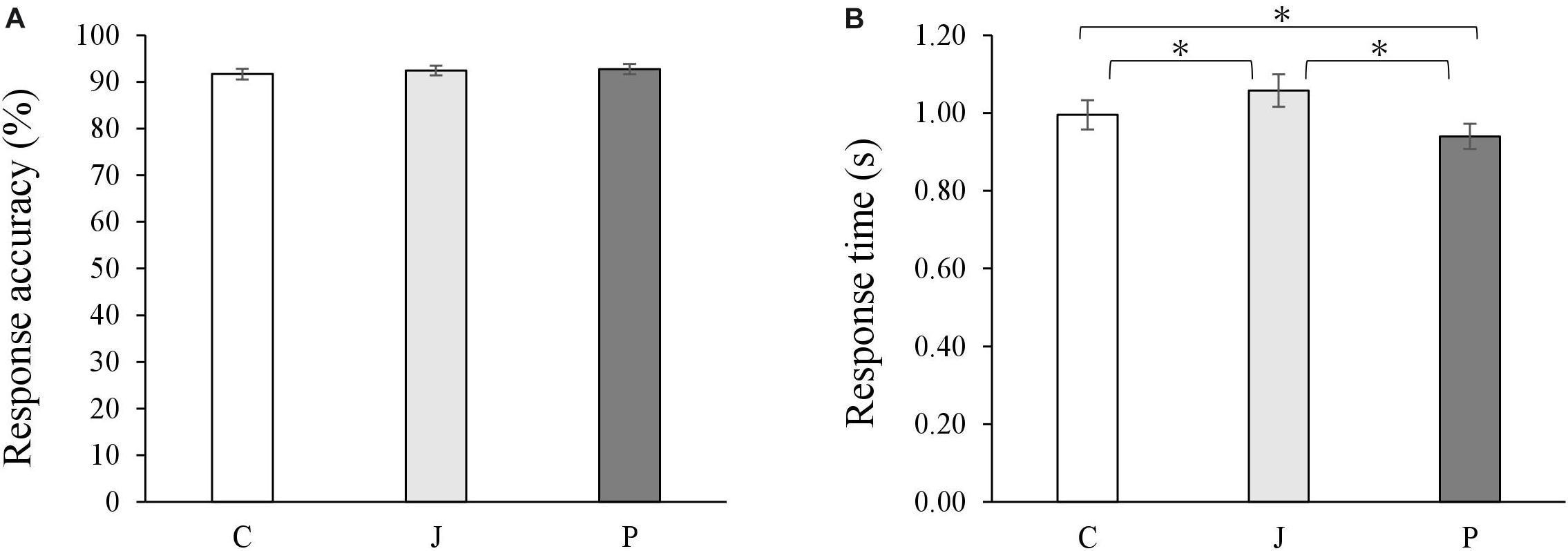
Figure 2. Average accuracies (A) and response time (B) on judging coherence of stimuli of all conditions. Bars represent means with standard errors. C represents the Chinese sentence condition, J represents the Japanese sentence condition, and P represents the picture condition (∗p < 0.05).
A significant difference was found pertaining to the condition on the response time [F(2,56) = 26.95, p < 0.001, = 0.49]. Participants responded faster to the picture (M = 0.94s, SEM = 0.03) than to the Chinese sentence (M = 1.00s, SEM = 0.04) and to the Japanese sentence (M = 1.06s, SEM = 0.04; Figure 2B). In a supplementary analysis, differences in the accuracy and the response time of the coherence judgment for all the conditions were analyzed (see Supplementary Material).
Univariate Analysis
We accomplished a voxel-based analysis of the whole brain activation to reveal activated neural regions for semantic processing of the Chinese sentence, the Japanese sentence, and the picture (p < 0.05, FWE-corrected, ke ≥ 15).
For the semantic processing of the Chinese sentence, a large predominantly left-hemisphere network was activated (Figure 3 and Table 1A). These regions included clusters spreading from the precentral gyrus (BA 6) and the supplementary motor area (SMA; BA 6) to the opercular part of the inferior frontal gyrus (Oper-IFG; BA 44); from the lateral inferior occipital gyrus (IOG; BA 18) to the fusiform gyrus (BA 37); and from the middle temporal gyrus (MTG; BA 21/22) to the superior parietal gyrus (SPG; BA 7) in the left hemisphere. The clusters that spread from the fusiform gyrus (BA 37) to the lingual gyrus (BA 18) and calcarine cortex (BA 17), and areas which included the MTG (BA 20) and the triangular part of the inferior frontal gyrus (Tri-IFG) in the right hemispheres were also found.
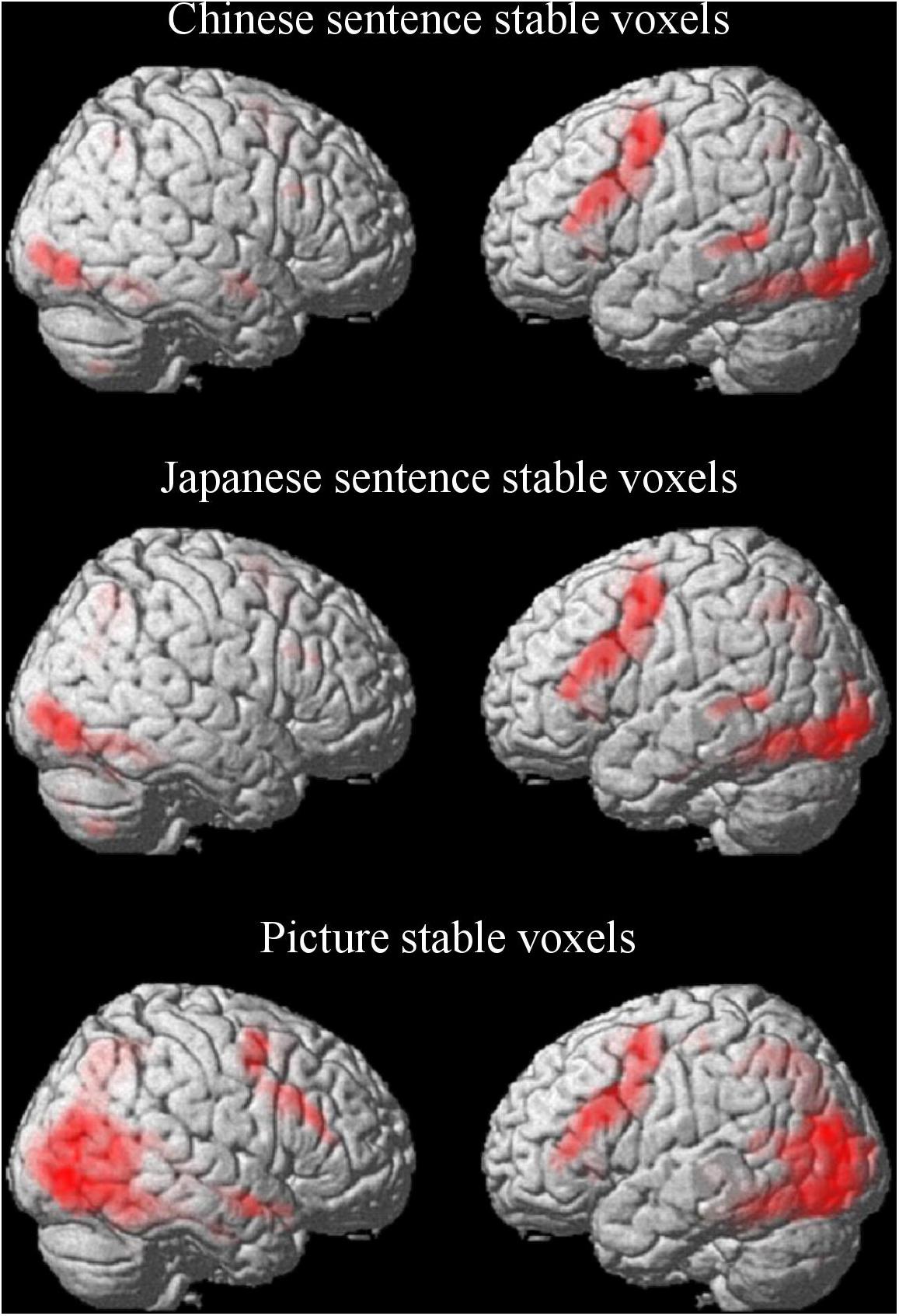
Figure 3. Stable voxels for the Chinese sentence, Japanese sentence and picture in semantic processing. Stable voxel clusters significant at p < 0.05, FWE-corrected, extent threshold = 15 voxels.
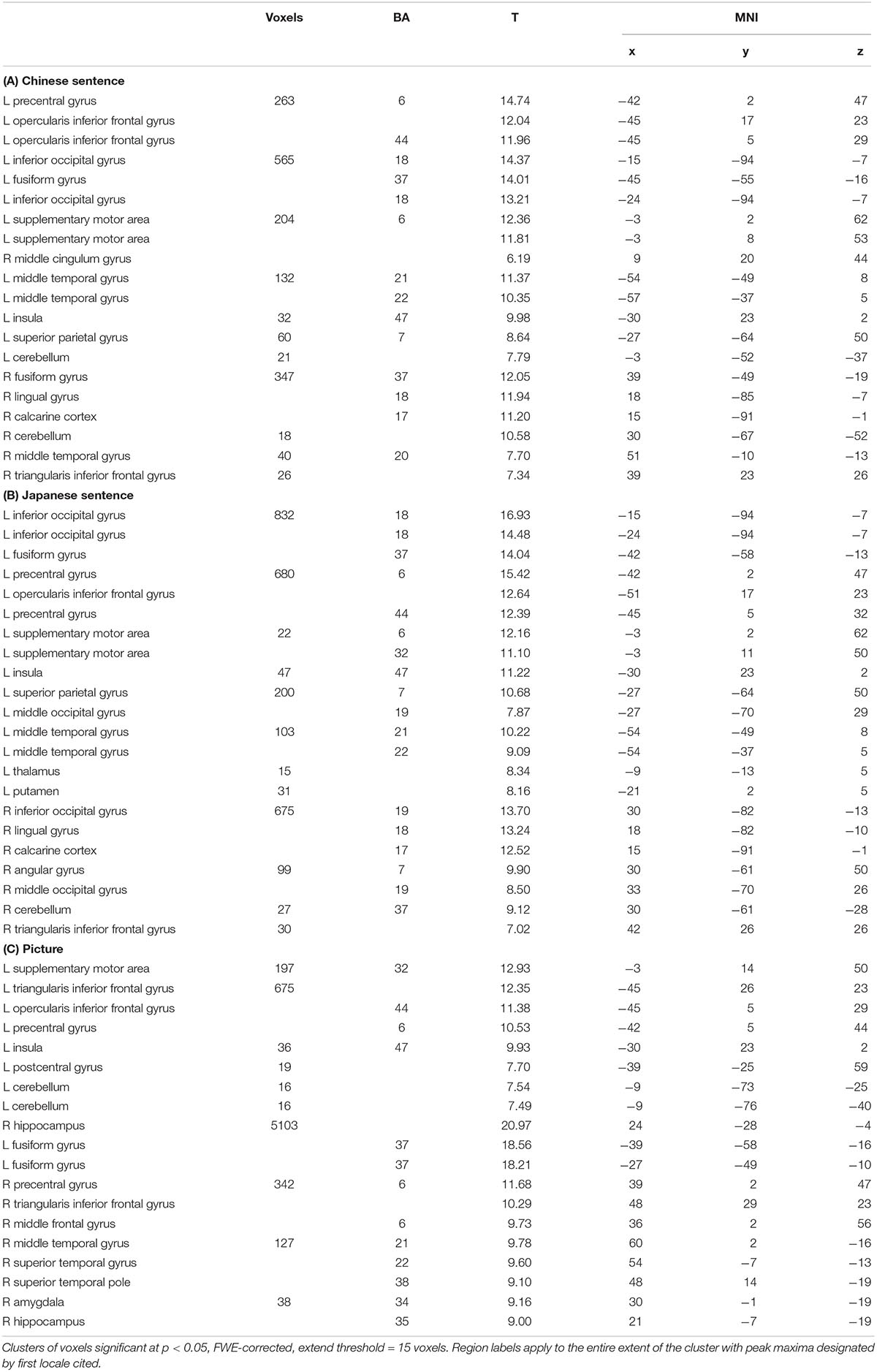
Table 1. Activation for Chinese sentence (A), Japanese sentence (B) and picture (C) semantic processing.
Similarly, for the semantic processing of the Japanese sentence, the left-hemisphere regions were predominantly activated and partially overlapping with the semantic processing of the Chinese sentence (Figure 3 and Table 1B). Clusters in the left hemisphere extended from the IOG (BA 18) to the fusiform gyrus (BA 37), from the precentral gyrus (BA 6) and the SMA (BA 6) to the Oper-IFG (BA 44), from the SPG (BA 7) to the MOG (BA 19), and the MTG (BA 21/22). In the right hemisphere, the clusters encompassed were the IOG (BA 19) to the lingual gyrus (BA 18) and the calcarine cortex (BA 17), as well as the AG (BA 7) to the MOG (BA 19), and the Tri-IFG.
In opposition to the Chinese and Japanese sentence processing, the semantic processing of the picture activated regions more bilaterally (Figure 3 and Table 1C). Except for the cluster spreading from the superior temporal pole (BA 38) via the superior temporal gyrus (STG; BA 22) to the MTG (BA 21) in the right hemisphere, the regions that were activated both in the left and right hemispheres were symmetrical to some degree. The first two regions were the cluster extended from the internal SMA (BA 32) and the precentral gyrus (BA 6) to the Tri-IFG (BA 44) in the left hemisphere, and the cluster extended from the precentral gyrus (BA 6) and the middle frontal gyrus (MFG; BA 6) to the Tri-IFG in the right hemisphere. The other two regions were the broad areas located in the occipital lobe spreading from the left fusiform gyrus to the right hippocampus as the peak locations.
Furthermore, we observed the greater activity in the left inferior parietal gyrus (BA 40), the right supramarginal gyrus, and middle occipital gyrus for coherent compared with incoherent semantic processing (details are provided in the Supplementary Material).
Searchlight MVPA Analysis
Within-Language/Modality Classification
Figure 4A and Table 2A exhibit the areas in which the significant classification accuracies were found for the Chinese sentence. These regions were located in the left AG (BA 39) and extended to the MOG (BA 19). No significant classification accuracies were found within the Japanese sentence.
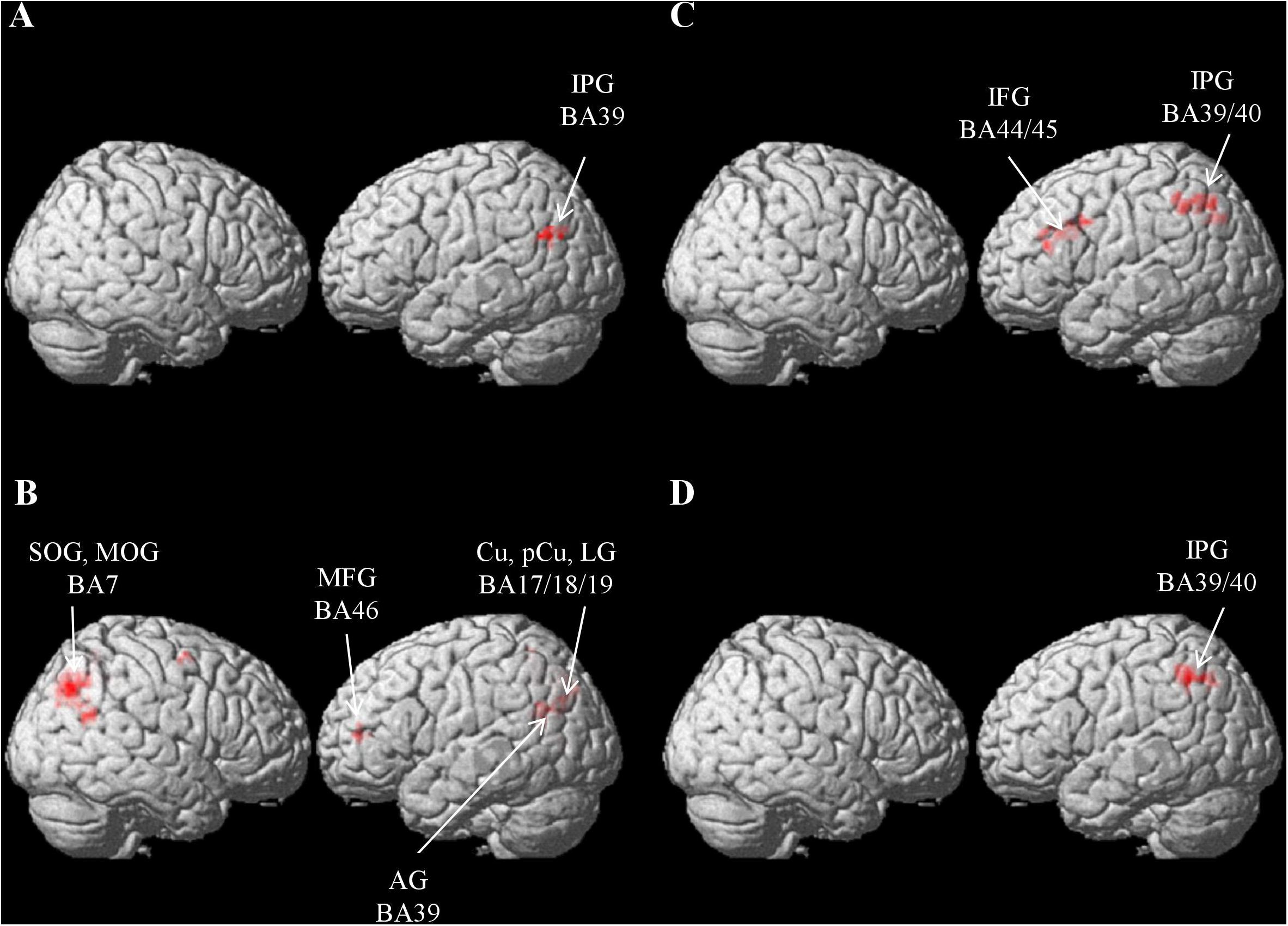
Figure 4. Results of the searchlight analysis, showing significant classification accuracies above chance level (50%) from averaged whole-brain maps from all the participants at a cluster level FWE corrected at p < 0.05. (A) Results for within-Chinese classification. (B) Results for within-picture classification. (C) Results for across-language classification. (D) Results for across-modality classification.
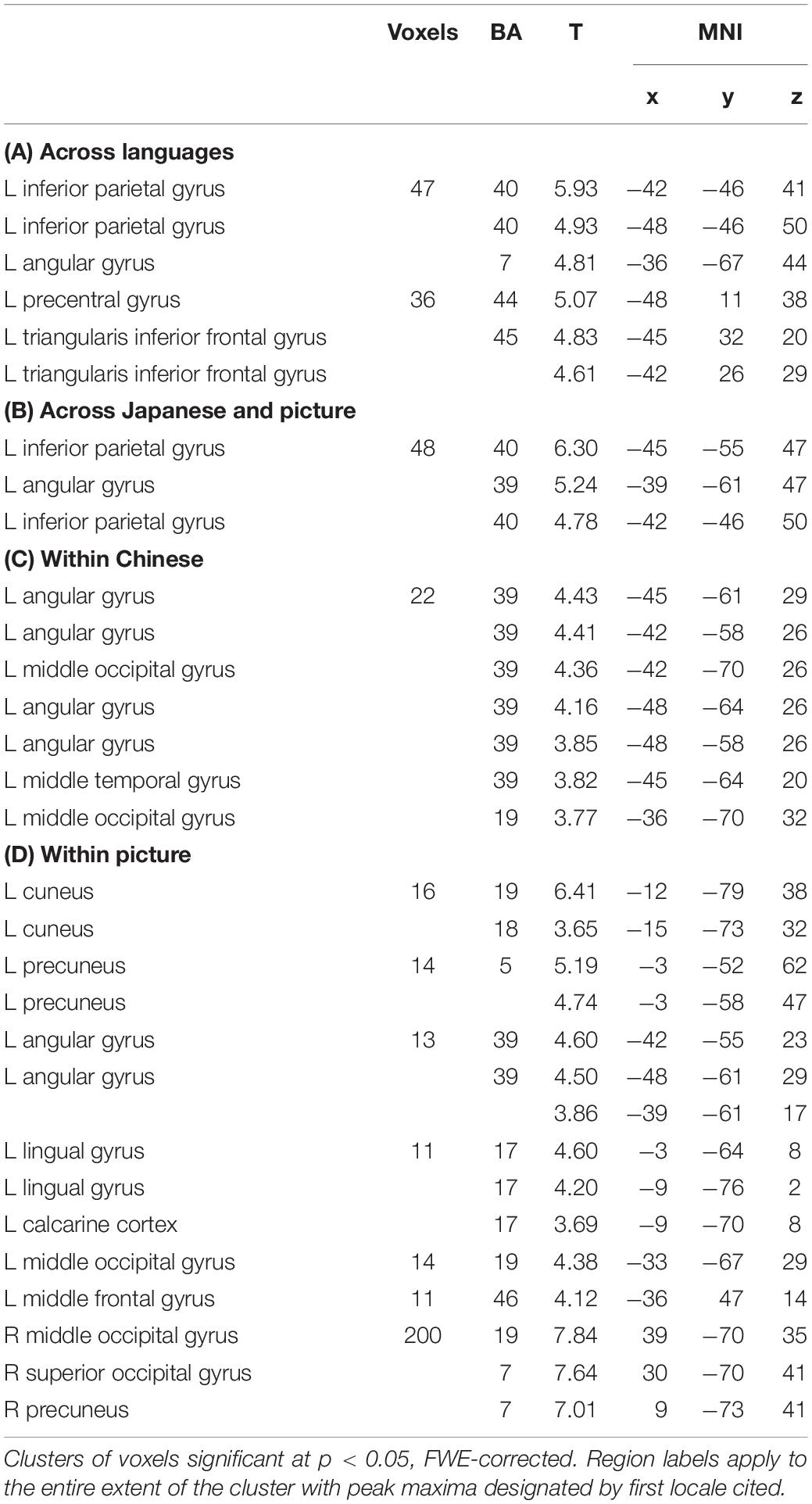
Table 2. Brain areas showed significant across-language (A), across-modality (B), within-Chinese (C) and within-picture (D) classification accuracies.
Areas involved in the classification of the pictures were more bilateral (Figure 4B and Table 2B) and included the left parieto-occipital regions spreading from the AG (BA 39) to the cuneus (BA 18/19), precuneus (BA 5), the lingual gyrus (BA 17/19), and the left MFG (BA46). The right MOG extending to the superior occipital gyrus (SOG; BA 7) and the precuneus (BA 7) were also noted.
Across-Language/Modality Classification
Significant across-language (i.e., Chinese sentence vs. Japanese sentence) classification accuracies were found in the left inferior parietal gyrus (IPG), which extends from the supramarginal gyrus (SMG; BA 40) to the AG (BA 39/7), and in the left precentral gyrus extending to the Oper and Tri-IFG (BA 44/45; Figure 4C and Table 2C).
Significant across-modality (i.e., Japanese sentence vs. picture) classification accuracy involved the left IPG extending from the SMG (BA 40) to the AG (BA 39/7) (Figure 4D and Table 2D). No significant classification accuracy was found between the Chinese sentence and the picture.
Results of using the TFCE also showed significant above chance classification accuracies for within- and across-language/modality classification. In the within-picture classification, significant classification accuracies were found in bilateral parieto-occipital regions (Supplementary Figure 4A). In the across-language classification, significant classification accuracies were found in the left IPG and the left IFG (Supplementary Figure 4B), though the regions significantly activated were smaller than those obtained using the searchlight MVPA. In contrast, significant classification accuracies were not observed for the within-Chinese sentences and the Japanese vs. picture classifications.
ROI Analysis
The mean classification accuracies in each ROI were contrasted with the chance level of accuracy (50%) using a one-sample t-test to accomplish the ROI analysis. For the within-Chinese sentence, the left BA 22 and BA 45 showed significant classification accuracies. For the within-Japanese sentence, the left BA 40, right BA 22, and BA 39 revealed significant classification accuracies. For the within-picture, the significant classification accuracies were shown in the bilateral BA 22, BA 39, and BA 40, the right BA 39, and the left BA 17, which was involved in primary visual information processing.
For the across-language classification, significant classification accuracies were shown in the bilateral BA 44, the left BA 39, BA 40, and BA 45. Across modalities (i.e., Japanese sentence vs. picture), significant accuracies were shown in the left BA 39 and BA 40, and in the right BA 45. In contrast to the searchlight analysis, the ROI analysis revealed significant cross-modality classification accuracies for the Chinese sentence vs. the picture in the bilateral BA 39 and BA 40 (Figure 5).
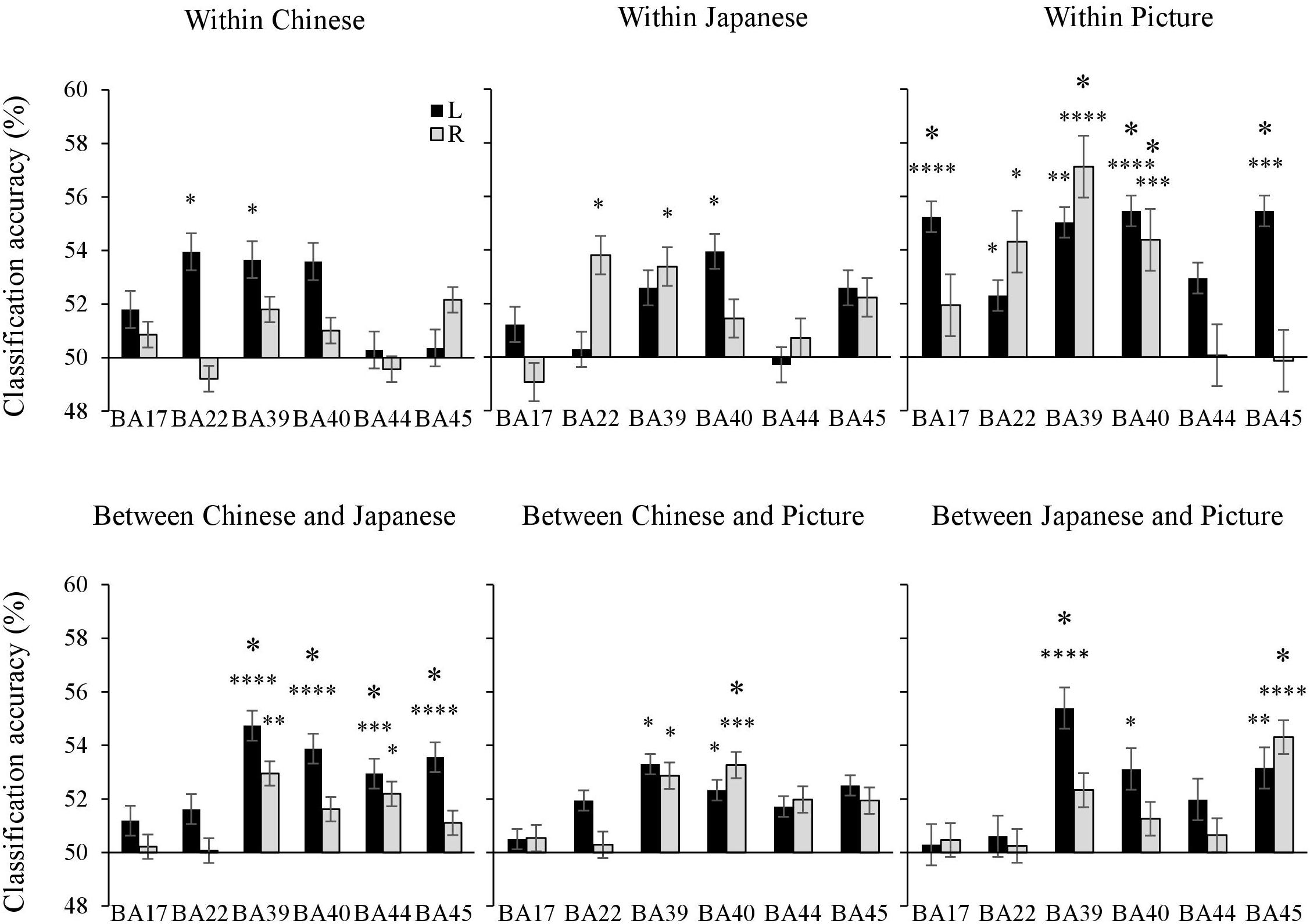
Figure 5. The mean classification accuracies in each ROI were contrasted with the chance level of accuracy (50%) using a one-sample t-test. Bars represent standard errors. The small and large asterisks indicate statistical significance of p values uncorrected and corrected for multiple comparisons using Holm-Bonferroni procedure. ∗p < 0.05, ∗∗p < 0.01, ∗∗∗p < 0.001, ∗∗∗∗p < 0.0001.
Discussion
The present study used MVPA to investigate the common neural system of the semantic processing during sentence comprehension across languages in bilinguals. The significant classification accuracies indicate the existence of a common neural semantic representation in the higher language processing level. More specifically, the common neural representation was found to be situated in the left inferior parietal gyrus extending from the angular gyrus to the supramarginal gyrus, and the opercular and triangular part of the left inferior frontal gyrus. The results of this study also suggest that the left inferior parietal gyrus, in particular, the left angular gyrus and supramarginal gyrus, is pivotal to the processing of semantics regardless of the modality.
Across Languages
The univariate analysis showed similar brain activation associated with the processing of the same sentence semantics for both Chinese and Japanese. This suggests that a common neural representation may exist across languages but could not allow the identification of the exact regions for which the MVPA was conducted.
Despite the inconsistent results revealed by previous studies investigating the common neural representation of word semantic processing across languages using the MVPA approach (Buchweitz et al., 2012; Correia et al., 2014; Van de Putte et al., 2017), it is possible to assume that the left temporoparietal conjunction regions are highly involved in the semantic processing of languages. Indeed, these regions were active when subjects were exposed to sentences or pictures depicting human events (Jouen et al., 2015). The present study observed significant classification accuracies in the left IPG, i.e., the AG (BA 39) and the SMG (BA 40), across languages for the semantic processing of sentences. Accordingly, the role of the left AG and SMG in the semantic processing of languages is suggested regardless of the processing level (word or sentence).
The semantics of languages are conveyed by symbols such as characters and/or sounds, which can be combined into words and/or sentences. Though the processes are divergent in the initial stages, the visual and auditory information must be mapped onto meanings to understand a sentence (Humphries et al., 2007). Thus, the neural pathways underpinning the visual and auditory semantic processing converge in the left AG (Bemis and Pylkkänen, 2013) for comprehension. Further, a series of further semantic processes are executed in the left AG to understand exactly the meanings conveyed by the words, especially by the sentences (Humphries et al., 2007). The most crucial process is adjusting the input information by verifying the already-existing knowledge. This manipulation may be executed in the left AG through retrieving the knowledge of the objects and events where it stored (Binder et al., 2009; Binder and Desai, 2011; Noonan et al., 2013). Then, the word semantic judging (Bonner et al., 2013) and naming and reading of the word (Seghier et al., 2010) could be executed. Constructing the meanings of the individual words (Price et al., 2016), the sentence could be comprehended (Pallier et al., 2011). Our findings reconfirm the established importance of the left AG in semantic processing, and implicate that the left AG is a critical region for semantic processing of languages transcending different languages and the processing levels. Meanwhile, the semantics conveyed by the sentences presented in the study concerned events in which entities interacted in space and time. Therefore, it also suggests that the left AG is undoubtedly necessary in the processing of event-related semantics (Binder and Desai, 2011; Seghier, 2013; Jouen et al., 2015; Baldassano et al., 2017).
The SMG, another part of the left IPG that is anterior to the AG, was also observed in significant classification accuracy across languages. This region is traditionally considered to underlie phonological processing such as phonological recognition, phonological control, and production (Booth et al., 2004; Prabhakaran et al., 2006). The SMG probably contributes preferentially to phonological aspects during word recognition. On the other hand, it is also reported to be critical for semantic processing (Stoeckel et al., 2009), especially in reading, which requires the recognition of visual stimuli and their linking to meanings (Sliwinska et al., 2012) as accomplished in our study. Further, the posterior part of the SMG is suggested to be the area where lexical and sublexical cues are integrated (Oberhuber et al., 2016), the lexical phonological retrieval is controlled and from the orthography to phonology is mapped (Price, 2018), and the meanings processed in the AG are bound to recognize the word (Lee et al., 2007). In the semantic processing of the sentence where words were formed, it is possible to assume that the continuous linking of lexical cues and meanings is required. Overall, as our study has indicated, the cooperation of both the left AG and SMG in the semantic processing of the sentence is demanded.
Another significant classification accuracy across languages was observed in divergence with previous MVPA studies of the semantic processing of words in the left inferior frontal gyrus, i.e., Oper-IFG (BA 44) and Tri-IFG (BA 45) which constitute Broca’s area, a classical language processing area. Broca’s area might underlie not only the language production but also various other language functions (Geschwind, 1970; Kim et al., 1997), one of them being the executive control of language. Apart from the comparatively simple processing such as the semantic processing of a sentence, the participants of this study were required to maintain the meanings of the first stimulus of the stimuli pair while processing the second stimulus to complete the evaluation task. Further, processes such as lexical retrieval and matching with previously held knowledge were needed to be executed simultaneously to accomplish the exact comprehension of the meanings. All the execution of these above processes is considered to be mandated by Broca’s area (Devlin et al., 2003; Whitney et al., 2011; Noonan et al., 2013; Ralph et al., 2017).
Another role of Broca’s area is the syntactic processing of language (Caplan, 2006; Grodzinsky and Santi, 2008; Friederici, 2011); BA 44 is especially considered to be the core region of the syntactic processing (Friederici, 2011, 2012) that provides strong cues for determining meanings (Humphries et al., 2007). As hypothesized, this syntactic processing-associated region was observed in the study. This may explain the discrepancy from the previous MVPA studies and indicate that the syntactic processing is critical for the sentence comprehension. Toward the determination of meanings of sentences, the syntactic information decoded by analyzing the constructions of the words and phrases which form the sentences will be mapped with the semantic information (Bookheimer, 2002; Friederici, 2011). Because of the preferential contribution of BA 44 to the syntactic processing, BA 44 is necessary to interact with the region that subserves the semantic processing, which is considered to be BA 45 (Friederici, 2011) to determine meanings. Based on the results of the present study, it is possible to assume that syntactic and semantic integration occur in Broca’s area as Hagoort (2005, 2014) suggested. In this processing, the syntactic working memory is also required, which is considered to be one role of Broca’s area (Fiebach et al., 2005; Makuuchi and Friederici, 2013). In the higher level of the semantic processing of language performed in our study, besides the semantic processing, sentence comprehension also demands both the executive control and the syntactic processing. Therefore, the involvement of Broca’s area is presumable.
Broca’s area presented more superior extension to the ventral part of the precentral gyrus (BA 6) in the present study. As Hagoort (2005, 2014) suggested, it is more appropriate to refer to the BA 44, BA 45, BA 47, and BA 6 of the left inferior frontal cortex as Broca’s area, because adjacent areas such as BA 47 and BA 6 are also involved in language processing. This finding of the present study is greatly consistent with Hagoort’s assumption. Likewise, this finding might indicate the relevance of the motor-related system (i.e., BA 6) during comprehension of action-related sentences (e.g., Hauk et al., 2004; Hauk and Pulvermüller, 2011; Jouen et al., 2015), and the activation of acoustic representations during speech comprehension (Hickok and Poeppel, 2004, 2007; Cheung et al., 2016). Meanwhile, Broca’s area is connected via the superior longitudinal fasciculus with the left AG and SMG. Hence, it makes sense that the robust neural representation associated with the higher level of semantic processing is situated in the left inferior parietal gyrus (i.e., the AG and the SMG) and the left inferior frontal gyrus (BA 44 and 45) (Horwitz et al., 1998; Frey et al., 2008; Kelly et al., 2010).
Across Modalities
A significant classification accuracy was observed in the left IPG (i.e., the AG and SMG) for semantic processing across modalities (i.e., Japanese sentence vs. picture and Chinese sentence vs. Japanese sentence). This result revealed the modality-independent common neural representation. The univariate analysis of the coherence judgment (see Supplementary Material) also showed the involvement of the left SMG for coherent semantic processing regardless of the modalities. These findings tend to support the idea proposed by Damasio (1989) and Meyer and Damasio (2009) that there are convergence zones where the features associated with different objects and events and/or information conveyed by different sensory systems are bound. The features and/or information were considered to be the processing of the meanings of the features and/or the information (Mahon and Caramazza, 2008). Despite the fact that the neural basis of the convergence zone is still controversial, the association of the temporoparietal regions overlapped to some degree (Jefferies, 2013; Simanova et al., 2014; Jouen et al., 2015; Wurm and Caramazza, 2019). Specifically, it is posited that the inferior parietal lobe, the ventral and lateral temporal lobes are involved in the higher-level convergence processing where the binding representation from multiple modalities encode an abstract or schematic concept (Binder and Desai, 2011; Simanova et al., 2014). Sentence comprehension requiring fluent conceptual combinations as in the present study demands the higher-level convergence processing of complex information. The information from the languages and modalities needs to be integrated with the stored knowledge in the convergence zone, which is the left IPG (Lau et al., 2008; Binder et al., 2009; Binder and Desai, 2011; Bonner et al., 2013; Seghier, 2013). Though the searchlight analysis evidenced the absence of significant classification accuracy between the Chinese sentence and the picture, the ROI analysis showed significant classification accuracy in the left BA 39 and BA 40. Hence, the results of the present investigation make it possible to indicate that the left inferior parietal gyrus (BA 39 and 40) is a modality-independent convergence zone for higher semantic processing.
Conclusion
This study aimed to investigate whether an across-language sentence comprehension system exists using MVPA with Chinese–Japanese bilinguals, and whether such a system shares a common foundation for the broader comprehension of meaning in images. The results first suggest that the existence of the common neural system across languages in the semantic processing of sentences is located in the left inferior parietal gyrus (BA 39 and BA 40) and in the left inferior frontal gyrus (BA 44 and BA 45), which is also known as Broca’s area. Second, the findings elucidate the specific functioning of the left inferior parietal gyrus as a modality-independent convergence zone, particularly in higher semantic processing as required for understanding sentences and images.
Data Availability Statement
The datasets generated for this study are available on request to the corresponding author.
Ethics Statement
This study was carried out in accordance with the recommendations of the Hokkaido University Institutional Review Board with written informed consent from all subjects. All subjects provided written informed consent in accordance with the Declaration of Helsinki. The protocol was approved by the Hokkaido University Institutional Review Board.
Author Contributions
ZH and KO conceived and designed the experiment. ZH performed the experiment, analyzed the data, and drafted the manuscript. HY and SN coordinated the data analysis. YY assisted in performing the experiment and collected the data. CM-L, JV-D, and PD contributed to conceptualization of the experiment and provided the material. PD reviewed the manuscript. KO supervised the experiment and the data analysis, reviewed and revised the manuscript.
Funding
This work was supported by Graduate Grant Program of the Graduate School of Letters, Hokkaido University, Japan.
Conflict of Interest
The authors declare that the research was conducted in the absence of any commercial or financial relationships that could be construed as a potential conflict of interest.
Supplementary Material
The Supplementary Material for this article can be found online at: https://www.frontiersin.org/articles/10.3389/fnhum.2019.00380/full#supplementary-material
Footnotes
- ^ http://www.jlpt.jp/about/candolist.html
- ^ https://www.gettyimages.fr/
- ^ https://github.com/markallenthornton/MatlabTFCE
References
Baldassano, C., Chen, J., Zadbood, A., Pillow, J. W., Hasson, U., and Norman, K. A. (2017). Discovering event structure in continuous narrative perception and memory. Neuron 95, 709–721. doi: 10.1016/j.neuron.2017.06.041
Bemis, D. K., and Pylkkänen, L. (2013). Basic linguistic composition recruits the left anterior temporal lobe and left angular gyrus during both listening and reading. Cereb. Cortex 23, 1859–1873. doi: 10.1093/cercor/bhs170
Binder, J. R., and Desai, R. H. (2011). The neurobiology of semantic memory. Trends Cogn. Sci. 15, 527–536. doi: 10.1016/j.tics.2011.10.001
Binder, J. R., Desai, R. H., Graves, W. W., and Conant, L. L. (2009). Where is the semantic system? A critical review and meta-analysis of 120 functional neuroimaging studies. Cereb. Cortex 19, 2767–2796. doi: 10.1093/cercor/bhp055
Bonner, M. F., Peelle, J. E., Cook, P. A., and Grossman, M. (2013). Heteromodal conceptual processing in the angular gyrus. Neuroimage 71, 175–186. doi: 10.1016/j.neuroimage.2013.01.006
Bookheimer, S. (2002). Functional MRI of language: new approaches to understanding the cortical organization of semantic processing. Annu. Rev. Neurosci. 25, 151–188. doi: 10.1146/annurev.neuro.25.112701.142946
Booth, J. R., Burman, D. D., Meyer, J. R., Gitelman, D. R., Parrish, T. B., and Mesulam, M. M. (2004). Development of brain mechanisms for processing orthographic and phonologic representations. J. Cogn. Neurosci. 16, 1234–1249. doi: 10.1162/0898929041920496
Buchweitz, A., Mason, R. A., Hasegawa, M., and Just, M. A. (2009). Japanese and English sentence reading comprehension and writing systems: an fMRI study of first and second language effects on brain activation. Biling Lang. Cogn. 12, 141–151. doi: 10.1017/S1366728908003970
Buchweitz, A., Shinkareva, S. V., Mason, R. A., Mitchell, T. M., and Just, M. A. (2012). Identifying bilingual semantic neural representations across languages. Brain Lang. 120, 282–289. doi: 10.1016/j.bandl.2011.09.003
Caplan, D. (2006). Why is broca’s area involved in syntax. Cortex 42, 469–471. doi: 10.1016/S0010-9452(08)70379-4
Catani, M., Jones, D. K., and Ffytche, D. H. (2005). Perisylvian language networks of the human brain. Ann. Neurol. 57, 8–16. doi: 10.1002/ana.20319
Chang, C. C., and Lin, C. J. (2011). LIBSVM: a library for support vector machines. ACM Trans. Intell. Syst. Technol. 2, 27:1–27:27. doi: 10.1145/1961189.1961199
Cheung, C., Hamiton, L. S., Johnson, K., and Chang, E. F. (2016). The auditory representation of speech sounds in human motor cortex. eLife 5:e12577. doi: 10.7554/eLife.12577.001
Correia, J., Formisano, E., Valente, G., Hausfeld, L., Jansma, B., and Bonte, M. (2014). Brain-based translation: fMRI decoding of spoken words in bilinguals reveals language-independent semantic representations in anterior temporal lobe. J. Neurosci. 34, 332–338. doi: 10.1523/JNEUROSCI.1302-13.2014
Cortes, C., and Vapnik, V. (1995). Support-vector networks. Mach. Learn. 20, 273–297. doi: 10.1007/BF00994018
Damasio, A. R. (1989). Time-locked multiregional retroactivation: a systems-level proposal for the neural substrates of recall and recognition. Cognition 33, 25–62. doi: 10.1016/0010-0277(89)90005-X
Devlin, J. T., Matthews, P. M., and Rushworth, M. F. S. (2003). Semantic processing in the left inferior prefrontal cortex: a combined functional magnetic resonance imaging and transcranial magnetic stimulation study. J. Cogn. Neurosci. 15, 71–84. doi: 10.1162/089892903321107837
Fiebach, C. J., Schlesewsky, M., Lohmann, G., Von Cramon, D. Y., and Friederici, A. D. (2005). Revisiting the role of Broca’s area in sentence processing: syntactic integration versus syntactic working memory. Hum. Brain Mapp. 24, 79–91. doi: 10.1002/hbm.20070
Frey, S., Campbell, J. S. W., Pike, G. B., and Petrides, M. (2008). Dissociating the human language pathways with high angular resolution diffusion fiber tractography. J. Neurosci. 28, 11435–11444. doi: 10.1523/JNEUROSCI.2388-08.2008
Friederici, A. D. (2011). The brain basis of language processing: from structure to function. Psychol. Rev. 91, 1357–1392. doi: 10.1152/physrev.00006.2011
Friederici, A. D. (2012). The cortical language circuit: from auditory perception to sentence comprehension. Trends Cogn. Sci. 16, 262–268. doi: 10.1016/j.tics.2012.04.001
Friston, K. J., Holmes, A. P., Worsley, K. J., Poline, J. B., Frith, C. D., Frackowiak, R. S. J., et al. (1995). Statistical parametric maps in functional imaging: a general linear approach. Hum. Brain Mapp. 2, 189–210. doi: 10.1002/hbm.460020402
Fromkin, V., Rodman, R., and Hyams, N. (2014). An Introduction to Language, 10th Edn. Boston: Wadsworth.
Grodzinsky, Y., and Friederici, A. D. (2006). Neuroimaging of syntax and syntactic processing. Curr. Opin. Neurobiol. 16, 240–246. doi: 10.1016/j.conb.2006.03.007
Grodzinsky, Y., and Santi, A. (2008). The battle for Broca’s region. Trends Cogn. Sci. 12, 474–480. doi: 10.1016/j.tics.2008.09.001
Hagoort, P. (2005). On Broca, brain, and binding: a new framework. Trends Cogn. Sci. 9, 416–423. doi: 10.1016/j.tics.2005.07.004
Hagoort, P. (2014). Nodes and networks in the neural architecture for language: Broca’s region and beyond. Curr. Opin. Neurobiol. 28, 136–141. doi: 10.1016/j.conb.2014.07.013
Hagoort, P. (2017). The core and beyond in the language-ready brain. Neurosci. Biobehav. Rev. 81, 194–204. doi: 10.1016/j.neubiorev.2017.01.048
Hauk, O., Johnsrude, I., and Pulvermü, F. (2004). Somatotopic representation of action words in human motor and premotor cortex. Neuron 41, 301–307. doi: 10.1016/S0896-6273(03)00838-9
Hauk, O., and Pulvermüller, F. (2011). The lateralization of motor cortex activation to action-words. Front. Hum. Neurosci. 5:149. doi: 10.3389/fnhum.2011.00149
Hickok, G., and Poeppel, D. (2004). Dorsal and ventral streams: a framework for understanding aspects of the functional anatomy of language. Cognition 92, 67–99. doi: 10.1016/j.cognition.2003.10.011
Hickok, G., and Poeppel, D. (2007). The cortical organization of speech processing. Nat. Rev. Neurosci. 8, 393–402. doi: 10.1038/nrn2113
Horwitz, B., Rumsey, J. M., and Donohue, B. C. (1998). Functional connectivity of the angular gyrus in normal reading and dyslexia. Proc. Natl. Acad. Sci. U.S.A. 95, 8939–8944. doi: 10.1073/pnas.95.15.8939
Humphries, C., Binder, J. R., Medler, D. A., and Liebenthal, E. (2007). Time course of semantic processes during sentence comprehension: an fMRI study. Neuroimage 36, 924–932. doi: 10.1016/j.neuroimage.2007.03.059
Jefferies, E. (2013). The neural basis of semantic cognition: converging evidence from neuropsychology, neuroimaging and TMS. Cortex 49, 611–625. doi: 10.1016/j.cortex.2012.10.008
Jouen, A. L., Ellmore, T. M., Madden, C. J., Pallier, C., Dominey, P. F., and Ventre-Dominey, J. (2015). Beyond the word and image: characteristics of a common meaning system for language and vision revealed by functional and structural imaging. Neuroimage 106, 72–85. doi: 10.1016/j.neuroimage.2014.11.024
Keller, T. A., Carpenter, P. A., and Just, M. A. (2001). The neural bases of sentence comprehension: a fMRI examination of syntactic and lexical processing. Cereb. Cortex 11, 223–237. doi: 10.1093/cercor/11.3.223
Kelly, C., Uddin, L. Q., Shehzad, Z., Margulies, D. S., Castellanos, F. X., Milham, M. P., et al. (2010). Broca’s region: linking human brain functional connectivity data and non-human primate tracing anatomy studies. Eur. J. Neurosci. 32, 383–398. doi: 10.1111/j.1460-9568.2010.07279.x
Kim, K. H. S., Relkin, N. R., Lee, K.-M., and Hirsch, J. (1997). Distinct cortical areas associated with native and second languages. Nature 388, 171–174. doi: 10.1038/40623
Kriegeskorte, N., Goebel, R., and Bandettini, P. (2006). Information-based functional brain mapping. Proc. Natl. Acad. Sci. U.S.A. 13, 3863–3868. doi: 10.1073/pnas.0600244103
Lau, E. F., Phillips, C., and Poeppel, D. (2008). A cortical network for semantics: (De)constructing the N400. Nat. Rev. Neurosci. 9, 920–933. doi: 10.1038/nrn2532
Lee, H., Devlin, J. T., Shakeshaft, C., Stewart, L. H., Brennan, A., Glensman, J., et al. (2007). Anatomical traces of vocabulary acquisition in the adolescent brain. J. Neurosci. 27, 1184–1189. doi: 10.1523/JNEUROSCI.4442-06.2007
Mahon, B. Z., and Caramazza, A. (2008). A critical look at the embodied cognition hypothesis and a new proposal for grounding conceptual content. J. Physiol. Paris 102, 59–70. doi: 10.1016/j.jphysparis.2008.03.004
Makuuchi, M., and Friederici, A. D. (2013). Hierarchical functional connectivity between the core language system and the working memory system. Cortex 49, 2416–2423. doi: 10.1016/j.cortex.2013.01.007
Marslen-Wilson, W., and Tyler, L. K. (1980). The temporal structure of spoken language understanding. Cognition 8, 1–71. doi: 10.1016/0010-0277(80)90015-3
Meyer, K., and Damasio, A. (2009). Convergence and divergence in a neural architecture for recognition and memory. Trends Neurosci. 32, 376–382. doi: 10.1016/j.tins.2009.04.002
Mitchell, T. M., Shinkareva, S. V., Carlson, A., Chang, K.-M., Malave, V. L., Mason, R. A., et al. (2008). Predicting human brain activity associated with the meanings of nouns. Science 320, 1191–1195. doi: 10.1126/science.1152876
National Institute for Japanese Language, and Linguistics (2018). The Balanced Corpus of Contemporary Written Japanese. Tokyo: National Institute for Japanese Language, and Linguistics.
Newman, S. D., Ikuta, T., and Burns, T. (2010). The effect of semantic relatedness on syntactic analysis: an fMRI study. Brain Lang. 113, 51–58. doi: 10.1016/j.bandl.2010.02.001
Nichols, T. E., and Holmes, A. P. (2001). Nonparametric permutation tests for functional neuroimaging: a primer with examples. Hum. Brain Mapp. 15, 1–25. doi: 10.1016/B978-012264841-0/50048-2
Noonan, K. A., Jefferies, E., Visser, M., and Lambon Ralph, M. A. (2013). Going beyond inferior prefrontal involvement in semantic control: evidence for the additional contribution of dorsal angular gyrus and posterior middle temporal cortex. J. Cogn. Neurosci. 25, 1824–1850. doi: 10.1162/jocn_a_00442
Oberhuber, M., Hope, T. M. H., Seghier, M. L., Parker Jones, O., Prejawa, S., Green, D. W., et al. (2016). Four functionally distinct regions in the left supramarginal gyrus support word processing. Cereb. Cortex 26, 4212–4226. doi: 10.1093/cercor/bhw251
Pallier, C., Devauchelle, A.-D., and Dehaene, S. (2011). Cortical representation of the constituent structure of sentences. Proc. Natl. Acad. Sci. U.S.A. 108, 2522–2527. doi: 10.1073/pnas.1018711108
Prabhakaran, R., Blumstein, S. E., Myers, E. B., Hutchison, E., and Britton, B. (2006). An event-related fMRI investigation of phonological-lexical competition. Neuropsychologia 44, 2209–2221. doi: 10.1016/j.neuropsychologia.2006.05.025
Price, A. R., Peelle, J. E., Bonner, M. F., Grossman, M., and Hamilton, R. H. (2016). Causal evidence for a mechanism of semantic integration in the angular gyrus as revealed by high-definition transcranial direct current stimulation. J. Neurosci. 36, 3829–3838. doi: 10.1523/jneurosci.3120-15.2016
Price, C. J. (2010). The anatomy of language: a review of 100 fMRI studies published in 2009. Ann. N. Y. Acad. Sci. 1191, 62–88. doi: 10.1111/j.1749-6632.2010.05444
Price, C. J. (2018). The evolution of cognitive models: from neuropsychology to neuroimaging and back. Cortex 107, 37–49. doi: 10.1016/j.cortex.2017.12.020
Ralph, M. A. L., Jefferies, E., Patterson, K., and Rogers, T. T. (2017). The neural and computational bases of semantic cognition. Nat. Rev. Neurosci. 18, 42–55. doi: 10.1038/nrn.2016.150
Seghier, M. L. (2013). The angular gyrus: multiple functions and multiple subdivisions. Neurosci. 19, 43–61. doi: 10.1177/1073858412440596
Seghier, M. L., Fagan, E., and Price, C. J. (2010). Functional subdivisions in the left angular gyrus where the semantic system meets and diverges from the default network. J. Neurosci. 30, 16809–16817. doi: 10.1523/JNEUROSCI.3377-10.2010
Shinkareva, S. V., Malave, V. L., Mason, R. A., Mitchell, T. M., and Just, M. A. (2011). Commonality of neural representations of words and pictures. Neuroimage 54, 2418–2425. doi: 10.1016/j.neuroimage.2010.10.042
Simanova, I., Hagoort, P., Oostenveld, R., and Van Gerven, M. A. J. (2014). Modality-independent decoding of semantic information from the human brain. Cereb. Cortex 24, 426–434. doi: 10.1093/cercor/bhs324
Sliwinska, M. W., Khadilkar, M., Campbell-Ratcliffe, J., Quevenco, F., and Devlin, J. T. (2012). Early and sustained supramarginal gyrus contributions to phonological processing. Front. Psychol. 3:161. doi: 10.3389/fpsyg.2012.00161
Smith, S. M., and Nichols, T. E. (2009). Threshold-free cluster enhancement: addressing problems of smoothing, threshold dependence and localisation in cluster inference. Neuroimage 44, 83–98. doi: 10.1016/j.neuroimage.2008.03.061
Stoeckel, C., Gough, P. M., Watkins, K. E., and Devlin, J. T. (2009). Supramarginal gyrus involvement in visual word recognition. Cortex 45, 1091–1096. doi: 10.1016/j.cortex.2008.12.004
Tan, L. H., Laird, A. R., Li, K., and Fox, P. T. (2005). Neuroanatomical correlates of phonological processing of Chinese characters and alphabetic words: a meta-analysis. Hum. Brain Mapp. 25, 83–91. doi: 10.1002/hbm.20134
Van de Putte, E., De Baene, W., Brass, M., and Duyck, W. (2017). Neural overlap of L1 and L2 semantic representations in speech: a decoding approach. Neuroimage 162, 106–116. doi: 10.1016/j.neuroimage.2017.08.082
Whitney, C., Kirk, M., O’Sullivan, J., Lambon Ralph, M. A., and Jefferies, E. (2011). The neural organization of semantic control: TMS evidence for a distributed network in left inferior frontal and posterior middle temporal gyrus. Cereb. Cortex 21, 1066–1075. doi: 10.1093/cercor/bhq180
Wurm, M. F., and Caramazza, A. (2019). Distinct roles of temporal and frontoparietal cortex in representing actions across vision and language. Nat. Commun. 10:289. doi: 10.1038/s41467-018-08084-y
Yang, Y., Wang, J., Bailer, C., Cherkassky, V., and Just, M. A. (2017a). Commonalities and differences in the neural representations of English, Portuguese, and Mandarin sentences: when knowledge of the brain-language mappings for two languages is better than one. Brain Lang. 175, 77–85. doi: 10.1016/j.bandl.2017.09.007
Yang, Y., Wang, J., Bailer, C., Cherkassky, V., and Just, M. A. (2017b). Commonality of neural representations of sentences across languages: predicting brain activation during Portuguese sentence comprehension using an English-based model of brain function. Neuroimage 146, 658–666. doi: 10.1016/j.neuroimage.2016.10.029
Keywords: semantic processing, sentence comprehension, bilingualism, fMRI, MVPA
Citation: Hu Z, Yang H, Yang Y, Nishida S, Madden-Lombardi C, Ventre-Dominey J, Dominey PF and Ogawa K (2019) Common Neural System for Sentence and Picture Comprehension Across Languages: A Chinese–Japanese Bilingual Study. Front. Hum. Neurosci. 13:380. doi: 10.3389/fnhum.2019.00380
Received: 25 June 2019; Accepted: 11 October 2019;
Published: 25 October 2019.
Edited by:
Hidehiko Okamoto, International University of Health and Welfare (IUHW), JapanReviewed by:
Andrea Leo, IMT School for Advanced Studies Lucca, ItalyBernadette Maria Jansma, Maastricht University, Netherlands
Copyright © 2019 Hu, Yang, Yang, Nishida, Madden-Lombardi, Ventre-Dominey, Dominey and Ogawa. This is an open-access article distributed under the terms of the Creative Commons Attribution License (CC BY). The use, distribution or reproduction in other forums is permitted, provided the original author(s) and the copyright owner(s) are credited and that the original publication in this journal is cited, in accordance with accepted academic practice. No use, distribution or reproduction is permitted which does not comply with these terms.
*Correspondence: Kenji Ogawa, b2dhd2FAbGV0Lmhva3VkYWkuYWMuanA=