- 1Department of Applied Physiology and Kinesiology, College of Health and Human Performance, University of Florida, Gainesville, FL, United States
- 2German Aerospace Center, Cologne, Germany
- 3KBRWyle, Houston, TX, United States
- 4Johnson Space Center, NASA, Houston, TX, United States
- 5Department of Neurology, University of Florida, Gainesville, FL, United States
Long duration head down tilt bed rest (HDBR) has been widely used as a spaceflight analog environment to understand the effects of microgravity on human physiology and performance. Reports have indicated that crewmembers onboard the International Space Station (ISS) experience symptoms of elevated CO2 such as headaches at lower levels of CO2 than levels at which symptoms begin to appear on Earth. This suggests there may be combinatorial effects of elevated CO2 and the other physiological effects of microgravity including headward fluid shifts and body unloading. The purpose of the current study was to investigate these effects by evaluating the impact of 30 days of 6° HDBR and 0.5% CO2 (HDBR + CO2) on mission relevant cognitive and sensorimotor performance. We found a facilitation of processing speed and a decrement in functional mobility for subjects undergoing HDBR + CO2 relative to our previous study of HDBR in ambient air. In addition, nearly half of the participants in this study developed signs of Spaceflight Associated Neuro-ocular Syndrome (SANS), a constellation of ocular structural and functional changes seen in approximately one third of long duration astronauts. This allowed us the unique opportunity to compare the two subgroups. We found that participants who exhibited signs of SANS became more visually dependent and shifted their speed-accuracy tradeoff, such that they were slower but more accurate than those that did not incur ocular changes. These small subgroup findings suggest that SANS may have an impact on mission relevant performance inflight via sensory reweighting.
New And Noteworthy
We examined the effects of long duration head down tilt bed rest coupled with elevated CO2 as a spaceflight analog environment on human cognitive and sensorimotor performance. We found enhancements in processing speed and declines in functional mobility. A subset of participants exhibited signs of Spaceflight Associated Neuro-ocular Syndrome (SANS), which affects approximately one in three astronauts. These individuals increased their visual reliance throughout the intervention in comparison to participants who did not show signs of SANS.
Introduction
Successful spaceflight missions depend upon high levels of human performance. However, it is well established that astronauts experience altered sensory perception (Kornilova, 1997a; Clement et al., 2013) and declines in manual control, balance and locomotion both in- and post-flight (Lackner and DiZio, 1996; Kornilova, 1997b; Reschke et al., 1998; Bock et al., 2003, 2010). Moreover, astronauts have also reported “space fog” (Welch et al., 2009), including mental slowing, poor concentration, and slowed performance because of “feeling different than usual” (Kanas and Manzey, 2008).
Body unloading, altered vestibular signals, and fluid shifts toward the head from microgravity are thought to contribute to sensorimotor declines (Hargens and Richardson, 2009). In addition, crewmembers residing in the confined compartment of the International Space Station (ISS) are exposed to elevated ambient CO2 levels averaging 0.5%, which is more than ten times greater than terrestrial levels (0.04%) (Law et al., 2014). The environment aboard the ISS poses a potential risk to mission success and the long-term health of astronauts, as prolonged exposure to elevated ambient CO2 results in increased cerebral blood flow and mild performance impairments (Manzey and Lorenz, 1998; Satish et al., 2012; Fisk et al., 2013; Allen et al., 2015, 2018). Moreover, space station crewmembers have exhibited symptoms of elevated CO2 exposure such as headaches occurring at a more mild level of CO2 elevation than for headache symptoms in Earth-based studies of elevated CO2 (Law et al., 2014). Thus, there may be an interactive effect of elevated CO2 and the fluid shifts toward the head which we (Lee et al., 2019) and others (Roberts et al., 2017) have shown occur with spaceflight.
Long duration, head down tilt bed rest (HDBR) has been widely used to simulate the physiological impacts of microgravity such as arterial pressure changes, unloading of the lower body, and cephalad fluid shifts (Hargens and Vico, 2016). HDBR has been shown to result in decreased postural stability and functional mobility (Reschke et al., 2009; Mulder et al., 2014; Koppelmans et al., 2015, 2017; Miller et al., 2018; Mulavara et al., 2018). Moreover, a previous study has shown that prolonged exposure (26 days) to moderately elevated CO2 (0.7 and 1.2% concentration) negatively impacts visuomotor function (Manzey and Lorenz, 1998).
We administered the same cognitive and sensorimotor assessments here as we previously applied in an ambient air HDBR study (Koppelmans et al., 2015), allowing us to examine the combined effects of elevated HDBR + CO2 relative to HDBR alone. This comparison is somewhat exploratory, however, as the duration of HDBR differed between the two studies (experimental protocols in both cases were established by NASA’s Flight Analogs Program). Here, our primary aim was to investigate the effects of 6° HDBR with sustained exposure to elevated CO2 levels (0.5%, HDBR + CO2) on a range of cognitive and sensorimotor tests. Basner et al. (2017) recently reported altered cognitive response strategies (speed-accuracy trade-offs) as a result of acute exposure (i.e., 26.5 h) to 0.5% CO2 combined with 12° HDBR. In light of these findings, we hypothesized that subjects would show increased response speed and diminished accuracy on a series of cognitive assessments during 30 days of HDBR + CO2.
Approximately one third of astronauts who complete long-duration missions (Lee et al., 2016) manifest optic nerve and/or ocular changes, a condition referred to as Spaceflight Associated Neuro-ocular Syndrome (SANS) (Mader et al., 2011). Interestingly, at the end of the HDBR + CO2 intervention, five of the 11 participants in the current study showed bilateral optic disc edema, a sign of SANS, with Modified Frisèn Scale grades between one (n = 4) and two (n = 1) (Laurie et al., 2019). This was the first time that signs of SANS have been seen in HDBR; the finding provided us with a unique opportunity to further characterize the performance profiles of the participants who did and did not develop signs of SANS. The subgroup comparison between those who showed signs of SANS and did not (hereinafter referred to as SANS and NoSANS) will be carried out as a secondary aim.
Materials and Methods
Study Design and Participants
In the present analyses we compared two groups, one that underwent a 70 day, 6° HDBR study (HDBR group) and another that underwent a 30 day, 6° HDBR study coupled with an elevation of 0.5% CO2 (HDBR + CO2). In both groups, we administered a battery of cognitive and sensorimotor tests before, during, and after the bedrest period.
HDBR + CO2
Our experiment was implemented within the larger VaPER (Visual impairment intracranial pressure and Psychological:envihab Research) study which was conducted at:envihab, an environmental medicine research facility at the German Aerospace Center (DLR) in Cologne, Germany. Healthy non-smoking adults aged between 24 and 55 years with a body mass index between 19–30 kg/m2 and free of any chronic illness and pain, elevated risk of thrombosis or bone fractures less than 1 year prior to the study were recruited. The potential volunteers were further screened for clearly defined medical exclusion criteria such as: chronic hypertension, diabetes, obesity, arthritis, hyperlipidemia, hepatic disease (A, C), or a disorder of calcium or bone metabolism. The subjects were also screened for any eye conditions that could significantly impact visual function. Volunteers that were medically eligible for the study subsequently underwent psychological screening.
Eleven individuals (6 males and 5 females) aged 25.3–50.3 years at the time of study admission participated. They were admitted to the study 14 days prior to the start of the 6° HDBR + CO2 intervention which lasted for 30 days. The level of atmospheric CO2 enrichment during bed rest (3.8 mmHG ambient partial pressure of carbon dioxide) was selected to match the average CO2 concentration onboard the ISS (Law et al., 2014).
All participants strictly adhered to the head down tilt position at all times. Subjects received a controlled diet and were provided with an 8-h sleep opportunity from 10:30 PM until 6:30 AM daily. They participated in multiple other NASA-funded studies during the HDBR + CO2 intervention such as ocular, intracranial pressure (ICP) measures and blood gas analysis. Participants were dismissed 14 days following completion of the bed rest phase; they received monetary compensation for their participation. The study procedure was approved by the local ethical commission of the regional medical association (Ärztekammer Nordrhein) as well as the University of Florida and NASA Institutional Review Boards. All subjects provided written informed consent at the German Aerospace Center in Cologne, Germany.
HDBR
The methods of our past ambient air HDBR study have been described in previous publications (Koppelmans et al., 2013, 2015, 2017; Cassady et al., 2016; Yuan et al., 2018). The participants were 18 males aged 25.8–39.8 years at the time of admission. These subjects were randomly assigned either to a no-exercise (n = 5), an aerobic and resistance exercise (n = 5), or a flywheel exercise group (n = 8). These groups were pooled for the analyses here.
The demographic information of the HDBR + CO2 and HDBR groups as well as the HDBR + CO2 subgroups by SANS status is provided in Table 1. All HDBR + CO2 and HDBR subjects passed an Air Force Class III equivalent physical examination. None of the participants were exposed to both experimental procedures.
Testing Timeline
HDBR + CO2
Behavioral measurements were obtained across six time points: (a) two baseline measurements 13 and 7 days before bed rest; (b) two measurements 7 and 29 days during bed rest; and (c) two measurements 5 and 12 days post bed rest. Functional mobility and balance were not tested during bed rest as they would have required participants to be upright. Instead, these two behaviors were tested on the first day following HDBR + CO2 completion; the data were obtained within approximately 3 h of participants first standing up.
HDBR
The assessment timeline of the HDBR subjects has been previously published (Koppelmans et al., 2015). Behavioral measurements were obtained at seven time points: (a) two measurements approximately 12 and 8 days before bed rest; (b) three measurements at approximately 8, 50, and 65 days in bed rest; and (c) the first day of standing up from bed rest for tests requiring upright stance, and days 7 and 13 post bed rest for all tests (see Figure 1 for testing time line and average assessment days for HDBR + CO2 and HDBR). Given that the HDBR duration and testing time points differed between the two studies, we compared the slopes of behavioral change over time to assess any additive effects of HDBR + CO2 over HDBR.
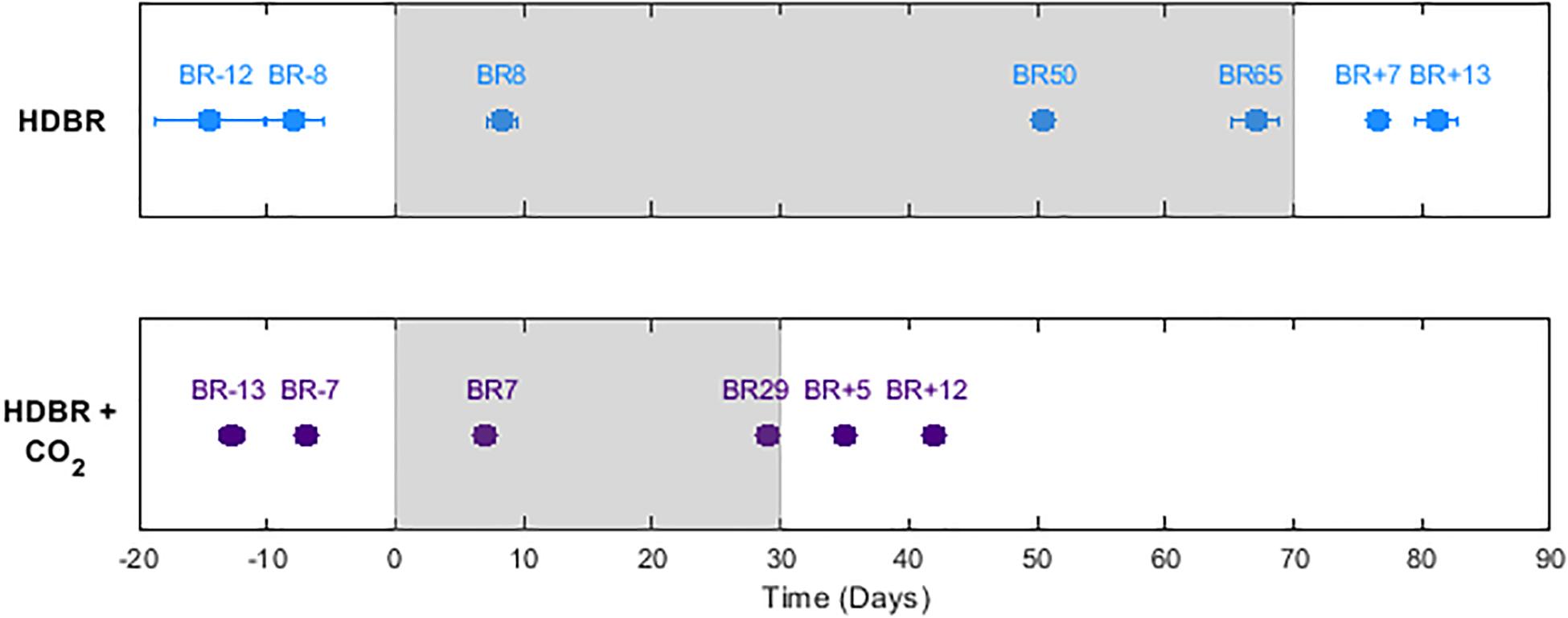
Figure 1. Assessment time line and mean testing days. HDBR + CO2 refers to the current data set, while HDBR refers to our previous 70 day bed rest study. BR-days refer to the number of days prior to entering bed rest, BR days are within the bed rest intervention, and BR + days are after exiting bed rest. The postural assessment and functional mobility test were also performed on R + 0.
Cognitive and Sensorimotor Tests
All tests were identical between the two studies, with the exception of the balance assessments. This will be described further below. A detailed overview of the cognitive and sensorimotor tests can be found in Koppelmans et al. (2013).
Neuropsychological Assessments
Standard neuropsychological tests were used to measure several cognitive constructs. The paper-and-pencil version of the digit symbol substitution test (Lezak, 2004) was used to assess processing speed; we determined completion time and accuracy. Thurstone’s 2D card rotation task was administered to measure spatial working memory performance (Ekstrom et al., 1976). The total and correct number of responses made within 3 min were measured as indicators of performance. The Purdue pegboard test was used to evaluate bimanual coordination (Tiffin and Asher, 1948). The time taken to bimanually insert small cylindrical metal pegs into 15 rows of two parallel holes was measured. The testing materials for these three assessments were mounted on a board that could be height-adjusted for each subject so that the subjects could comfortably perform the test in the head down tilt position.
The rod and frame test (RFT) (Kindrat, 2011; Nair et al., 2018) was used to measure reliance on visual vs. other cues (vestibular, proprioceptive) for vertical perception. Each subject’s field dependency and error profile were calculated based on the degrees of deviation between the perceived and true vertical. The rod angle error relative to the actual vertical was measured for each of the trials. Constant error measures the subjects’ average bias in estimation of the true vertical across all trials and is characteristic of each subject (Nyborg, 1974). The frame effect is the main measure of visual dependence, which shows the magnitude of the error in the subjects’ estimate of the vertical caused by the tilted frame regardless of direction of frame or rod tilt, while also taking into account individual subjects’ bias (Nyborg, 1974). Response consistency refers to the within subject variability of scores obtained across the same rod/frame tilt combination. Given that higher values on this measure refer to greater variability, we refer to this variable as response variability from here forward.
Visuospatial processing was assessed using the 3D Cube Mental Rotation Task (Shepard and Metzler, 1988). The response time and accuracy to determine the 3-dimensionally rotated form of the previously seen groups of cubes were indicators of performance. Participants were lying on their side to complete the RFT and mental rotation tasks so that they could view a monitor placed bedside.
Single and Dual Tasking
Participants performed a finger tapping motor task under single and dual task conditions (Yuan et al., 2016) while in an MRI scanner; only behavioral results are presented here. Two stimulus boxes were presented on the screen side-by-side. An “x” appeared in one of the two locations randomly with an inter-stimulus interval of 800 ms; subjects were instructed to press the corresponding button with the left or right index finger as quickly as possible. The secondary counting task required subjects to watch a square that was changing colors at a rate of 3 Hz and to count the number of times a target color appeared. Target color appearance was low (1–3%) so that subjects had to remain vigilant. Participants performed the two tasks in isolation and simultaneously. Tap reaction time, tap accuracy and counting accuracy were analyzed as performance measures.
Functional Mobility Test
The 6.0 m × 4.0 m functional mobility test (FMT) course consists of several foam obstacles such as hurdles, pylons and bars presenting a series of mobility challenges; the test has been shown to be sensitive to the effects of spaceflight and bed rest (Reschke et al., 2009; Mulavara et al., 2010; Kopplemans et al., 2017). The first half of the FMT course was set up on a hard floor, whereas the second part was set up on a base of medium density foam to increase postural challenges. Participants were instructed to walk through the course as quickly and safely as possible, without touching any of the obstacles. All participants wore the same brand canvas tennis shoes during the mobility tests, and completed the course a total of 10 times. Here, we analyzed the time to complete the first trial as we have found that this first trial is most sensitive to intervention-associated changes in mobility. The completion time of the first (i.e., hard surface) and second (i.e., compliant foam surface) half of the course during the first trial were separately evaluated.
Posturography
A posturography test (Black, 2001) was carried out in order to assess the subjects’ ability to control their balance. Details of the test have been previously described in Mulder et al. (2014). The subjects were instructed to maintain a stable upright posture in open stance with their arms folded across their chest for 30 s on a foam pad that rested on a force platform (Leonardo Mechanograph, Novotec Medical GmbH, Pforzheim, Germany). The feet were aligned against the guidelines demarcated on the foam pad in order to maintain consistent foot positioning between and within subjects. Balance control was measured in three conditions: (a) eyes open with the head erect (EO); (b) eyes closed with the head erect (EC); and (c) eyes closed with dynamic head tilts (ECDHT), where the subjects rhythmically made head pitch motions of ±20° synchronized to a 0.33 Hz metronome tone. Each condition was performed three times, where the order of conditions was semi-randomized to ensure no identical conditions repeated back-to-back.
The balance assessment of the HDBR study utilized a computerized dynamic posturography system (Equitest, NeuroCom International, Clackamas, OR, United States) (Reschke et al., 2009). The subjects performed two conditions with their eyes closed, on a sway-referenced base; one with head erect (Sensory Organization Test 5) and another with dynamic head tilts (following a 0.33 Hz-paced audible tone). Each condition consisted of three 20-s trials. Based on the similarity of the balance challenges, only the EC and ECDHT conditions will be statistically analyzed for the HDBR + CO2 and HDBR group comparison.
For all three balance control conditions, we selected the median score of the three trials to prevent effects of outliers. The anterior-posterior (AP) peak-to-peak center-of-mass sway angle was used to compute an equilibrium score (EQ).
Statistical Analyses
HDBR + CO2
We conducted mixed model linear regression analyses on the HDBR + CO2 participants, entering time as a continuous variable to assess the effect of the intervention on performance. We omitted the first time point as a practice session. In addition, we excluded the post-HDBR time points for this initial analysis as the intervention effect was our main interest. We used mixed effect models to control for the correlation between repeat visits of the same individual. Moreover, age and sex were entered as covariates to control for their potential confounding effects.
Effects of Group and Time in HDBR + CO2 and HDBR Subjects
The intervals between test dates and the overall study duration differed for the HDBR + CO2 and HDBR studies. Thus, we entered time as a continuous variable when comparing HDBR + CO2 and HDBR effects. Linear mixed model analysis was used to examine group × time differences between HDBR + CO2 and HDBR subjects. The subject variable was entered as a random intercept. First, in order to examine the additive effect of CO2, we tested the simple effects of time, group, and group by time for each outcome measure. We included data from the second baseline measurement and the next two time points, which were collected within HDBR. We also compared the recovery time course between the two groups. For this analysis, we included data from the last HDBR assessment and the following two post-HDBR time points. We limited investigation of recovery effects to the measurements that exhibited significant HDBR-related group × time interactions. Age at admission, sex and exercise group status were entered as covariates.
For completeness, the abovementioned HDBR + CO2 analytic procedures were repeated within the HDBR group. Age and exercise group were entered as covariates to control for their potential confounding effects.
Effects of Group and Time in SANS and NoSANS Subjects
The SANS vs. NoSANS subgroup comparison analysis was carried out in a similar fashion to that of the HDBR + CO2 vs. HDBR comparison described above. For this analysis, age at admission and sex were entered into the model as covariates.
Restricted maximum likelihood (REML) was used in all linear mixed model analyses because its estimation is less sensitive to small sample bias than traditional maximum likelihood (Van Dongen, 1999). Alpha levels were set at 0.05 for all analyses. SPSS25 was used for all statistical analyses (IBM Corp. Released 2017. IBM SPSS Statistics for Windows, Version 25.0. Armonk, NY: IBM Corp.).
Model residuals were checked for normality using Shapiro–Wilk tests and via visual inspection of Q-Q plots. If distributions deviated from normality, the data were log transformed. In case the log transformation did not improve the normality of the residuals, the analysis was repeated without extreme outlier residual data points exceeding the third quartile. In cases here the models based on the untransformed and transformed data agreed after removing the outliers, we present the results based on the untransformed data for ease of interpretation.
Results
HDBR + CO2 Intervention Effect
For the HDBR + CO2 group, a significant main effect of time was observed for the time to complete the digit symbol substitution task. There was also a significant main effect of time for the completion time, accuracy and percent complete of the total 80-item 2D card rotation task. Additionally, although at a trend level (p = 0.05), a main effect of time was observed for the RFT response variability (see Table 2). The time to complete the digit symbol substitution task and the RFT response variability score decreased (RFT within subject responses became less variable) as the HDBR + CO2 intervention progressed. The 2D card rotation accuracy and the percentage of completed items increased with time, and the time to complete the task decreased as a function of time spent in the HDBR + CO2 environment (Supplementary Figure S1). There was also a significant main effect of time for the digit symbol substitution task accuracy after removing outliers (β = 0.012, p = 0.035). A few measures exhibited negatively skewed distributions; the RFT frame effect, single condition counting accuracy and dual condition tapping accuracy were all near ceiling, resulting in skewed distributions. As log transformation did not yield normally distributed residuals for these measures, the results with these tests should be interpreted with caution.
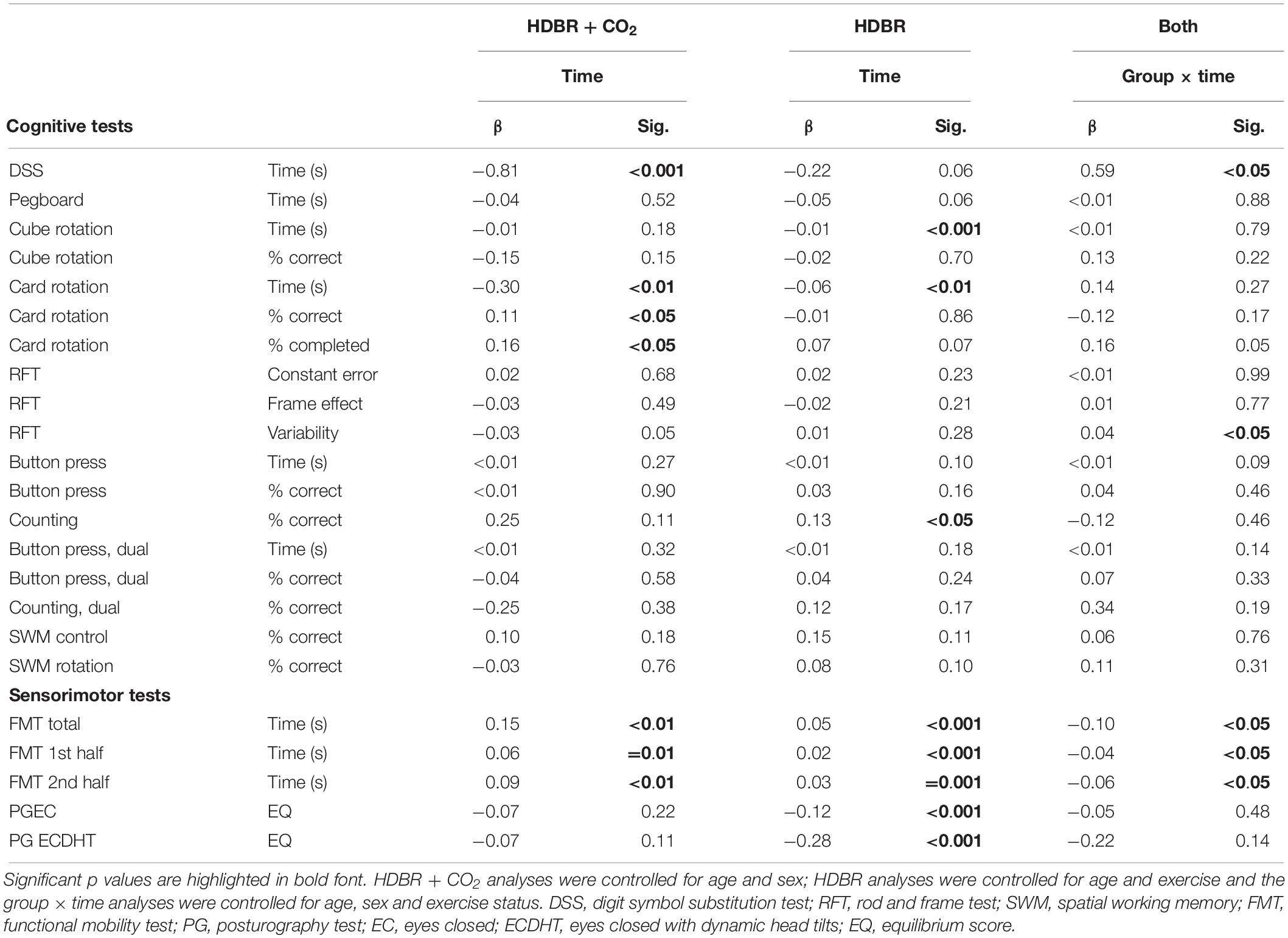
Table 2. Results from the statistical models evaluating the effect of time for the HDBR + CO2 and HDBR groups, and the group × time interaction.
HDBR + CO2 vs. HDBR
There was no statistically significant difference in age between the HDBR + CO2 and HDBR groups. A significant group by time interaction effect was observed for the digit symbol substitution task completion time (Figure 2A) and RFT response variability (Table 2 and Figure 2B). The degree of performance speed enhancement was greater in the HDBR + CO2 group than that of the HDBR group. Similarly, the RFT response variability continued to decrease over time in the HDBR + CO2 group while it remained unchanged in the HDBR group. Additionally, significant group by time interaction effects were observed for the total, first and second half FMT completion time (see Figure 2C); the degree of slowing from pre- to post-intervention was greater in the HDBR + CO2 group.
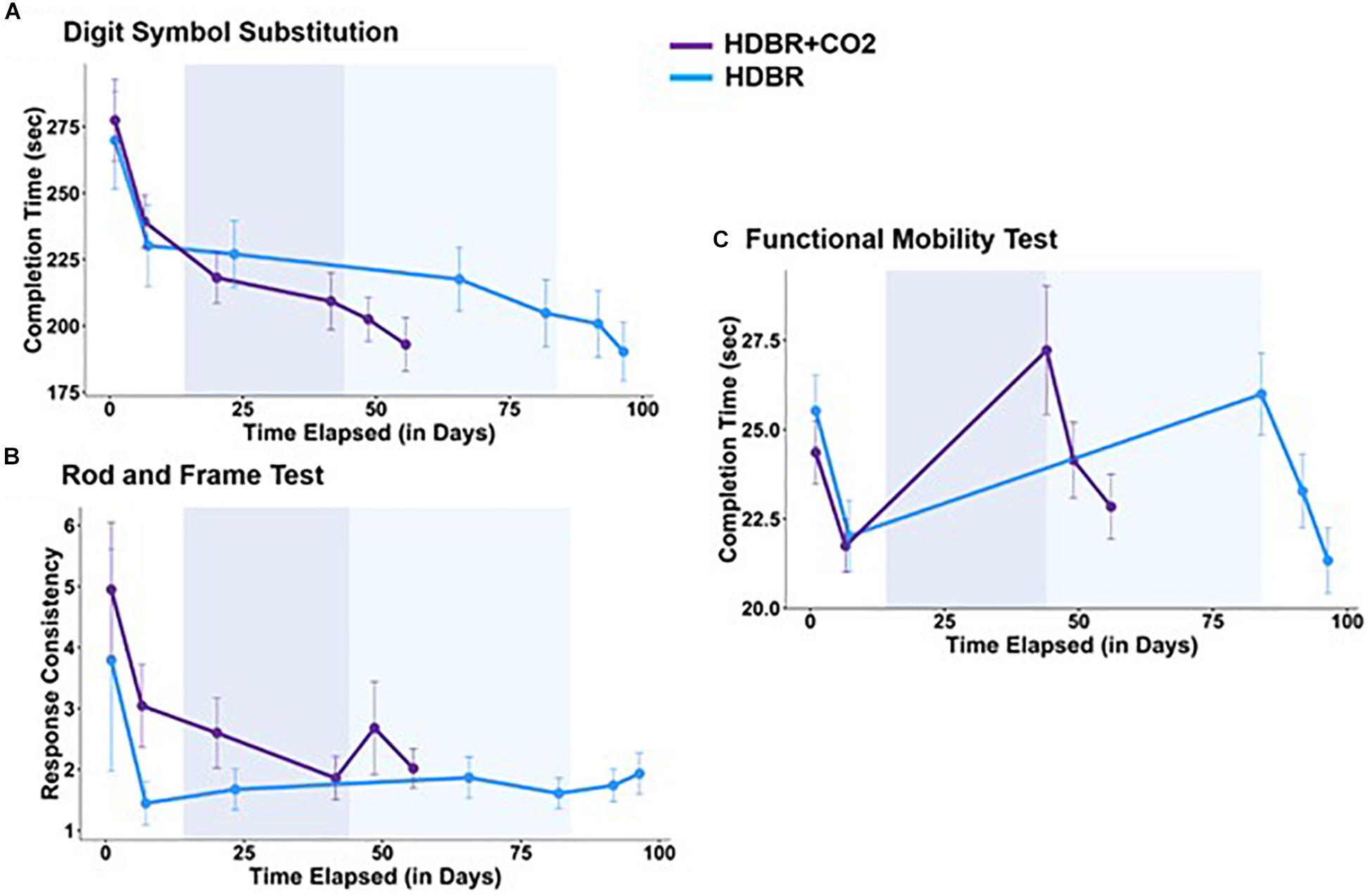
Figure 2. Group by time differential effects during bed rest were observed for (A) the digit symbol substitution completion time, (B) rod and frame test (RFT) response consistency, and (C) the functional mobility test (FMT). No differences were observed in recovery rate. The first two time points on each graph reflect pre bed rest testing. For the digit symbol test and the RFT, the next two time points were collected in bed rest, and the last two after bed rest (the bed rest phase of the HDBR and HDBR + CO2 groups are demarcated by shaded boxes of the corresponding color of the groups). For the FMT, the first two time points are pre bed rest and the last three are following bed rest. The error bars indicate SEM.
None of the measures showed significant group × time interactions post-intervention, supporting that participants recovered their performance following the HDBR + CO2 and HDBR interventions at the same rate (Table 3).
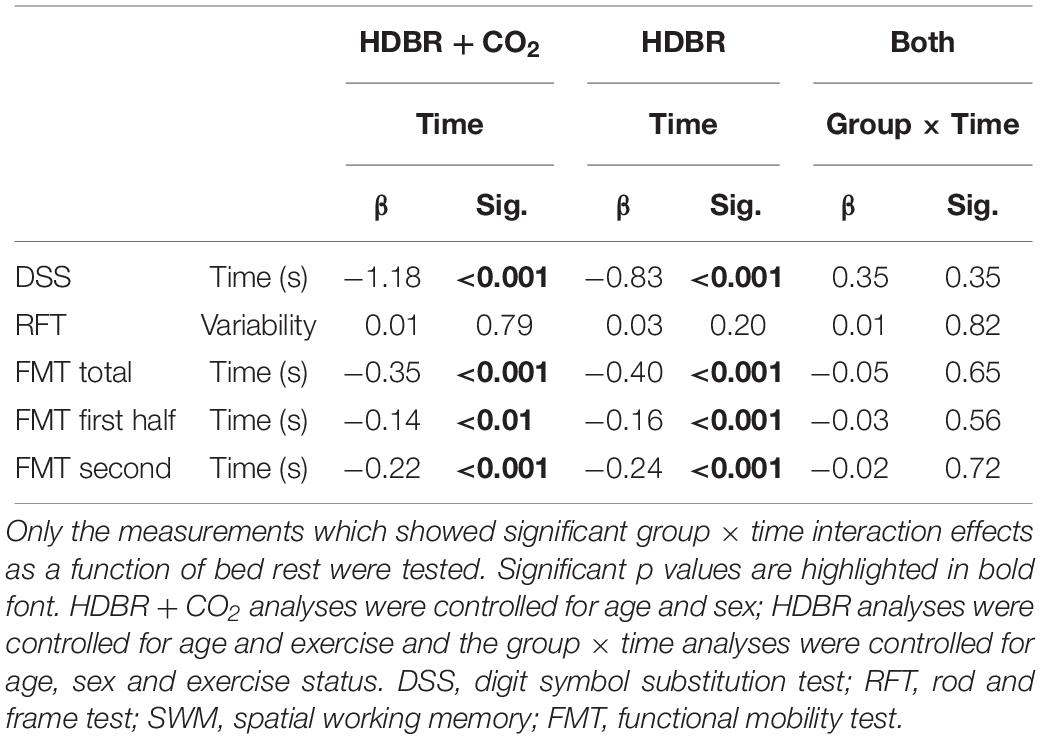
Table 3. Results from the statistical models evaluating the effect of time for the HDBR + CO2 and HDBR groups, and the group × time interaction during recovery.
HDBR + CO2 SANS vs. NoSANS
There was no statistically significant age difference between those who developed signs of SANS and those who did not (NoSANS) (see Table 1 and Supplementary Figure S2). Within each sex group, there was also no statistically significant age difference between SANS and NoSANS participants, but it should be noted that stratification into sex and SANS groups greatly reduces the sample size per cell.
A stratified analysis comparing performance for SANS and NoSANS participants revealed a significant group by time effect on the RFT frame effect (Figure 3A) and response variability (Table 4 and Figure 3B). The results indicated that the SANS group became increasingly visually dependent as the intervention progressed, while the NoSANS subgroup became less so. The RFT response variability for SANS also decreased during HDBR + CO2 relative to that of the NoSANS subgroup. Thus the SANS group became more consistently visually dependent.
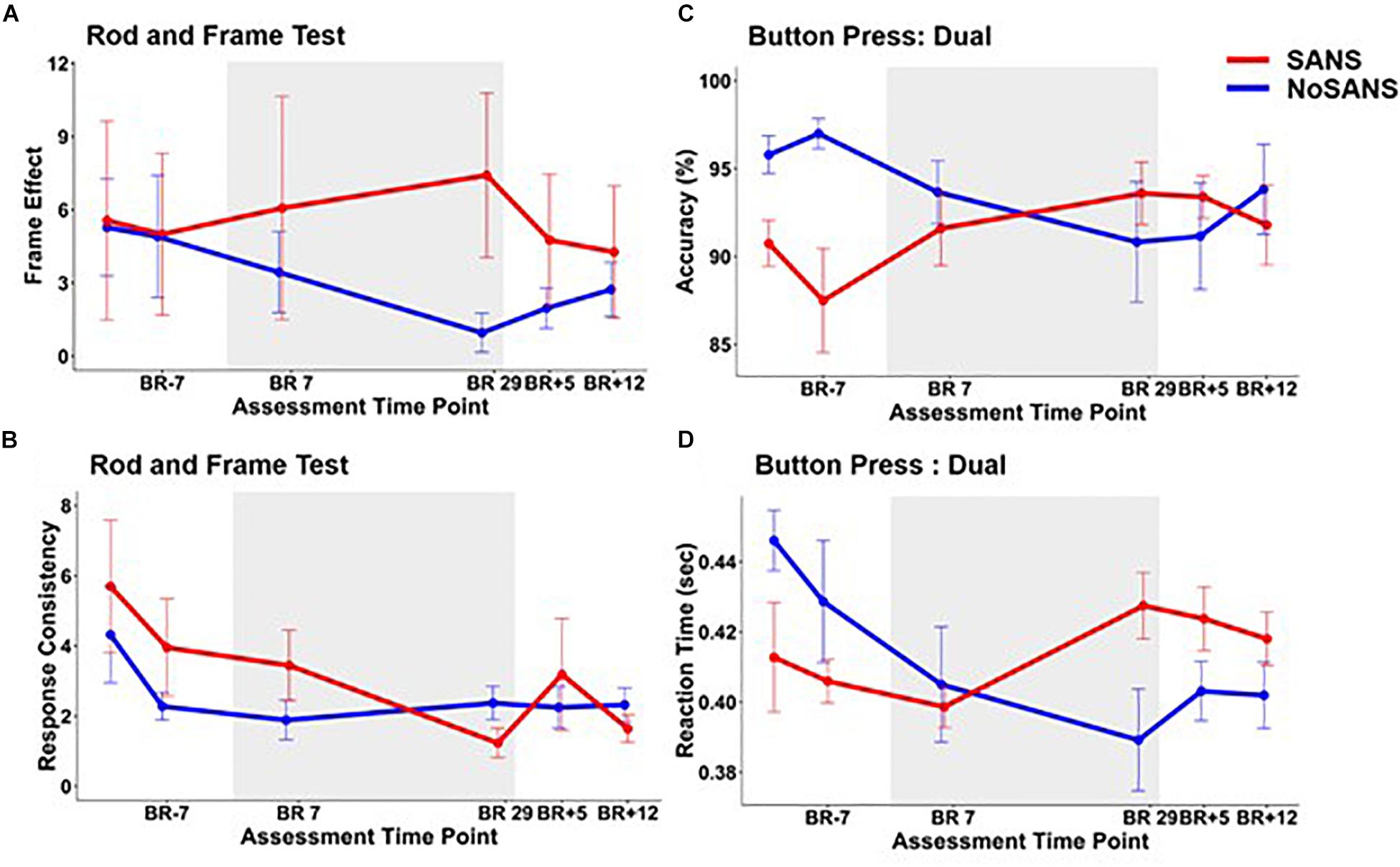
Figure 3. The SANS and NoSANS groups exhibited significantly different changes over time during bed rest on the rod and frame test (A) frame effect and (B) response consistency measures and also on the dual task button press (C) accuracy, and (D) reaction time. BR-days refer to the number of days prior to entering bed rest, BR days are within the bed rest intervention, and BR + days are after exiting bed rest. The gray shaded box indicates the time in which subjects were in bed rest. The error bars indicate SEM.
There was also a significant subgroup by time interaction for finger tapping accuracy under both single and dual task conditions and for finger tapping reaction time under the dual task condition. In both single and dual task conditions the accuracy of performance for the SANS subgroup increased, while the NoSANS subgroup decreased during the intervention (Figure 3C). The SANS subgroup dual task reaction time slowed down as the intervention progressed as opposed to the facilitation observed in the NoSANS group (Figure 3D).
A significant group by time interaction was observed in the eyes open, head erect (EO) posturography test condition. In comparison to the NoSANS group, the SANS group showed less decrements in postural control in this EO condition following the HDBR + CO2 intervention (Figure 4).
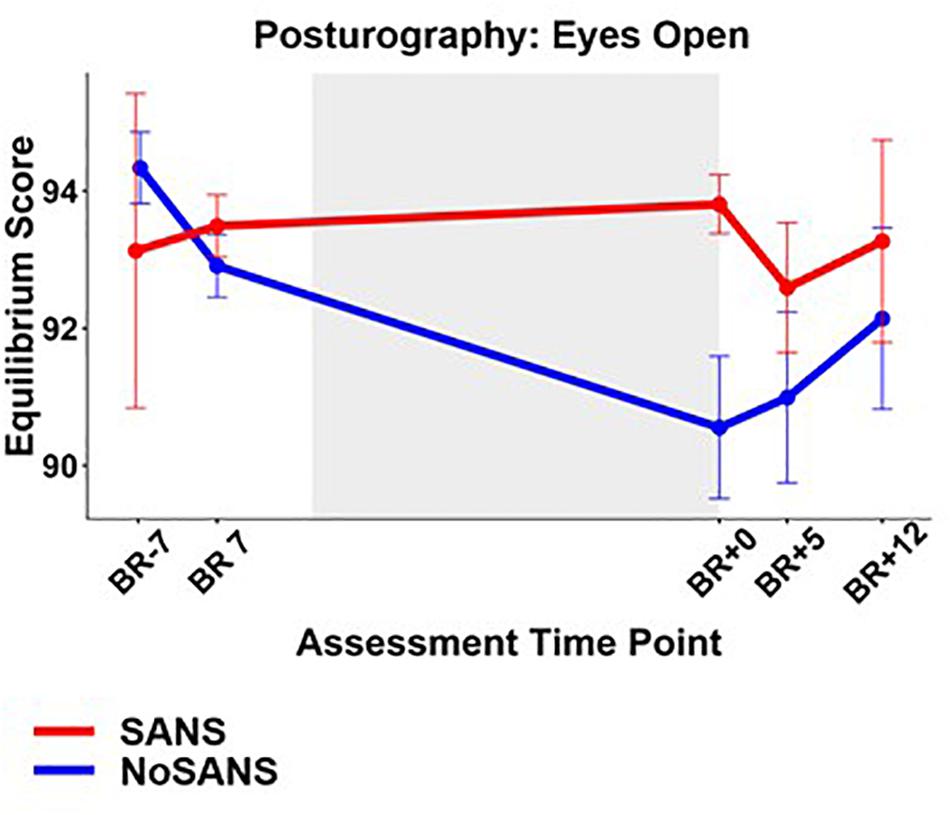
Figure 4. The SANS and NoSANS groups exhibited significantly different changes from pre- to post HDBR + CO2 on the balance performance of the eyes open condition of the posturography. BR-days refer to the number of days prior to entering bed rest, BR days are within the bed rest intervention, and BR + days are after exiting bed rest. The gray shaded box indicates the time in which subjects were in bed rest. The error bars indicate SEM.
Discussion
Here we investigated the combined effects of HDBR and increased CO2 concentration on a range of cognitive and sensorimotor assessments. We found a greater facilitation of processing speed and larger decrements in functional mobility relative to our previous HDBR study. The HDBR and HDBR + CO2 groups did not differ in digit symbol and functional mobility performance change over time post bed rest, suggesting an HDBR + CO2 specific facilitation of processing speed and decline of functional mobility, with recovery occurring at the same rate once subjects were out of the HDBR + CO2 and HDBR environments.
Although the HDBR + CO2 subjects showed improving performance in the 2D card rotation task, the degree of change was not greater than that of the HDBR group which showed similar patterns of change. This performance change seen in the HDBR + CO2 group would most likely reflect practice effects. Our finding of faster processing speed in the HDBR + CO2 subjects in comparison to the HDBR subjects is in line with previous reports of enhanced cognition under short duration (i.e., 26 h) 12° HDBR combined with 0.5% CO2 (Basner et al., 2018). Astronauts have also exhibited processing speed changes inflight, which fluctuate with reported arousal levels (Kelly et al., 2005). Although the exact CO2 level and response of the astronauts in that report are difficult to determine, increased vigilance and arousal as a result of CO2 exposure (Guyenet et al., 2010) in the current protocol may have contributed to the facilitation of processing speed. It is also possible that the cerebral vasodilation that occurs with elevated CO2 (Sliwka et al., 1998), which selectively favors frontal lobe perfusion (Bhogal et al., 2015), could have resulted in the enhanced processing speed here. This is particularly the case given frontal involvement in processing speed and efficiency (Rypma et al., 2006). One caveat however is that participants in the current study did not demonstrate a significant change in end-tidal PCO2 from beginning to end of the intervention (Laurie et al., 2019). This is difficult to interpret, however, given that these measures were not collected throughout the HDBR + CO2 campaign. Thus the lack of change might reflect adaptation over time.
The RFT, which measures an individual’s vertical perception (Witkin et al., 1954), also yields measures of RFT response variability. While the HDBR group maintained low response variability throughout the study, the HDBR + CO2 group demonstrated progressive decreases in response variability. Typically, more variable responses on this task indicate subject disorientation in the RFT (Nyborg, 1974). It is noteworthy that despite the baseline difference where the HDBR + CO2 group showed less consistent responses, this variability decreased as a function of time spent in the HDBR + CO2 condition.
The HDBR + CO2 intervention resulted in significant slowing to traverse the entire, first, and second half of the FMT course. This is in line with a previous long term bed rest intervention (Koppelmans et al., 2015) and spaceflight effects (Mulavara et al., 2010), which also led to declines in functional mobility. While reduced loading and usage of the lower limbs and sensory reweighting are the proposed major factors driving such findings (Lee et al., 2019), it is interesting that a greater degree of decrement was observed in the HDBR + CO2 group in comparison to HDBR, despite the relatively shorter intervention duration. Although the potentially protective effects of exercise in some of the HDBR group may have impacted these findings, this is unlikely to be the main driving force as we covaried for the exercise status in our analyses. Unlike the facilitation of psychomotor speed, the addition of elevated CO2 to HDBR led to a decrements in whole body functional mobility.
Differential Changes for SANS vs. NoSANS
Previous bed rest studies have not observed signs of SANS; the fact that five individuals did here allowed us to compare SANS and NoSANS subgroups (Laurie et al., 2019). This is important, as SANS has recently been shown to affect approximately 1/3 of crewmembers participating in missions of 6 months or longer (Lee et al., 2016). These two subgroups differed on several of our measures. Performance on the RFT relies on multisensory integration processes (Bronstein, 1999; Isableu et al., 2008; Lopez et al., 2008). Interestingly, posture control involves similar processes (Massion, 1994) where alteration in one sensory input results in multisensory reweighting (Ernst and Bulthoff, 2004). Moreover, field-dependent individuals who tend to base their perception of verticality on external cues are less stable and more dependent on visual cues for balance than individuals who are field independent. In line with these characteristics, we found that SANS individuals increased their reliance on visual cues during the HDBR + CO2 intervention. The NoSANS subgroup, in contrast, became more field-independent. In addition, individuals in the SANS subgroup decreased their response variability during the intervention. The SANS subgroup also showed higher levels of balance control in the eyes open condition. This profile of increased visual dependency with SANS is worth noting; as visual dependency has been associated with poorer navigation (Willey and Jackson, 2014) this may impact the re-adaptive process of astronaut. While it may seem counterintuitive to have ocular declines associated with increased reliance on vision, the signs of SANS here were quite mild in comparison to what is seen with spaceflight. The participants here exhibited ocular disc edema, whereas astronauts with SANS also have visual acuity changes, globe flattening, and other ocular structural changes. Perhaps the increased reliance on vision is a compensatory response to the early stages of SANS development.
The SANS subgroup showed increased finger tapping accuracy in both the single and dual task conditions while accuracy declined for the NoSANS group. Slowing in the SANS group may reflect an implicit strategy to maintain high levels of accuracy.
Limitations
Some of the opposing changes we observed in the SANS and NoSANS subgroups may have hindered our ability to detect overall group effects in response to HDBR + CO2. Moreover, the sample size of the HDBR + CO2 group is quite small (n = 11); replication should be performed before the results are broadly generalized. In addition, having a control group with the same testing schedule would help to better quantify changes.
This is the first time that symptoms of SANS have been reported in a spaceflight analog environment. While our findings are compelling and suggest implications for mission relevant behaviors, further investigation is warranted to determine whether these symptoms are comparable to the syndrome as manifested in astronauts.
Conclusion
The addition of elevated ambient carbon dioxide at 0.5% concentration may be protective against long-term HDBR induced processing speed deficits. However, the combination of HDBR + CO2 may exacerbate the HDBR-associated degradation of multisensory integration. Interestingly, exposure to HDBR + CO2 elicited differential physiological responses across individuals, resulting in a subgroup that exhibited signs of SANS. Moreover, we observed differential performance profiles between SANS and NoSANS subgroups, where SANS individuals showed an increased reliance on visual cues and a shift in speed-accuracy trade off. Although these results were obtained with a small sample, they suggest that SANS may negatively impact mission relevant performance. Furthermore, as this study shares implications for home-bound patients with air quality or respiratory concerns, the information and benefits resulting from this project may be far-reaching.
Data Availability Statement
The datasets generated for this study are available on request to the corresponding author.
Ethics Statement
The studies involving human participants were reviewed and approved by the Ärztekammer Nordrhein as well as the University of Florida and NASA Institutional Review Boards. The patients/participants provided their written informed consent to participate in this study.
Author Contributions
RS, AM, and JB designed the study and secured the funding. JL, YD, and IK contributed to the data collection and analyses. JL drafted the manuscript and all authors participated in editing.
Funding
This work was supported by the NASA grant #80NSSC17K0021, National Space Biomedical Research Institute (NASA NCC 9–58 and NSBRI SA02802) NASA’s Flight Analogs Project, and the National Institutes of Health and National Center for Advancing Translational Sciences, 1UL1RR029876-01.
Conflict of Interest
The authors declare that the research was conducted in the absence of any commercial or financial relationships that could be construed as a potential conflict of interest.
The reviewer RG declared a past co-authorship with the authors YD, IK, AM, JB, and RS to the handling Editor.
Acknowledgments
The authors gratefully acknowledge Dr. Edwin Mulder and the VaPER study staff for their support throughout the duration of the project.
Supplementary Material
The Supplementary Material for this article can be found online at: https://www.frontiersin.org/articles/10.3389/fnhum.2019.00355/full#supplementary-material
References
Allen, J. G., MacNaughton, P., Cedeno-Laurent, J. G., Cao, X., Flanigan, S., Vallarino, J., et al. (2018). Airplane pilot flight performance on 21 maneuvers in a flight simulator under varying carbon dioxide concentrations. J. Expo. Sci. Environ. Epidemiol. 29, 457–468. doi: 10.1038/s41370-018-0055-8
Allen, J. G., MacNaughton, P., Satish, U., Santanam, S., Vallarino, J., and Spengler, J. D. (2015). Associations of cognitive function scores with carbon dioxide, ventilation, and volatile organic compound exposures in office workers: a controlled exposure study of green and conventional office environments. Environ. Health Perspect. 124, 805–812. doi: 10.1289/ehp.1510037
Basner, M., Nasrini, J., Hermosillo, E., McGuire, S., Dinges, D. F., Moore, T. M., et al. (2017). Effects of- 12° head-down tilt with and without elevated levels of CO2 on cognitive performance: the SPACECOT study. J. Appl. Physiol. 124, 750–760. doi: 10.1152/japplphysiol.00855.2017
Basner, M., Nasrini, J., Hermosillo, E., McGuire, S., Dinges, D. F., Moore, T. M., et al. (2018). Effects of -12 degrees head-down tilt with and without elevated levels of CO2 on cognitive performance: the SPACECOT study. J. Appl. Physiol. 124, 750–760. doi: 10.1152/japplphysiol.00855.2017
Bhogal, A. A., Philippens, M. E., Siero, J. C., Fisher, J. A., Petersen, E. T., Luijten, P. R., et al. (2015). Examining the regional and cerebral depth-dependent BOLD cerebrovascular reactivity response at 7T. Neuroimage 114, 239–248. doi: 10.1016/j.neuroimage.2015.04.014
Black, F. O. (2001). What can posturography tell us about vestibular function? Ann. N. Y. Acad. Sci. 942, 446–464. doi: 10.1111/j.1749-6632.2001.tb03765.x
Bock, O., Abeele, S., and Eversheim, U. (2003). Sensorimotor performance and computational demand during short-term exposure to microgravity. Aviat. Space Environ. Med. 74, 1256–1262.
Bock, O., Weigelt, C., and Bloomberg, J. J. (2010). Cognitive demand of human sensorimotor performance during an extended space mission: a dual-task study. Aviat. Space Environ. Med. 81, 819–824. doi: 10.3357/asem.2608.2010
Bronstein, A. M. (1999). The interaction of otolith and proprioceptive information in the perception of verticality: the effects of labyrinthine and CNS disease. Ann. N. Y. Acad. Sci. 871, 324–333. doi: 10.1111/j.1749-6632.1999.tb09195.x
Cassady, K., Koppelmans, V., Reuter-Lorenz, P., De Dios, Y., Gadd, N., Wood, S., et al. (2016). Effects of a spaceflight analog environment on brain connectivity and behavior. Neuroimage 141, 18–30. doi: 10.1016/j.neuroimage.2016.07.029
Clement, G., Skinner, A., and Lathan, C. (2013). Distance and size perception in astronauts during long-duration spaceflight. Life (Basel) 3, 524–537. doi: 10.3390/life3040524
Ekstrom, R. B., French, J. W., Harman, H. H., and Dermen, D. (1976). Manual for Kit of Factor-Referenced Cognitive Tests. Princeton, NJ: Educational testing service.
Ernst, M. O., and Bulthoff, H. H. (2004). Merging the senses into a robust percept. Trends Cogn. Sci. 8, 162–169. doi: 10.1016/j.tics.2004.02.002
Fisk, W. J., Satish, U., Mendell, M. J., Hotchi, T., and Sullivan, D. (2013). Is CO2 an indoor pollutant? Higher levels of CO2 may diminish decision making performance. ASHRAE J. 55:LBNL-6148E.
Guyenet, P. G., Stornetta, R. L., and Bayliss, D. A. (2010). Central respiratory chemoreception. J. Comp. Neurol. 518, 3883–3906. doi: 10.1002/cne.22435
Hargens, A. R., and Richardson, S. (2009). Cardiovascular adaptations, fluid shifts, and countermeasures related to space flight. Respir. Physiol. Neurobiol. 169(Suppl. 1), S30–S33. doi: 10.1016/j.resp.2009.07.005
Hargens, A. R., and Vico, L. (2016). Long-duration bed rest as an analog to microgravity. J. Appl. Physiol. 120, 891–903. doi: 10.1152/japplphysiol.00935.2015
Isableu, B., Gueguen, M., Fourré, B., Giraudet, G., and Amorim, M.-A. (2008). Assessment of visual field dependence: comparison between the mechanical 3D rod-and-frame test developed by Oltman in 1968 with a 2D computer-based version. J. Vestib. Res. 18, 239–247.
Kanas, N., and Manzey, D. (2008). Space Psychology and Psychiatry. Berlin: Springer Science & Business Media.
Kelly, T. H., Hienz, R. D., Zarcone, T. J., Wurster, R. M., and Brady, J. V. (2005). Crewmember performance before, during, and after spaceflight. J. Exp. Ana. Behav. 84, 227–241. doi: 10.1901/jeab.2005.77-04
Koppelmans, V., Erdeniz, B., De Dios, Y. E., Wood, S. J., Reuter-Lorenz, P. A., Kofman, I., et al. (2013). Study protocol to examine the effects of spaceflight and a spaceflight analog on neurocognitive performance: extent, longevity, and neural bases. BMC Neurol. 13:205. doi: 10.1186/1471-2377-13-205
Koppelmans, V., Mulavara, A. P., Yuan, P., Cassady, K. E., Cooke, K. A., Wood, S. J., et al. (2015). Exercise as potential countermeasure for the effects of 70 days of bed rest on cognitive and sensorimotor performance. Front. Syst. Neurosci. 9:121. doi: 10.3389/fnsys.2015.00121
Koppelmans, V., Pasternak, O., Bloomberg, J. J., De Dios, Y. E., Wood, S. J., Riascos, R., et al. (2017). Intracranial fluid redistribution but no white matter microstructural changes during a spaceflight analog. Sci. Rep. 7:3154. doi: 10.1038/s41598-017-03311-w
Kopplemans, V., Bloomberg, J. J., De Dios, Y. E., Wood, S. J., Reuter-Lorenz, P. A., Kofman, I. S., et al. (2017). Brain plasticity and sensorimotor deterioration as a function of 70 days head down tilt bed rest. PLoS One 2:8. doi: 10.1371/journal.pone.0182236
Kornilova, L. N. (1997b). Vestibular function and sensory interaction in altered gravity. Adv. Space Biol. Med. 6, 275–313. doi: 10.1016/s1569-2574(08)60087-8
Lackner, J. R., and DiZio, P. (1996). Motor function in microgravity: movement in weightlessness. Curr. Opin. Neurobiol. 6, 744–750. doi: 10.1016/s0959-4388(96)80023-7
Laurie, S. S., Macias, B. R., Dunn, J. T., Young, M., Stern, C., Lee, S. M. C., et al. (2019). Optic disc edema after 30 days of strict head-down tilt bed rest. Ophthalmology 126, 467–468. doi: 10.1016/j.ophtha.2018.09.042
Law, J., Van Baalen, M., Foy, M., Mason, S. S., Mendez, C., Wear, M. L., et al. (2014). Relationship between carbon dioxide levels and reported headaches on the international space station. J. Occup. Environ. Med. 56, 477–483. doi: 10.1097/JOM.0000000000000158
Lee, A. G., Tarver, W. J., Mader, T. H., Gibson, C. R., Hart, S. F., and Otto, C. A. (2016). Neuro-ophthalmology of space flight. J. Neuro. Ophthalmol. 36, 85–91. doi: 10.1097/wno.0000000000000334
Lee, J. K., Koppelmans, V., Riascos, R. F., Hasan, K. M., Pasternak, O., Mulavara, A. P., et al. (2019). Spaceflight-associated brain white matter microstructural changes and intracranial fluid redistribution. JAMA Neurol. 76, 412–419. doi: 10.1001/jamaneurol.2018.4882
Lopez, C., Lacour, M., Léonard, J., Magnan, J., and Borel, L. (2008). How body position changes visual vertical perception after unilateral vestibular loss. Neuropsychologia 46, 2435–2440. doi: 10.1016/j.neuropsychologia.2008.03.017
Mader, T. H., Gibson, C. R., Pass, A. F., Kramer, L. A., Lee, A. G., Fogarty, J., et al. (2011). Optic disc edema, globe flattening, choroidal folds, and hyperopic shifts observed in astronauts after long-duration space flight. Ophthalmology 118, 2058–2069. doi: 10.1016/j.ophtha.2011.06.021
Manzey, D., and Lorenz, B. (1998). Joint NASA-ESA-DARA Study. Part three: effects of chronically elevated CO2 on mental performance during 26 days of confinement. Aviat. Space Environ. Med. 69, 506–514.
Miller, C. A., Kofman, I. S., Brady, R. R., May-Phillips, T. R., Batson, C. D., Lawrence, E. L., et al. (2018). Functional task and balance performance in bed rest subjects and astronauts. Aerosp. Med. Hum. Perform. 89, 805–815. doi: 10.3357/AMHP.5039.2018
Mulavara, A., Peters, B. T., Miller, C. A., Kofman, I. S., Reschke, M. F., Taylor, L. C., et al. (2018). Physiological and functional alterations after spaceflight and bedrest. MSSE 50, 1961–1980. doi: 10.1249/mss.0000000000001615
Mulavara, A. P., Feiveson, A. H., Fiedler, J., Cohen, H., Peters, B. T., Miller, C., et al. (2010). Locomotor function after long-duration space flight: effects and motor learning during recovery. Exper. Brain Res. 202, 649–659. doi: 10.1007/s00221-010-2171-0
Mulder, E., Linnarsson, D., Paloski, W., Rittweger, J., Wuyts, F., Zange, J., et al. (2014). Effects of five days of bed rest with and without exercise countermeasure on postural stability and gait. J. Musculoskelet. Neuronal Interact. 14, 359–366.
Nair, M., Mulavara, A. P., Bloomberg, J. J., Sangi-Haghpeykar, H., and Cohen, H. S. (2018). Visual dependence and spatial orientation in benign paroxysmal positional vertigo. J. Vestib. Res. 27, 279–286. doi: 10.3233/VES-170623
Nyborg, H. (1974). A method for analysing performance in the rod-and-frame test. Scand. J. Psychol. 15, 119–123. doi: 10.1111/j.1467-9450.1974.tb00563.x
Reschke, M. F., Bloomberg, J. J., Harm, D. L., Paloski, W. H., Layne, C., and McDonald, V. (1998). Posture, locomotion, spatial orientation, and motion sickness as a function of space flight. Brain Res. Brain Res. Rev. 28, 102–117. doi: 10.1016/s0165-0173(98)00031-9
Reschke, M. F., Bloomberg, J. J., Paloski, W. H., Mulavara, A. P., Feiveson, A. H., and Harm, D. L. (2009). Postural reflexes, balance control, and functional mobility with long-duration head-down bed rest. Aviat. Space Environ. Med. 80(5 Suppl.), A45–A54.
Roberts, D. R., Albrecht, M. H., Collins, H. R., Asemani, D., Chatterjee, A. R., Spampinato, M. V., et al. (2017). Effects of spaceflight on astronaut brain structure as indicated on MRI. New Engl. J. Med. 377, 1746–1753. doi: 10.1056/NEJMoa1705129
Rypma, B., Berger, J. S., Prabhakaran, V., Bly, B. M., Kimberg, D. Y., Biswal, B. B., et al. (2006). Neural correlates of cognitive efficiency. Neuroimage 33, 969–979. doi: 10.1016/j.neuroimage.2006.05.065
Satish, U., Mendell, M. J., Shekhar, K., Hotchi, T., Sullivan, D., Streufert, S., et al. (2012). Is CO2 an indoor pollutant? Direct effects of low-to-moderate CO2 concentrations on human decision-making performance. Environ. Health Perspect. 120:1671. doi: 10.1289/ehp.1104789
Shepard, S., and Metzler, D. (1988). Mental rotation: effects of dimensionality of objects and type of task. J. Exp. Psychol. 14:3. doi: 10.1037//0096-1523.14.1.3
Sliwka, U., Krasney, J. A., Simon, S. G., Schmidt, P., and Noth, J. (1998). Effects of sustained low-level elevations of carbon dioxide on cerebral blood flow and autoregulation of the intracerebral arteries in humans. Aviat. Space Environ. Med. 69, 299–306.
Tiffin, J., and Asher, E. J. (1948). The Purdue Pegboard: norms and studies of reliability and validity. J. Appl. Psychol. 32:234. doi: 10.1037/h0061266
Van Dongen, S. (1999). The statistical analysis of fluctuating asymmetry: REML estimation of a mixed regression model. J. Evol. Biol. 12, 94–102. doi: 10.1046/j.1420-9101.1999.00012.x
Welch, R. B., Hoover, M., and Southward, E. F. (2009). Cognitive performance during prismatic displacement as a partial analogue of “space fog”. Aviat. Space Environ. Med. 80, 771–780. doi: 10.3357/asem.2415.2009
Willey, C. R., and Jackson, R. E. (2014). Visual field dependence as a navigational strategy. Atten. Percept. Psychophys. 76, 1036–1044. doi: 10.3758/s13414-014-0639-x
Witkin, H. A., Lewis, H. B., Hertzman, M., Machover, K., Meissner, P. B., and Wapner, S. (1954). Personality Through Perception: An Experimental and Clinical Study. Oxford: Harper.
Yuan, P., Koppelmans, V., Reuter-Lorenz, P., De Dios, Y., Gadd, N., Wood, S., et al. (2018). Vestibular brain changes within 70 days of head down bed rest. Hum. Brain Mapp. 39, 2753–2763. doi: 10.1002/hbm.24037
Keywords: cognition, sensorimotor, CO2, bed rest, spaceflight, SANS (Spaceflight Associated Neuro-ocular Syndrome)
Citation: Lee JK, De Dios Y, Kofman I, Mulavara AP, Bloomberg JJ and Seidler RD (2019) Head Down Tilt Bed Rest Plus Elevated CO2 as a Spaceflight Analog: Effects on Cognitive and Sensorimotor Performance. Front. Hum. Neurosci. 13:355. doi: 10.3389/fnhum.2019.00355
Received: 22 June 2019; Accepted: 23 September 2019;
Published: 17 October 2019.
Edited by:
Peter Sörös, University of Oldenburg, GermanyReviewed by:
Claudia Altamura, Campus Bio-Medico University, ItalyRahul Goel, Baylor College of Medicine, United States
Copyright © 2019 Lee, De Dios, Kofman, Mulavara, Bloomberg and Seidler. This is an open-access article distributed under the terms of the Creative Commons Attribution License (CC BY). The use, distribution or reproduction in other forums is permitted, provided the original author(s) and the copyright owner(s) are credited and that the original publication in this journal is cited, in accordance with accepted academic practice. No use, distribution or reproduction is permitted which does not comply with these terms.
*Correspondence: Rachael D. Seidler, cmFjaGFlbHNlaWRsZXJAdWZsLmVkdQ==