- 1Joint and Operational Analysis Division, Defence Science and Technology Group, Edinburgh, SA, Australia
- 2Institute of Integrated and Intelligent Systems, Griffith University, Nathan, QLD, Australia
Transcranial direct current stimulation (tDCS) is a non-invasive brain stimulation technique which provides unique potential to directly improve human capability on a temporary, at needs, basis. The purpose of this paper is to consider the utility of tDCS through analysis of the potential risks and benefits in the context of defence service personnel. First, we look at the potential benefits, focusing primarily on warfighter survivability and enriching cognition quality in support of command and control. Second, we look at the potential detriments to tDCS military use, focusing on adverse effects, safety considerations, and risk. Third, we examine how the level of risk can be mitigated through military doctrine development focusing on safety parameters and exclusion criteria. Finally, we explore the future prospects of military tDCS use, particularly in terms of addressing gaps in the literature so that tDCS can be used ethically and efficaciously at the level of individual personnel.
Introduction
The aspiration to increase cognitive and physical performance to foster protection from predators is nothing new. The boundaries of human performance have been tested and pushed through methods such as physical training, education, technology, and religion, for centuries (Levasseur-Moreau et al., 2013). More recently, evidence has emerged that aspects of cognition and motor performance can be enhanced through a technique called transcranial direct current stimulation (tDCS). tDCS is a non-invasive1 technique that modulates cortical tissue excitability, increasing or decreasing cerebral activity by applying a very low direct current (usually no more than 2 mA; 0.06 mA/cm2 over a 35 cm2 pad) from electrodes placed on the scalp. Approximately half of the current delivered to the head reaches and stimulates the brain (Dymond et al., 1975). Research has shown a wide range of cognitive effects, mainly dependent on the site and polarity of stimulation (the montage), and duration and pattern of current flow (the protocol). Generally brain function is increased under the positive anode; with either little effect or decreased function under the cathodal placement site (Jacobson et al., 2012). tDCS does not directly cause or block the firing of neurons; the immediate effects are thought to be a result of polarity-specific shifts in resting membrane potential (Sparing and Mottaghy, 2008). Enhancement effects of tDCS last beyond the duration of stimulation and can be detected up to 24 h after stimulation (depending on the montage, protocol, and cognitive aspect tested; Utz et al., 2010). The mechanism for these enduring effects are thought to be a result of long-term potentiation and long-term depression of neuronal synapses (Nitsche et al., 2008).
The utility of cognitive enhancement techniques have been examined by a number of authors, and non-invasive brain stimulation has been found to offer significant socioeconomic benefits, such as increasing productivity and growth (Buchanan, 2008), reducing inequality (Hai and Heckman, 2017), and encouraging social justice (Savulescu, 2006). However, these outcomes have little relevance in the context of enhancement for the military purposes considered in this paper. The utility of cognitive enhancement is different in military situations, as benefits must be weighed against the potential harms in circumstances where the ability to complete objectives safely and make better decisions can mean the difference between life and death (Wolfendale, 2008; McKinley et al., 2012).
The main purpose of this paper is to examine whether tDCS use can be ethical and efficacious in the enhancement of military personnel. First, an overview of the potential benefits of tDCS in terms of expanding warfighter-level capability and command and control decision making is provided. This is followed by a discussion of the potential harms the technology may produce for the military, including safety issues, adverse effects, risk of hitherto unobserved and undetected effects, risk of self-administration by military personnel, and how these issues represent a threat to military capability. Additionally, we examine how some of these risks can be mitigated. While it is not the purpose of the paper to propose a comprehensive set of guidelines covering military tDCS use, a number of recommendations are provided that can inform future doctrine development, including safety parameters and exclusion criteria. This section provides a frame of reference for the assumptions that are applied to the discussion of safety and risk. Finally, we identify the future prospects of tDCS use in the military, focusing on gaps in the existing literature which need to be addressed so that tDCS can be delivered ethically and efficaciously, and with the predictable and reliable individual-level effects that are required so that harm to military capability can be avoided.
Value to Defence
National security involves a number of aspects of military defence, including warfighting, activity in preparation for war, and measures to prevent war (Paleri, 2008). While the core fundamental skills of soldiering have remained stable over time (movement, communication, and operating weapons; United States Department of the Army, 2005), the full gamut of defence activities has become increasingly complex. Seemingly straightforward skills must be coordinated and performed under great stress in dynamic, demanding, and unpredictable environments2. Conceptually, coordinated military action is achieved via three core elements of command and control: capability, which is utilised to achieve intent, given awareness (Lambert and Scholz, 2007). The rapid development of equipment, weapons, and systems means that warfighters operate in increasingly complex environments (Redmore, 2009), and high quality cognition is required in support of command and control (e.g., van Creveld, 1985; Storr, 2009). However, ever-increasing decision speed and data volume processing requirements are becoming progressively more mismatched with current human capabilities (United States Air Force Chief Scientist, 2010), and the ability to perform at the necessarily high level is hindered by the fact that every aspect of cognitive performance is degraded under the stress of combat conditions (Lieberman et al., 2005). In this section, the potential of tDCS to bridge this gap by supporting warfighter command and control is explored across the three core areas; achieving situational awareness, the formation (and communication) of intent, and capability.
The ability to make quick assessments of complex and rapidly changing situations is vital across all service branches and ranks, so maintaining situational awareness is an essential prerequisite of performing tasks in real-time (Tirre and Gugerty, 1999). A review conducted by Hartel et al. (1991) concluded that situational awareness deficits were the primary cause of military aviation related incidents, and Murray et al. (2010) attributed the majority of critical incidents involving naval Marine Corps infantry to failures of perception regarding consequential environmental or situational features. As presented in Table 1, there are signs that tDCS could improve a number of cognitive aspects relating to the attainment and maintenance of situation awareness. As well as the functioning of the perceptual senses (e.g., visual and auditory) themselves, attention and working memory are critical factors underlying in the acquisition of information in support of situational awareness (Ribeaux and Poppleton, 1978; Endsley, 1995).
Coordinated action must be achieved through the establishment of common intent (Pigeau and McCann, 2000). Commanders are tasked with making sound judgments and decisions while facing complex problems in unpredictable situations (Crabbe, 2000), so highly sophisticated cognitive skills are required to support commanders’ ability to form achievable intent (Allen and Gerras, 2009). Increasingly, decision-making authority is dispersed to lower ranks as increasing warfare dynamism requires a higher level of decision making immediacy to exploit time-limited tactical opportunities (i.e., “mission command”; Joint Chiefs of Staff, 2012; Australian Army, 2017). This shift towards decentralisation of command initiative means that the levels of command are unavoidably blurred (Pigeau and McCann, 2000), so the requirement to make sound decision-making is increasingly applicable down the chain. tDCS has shown the potential to improve a number of aspects of cognition relevant to the formulation of intent, including planning, problem solving, probabilistic assessment, and acceptance of risk3. Once intent is reached, it must be shared through effective and efficient communication in order to achieve coordinated action (Pigeau and McCann, 2000). As shown in Table 2, tDCS has shown potential in both the formation and communication of intent.
The third component of the trinity of elements associated with achieving coordinated action (Lambert and Scholz, 2007) is capability. Warfighters need sufficient and specific levels of mental and physical preparedness to be capable of carrying out commanders’ intent (Royal Australian Air Force, 2017; Australian Army, 2018), and tDCS shows promise in a number of areas related to the capability of individual warfighters, including physical competence, training and education, and resilience. The areas of tDCS which show promise in assisting personnel to increase their capability to complete demanding undertakings while managing physical and psychological stressors are presented in Table 3.
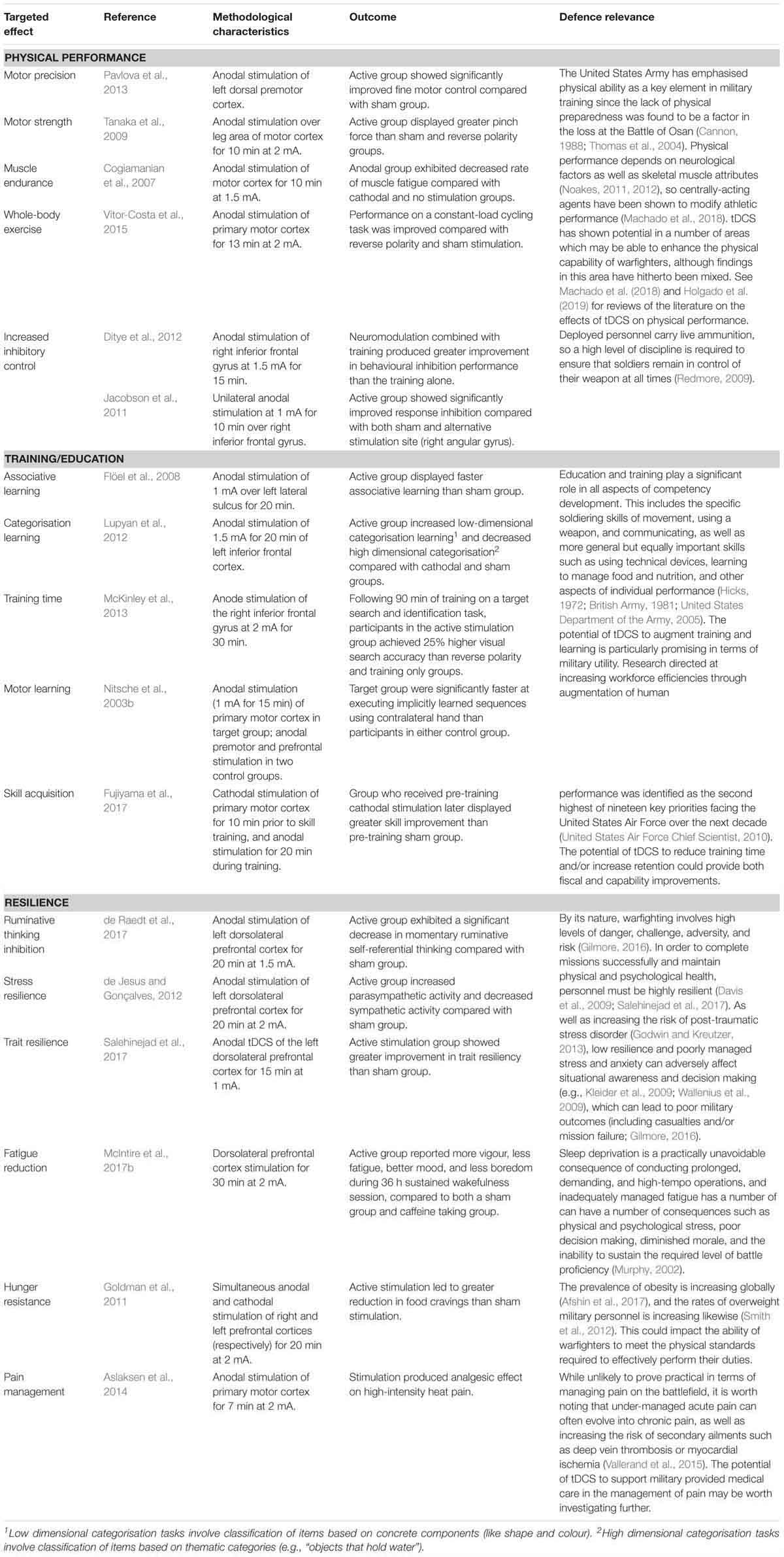
Table 3. The potential of tDCS in readying military personnel for the physical and psychological requirements and stressors of warfighting.
Risks
There has been a great deal of research on the safety of tDCS which indicates that the technique is broadly safe, and does not cause permanent or severe damage or discomfort when used according to appropriate guidelines (such as those suggested in the Risk Mitigation section of the present paper). In a meta-analysis conducted by Brunoni et al. (2011) which examined the effects of tDCS in humans in every available paper from 1998 to 2010, no serious or ongoing side effects were reported. However, tDCS is not entirely risk-free. The technique is still relatively new, and has been associated with mild and transient discomfort in some people, including reported tingling, itching, and burning sensations under the electrodes.
The most common adverse effect of tDCS is a mild burning/itching sensation at the point of contact with the electrodes (Been et al., 2007). Light itching under the stimulation electrodes occurs in approximately 30% of cases (Poreisz et al., 2007). Although sensory side effects are reasonably common, severity is typically low (Kessler et al., 2012). Falcone et al. (2012) conducted a study in which participants rated each common sensation on a 10 point Likert scale, where 1 indicated no sensation and 10 indicated extreme sensation. Mean scores in the active condition for itching, heat, and tingling were 1.81, 1.44, and 2.51, respectively. The most serious adverse effects were two reported cases of skin lesions following repeated tDCS sessions (Palm et al., 2008; Frank et al., 2010). The authors suggested that an accumulation of impurities from tap water on the electrodes caused the reaction. The risk of both skin lesions and itching/burning sensations can be minimised by soaking the connecting sponges in sodium chloride (saline) solution rather than water before commencement of stimulation (DaSilva et al., 2011).
Mild headaches are sometimes observed. They are generally brief and are more commonly reported by frequent headache sufferers (e.g., 3.9% in healthy subjects vs. 11.1% in migraine patients; Poreisz et al., 2007). Some studies have reported a higher incidence of headaches in sham conditions than active stimulation conditions (14.8% active vs. 16.2% sham; Brunoni et al., 2011), though these findings have not been consistent across studies (e.g., Kessler et al., 2012). The extent to which headaches are a direct effect of tDCS is unclear, with secondary factors (such as pressure from the strap that keeps the electrodes in place) likely also playing a role. Nausea has been also experienced within 2 h of tDCS, though such reports are infrequent (less than 3% of participants; Poreisz et al., 2007).
Fatigue is sometimes reported by participants following tDCS studies. For example, Brunoni et al. (2011) found that a small to moderate level of fatigue was experienced by 35.3% of participants. However, it is not clear whether fatigue is directly caused by the stimulation itself, or is the result of a secondary cause such as an increase in concentration to complete the tasks that are often completed in conjunction with tDCS (i.e., what is being measured by the particular study; often problem solving related tasks), or by an increase in nervous energy experienced by participants undergoing an unfamiliar technique involving wires, straps, and electrodes. Conversely, there is evidence that particular stimulation montages can in fact reduce fatigue (e.g., McIntire et al., 2017b).
Overall, transient minor discomfort is present in about 10.4–17.7% of participants (Poreisz et al., 2007; Brunoni et al., 2011); not acute enough to cause subjects to request early termination of stimulation. Nevertheless, all documented adverse effects need to be considered and managed in any military tDCS programme. It is the view of the current authors that since all documented harms are either minor or can be mitigated, there is no case for dismissing military tDCS use on the basis of reported adverse effects. However, the potential for delayed and/or unknown harms also need to be considered.
Given the relative recency of tDCS, it is possible that hitherto unencountered effects could later emerge in some people. Long-term risk falls broadly into two categories: delayed effects that emerge after short term use, and cumulative harm following prolonged use. Although tDCS has only become heavily researched in recent years, the possibility of delayed adverse effects is somewhat mitigated by the fact that various forms of direct current have been used for over 100 years (e.g., for the treatment of melancholy in 1804; Fox, 2011), yet no delayed effects have been documented to date. tDCS has been heavily studied over the last decade, and although it is not clear whether it may cause negative consequences that take more than 10 years to materialise, there is no evidence to suggest this is the case. Risks associated with prolonged use are not well understood either. Given evidence that repetitive daily tDCS can lead to lasting long-term targeted changes (Agnew and McCreery, 1987), it follows that repetitive tDCS could cause long-lasting detrimental effects. Overall, little is known about either form of long-term risk, but there is currently no evidence that tDCS causes long-term damage when used within appropriate guidelines.
It is currently unclear how cognitive functioning in non-target areas is effected by tDCS. The precise path the electrical current follows is not fully understood (McKinley et al., 2012) and cannot easily be controlled, so brain regions interconnected to the target area under the electrodes could receive a portion of the current (Sadleir et al., 2010). Subsequent activations and deactivations associated with electrical stimulation can even occur in remote locations across both cerebral hemispheres (Utz et al., 2010). Moreover, activity in areas of the brain not directly under the electrodes may be reduced, creating an overall equilibrium between the cognitive areas of interest and other cognitive areas (Hilgetag et al., 2001). While most tDCS studies report enhancement of function, measures are generally limited to a specific area of cognition relating to the identified functional specialisation of the underlying brain region. Brem et al. (2014) describe a “net zero-sum model” in which the principle of conservation of energy governs the allocation of brain resources. This represents a risk in the military, where the possibility of unpredictable cognitive or physical deficits could make already dangerous military situations even riskier. More research employing multiple assessments across various areas of cognition needs to be conducted.
It is also unknown whether tDCS has the potential to be addictive. Because the neurotransmitter dopamine is involved in both the reward-related processes associated with addiction (Nutt et al., 2015) and high level cognitive functioning (e.g., Nieoullon, 2002; Cools, 2011), many forms of cognitive enhancement have been found to be addictive (such as central nervous system stimulants; Dackis and Gold, 2008). There is some limited evidence associating tDCS with altered dopamine levels (e.g., Fonteneau et al., 2018), but it is unclear whether this association is a result of direct dopaminergic modulation (such as is the case with stimulants like amphetamines and modafinil; Fleckenstein et al., 2007; Volkow et al., 2009). The size of dopaminergic effect4 appears to be more aligned with indirect dopamine concentrations5 than with drugs that directly increase dopamine levels in the reward system6, so the effect of tDCS on dopamine levels appears subtle. No issues with addiction have hitherto been reported in the literature, and the enduring effects of tDCS across multiple sessions appear more disposed towards accumulation of effect than development of tolerance (Alonzo et al., 2012). While the extant evidence does not suggest addictive potential, addiction is a multidimensional occurrence that cannot be predicted by any single neurological (Sinha, 2011) or psychosocial (Nation and Heflinger, 2006) variable, so the possibility of risk cannot be discarded. More research is required in this area; particularly given the significantly increased likelihood towards developing addiction observed in military populations (e.g., Lande et al., 2008; Kelsall et al., 2015).
The fact that techniques like tDCS are described as ‘non-invasive’ may produce the misleading impression that improper administration is less problematic or dangerous than overtly internal forms of enhancement7. tDCS has been described in the popular press, and there are several do-it-yourself (DIY) websites describing how to build (poor quality) tDCS apparatuses. All that is needed to build a device at home is a 9v battery, around $40 (US) of electronic parts, and instructions available through forums and dedicated websites (Fitz and Reiner, 2015). DIY brain stimulation is becoming so common that governmental bodies have begun issuing warnings on the dangers of using unapproved devices purchased through the internet (e.g., California Department of Public Health, 2013). It can therefore be expected that military personnel may be interested in self-administration using machines made at home or purchased online. This type of use carries a greater level of risk due to the lack of essential safety features, component redundancy, safe administration guidelines, and expert knowledge of appropriate montage and protocol setup that would be present in professional settings. Anecdotes of serious and permanent side effects have been described in online discussion forums, even including loss of consciousness (Dcminsf, 2014; Packmanta, 2014). Self-administration increases the risk of inappropriate or dangerous montages. For example, in one extreme (but isolated) case, direct current was used to stimulate the brainstem, leading to a number of serious (albeit temporary) adverse effects including disturbed breathing, speech arrest and psychosis (Lippold and Redfearn, 1964). Additionally, self-administration could cause additional risk in military situations where unanticipated or inconsistent effects could have considerable consequences for the capability of warfighters and their ability to properly complete their mission objectives.
Risk Mitigation
It is important to note that with the exception of DIY tDCS, the risks and safety issues discussed in the previous section are underpinned by assumptions about how tDCS is delivered. As well as providing a frame of reference to make these assumptions explicit, this section serves the second purpose of providing guidance on how tDCS guidelines ought to be developed to ensure that tDCS can be delivered safely and efficaciously in military environments. Specifically, this section explores general safety procedures and screening criteria for personnel in which tDCS may be contraindicated.
While tDCS is generally safe, it has been found to cause permanent damage in animal studies when high levels of current were used. There is a risk of causing tissue damage as the electrical stimulation begins to heat brain tissue (Agnew and McCreery, 1987). In a study involving rats, Liebetanz et al. (2009) found that brain lesions formed when current densities exceeded 143 A/m2. Studies and treatment involving humans typically use current less than 0.0057 A/m2 (2 mA through a 35 cm2 pad). While there is some variation in the current limits recommended by key papers on the topic, most recommendations fall within the 1.5–3 mA range. For military operational stimulation, we recommend a current density limit of 2 mA (four orders of magnitude less than the current densities shown to cause lesions in rats). For military research purposes however, a limit of 1.5 mA may be more appropriate to ensure adequate participant blinding (Hummel and Cohen, 2005), since some participants are able to distinguish between active and sham stimulation at intensities at or above 2 mA (O’Connell et al., 2012). Medically certified tDCS devices are fitted with current limiting features, preventing accidental exposure to damaging current levels, and should be the only devices considered for military use. tDCS devices should also undergo regular electrical verification and validation to ensure the equipment is calibrated correctly, and continues to meet safety requirements and specifications.
Several papers within the current body of relevant literature have proposed universal tDCS safety standards and limits. For example, see Nitsche et al. (2003a) or Iyer et al. (2005) for discussion on general safety and limits, and DaSilva et al. (2011)8 for direct procedural guidelines on employing standardised tDCS protocol, including materials, measurements, skin preparation, electrode positioning, tDCS administration, and evaluation. There is currently no case for procedural guidelines covering stimulation in military contexts to deviate substantially from general guidelines, so we recommend that any military use of tDCS is consistent with guidelines and recommendations stipulated in the literature cited above.
In some studies, participants experienced temporary mood, personality and behavioural changes following tDCS. Several stimulation montages have been found to modulate relevant neural systems so as to affect a number of key aspects associated with social interaction and empathy, including resonance, self-other discrimination, and mentalisation (Hétu et al., 2012). Additionally, tDCS is known to have other effects on personality and behaviour in areas such as emotion (e.g., emotional aspects associated with pain; Maeoka et al., 2012), honesty (e.g., lying and deception; Priori et al., 2008), mood (e.g., depression/sadness; Ferrucci et al., 2009), comfort with risk (e.g., risk-taking and reward seeking behaviour; Fecteau et al., 2007), and even personal ethics and morality (e.g., judgments on the moral permissibility of attempted harms; Young et al., 2010). Influencing mood, personality and behaviour is not trivial. As put by Hamilton et al. (2011, p. 189), “tDCS can transiently alter an individual’s understanding of and relationship to others in ways that touch directly on the neural basis of ethical and moral thought and behaviour”. While it is worth noting that this type of stimulation could potentially be used beneficially in some circumstances, there are a number of ethical issues that need to be addressed that go beyond the intended scope of this paper. Regardless of ethical considerations, an unanticipated or unpredictable alteration of personality or behaviour represents a tangible threat to capability. Our current recommendation for military-based tDCS research, therefore, is that ongoing mood and personality should be closely monitored and reported, particularly in research areas involving novel and underreported electrode montages.
Another important factor to ensure safe delivery of tDCS concerns the personnel that are selected. tDCS affects participants with particular psychological, mood, and physiological conditions differently to healthy participants (Poreisz et al., 2007). Stringent exclusion criteria therefore need to be set out in military guidelines, covering both research and operational tDCS applications. Recommendations on exclusion criteria differ across key papers, and there is some variation on which criteria should be included. In the context of warfighting, the consequences of unpredictable or adverse outcomes go beyond the general human research principle of avoiding unnecessary distress and injury. In circumstances where adverse effects could prevent personnel from being able to perform their role to their full ability, the capability of the individual or unit may be at risk. In cases where contradictory recommendations exist, the more conservative approach should therefore generally be adopted.
Personnel should be excluded from tDCS if they have suffered from any skin diseases, have metallic implants near the electrodes, or suffer from any unstable medical condition or illness that could increase the risks of stimulation (Nitsche et al., 2003a). This includes (but is not limited to) neurological diseases, chronic eczema, and signs of epilepsy. Personnel taking medication known to affect the central nervous system should also be excluded, including narcoleptic and antiepileptic drugs, antidepressants, benzodiazepines and L-Dopa (Utz et al., 2010)9. Pregnancy is not a strict contraindication in tDCS research (DaSilva et al., 2011); however, we suggest that women who are pregnant or suspect they may be pregnant should be excluded as a matter of precaution. Participants with latent psychiatric illness (indicated by first degree relatives suffering from a psychotic disorder) should also be excluded (Meiron and Lavidor, 2013).
While there is nothing unique to military situations which require different safety guidelines to those used in therapeutic stimulation or cognitive enhancement of civilian populations, a different focus is required during screening processes. Conditions that are more prevalent in military personnel, such as post-traumatic stress disorder (Armenta et al., 2018), a history of addiction (Kelsall et al., 2015), or having metal implanted in the body (e.g., shrapnel; Gawande, 2004), should explicitly be stated and screened for. It should be noted that particular mental disorders are more prevalent in military personnel than in the wider civilian population. For example, a recent study found that 22% of Australian Defence Force members met ICD-10 (Tenth Revision of the International Statistical Classification of Diseases and Related Health Problems; World Health Organization, 1992) criteria for diagnosis of a mental health disorder at some point within the preceding 12 months period (van Hooff et al., 2014). Additionally, there is evidence that military personnel often do not disclose mental health issues due to perceptions of stigmatisation and career damage (e.g., Rüsch et al., 2017), so additional practical steps may be required in the military screening process. This would likely need to include a mechanism for impartial participant selection, such as encouraging potential stimulation recipients to consult with non-defence affiliated general practitioners, and acting on their eligibility recommendations without requiring justification or explanation (similar to some civilian pre-employment medical assessment procedures).
Finally, the risk of self-administration by defence personnel could be reduced through proactivity on the part of defence organisations. If tDCS were to be an area of active inquiry, military personnel could be involved in formal research rather than self-experimentation. If military research ultimately demonstrates that tDCS enhancement is worthwhile, the technology could then be implemented and administered safely, effectively, and ethically. If, however, it is concluded that the risks are too high and/or the benefits too variable, the informed position specific to military use will likely reduce (if not end) the temptation for private use by personnel. Either way, it would be beneficial for military organisations to have a concrete position on tDCS based on military-specific research.
Future Prospects
A number of significant gaps remain in the tDCS body of literature in areas relevant to military use. Despite the considerable volume of literature indicating the potential of tDCS to enhance performance in areas relevant to military organisations, the case for its utility is somewhat diminished by the fact that it is currently unclear how much of the literature can be generalised into military situations. For tDCS to be truly beneficial, more research needs to be conducted investigating the applied aspects of using tDCS in military environments. This can be broken down into five main areas; inter-individual differences, the generalisability of research involving general populations to military personnel, whether the effects sizes of tDCS are large enough to make any practical difference to military capability and mission outcomes, the generalisability of laboratory based research into operational environments, and how tDCS could fit into established military personnel enhancement programmes.
The effects of tDCS are subject to a high amount of inter-individual variability, so stimulation of identical brain regions (via presumed locations under the skull bone) can cause different effects for different people or in different situations. While some authors have noted the requirement of relatively predictable and repeatable outcomes for the majority of stimulated individuals to justify tDCS as a treatment device in clinical settings (e.g., de Berker et al., 2013; Wiethoff et al., 2014), this requirement is arguably even more important in the military. Given that military situations often have life or death consequences, mean (average) group-level improvements are insufficient where the performance of some warfighters is reduced. Aside from the ethical responsibility to ensure that warfighters are not impeded during high risk situations, the overall effectiveness of the military team could also be diminished where a capability reduction of a single member encumbers the rest of the team. There is, therefore, a strong argument that the ideal goal should be for everyone’s performance to increase, with a minimum requirement that no one goes backward. Consequently, military tDCS use should be tailored to individual personnel.
Individually tailored stimulation is likely to be very difficult to implement. The effects of stimulation are affected by many factors and there is currently no standard process for calculating the likely response to stimulation to optimise target effects for any specific individual. The benefits of stimulation are not uniform across any given montage/protocol, with outcomes dependent on complex interactions between stimulation configurations and inter-individual differences in anatomical and cognitive characteristics (Sarkar et al., 2014). A non-exhaustive list of factors that can influence the effects of tDCS on any given individual is presented in Table 4.
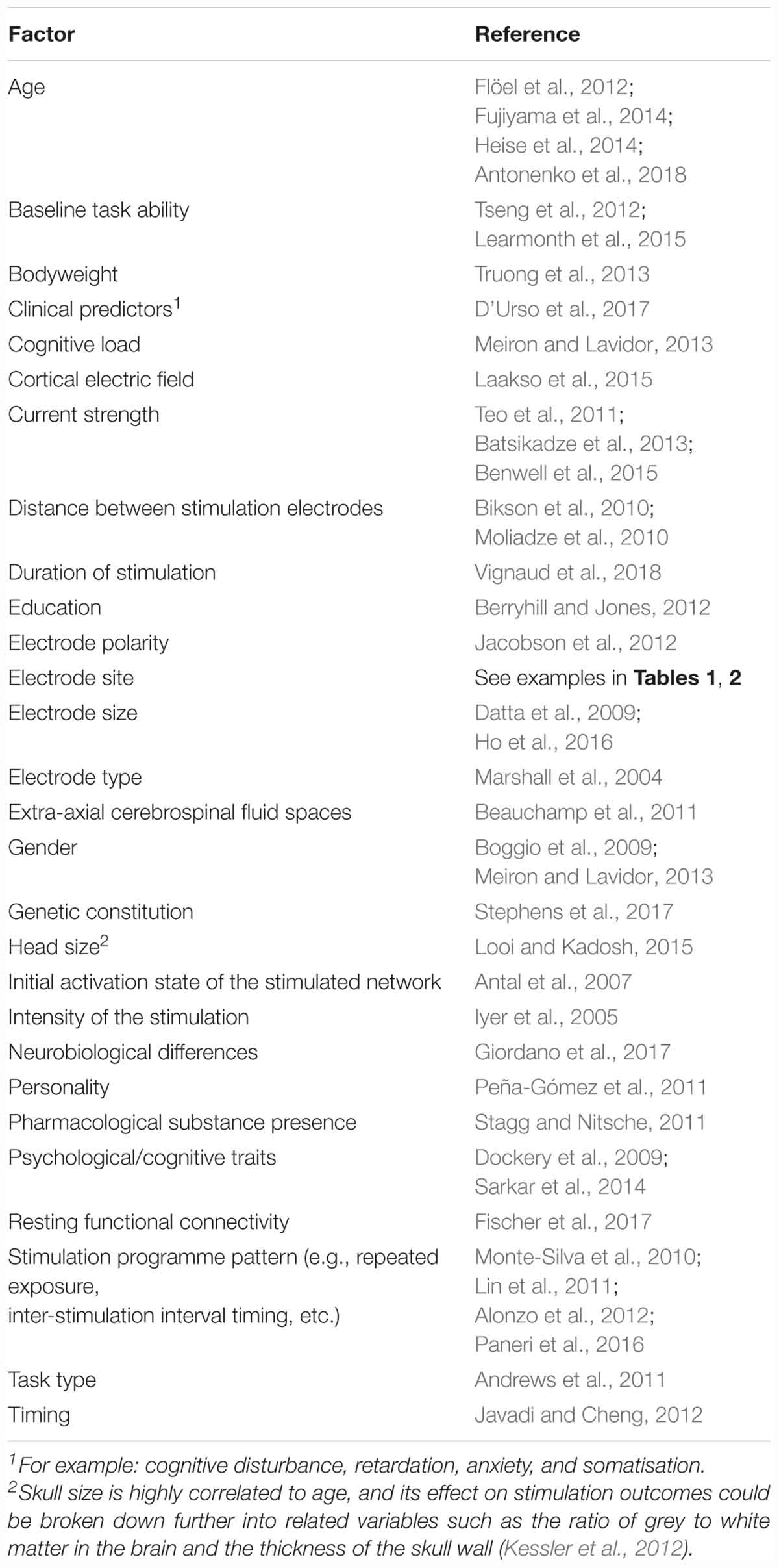
Table 4. Variables known to affect the cognitive, motor, and behavioural outcomes of tDCS (derived in part from Li et al., 2015, pp. 9–14).
As well as having a large number of variables to consider, the difficulty of predicting an individual’s response is further amplified by the fact that many of the variables interact in complex ways. The majority of relationships between factors and the enhancement (or diminution) effects are neither linear nor monotonic (Laakso et al., 2015). For example, even the basic notion that activity tends to increase under the anodal electrode and decrease (or remain stable) under the cathodal electrode depends on the targeted brain region (Jacobson et al., 2012). Additionally, the effects can sometimes be completely reversed by factors like current strength (Batsikadze et al., 2013), gender (Meiron and Lavidor, 2013), or by complex interactions between variables (e.g., polarity × current strength × baseline task performance level; Benwell et al., 2015).
We therefore argue that delivering stimulation to individual military personnel based on evidence of group-level effects may be inappropriate, and any stimulation programme would be better delivered in a way that ensures efficacy. However, factoring in such a large number of complex interacting variables for each individual is incredibly problematic, and there is a strong likelihood that there are other influencing variables that have yet to be realised given that very little research has been conducted on the variability of effects. One potential approach is to simply perform tDCS on participants during an assessment session, and then divide participants into groups based on their level of activation (e.g., Wiethoff et al., 2014; López-Alonso et al., 2015). Generally, around three quarters of participants who are identified as responding positively in an initial session are likely to respond likewise in a later session. For example, López-Alonso et al. (2015) found that of the 60% of participants identified as responders in an initial session, 78% responded correspondingly in the second stimulation session. Although the authors concluded that cortical excitability responses in an initial session can reliably predict the responses of 69%10 of participants in a subsequent session (López-Alonso et al., 2015), this kind of strategy is not sufficient to predict the effects of tDCS for all personnel in all situations. Response patterns to tDCS are not represented bimodally (Fujiyama et al., 2014), so a more nuanced method than dichotomous splitting is required.
Other researchers have adopted more neurological focused approaches, using neuroimaging and modelling techniques to predict current flow based on individuals’ unique physiology/anatomy. These techniques include magnetoencephalography (Garcia-Cossio et al., 2016), electroencephalography (Schestatsky et al., 2013), functional magnetic resonance imaging based neuronavigation (Looi and Kadosh, 2015), surface-based registration11 processing of electric fields (Laakso et al., 2015), human head simulations (Datta et al., 2011), and dynamic causal modelling12 of neural network activity (de Berker et al., 2013). While this research has demonstrated that neuroimaging based simulations can successfully be used to better understand individual differences and optimise tDCS montages for individual participants based on brain current flow predications, practical utilisation of such techniques appear to be prohibitively difficult and time consuming (Davis et al., 2013). Many of the mechanisms involved in the effects of tDCS on brain excitability remain inadequately understood or disputed. Further studies are necessary to elucidate the mode of action of tDCS and determine the best paradigm of stimulation depending on the goals (Roche et al., 2015). Predicting the effects of tDCS can therefore only be so accurate before the physiological mechanisms producing the enhancements are adequately understood. Therefore, this is an area where more research is required, both in terms of specific tDCS neuroimaging research, and fundamental research towards developing a more comprehensive neurophysiological understanding.
Another required area of investigation is to establish the validity of the known effects of tDCS in the military context. Although the question of the real-world applicability of research conducted in artificial laboratory environments is by no means unique to tDCS or the military, the issue is more pronounced in military environments where warfighters experience extreme conditions such as stress, fatigue, and neurophysiological effects associated with exposure to danger (Canli et al., 2007). This is important because the effects of tDCS can be directly influenced by the state of brain activation at the time of stimulation (Canli et al., 2007; Levasseur-Moreau et al., 2013). For the general body of tDCS literature to be relevant to the military, the effects of tDCS need to be tested under conditions closely mimicking real-world military situations so the impact of external variables (such as stressful and high fatigue situations) can be better understood. It is strongly possible that a set of military tasking can be identified in which tDCS should not be used because of the likelihood of negative unintended consequences. In particular, it is the view of the current authors that the use of tDCS in situations likely to trigger strong emotional responses (such as those involving deadly force) is inappropriate until more is known about the interactions between environmental stressors, individual differences, and the effects of stimulation.
Another issue that needs to be considered is whether enhancement effects are large enough to have any meaningful impact (Levasseur-Moreau et al., 2013). This problem is not unique to tDCS research, as the suitability of emphasising statistical significance over effect sizes has been questioned for some time (e.g., Carver, 1978). However, it is particularly relevant in the context of examining technology with inherent risks. tDCS research has typically focused on general population enhancement and clinical population rehabilitation, and few studies directly address whether the effect sizes are large enough to be valuable for military purposes. However, there is some evidence suggesting that particular areas of interest can indeed be affected by tDCS in a meaningful way. Consider the following example from Levasseur-Moreau et al. (2013, p. 8):
“Pascual-Leone et al. (2012) estimated a mean reduction of 32 ms from studies using NIBS [non-invasive brain stimulation] to improve motor RT [reaction times]. In the specific context of speed shooting performances, ∼13 ms would be the difference between elite and rookie police officers (Vickers and Lewinski, 2012). Therefore, an improvement of 32 ms may make a vital difference in the context of a one-on-one gunfight or during aircraft combat (dogfight).”
However, it remains unclear what effect pre-existing skill level has on the level of benefit gained through tDCS. Given that other forms of enhancement (such as training) are subject to diminishing returns in levels of improvement, it is likely that a similar tapering is present in tDCS enhancement. Members of elite level Special Forces units, for example, may not experience the same level of improvement in their already highly refined skill set. Further research is required to confirm the model of diminishing gains, and determine the situations in which the enhancement effects of tDCS are large enough to have practical significance outweighing potential costs and risks.
Finally, it is worth noting that many of the effects elicited by tDCS can be achieved through other enhancement strategies, such as cognitive training (e.g., Biggs et al., 2015), fitness interventions (e.g., Hillman et al., 2008), meditation (e.g., Lutz et al., 2008), mindfulness training (e.g., Zeidan et al., 2010), nutrition (e.g., Burkhalter and Hillman, 2011), and video game training (e.g., Green and Bavelier, 2003). Many of these methods are already being utilised in existing military training programmes and interventions (e.g., Burton et al., 2011; Mead, 2013; Aidman, 2017). For tDCS to be valuable in the context of military enhancement, it must produce positive effects that are superior, additive, multiplicative, or associated with less adverse events than existing enhancement methods. Given that the mechanism of tDCS appears to alter resting membrane potential rather than directly producing action potentials, tDCS may be more beneficial in the complementation of existing methods rather than their replacement. While very little specific research has been conducted in this area, there is some evidence that tDCS can produce better outcomes than certain types of training (e.g., motor training; Pan et al., 2017), and that tDCS can increase the efficacy of cognitive training programmes in areas like dieting self-discipline (Forcano et al., 2018). Additional research establishing the interaction effects between tDCS and current military enhancement interventions is required before the efficacious delivery of tDCS can be ensured.
Conclusion
The aim of this paper was to examine whether military tDCS use can be efficacious and ethical in military settings. Our assessment is that tDCS offers a number of cognitive, motor, and perceptual enhancement opportunities which could provide value in military situations like training and operations. There is potential scope for use in a number of key areas that directly affect practical battlefield advantage and survivability, such as deceptive capabilities, risk-taking, threat detection, perception, and physiological improvement. Additionally, tDCS has the potential to improve command and control decision making by enhancing aspects of cognition, such as planning, problem solving, memory, and probabilistic assessment.
As well as benefits, tDCS poses a number of risks that need to be considered, and in some cases addressed by military organisations. Overall, it is the opinion of the current authors that the safety concerns are manageable, and the risks moderate. The technique has been tested on thousands of subjects, with most studies reporting only temporary minor adverse effects such as itching, tingling, and headaches. The most severe adverse consequence of tDCS reported to date was abrasions at the locations of the electrodes (which can be mitigated through the application of saline soaked sponges enclosing conductive rubber electrodes). The intensity and rate of occurrence of adverse events are so low that some effects (such as headaches) are reported in similar rates between sham and active stimulation. Assuming that the equipment used can reliably deliver the current evenly and steadily (i.e., using medically certified equipment), the device is well-maintained, appropriate safety guidelines specific to the military are in place, and systematic screening is conducted to ensure that stimulation is performed only on suitable personnel, the risk of experiencing adverse effects appears to be no greater in military populations than in general populations.
Given the potential of tDCS to increase warfighter capabilities in support of command and control on a temporary, at needs basis, we submit that the potential benefits of tDCS have the potential to outweigh the limited and mitigable risks. In situations where tDCS can be used for the purpose of completing military objectives safely and where a marginal increase in performance can mean the difference between life and death, consequentialist arguments in support of military tDCS enhancement are compelling. However, this assumes that specific desired effects can be reliably delivered to individual warfighters. The effects are neither normally distributed nor even multimodally distributed, so there is a requirement to go beyond group-level findings that fail to account for the large number of inter-individual and environmental variables that affect the outcomes of stimulation.
Despite the potential of military tDCS application, there are a number of other open questions that need to be addressed before efficacious and ethical military stimulation is possible. It is unclear how applicable the current body of literature is in areas of military application. Research needs to be conducted investigating how specific effects of tDCS can be generalised across civilian and military populations given environmental factors and individual differences. While efficacious stimulation appears feasible, more research is required before tDCS can have a practical and substantial impact on capability and mission outcomes in the context of the current military training and enhancement landscape. As well as these empirical issues, a number of ethical questions require attention. It was not the purpose of this paper to take normative ethical stances beyond any apparent utilitarian conclusions that emerge from discussion of benefits and risk. Consequently, other ethical questions like the moral responsibility of warfighters’ actions while experiencing the effects of tDCS, and how traditional bioethical principles (such as respect for persons and justice) can be maintained, need to be considered separately.
As DIY tDCS becomes increasingly inexpensive and popular, the risk of military personnel experimenting with potentially dangerous devices or inadvertently using counterproductive montages increases. Given the pressing risks associated with self-administration, it would be preferable for military organisations to play a proactive role in addressing the remaining empirical and philosophical questions. If a military-based research programme were to conclude that tDCS is unable to provide genuine warfighting advantage, the temptation for riskier informal use by military personnel would be reduced (if not ended). If, however, the remaining issues (such as inter-individual variation) are adequately resolved, tDCS could then be administered in a controlled way that ensures safe, ethical, reliable, and efficacious delivery.
Overall, tDCS is a safe technique which may have potential for military application. Prospective benefits outweigh the safety risks when tDCS is conducted within established limits, and ethical concerns can be addressed through planning and policy. tDCS is acceptably safe when used in controlled conditions, and while additional research is required in a number of areas, tDCS has the potential to be valuable to military organisations once these issues are addressed and appropriate guidelines are enacted. While it is the view of the current authors that the state of the literature has yet to reach the point where ethical and efficacious delivery is possible in military settings, tDCS is presently fertile ground for military specific research.
Author Contributions
SD was responsible for writing and preparation of the manuscript. SD and GS were jointly responsible for conceptualisation (scope, ideas, and substance) and editing (including critical review and revision).
Conflict of Interest Statement
The authors declare that the research was conducted in the absence of any commercial or financial relationships that could be construed as a potential conflict of interest.
Acknowledgments
The authors thank John Hansen for administrative support, and both Jacqui Willans-Price and John Hansen for proofreading assistance and offering valuable comments and suggestions.
Footnotes
- ^ The term “non-invasive” is used in the sense that no physical incision or insertion is made.
- ^ The Prussian general and theorist Carl von Clausewitz, for example, wrote on the “friction of war,” where “everything is very simple in war, but the simplest thing is difficult” (von Clausewitz, 1989, p. 119).
- ^ Streufert and Streufert (1968) argue that military situations often have an optimal level of risk which should ideally be matched to personnel with complementary risk taking propensities. Directly adjusting risk-taking behaviour could assist in this calibration (albeit while raising ethical issues that are beyond the scope of the present paper).
- ^ Indicated by a 14–34% decrease in the dopamine antagonist [11C]raclopride (Fonteneau et al., 2018).
- ^ Indirect in the sense that the dopaminergic affects are mediated through sensory organs (Heinz et al., 2012). For example, exercise (Hattori et al., 1994) and music consumption (Suzuki et al., 2008) can indirectly increase dopamine levels by approximately 50–100%.
- ^ Such as cocaine and amphetamine, which have been found to stimulate dopamine in the nucleus accumbens by up to 1000%, respectively (Di Chiara and Imperato, 1988).
- ^ Although tDCS does not involve incision or insertion, the technique is invasive in the sense that current spreads into adjacent brain tissue. This has led some authors (e.g., Davis et al., 2013) to contend that the term “non-invasive brain stimulation” may be inappropriate.
- ^ For a visual demonstration of tDCS procedure, see the online version of the article available at http://www.jove.com/video/2744/electrode-positioning-montage-transcranial-direct-current.
- ^ A specific list of drugs that interact with transcranial magnetic stimulation (TMS) is given by Rossi et al. (2009), and it is reasonable to assume that the same exclusion list is appropriate for tDCS studies (Davis et al., 2013).
- ^ This is an overall figure that also includes first session non-responders (which are significantly less reliable, with only 56% continuing not to respond in the second session).
- ^ Surface-based registration uses a spherical surface-based coordinate system which maps individuals’ brains based on structural and functional features (Fischl et al., 1999).
- ^ Dynamic causal modelling is a method for modelling connectivity in distributed neuronal response networks (Friston et al., 2003).
References
Afshin, A., Forouzanfar, M. H., Reitsma, M. B., Sur, P., Estep, K., Lee, A., et al. (2017). Health effects of overweight and obesity in 195 countries over 25 years. N. Engl. J. Med. 377, 13–27. doi: 10.1056/NEJMoa1614362
Agnew, W. F., and McCreery, D. B. (1987). Considerations for safety in the use of extracranial stimulation for motor evoked potentials. Neurosurgery 20, 143–147. doi: 10.1097/00006123-198701000-00030
Aidman, E. (2017). The cognitive fitness framework: a roadmap for systematic, evidence-based mental skills training and performance enhancement. J. Sci. Med. Sport 20(Suppl. 2):S50. doi: 10.1016/j.jsams.2017.09.159
Allen, C. D., and Gerras, S. J. (2009). Developing creative and critical thinkers. Mil. Rev. 89, 77–83.
Alonzo, A., Brassil, J., Taylor, J. L., Martin, D., and Loo, C. K. (2012). Daily transcranial direct current stimulation (tDCS) leads to greater increases in cortical excitability than second daily transcranial direct current stimulation. Brain Stimul. 5, 208–213. doi: 10.1016/j.brs.2011.04.006
Andrews, S. C., Hoy, K. E., Enticott, P. G., Daskalakis, Z. J., and Fitzgerald, P. B. (2011). Improving working memory: the effect of combining cognitive activity and anodal transcranial direct current stimulation to the left dorsolateral prefrontal cortex. Brain Stimul. 4, 84–89. doi: 10.1016/j.brs.2010.06.004
Antal, A., Terney, D., Poreisz, C., and Paulus, W. (2007). Towards unravelling task-related modulations of neuroplastic changes induced in the human motor cortex. Eur. J. Neurosci. 26, 2687–2691. doi: 10.1111/j.1460-9568.2007.05896.x
Antonenko, D., Nierhaus, T., Meinzer, M., Prehn, K., Thielscher, A., Ittermann, B., et al. (2018). Age-dependent effects of brain stimulation on network centrality. Brain Stimul. 176, 71–82. doi: 10.1016/j.neuroimage.2018.04.038
Armenta, R. F., Rush, T., LeardMann, C. A., Millegan, J., Cooper, A., Hoge, C. W., et al. (2018). Factors associated with persistent posttraumatic stress disorder among U.S. military service members and veterans. BMC Psychiatry 18:48. doi: 10.1186/s12888-018-1590-5
Aslaksen, P. M., Vasylenko, O., and Fagerlund, A. J. (2014). The effect of transcranial direct current stimulation on experimentally induced heat pain. Exp. Brain Res. 232, 1865–1873. doi: 10.1007/s00221-014-3878-0
Australian Army (2017). Land Warfare Doctrine 1: The Fundamentals of Land Power. Canberra: Australian Department of Defence.
Australian Army (2018). Land Warfare Doctrine 1-0: Personnel. Canberra: Australian Department of Defence.
Batsikadze, G., Moliadze, V., Paulus, W., Kuo, M. F., and Nitsche, M. A. (2013). Partially non-linear stimulation intensity-dependent effects of direct current stimulation on motor cortex excitability in humans. J. Physiol. 591, 1987–2000. doi: 10.1113/jphysiol.2012.249730
Beauchamp, M. S., Beurlot, M. R., Fava, E., Nath, A. R., and Parikh, N. A. (2011). The developmental trajectory of brain-scalp distance from birth through childhood: implications for functional neuroimaging. PLoS One 6:e24981. doi: 10.1371/journal.pone.0024981
Been, G., Ngo, T. T., Miller, S. M., and Fitzgerald, P. D. (2007). The use of tDCS and CVS as methods of non-invasive brain stimulation. Brain Res. Rev. 56, 346–361. doi: 10.1016/j.brainresrev.2007.08.001
Benwell, C. S. Y., Learmonth, G., Miniussi, C., Harvey, M., and Thut, G. (2015). Non-linear effects of transcranial direct current stimulation as a function of individual baseline performance: evidence from biparietal tDCS influence on lateralized attention bias. Cortex 69, 152–165. doi: 10.1016/j.cortex.2015.05.007
Berryhill, M. E., and Jones, K. T. (2012). tDCS selectively improves working memory in older adults with more education. Neurosci. Lett. 521, 148–151. doi: 10.1016/j.neulet.2012.05.074
Berryhill, M. E., Wencil, E. B., Coslett, H. B., and Olsona, I. R. (2010). A selective working memory impairment after transcranial direct current stimulation to the right parietal lobe. Neurosci. Lett. 479, 312–316. doi: 10.1016/j.neulet.2010.05.087
Biggs, A. T., Cain, M. S., and Mitroff, S. R. (2015). Cognitive training can reduce civilian casualties in a simulated shooting environment. Psychol. Sci. 26, 1164–1176. doi: 10.1177/0956797615579274
Bikson, M., Datta, A., Rahman, A., and Scaturro, J. (2010). Electrode montages for tDCS and weak transcranial electrical stimulation: role of “return” electrode’s position and size. Clin. Neurophysiol. 121, 1976–1978. doi: 10.1016/j.clinph.2010.05.020
Binney, R. J., Ashaie, S. A., Zuckerman, B. M., Hung, J., and Reilly, J. (2018). Frontotemporal stimulation modulates semantically-guided visual search during confrontation naming: a combined tDCS and eye tracking investigation. Brain Lang. 18, 14–23. doi: 10.1016/j.bandl.2018.04.004
Boggio, P. S., Fregn, F., Valasek, C., Ellwood, S., Chi, R., Gallate, J., et al. (2009). Temporal lobe cortical electrical stimulation during the encoding and retrieval phase reduces false memories. PLoS One 4:e4959. doi: 10.1371/journal.pone.0004959
Brem, A.-K., Fried, P. J., Horvath, J. C., Robertsona, E. M., and Pascual-Leone, A. (2014). Is neuroenhancement by noninvasive brain stimulation a net zero-sum proposition? Neuroimage 85, 1058–1068. doi: 10.1016/j.neuroimage.2013.07.038
Brunoni, A. R., Amadera, J., Berbel, B., Volz, M. S., Rizzerio, B. G., and Fregn, F. (2011). A systematic review on reporting and assessment of adverse effects associated with transcranial direct current stimulation. Neuropsychopharmacology 14, 1133–1145. doi: 10.1017/S1461145710001690
Buchanan, A. (2008). Enhancement and the ethics of development. Kennedy Inst. Ethics J. 18, 1–34. doi: 10.1353/ken.0.0003
Burkhalter, T. M., and Hillman, C. H. (2011). A narrative review of physical activity, nutrition, and obesity to cognition and scholastic performance across the human lifespan. Adv. Nutr. 2, 201S–206S. doi: 10.3945/an.111.000331
Burton, P. S., Nance, J. H., and Walton, D. C. (2011). Lethal Weapon: DRSE builds SOF’s greatest weapon - the minds of its soldiers. Spec. Warf. 24, 10–14.
California Department of Public Health (2013). CDPH Warns Consumers Not to Use TDCS Home Device Kit. Available at: http://www.cdph.ca.gov/Pages/NR13-029.aspx
Canli, T., Brandon, S., Casebeer, W., Crowley, P. J., DuRousseau, D., Greely, H. T., et al. (2007). Neuroethics and national security. Am. J. Bioeth. 7, 3–13. doi: 10.1080/15265160701290249
Cannon, M. (1988). Task Force Smith: a study in (un)preparedness and (ir)responsibility. Mil. Rev. 68, 63–73.
Carretta, T. R., Perry, D. C. J., and Ree, M. J. (1996). Prediction of situational awareness in F-15 pilots. Int. J. Aviat. Psychol. 6, 21–41. doi: 10.1207/s15327108ijap0601_2
Carver, R. P. (1978). The case against statistical significance testing. Harv. Educ. Rev. 48, 378–399. doi: 10.17763/haer.48.3.t490261645281841
Casali, J. G., Talcott, K., Keady, J. P., and Killion, M. C. (2011). “Warfighter auditory situation awareness: locating the shooter with and without hearing protection,” in Proceedings of the Human Systems Integration Symposium (HSIS 2011), (Alexandria, VA: American Society of Naval Engineers),45–56.
Chi, R. P., and Snyder, A. W. (2011). Facilitate insight by non-invasive brain stimulation. PLoS One 6:e16655. doi: 10.1371/journal.pone.0016655
Clark, V. P., Coffman, B. A., Mayer, A. R., Weisend, M. P., Lane, T. D., Calhoun, V. D., et al. (2012). TDCS guided using fMRI significantly accelerates learning to identify concealed objects. Neuroimage 59, 117–128. doi: 10.1016/j.neuroimage.2010.11.036
Coffman, B. A., Trumbo, M. C., Flores, R. A., Garcia, C. M., van der Merwe, A. J., Wassermann, E. M., et al. (2012). Impact of tDCS on performance and learning of target detection: interaction with stimulus characteristics and experimental design. Neuropsychologia 50, 1594–1602. doi: 10.1016/j.neuropsychologia.2012.03.012
Cogiamanian, F., Marceglia, S., Ardolino, G., Barbieri, S., and Priori, A. (2007). Improved isometric force endurance after transcranial direct current stimulation over the human motor cortical areas. Eur. J. Neurosci. 26, 242–249. doi: 10.1111/j.1460-9568.2007.05633.x
Cools, R. (2011). Dopaminergic control of the striatum for high-level cognition. Curr. Opin. Neurobiol. 21, 402–407. doi: 10.1016/j.conb.2011.04.002
Crabbe, R. R. (2000). “The nature of command,” in The Human in Command: Exploring the Modern Military Experience, eds R. Pigeau and C. McCann (Toronto: Springer), 9–16.
Dackis, C. A., and Gold, M. S. (2008). Addictiveness of central stimulants. Adv. Alcohol Subst. Abuse 9, 9–26. doi: 10.1300/J251v09n01_02
DaSilva, A. F., Volz, M. S., Bikson, M., and Fregni, F. (2011). Electrode positioning and montage in transcranial direct current stimulation. J. Vis. Exp. 51:2744. doi: 10.3791/2744
Datta, A., Baker, J. M., Bikson, M., and Fridriksson, J. (2011). Individualized model predicts brain current flow during transcranial direct-current stimulation treatment in responsive stroke patient. Brain Stimul. 4, 169–174. doi: 10.1016/j.brs.2010.11.001
Datta, A., Bansal, V., Diaz, J., Patel, J., Reato, D., and Bikson, M. (2009). Gyri-precise head model of transcranial direct current stimulation: improved spatial focality using a ring electrode versus conventional rectangular pad. Brain Stimul. 2, 201–207. doi: 10.1016/j.brs.2009.03.005
Davis, M. C., Luecken, L., and Lemery-Chalfant, K. (2009). Resilience in common life: introduction to the special issue. J. Pers. 77, 1637–1644. doi: 10.1111/j.1467-6494.2009.00595.x
Davis, N. J., Gold, E., Pascual-Leone, A., and Bracewell, R. M. (2013). Challenges of proper placebo control for non-invasive brain stimulation in clinical and experimental applications. Eur. J. Neurosci. 38, 2973–2977. doi: 10.1111/ejn.12307
Dcminsf (2014). Avoiding Burns and Scars. [reddit thread]. Available at: http://www.reddit.com/r/tDCS/comments/1xrjyh/avoiding_burns_and_scars/
de Berker, A. O., Bikson, M., and Bestmann, S. (2013). Predicting the behavioral impact of transcranial direct current stimulation: issues and limitations. Front. Hum. Neurosci. 7:613. doi: 10.3389/fnhum.2013.00613
de Jesus, S. N., and Gonçalves, M. (2012). P-1372 - Modulation of the autonomic nervous system by transcranial direct current stimulation: preliminary results of a pilot study with relevance to resilience to stress science. Eur. Psychiatry 27:1. doi: 10.1016/S0924-9338(12)75539-6
de Raedt, R., Remue, J., Loeys, T., Hooley, J. M., and Baeken, C. (2017). The effect of transcranial direct current stimulation of the prefrontal cortex on implicit self-esteem is mediated by rumination after criticism. Behav. Res. Ther. 99, 138–146. doi: 10.1016/j.brat.2017.10.009
Di Chiara, G., and Imperato, A. (1988). Drugs abused by humans preferentially increase synaptic dopamine concentrations in the mesolimbic system of freely moving rats. Proc. Natl. Acad. Sci. U.S.A. 85, 5274–5278. doi: 10.1073/pnas.85.14.5274
Ditye, T., Jacobson, L., Walsh, V., and Lavidor, M. (2012). Modulating behavioral inhibition by tDCS combined with cognitive training. Exp. Brain Res. 219, 363–368. doi: 10.1007/s00221-012-3098-4
Dockery, C. A., Hueckel-Weng, R., Birbaumer, N., and Plewnia, C. (2009). Enhancement of planning ability by transcranial direct current stimulation. J. Neurosci. 29, 7271–7277. doi: 10.1523/JNEUROSCI.0065-09.2009
D’Urso, G., Dell’Osso, B., Rossi, R., Brunoni, A. R., Bortolomasi, M., Ferrucci, R., et al. (2017). Clinical predictors of acute response to transcranial direct current stimulation (tDCS) in major depression. J. Affect. Disord. 219, 25–30. doi: 10.1016/j.jad.2017.05.019
Dymond, A. M., Coger, R. W., and Serafetinides, E. A. (1975). Intracerebral current levels in man during electrosleep therapy. Biol. Psychiatry 10, 101–104.
Endsley, M. R. (1995). Toward a theory of situation awareness in dynamic systems. Hum. Factors 37, 32–64. doi: 10.1518/001872095779049543
Falcone, B., Coffman, A., Clark, V. P., and Parasuraman, R. (2012). Transcranial direct current stimulation augments perceptual sensitivity and 24-hour retention in a complex threat detection task. PLoS One 7:e34993. doi: 10.1371/journal.pone.0034993
Fecteau, S., Knoch, D., Fregni, F., Sultani, N., Boggio, P., and Pascual-Leone, A. (2007). Diminishing risk-taking behaviour by modulating activity in the prefrontal cortex: a direct current stimulation study. Neuroscience 27, 12500–12505. doi: 10.1523/JNEUROSCI.3283-07.2007
Ferrucci, R., Bortolomasi, M., Vergari, M., Tadini, L., Salvoro, B., Giacopuzzi, M., et al. (2009). Transcranial direct current stimulation in severe, drug-resistant major depression. J. Affect. Disord. 118, 215–219. doi: 10.1016/j.jad.2009.02.015
Fischer, D. B., Fried, P. J., Ruffini, G., Ripolles, O., Salvador, R., Banus, J., et al. (2017). Multifocal tDCS targeting the resting state motor network increases cortical excitability beyond traditional tDCS targeting unilateral motor cortex. Neuroimage 157, 34–44. doi: 10.1016/j.neuroimage.2017.05.060
Fischl, B., Sereno, M. I., Tootell, R. B., and Dale, A. M. (1999). High-resolution intersubject averaging and a coordinate system for the cortical surface. Hum. Brain Mapp. 8, 272–284. doi: 10.1002/(SICI)1097-0193(1999)8:4<272::AID-HBM10>3.0.CO;2-4
Fitz, N. S., and Reiner, P. B. (2015). The challenge of crafting policy for do-it-yourself brain stimulation. J. Med. Ethics 41, 410–412. doi: 10.1136/medethics-2013-101458
Fleckenstein, A. E., Volz, T. J., Riddle, E. L., Gibb, J. W., and Hanson, G. R. (2007). New insights into the mechanism of action of amphetamines. Annu. Rev. Pharmacol. Toxicol. 47, 681–698. doi: 10.1146/annurev.pharmtox.47.120505.105140
Flöel, A., Rösser, N., Michka, O., Knecht, S., and Breitenstein, C. (2008). Noninvasive brain stimulation improves language learning. J. Cogn. Neurosci. 20, 1415–1422. doi: 10.1162/jocn.2008.20098
Flöel, A., Suttorp, W., Kohl, O., Kürten, J., Lohmann, H., Breitenstein, C., et al. (2012). Non-invasive brain stimulation improves object-location learning in the elderly. Neurobiol. Aging 33, 1682–1689. doi: 10.1016/j.neurobiolaging.2011.05.007
Fonteneau, C., Redoute, J., Haesebaert, F., Le Bars, D., Costes, N., Suaud-Chagny, M.-F., et al. (2018). Frontal transcranial direct current stimulation induces dopamine release in the ventral striatum in human. Cereb. Cortex 28, 2636–2646. doi: 10.1093/cercor/bhy093
Forcano, L., de la Torre, R., Mata, F., and Verdejo-Garcia, A. (2018). Cognitive and neuromodulation strategies for unhealthy eating and obesity: systematic review and discussion of neurocognitive mechanisms. Neurosci. Biobehav. Rev. 87, 161–191. doi: 10.1016/j.neubiorev.2018.02.003
Frank, E., Wilfurth, S., Landgrebe, M., Eichhammer, P., Hajak, G., and Langguth, B. (2010). Anodal skin lesions after treatment with transcranial direct current stimulation. Brain Stimul. 3, 58–59. doi: 10.1016/j.brs.2009.04.002
Friston, K. J., Harrison, L., and Penny, W. (2003). Dynamic causal modelling. Neuroimage 19, 1273–1302. doi: 10.1016/S1053-8119(03)00202-7
Fujiyama, H., Hinder, M. R., Barzideh, A., de Vijver, C. V., Badache, A. C., Manrique-C, M. N., et al. (2017). Preconditioning tDCS facilitates subsequent tDCS effect on skill acquisition in older adults. Neurobiol. Aging 51, 31–42. doi: 10.1016/j.neurobiolaging.2016.11.012
Fujiyama, H., Hyde, J., Hinder, M. R., Kim, S. J., McCormack, G. H., Vickers, J. C., et al. (2014). Delayed plastic responses to anodal tDCS in older adults. Front. Aging Neurosci. 6:115. doi: 10.3389/fnagi.2014.00115
Garcia-Cossio, E., Witkowski, M., Robinson, S. E., Cohen, L. G., Birbaumer, N., and Soekadar, S. R. (2016). Simultaneous transcranial direct current stimulation (tDCS) and whole-head magnetoencephalography (MEG): assessing the impact of tDCS on slow cortical magnetic fields. Neuroimage 140, 33–40. doi: 10.1016/j.neuroimage.2015.09.068
Garinther, G. R., and Peters, L. J. (1990). Impact of communications on armor crew performance: investigating the impact of noise and other variables on mission effectiveness. Army RD&A Bull. 1, 1–5.
Gawande, A. (2004). Casualties of war — military care for the wounded from Iraq and Afghanistan. N. Engl. J. Med. 351, 2471–2475. doi: 10.1056/NEJMp048317
Gilmore, P. W. (2016). Leading a Resilient Force: Insights of an Australian General. Canberra: Australian Department of Defence.
Giordano, J., Bikson, M., Kappenman, E. S., Clark, V. P., Coslett, H. B., Hamblin, M. R., et al. (2017). Mechanisms and effects of transcranial direct current stimulation. Dose Response 15, 1–22. doi: 10.1177/1559325816685467
Gladwin, T. E., den Uyl, T. E., and Wiers, R. W. (2012). Anodal tDCS of dorsolateral prefontal cortex during an Implicit Association Test. Neurosci. Lett. 517, 82–86. doi: 10.1016/j.neulet.2012.04.025
Godwin, E. E., and Kreutzer, J. S. (2013). Embracing a new path to emotional recovery: adopting resilience theory in post-TBI psychotherapy. Brain Inj. 27, 637–639. doi: 10.3109/02699052.2012.750745
Goldman, R. L., Borckardt, J. J., Frohman, H. A., O’Neil, P. M., Madan, A., Campbell, L. K., et al. (2011). Prefrontal cortex transcranial direct current stimulation (tDCS) temporarily reduces food cravings and increases the self-reported ability to resist food in adults with frequent food craving. Apetite 56, 741–746. doi: 10.1016/j.appet.2011.02.013
Gonzalez, C., and Wimisberg, J. (2007). Situation awareness in dynamic decision making: effects of practice and working memory. J. Cogn. Eng. Decis. Mak. 1, 56–74. doi: 10.1177/155534340700100103
Green, C. S., and Bavelier, D. (2003). Action video game modifies visual selective attention. Nature 423, 534–537. doi: 10.1038/nature01647
Gronlund, S. D., Dougherty, M. R. P., Ohrt, D. D., Thomson, G. L., Bleckley, M. K., Bain, D. L., et al. (1997). The Role of Memory in Air Traffic Control (DOT/FAA/AM-97/22). Washington, DC: Office of Aviation Medicine.
Gutzwiller, R. S., and Clegg, B. A. (2013). The role of working memory in levels of situation awareness. J. Cogn. Eng. Decis. Mak. 7, 141–154. doi: 10.1177/1555343412451749
Hai, R., and Heckman, J. J. (2017). Inequality in human capital and endogenous credit constraints. Rev. Econ. Dyn. 25, 4–36. doi: 10.1016/j.red.2017.01.001
Hamilton, R. H., Messing, S., and Chatterjee, A. (2011). Rethinking the thinking cap: ethics of neural enhancement using noninvasive brain stimulation. Neurology 76, 187–193. doi: 10.1212/WNL.0b013e318205d50d
Hampstead, B. M., Brown, G. S., and Hartley, J. F. (2014). Transcranial direct current stimulation modulates activation and effective connectivity during spatial navigation. Brain Stimul. 7, 314–324. doi: 10.1016/j.brs.2013.12.006
Hartel, C. E., Smith, K., and Prince, C. (1991). “Defining aircrew coordination: searching mishaps for meaning,” in Proceedings of the Sixth International Symposium on Aviation Psychology, ed. R. S. Jensen (Columbus: Ohio State University Dept. of Aviation).
Hattori, S., Naoi, M., and Nishino, H. (1994). Striatal dopamine turnover during treadmill running in the rat: relation to the speed of running. Brain Res. Bull. 35, 41–49. doi: 10.1016/0361-9230(94)90214-3
Hecht, D., Walsh, V., and Lavidor, M. (2010). Transcranial direct current stimulation facilitates decision making in a probabilistic guessing task. J. Neurosci. 30, 4241–4245. doi: 10.1523/JNEUROSCI.2924-09.2010
Heinz, A., Kipke, R., Heimann, H., and Wiesing, U. (2012). Cognitive neuroenhancement: false assumptions in the ethical debate. J. Med. Ethics 38, 372–375. doi: 10.1136/medethics-2011-100041
Heinze, K., Ruh, N., Nitschke, K., Reis, J., Fritsch, B., Unterrainer, J. M., et al. (2014). Transcranial direct current stimulation over left and right DLPFC: lateralized effects on planning performance and related eye movements. Biol. Psychol. 102, 130–140. doi: 10.1016/j.biopsycho.2014.07.019
Heise, K. F., Niehoff, M., Feldheim, J. F., Liuzzi, G., Gerloff, C., and Hummel, F. C. (2014). Differential behavioral and physiological effects of anodal transcranial direct current stimulation in healthy adults of younger and older age. Front. Aging Neurosci. 6:146. doi: 10.3389/fnagi.2014.00146
Hétu, S., Taschereau-Dumouchel, V., and Jackson, P. L. (2012). Stimulating the brain to study social interactions and empathy. Brain Stimul. 5, 95–102. doi: 10.1016/j.brs.2012.03.005
Hilgetag, C. C., Théoret, H., and Pascual-Leone, A. (2001). Enhanced visual spatial attention ipsilateral to rTMS-induced ’virtual lesions’ of human parietal cortex. Nat. Neurosci. 4, 953–957. doi: 10.1038/nn0901-953
Hillman, C. H., Erickson, K. I., and Kramer, A. F. (2008). Be smart, exercise your heart: exercise effects on brain and cognition. Nat. Rev. Neurosci. 9, 59–65. doi: 10.1038/nrn2298
Ho, K.-A., Taylor, J. L., Chew, T., Gálvez, V., Alonzo, A., Bai, S., et al. (2016). The effect of transcranial direct current stimulation (tDCS) electrode size and current intensity on motor cortical excitability: evidence from single and repeated sessions. Brain Stimul. 9, 1–7. doi: 10.1016/j.brs.2015.08.003
Holgado, D., Vadillo, M. A., and Sanabria, D. (2019). The effects of transcranial direct current stimulation on objective and subjective indexes of exercise performance: a systematic review and meta-analysis. Brain Stimul. 12, 242–250. doi: 10.1016/j.brs.2018.12.002
Hummel, F. C., and Cohen, L. G. (2005). Improvement of motor function with noninvasive cortical stimulation in a patient with chronic stroke. Neurorehab. Neural Repair 19, 14–19. doi: 10.1177/1545968304272698
Iyer, M. B., Mattu, U., Grafman, J., Lomarev, M., Sato, S., and Wassermann, E. M. (2005). Safety and cognitive effect of frontal DC brain polarization in healthy individuals. Neurology 64, 872–875. doi: 10.1212/01.WNL.0000152986.07469.E9
Jacobson, L., Javitt, D. C., and Lavidor, M. (2011). Activation of inhibition: diminishing impulsive behavior by direct current stimulation over the inferior frontal gyrus. J. Cogn. Neurosci. 23, 3380–3387. doi: 10.1162/jocn_a_00020
Jacobson, L., Koslowsky, M., and Lavidor, M. (2012). tDCS polarity effects in motor and cognitive domains: a meta-analytical review. Exp. Brain Res. 216, 1–10. doi: 10.1007/s00221-011-2891-9
Javadi, A. H., and Cheng, P. (2012). Transcranial direct current stimulation (tDCS) enhances reconsolidation of long-term memory. Brain Stimul. 6, 668–674. doi: 10.1016/j.brs.2012.10.007
Joint Chiefs of Staff (2012). Joint Publication 3-13.4: Military Deception. Washington, DC: United States Department of Defense.
Karim, A. A., Schneider, M., Lotze, M., Veit, R., Sauseng, P., Braun, C., et al. (2010). The truth about lying: Inhibition of the anterior prefrontal cortex improves deceptive behavior. Cereb. Cortex 20, 205–213. doi: 10.1093/cercor/bhp090
Kelsall, H. L., Wijesinghe, M. S. D., Creamer, M. C., McKenzie, D. P., Forbes, A. B., Page, M. J., et al. (2015). Alcohol use and substance use disorders in Gulf War, Afghanistan, and Iraq War veterans compared with nondeployed military personnel. Epidemiol. Rev. 37, 38–54. doi: 10.1093/epirev/mxu014
Kessler, S. K., Turkeltaub, P. E., Benson, J. G., and Hamilton, R. H. (2012). Differences in the experience of active and sham transcranial direct current stimulation. Brain Stimul. 5, 155–162. doi: 10.1016/j.brs.2011.02.007
Kleider, H. M., Parrott, D. J., and King, T. Z. (2009). Shooting behaviour: how working memory and negative emotionality influence police officer shoot decisions. Appl. Cogn. Psychol. 24, 707–717. doi: 10.1002/acp.1580
Klem, G. H., Luders, H. O., Jasper, H. H., and Elger, C. (1999). The ten-twenty electrode system of the International Federation. Electroencephalogr. Clin. Neurophysiol. Suppl. 52, 3–6.
Kraft, A., Roehmel, J., Olma, M. C., Schmidt, S., Irlbacher, K., and Brandt, S. A. (2010). Transcranial direct current stimulation affects visual perception measured by threshold perimetry. Exp. Brain Res. 207, 283–290. doi: 10.1007/s00221-010-2453-6
Laakso, I., Tanaka, S., Koyama, S., De Santis, V., and Hirata, A. (2015). Inter-subject variability in electric fields of motor cortical tDCS. Brain Stimul. 8, 906–913. doi: 10.1016/j.brs.2015.05.002
Lambert, D. A., and Scholz, J. B. (2007). Ubiquitous command and control. Intell. Decis. Technol. 1, 157–173. doi: 10.3233/IDT-2007-1305
Lande, R. G., Marin, B. A., Chang, A. S., and Lande, G. R. (2008). Survey of alcohol use in the U.S. Army. J. Addict. Dis. 27, 115–121. doi: 10.1080/10550880802122711
Learmonth, G., Thut, G., Benwell, C. S. Y., and Harvey, M. (2015). The implications of state-dependent tDCS effects in aging: Behavioural response is determined by baseline performance. Neuropsychologia 74, 108–119. doi: 10.1016/j.neuropsychologia.2015.01.037
Levasseur-Moreau, J., Brunelin, J., and Fecteau, S. (2013). Non-invasive brain stimulation can induce paradoxical facilitation. Are these neuroenhancements transferable and meaningful to security services? Front. Hum. Neurosci. 7:449. doi: 10.3389/fnhum.2013.00449
Li, L. M., Uehara, K., and Hanakawa, T. (2015). The contribution of interindividual factors to variability of response in transcranial direct current stimulation studies. Front. Cell. Neurosci. 9:181. doi: 10.3389/fncel.2015.00181
Lieberman, H. R., Bathalon, G. P., Falco, C. M. III, Morgan, C. A., Niro, P. J., and Tharion, W. J. (2005). The fog of war: decrements in cognitive performance and mood associated with combat-like stress. Aviat. Space Environ. Med. 76(7 Suppl.), C7–C14.
Liebetanz, D., Koch, R., Mayenfels, S., König, F., Paulus, W., and Nitsche, M. A. (2009). Safety limits of cathodal transcranial direct current stimulation in rats. Clin. Neurophysiol. 120, 1161–1167. doi: 10.1016/j.clinph.2009.01.022
Lin, C. H. J., Knowlton, B. J., Chiang, M. C., Iacoboni, M., Udompholkul, P., and Wu, A. D. (2011). Brain-behavior correlates of optimizing learning through interleaved practice. Neuroimage 56, 1758–1772. doi: 10.1016/j.neuroimage.2011.02.066
Lippold, O. C. J., and Redfearn, J. W. T. (1964). Mental changed resulting from the passage of small direct currents through the human brain. Br. J. Psychol. 110, 768–972. doi: 10.1192/bjp.110.469.768
Loftus, A. M., and Nicholls, M. E. R. (2012). Testing the activation–orientation account of spatial attentional asymmetries using transcranial direct current stimulation. Neuropsychologia 50, 2573–2576. doi: 10.1016/j.neuropsychologia.2012.07.003
Looi, C. Y., and Kadosh, R. C. (2015). “The use of transcranial direct current stimulation for cognitive enhancement,” in Cognitive Enhancement: Pharmacologic, Environmental and Genetic Factors, eds S. Knafo and C. Venero (Amsterdam: Elsevier), 307–341.
López-Alonso, V., Fernández-Del-Olmo, M., Costantini, A., González-Henríquez, J. J., and Cheeran, B. J. (2015). Intra-individual variability in the response to anodal transcranial direct current stimulation. Clin. Neurophysiol. 126, 2342–2347. doi: 10.1016/j.clinph.2015.03.022
Lupyan, G., Mirman, D., Hamilton, R. H., and Thompson-Schill, S. L. (2012). Categorization is modulated by transcranial direct current stimulation over left prefrontal cortex. Cognition 124, 36–49. doi: 10.1016/j.cognition.2012.04.002
Lutz, A., Slagter, H. A., Dunne, J. D., and Davidson, R. J. (2008). Attention regulation and monitoring in meditation. Trends Cogn. Sci. 12, 163–169. doi: 10.1016/j.tics.2008.01.005
Machado, D. G. D. S., Unal, G., Andrade, S. M., Moreira, A., Altimari, L. R., Brunoni, A. R., et al. (2018). Effect of transcranial direct current stimulation on exercise performance: a systematic review and meta-analysis. Brain Stimul. doi: 10.1016/j.brs.2018.12.227 [Epub ahead of print].
Maeoka, H., Matsuo, A., Hiyamizu, M., Morioka, S., and Ando, H. (2012). Influence of transcranial direct current stimulation of the dorsolateral prefrontal cortex on pain related emotions: a study using electroencephalographic power spectrum analysis. Neurosci. Lett. 512, 12–16. doi: 10.1016/j.neulet.2012.01.037
Marshall, L., Mölle, M., Hallschmid, M., and Born, J. (2004). Transcranial direct current stimulation during sleep improves declarative memory. J. Neurosci. 24, 9985–9992. doi: 10.1523/JNEUROSCI.2725-04.2004
McIntire, L. K., McKinley, R. A., Nelson, J. M., and Goodyear, C. (2017a). Transcranial direct current stimulation (tDCS) interferes with cognitive bias mitigation learning. Brain Stimul. 10:354. doi: 10.1016/j.brs.2017.01.039
McIntire, L. K., McKinley, R. A., Nelson, J. M., and Goodyear, C. (2017b). Transcranial direct current stimulation versus caffeine as a fatigue countermeasure. Brain Stimul. 10, 1070–1078. doi: 10.1016/j.brs.2017.08.005
McKinley, R. A., Bridges, N., Walters, C. M., and Nelson, J. M. (2012). Modulating the brain at work using noninvasive transcranial stimulation. Neuroimage 59, 129–137. doi: 10.1016/j.neuroimage.2011.07.075
McKinley, R. A., McIntire, L. K., and Weisend, M. P. (2013). Acceleration of image analyst training with transcranial direct current stimulation. Behav. Neurosci. 127, 936–946. doi: 10.1037/a0034975
Meiron, O., and Lavidor, M. (2013). Unilateral prefrontal direct current stimulation effects are modulated by working memory load and gender. Brain Stimul. 6, 433–439. doi: 10.1016/j.brs.2012.05.014
Moliadze, V., Antal, A., and Paulus, W. (2010). Electrode-distance dependent after-effects of transcranial direct and random noise stimulation with extracephalic reference electrodes. Clin. Neurophysiol. 121, 2165–2171. doi: 10.1016/j.clinph.2010.04.033
Monte-Silva, K., Kuo, M. F., Liebetanz, D., Paulus, W., and Nitsche, M. A. (2010). Shaping the optimal repetition interval for cathodal transcranial direct current stimulation (tDCS). J. Neurophysiol. 103, 1735–1740. doi: 10.1152/jn.00924.2009
Murphy, P. J. (2002). Fatigue Management During Operations: A Commander’s Guide. Puckapunyal: Land Warfare Development Centre.
Murray, S. A., Ensign, W., Yanagi, M., and Ssc Pacific. (2010). Combat Situation Awareness (CSA) Model-Based Characterizations of Marine Corps Training and Operations (TR-1994). Arlington, VA: Office of Naval Research. doi: 10.21236/ADA535225
Nation, M., and Heflinger, C. A. (2006). Risk factors for serious alcohol and drug use: the role of psychosocial variables in predicting the frequency of substance use among adolescents. Am. J. Drug Alcohol Abuse 32, 415–433. doi: 10.1080/00952990600753867
Nelson, J. T., McKinley, R. A., Golob, E. J., Warm, J. S., and Parasuraman, R. (2014). Enhancing vigilance in operators with prefrontal cortex transcranial direct current stimulation (tDCS). Neuroimage 85, 909–917. doi: 10.1016/j.neuroimage.2012.11.061
Nieoullon, A. (2002). Dopamine and the regulation of cognition and attention. Prog. Neurobiol. 67, 53–83. doi: 10.1016/S0301-0082(02)00011-4
Nitsche, M. A., Cohen, L. G., Wassermann, E. M., Priori, A., Lang, N., Antal, A., et al. (2008). Transcranial direct current stimulation: state of the art 2008. Brain Stimul. 1, 206–223. doi: 10.1016/j.brs.2008.06.004
Nitsche, M. A., Liebetanz, D., Lang, N., Antal, A., Tergau, F., and Paulus, W. (2003a). Safety criteria for transcranial direct current stimulation (tDCS) in humans. Clin. Neurophysiol. 114, 2220–2222. doi: 10.1016/S1388-2457(03)00235-9
Nitsche, M. A., Schauenburg, A., Lang, N., Liebetanz, D., Exner, C., Paulus, W., et al. (2003b). Facilitation of implicit motor learning by weak transcranial direct current stimulation of the primary motor cortex in the human. J. Cogn. Neurosci. 15, 619–626. doi: 10.1162/089892903321662994
Noakes, T. D. (2011). Time to move beyond a brainless exercise physiology: the evidence for complex regulation of human exercise performance. Appl. Physiol. Nutr. Metab. 36, 23–35. doi: 10.1139/H10-082
Noakes, T. D. (2012). Fatigue is a brain-derived emotion that regulates the exercise behavior to ensure the protection of whole body homeostasis. Front. Physiol 3:82. doi: 10.3389/fphys.2012.00082
Nutt, D. J., Lingford-Hughes, A., Erritzoe, D., and Stokes, P. R. (2015). The dopamine theory of addiction: 40 years of highs and lows. Nat. Rev. Neurosci. 16, 305–312. doi: 10.1038/nrn3939
O’Connell, N. E., Cossar, J., Marston, L., Wand, B. M., Bunce, D., Moseley, G. L., et al. (2012). Rethinking clinical trials of transcranial direct current stimulation: Participant and assessor blinding is inadequate at intensities of 2mA. PLoS One 7:e47514. doi: 10.1371/journal.pone.0047514
Packmanta (2014). First Time Using Foc.us: Saw Bright White Flash, Passed Out Momentarily. Help. Available at: http://www.reddit.com/r/tDCS/comments/1y1vlz/first_time_using_focus_saw_bright_white_flash/
Paleri, P. (2008). National Security: Imperatives and Challenges. New Delhi: Tata McGraw-Hill Education.
Palm, U., Keeser, D., Schiller, C., Fintescu, Z., Reisinger, E., Nitsche, M. A., et al. (2008). Skin lesions after treatment with transcranial direct current stimulation (tDCS). Brain Stimul. 1, 386–387. doi: 10.1016/j.brs.2008.04.003
Pan, L., Zhang, D., Jiang, N., Sheng, X., and Zhu, X. (2017). Transcranial direct current stimulation versus user training on improving online myoelectric control for amputees. J. Neural Eng. 14:046019. doi: 10.1088/1741-2552/aa758e
Paneri, B., Adair, D., Thomas, C., Khadka, N., Patel, V., Tyler, W. J., et al. (2016). Tolerability of repeated application of transcranial electrical stimulation with limited outputs to healthy subjects. Brain Stimul. 9, 740–754. doi: 10.1016/j.brs.2016.05.008
Pascual-Leone, A., Horvath, J. C., and Robertson, E. M. (2012). “Enhancement of normal cognitive abilities through noninvasive brain stimulation,” in Cortical Connectivity: Brain Stimulation for Assessing and Modulating Cortical Connectivity and Function, eds R. Chen and J. C. Rothwell (Berlin: Springer), 207–249.
Pavlova, E., Kuo, M.-F., Nitsche, M. M., and Borg, J. (2013). P 77. Transcranial direct current stimulation of the premotor cortex aimed to improve fine motor skills of the hand. Clin. Neurophysiol. 124, e101–e102. doi: 10.1016/j.clinph.2013.04.155
Peña-Gómez, C., Vidal-Piñeiro, D., Clemente, I. C., Pascual-Leone,Á, and Bartrés-Faz, D. (2011). Down-regulation of negative emotional processing by transcranial direct current stimulation: effects of personality characteristics. PLoS One 6:e22812. doi: 10.1371/journal.pone.0022812
Pigeau, R., and McCann, C. (2000). “Redefining command and control,” in The Human in Command: Exploring the Modern Military Experience, eds R. Pigeau and C. McCann (Toronto: Springer), 163–184.
Poreisz, C., Boros, K., Antal, A., and Paulus, W. (2007). Safety aspects of transcranial direct current stimulation concerning healthy subjects and patients. Brain Res. Bull. 72, 208–214. doi: 10.1016/j.brainresbull.2007.01.004
Priori, A., Mameli, F., Cogiamanian, F., Marceglia, S., Tiriticco, M., Mrakic-Sposta, S., et al. (2008). Lie-specific involvement of dorsolateral prefrontal cortex in deception. Cereb. Cortex 18, 451–455. doi: 10.1093/cercor/bhm088
Ragert, P., Vandermeeren, Y., Camus, M., and Cohen, L. G. (2008). Improvement of spatial tactile acuity by transcranial direct current stimulation. Clin. Neurophysiol. 119, 805–811. doi: 10.1016/j.clinph.2007.12.001
Ribeaux, P., and Poppleton, S. E. (1978). Psychology and Work: An Introduction. London: Macmillan. doi: 10.1007/978-1-349-15983-3
Roche, N., Geiger, M., and Bussel, B. (2015). Mechanisms underlying transcranial direct current stimulation in rehabilitation. Ann. Phys. Rehabil. Med. 58, 214–219. doi: 10.1016/j.rehab.2015.04.009
Rossi, S., Hallett, M., Rossini, P., Pascual-Leone, A., and Safety of Tms Consensus Group. (2009). Safety, ethical considerations, and application guidelines for the use of transcranial magnetic stimulation in clinical practice and research. Clin. Neurophysiol. 120, 2008–2039. doi: 10.1016/j.clinph.2009.08.016
Royal Australian Air Force (2017). Air Forcer Doctrine Publication 1: The Air Force Approach to Personnel Capability Support. Canberra: Australian Department of Defence.
Rüsch, N., Rosé, C. P., Holzhausen, F., Mulfinger, N., Krumm, S., Corrigan, P. W., et al. (2017). Attitudes towards disclosing a mental illness among German soldiers and their comrades. Psychiatry Res. 258, 200–206. doi: 10.1016/j.psychres.2017.08.028
Sadleir, R. J., Vannorsdall, T. D., Schretlen, D. J., and Gordon, B. (2010). Transcranial direct current stimulation (tDCS) in a realistic head model. Neuroimage 51, 1310–1318. doi: 10.1016/j.neuroimage.2010.03.052
Salehinejad, M. A., Nejati, V., and Derakhshan, M. (2017). Neural correlates of trait resiliency: evidence from electrical stimulation of the dorsolateral prefrontal cortex (dLPFC) and orbitofrontal cortex (OFC). Pers. Individ. Dif. 106, 209–216. doi: 10.1016/j.paid.2016.11.005
Sarkar, A., Dowker, A., and Kadosh, R. C. (2014). Cognitive enhancement or cognitive cost: trait-specific outcomes of brain stimulation in the case of mathematics anxiety. J. Neurosci. 34, 16605–16610. doi: 10.1523/JNEUROSCI.3129-14.2014
Savulescu, J. (2006). “Genetic interventions and the ethics of enhancement of human beings,” in The Oxford Handbook on Bioethics, ed. B. Steinbock (Oxford: Oxford University Press), 516–535.
Schestatsky, P., Morales-Quezada, L., and Fregni, F. (2013). Simultaneous EEG monitoring during transcranial direct current stimulation. J. Vis. Exp. 76:e50426. doi: 10.3791/50426
Schülke, R., and Straube, B. (2017). Modulating the assessment of semantic speech-gesture relatedness via transcranial direct current stimulation of the left frontal cortex. Brain Stimul. 10, 223–230. doi: 10.1016/j.brs.2016.10.012
Sinha, R. (2011). New findings on biological factors predicting addiction relapse vulnerability. Curr. Psychiatry Rep. 13, 398–405. doi: 10.1007/s11920-011-0224-0
Smith, T. J., Marriott, B. P., Dotson, L., Bathalon, G. P., Funderburk, L., White, A., et al. (2012). Overweight and obesity in military personnel: sociodemographic predictors. Obesity 20, 1534–1538. doi: 10.1038/oby.2012.25
Sparing, R., and Mottaghy, F. M. (2008). Noninvasive brain stimulation with transcranial magnetic or direct current stimulation (TMS/tDCS)–from insights into human memory to therapy of its dysfunction. Methods 44, 329–337. doi: 10.1016/j.ymeth.2007.02.001
Stagg, C. J., and Nitsche, M. A. (2011). Physiological basis of transcranial direct current stimulation. Neuroscientist 17, 37–53. doi: 10.1177/1073858410386614
Stephens, J. A., Jones, K. T., and Berryhill, M. E. (2017). Task demands, tDCS intensity, and the COMT val158met polymorphism impact tDCS-linked working memory training gains. Sci. Rep. 7:13463. doi: 10.1038/s41598-017-14030-7
Streufert, S., and Streufert, S. C. (1968). Risk Taking in Military and Economic Decision Making: An Analysis via an Experimental Simulation (Technical Report No. 16). Arlington, VA: Office of Naval Research.
Suzuki, M., Okamura, N., Kawachi, Y., Tashiro, M., Arao, H., Hoshishiba, T., et al. (2008). Discrete cortical regions associated with the musical beauty of major and minor chords. Cogn. Affect. Behav. Neurosci. 8, 126–131. doi: 10.3758/CABN.8.2.126
Tanaka, S., Hanakawa, T., Honda, M., and Watanabe, K. (2009). Enhancement of pinch force in the lower leg by anodal transcranial direct current stimulation. Exp. Brain Res. 196, 459–465. doi: 10.1007/s00221-009-1863-9
Tang, M. F., and Hammond, G. R. (2013). Anodal transcranial direct current stimulation over auditory cortex degrades frequency discrimination by affecting temporal, but not place, coding. Eur. J. Neurosci. 38, 2802–2811. doi: 10.1111/ejn.12280
Teo, J. T., Swayne, O. B., Cheeran, B. J., Greenwood, R. J., and Rothwell, J. C. (2011). Human theta burst stimulation enhances subsequent motor learning and increases performance variability. Cereb. Cortex 21, 1627–1638. doi: 10.1093/cercor/bhq231
Thomas, J. L., Adler, A. B., Wittels, P., Enne, R., and Johannes, B. (2004). Comparing elite soldiers’ perceptions of psychological and physical demands during military training. Mil. Med. 169, 526–530. doi: 10.7205/MILMED.169.7.526
Tirre, W., and Gugerty, L. (1999). A Cognitive Correlates Analysis of Situation Awareness (AFRL-HE-AZ-TR-1998-0086). Mesa, AZ: United States Air Force Research Laboratory.
Truong, D. Q., Magerowski, G., Blackburn, G. L., Bikson, M., and Alonso-Alonso, M. (2013). Computational modeling of transcranial direct current stimulation (tDCS) in obesity: impact of head fat and dose guidelines. Neuroimage Clin. 2, 759–766. doi: 10.1016/j.nicl.2013.05.011
Tseng, P., Hsu, T.-Y., Chang, C.-F., Tzeng, O. J. L., Hung, D. L., Muggleton, N. G., et al. (2012). Unleashing potential: Transcranial direct current stimulation over the right posterior parietal cortex improves change detection in low-performing individuals. J. Neurosci. 32, 10554–10561. doi: 10.1523/JNEUROSCI.0362-12.2012
United States Air Force Chief Scientist (2010). Report on Technology Horizons: A Vision for Air Force Science & Technology During 2010-2030 (TR: XCUSAF). Washington, DC: United States Air Force. doi: 10.21236/ADA525912
United States Department of the Army (2005). Map Reading and Land Navigation (Field Manual 3-25.26). Washington, DC: United States Department of the Army.
Utz, K. S., Dimova, V., Oppenländer, K., and Kerkhoff, G. (2010). Electrified minds: transcranial direct current stimulation (tDCS) and Galvanic Vestibular Stimulation (GVS) as methods of non-invasive brain stimulation in neuropsychology–A review of current data and future implications. Neuropsychologia 48, 2789–2810. doi: 10.1016/j.neuropsychologia.2010.06.002
Vallerand, A. H., Cosler, P., Henningfield, J. E., and Galassini, P. (2015). Pain management strategies and lessons from the military: a narrative review. Pain Res. Manag. 20, 261–268. doi: 10.1155/2015/196025
van Hooff, M., McFarlane, A. C., Davies, C. E., Searle, A. K., Fairweather-Schmidt, A. K., Verhagen, A., et al. (2014). The Australian Defence Force mental health prevalence and wellbeing study: design and methods. Eur. J. Psychotraumatol. 5:23950. doi: 10.3402/ejpt.v5.23950
Vickers, J. N., and Lewinski, W. (2012). Performing under pressure: gaze control, decision making and shooting performance of elite and rookie police officers. Hum. Mov. Sci. 31, 101–117. doi: 10.1016/j.humov.2011.04.004
Vignaud, P., Mondino, M., Poulet, E., Palm, U., and Bruneli, J. (2018). Duration but not intensity influences transcranial direct current stimulation (tDCS) after-effects on cortical excitability. Neurophysiol. Clin. 48, 89–92. doi: 10.1016/j.neucli.2018.02.001
Vitor-Costa, M., Okuno, N. M., Bortolotti, H., Bertollo, M., Boggio, P. S., Fregni, F., et al. (2015). Improving cycling performance: Transcranial direct current stimulation increases time to exhaustion in cycling. PLoS One 10:e0144916. doi: 10.1371/journal.pone.0144916
Volkow, N. D., Fowler, J. S., Logan, J., Alexoff, D., Zhu, W., Telang, F., et al. (2009). Effects of modafinil on dopamine and dopamine transporters in the male human brain: clinical implications. JAMA 301, 1148–1154. doi: 10.1001/jama.2009.351
von Clausewitz, C. (1989). On War, eds and trans. M. Howard and P. Paret (Princeton, NJ: Princeton University Press).
Wallenius, C., Larsson, G., and Johansson, C. R. (2009). Military observers’ reactions and performance when facing danger. Mil. Psychol. 16, 211–229. doi: 10.1207/s15327876mp1604_1
Wang, J., Wang, Y., Hu, Z., and Li, X. (2014). Transcranial direct current stimulation of the dorsolateral prefrontal cortex increased pain empathy. Neuroscience 281, 202–207. doi: 10.1016/j.neuroscience.2014.09.044
Wiethoff, S., Hamada, M., and Rothwell, J. C. (2014). Variability in response to transcranial direct current stimulation of the motor cortex. Brain Stimul. 7, 468–475. doi: 10.1016/j.brs.2014.02.003
Wolfendale, J. (2008). Performance-enhancing technologies and moral responsibility in the military. Am. J. Bioeth. 8, 28–38. doi: 10.1080/15265160802014969
World Health Organization (1992). The ICD-10 Classification of Mental and Behavioural Disorders: Clinical Descriptions and Diagnostic Guidelines. Geneva: World Health Organization.
Ye, H., Chen, S., Huang, D., Zheng, H., Jia, Y., and Luo, J. (2015). Modulation of neural activity in the temporoparietal junction with transcranial direct current stimulation changes the role of beliefs in moral judgment. Front. Hum. Neurosci. 9:659. doi: 10.3389/fnhum.2015.00659
Young, L., Camprodon, J. A., Hauser, M., Pascual-Leone, A., and Saxe, R. (2010). Disruption of the right temporoparietal junction with transcranial magnetic stimulation reduces the role of beliefs in moral judgments. Proc. Natl. Acad. Sci. U.S.A. 107, 6753–6758. doi: 10.1073/pnas.0914826107
Keywords: biomedical enhancement, brain stimulation, cognitive enhancement, decision making, military, neuro-enhancement, transcranial direct current stimulation, tDCS
Citation: Davis SE and Smith GA (2019) Transcranial Direct Current Stimulation Use in Warfighting: Benefits, Risks, and Future Prospects. Front. Hum. Neurosci. 13:114. doi: 10.3389/fnhum.2019.00114
Received: 12 December 2018; Accepted: 14 March 2019;
Published: 18 April 2019.
Edited by:
Stephane Perrey, Université de Montpellier, FranceReviewed by:
Wei-Peng Teo, Nanyang Technological University, SingaporeRenato Massaferri, Universidade da Força Aérea Brazileira, Brazil
Copyright © 2019 Davis and Smith. This is an open-access article distributed under the terms of the Creative Commons Attribution License (CC BY). The use, distribution or reproduction in other forums is permitted, provided the original author(s) and the copyright owner(s) are credited and that the original publication in this journal is cited, in accordance with accepted academic practice. No use, distribution or reproduction is permitted which does not comply with these terms.
*Correspondence: Steven E. Davis, steven.davis@dst.defence.gov.au