- Cognitive Neuroscience Unit, School of Psychology, Deakin University, Geelong, VIC, Australia
Human responses to non-invasive brain stimulation (NIBS) techniques can be highly variable. Recently, priming protocols involving a conditioning round of NIBS applied to a target brain region prior to the application of a test protocol have shown promise in inducing more reliable effects. We investigated whether intra- or inter-regional priming of the left primary motor cortex (M1) using continuous theta burst stimulation (cTBS) can induce consistent, and reliable modulation of corticospinal excitability. Twenty healthy adults (six males) underwent four cTBS protocols. For intra-regional priming, cTBS was applied twice to the left M1 (M1-M1). For inter-regional M1 priming, cTBS was applied to the ipsilateral (left) dorsal premotor cortex (dPMC-M1), and ipsilateral (left) dorsolateral prefrontal cortex (DLPFC-M1). In the control condition, sham stimulation was applied to left M1, followed by active cTBS also applied to the left M1 (sham-M1). Each round of cTBS was separated by 10 min. Neuroplastic responses were indexed using motor evoked potentials (MEPs) elicited from the left M1 hand region, and measured from the contralateral first dorsal interosseous (right hand). MEP measurements were taken before the first round of cTBS priming, then immediately, 10, 20 and 30 min after the second test round of cTBS. The primary two-way repeated measures ANOVA revealed no significant differences in MEP responses across each condition (no main effects or interaction). Intra- and inter-regional priming of the left M1 using cTBS does not induce consistent neuroplastic effects. Further work is required to identify factors which contribute to such variability in human responses to NIBS.
Introduction
Neuroplasticity refers to the brain’s capacity for neural restructuring, and underlies our capacity to acquire knowledge, retain memories, and to recover from injury and trauma (Hebb, 1949; Pascual-Leone et al., 2005). Some of the most well characterized forms of neuroplasticity include long-term depression (LTD) and long term potentiation (LTP; Malenka and Bear, 2004). In the human neocortex, similar LTD- and LTP-like effects can be induced using non-invasive brain stimulation (NIBS) techniques (Fitzgerald et al., 2006; Ridding and Ziemann, 2010; Chung et al., 2016). Correspondingly, a large body of work is now dedicated to the application of NIBS for investigative, and clinical applications (Wassermann and Lisanby, 2001; Daskalakis, 2005; Guse et al., 2010; Jaafari et al., 2012).
Although NIBS techniques can modulate corticospinal excitability, the neuroplastic effects reported in humans are often highly variable, thus limiting their potential utility (Ridding and Ziemann, 2010; Pell et al., 2011; Hamada et al., 2012; Goldsworthy et al., 2014). One method that may induce more homogenous and predictable effects involves “priming” a target region with a conditioning bout of NIBS before administration of the test protocol (Karabanov et al., 2015). Recently, Goldsworthy et al. (2012, 2015) have shown that two rounds of continuous theta burst stimulation (cTBS; a NIBS technique involving the delivery of very high frequency electromagnetic pulse bursts in the theta range) applied intra-regionally to the human primary motor cortex (M1) can induce strong, and persistent suppression of corticospinal excitability lasting up to 2 h.
Another approach which may yield effective protocols for inducing robust neuroplastic effects is through inter-regional priming (Karabanov et al., 2015; Müller-Dahlhaus and Ziemann, 2015). In general, the effects of intra- and inter-regional priming using NIBS techniques can be described using the Bienenstock, Cooper and Munro (BCM) theory of homeostatic metaplasticity (Bienenstock et al., 1982; Karabanov et al., 2015). One of the predictions made by the BCM model is the inhibitory effects of an LTD-inducing paradigm can be reversed into facilitation if it is preceded by low-frequency stimulation (i.e., a LTD paradigm). For example, Pötter-Nerger et al. (2009) found that the usual inhibitory LTD-like effects on M1 corticospinal excitability induced by paired associative stimulation (PASN20–5 ms; a NIBS technique involving carefully timed electrical stimulation applied to peripheral nerves combined with transcranial magnetic stimulation; TMS) paradigm can be reversed into facilitation if it is first preceded by low-frequency repetitive TMS (rTMS1 Hz) applied to the ipsilateral dorsal premotor cortex (dPMC). Similarly, Hamada et al. (2009) have shown that the usual inhibitory LTD-like effects on M1 excitability induced by quadripulse stimulation (QPS30 ms; a NIBS technique involving the delivery of four precisely timed TMS pulses) can also be reversed into facilitation if preceded by low-frequency QPS50 ms applied over the supplementary motor area (SMA). Similar homeostatic metaplasttic effects have been demonstrated following intra-regional priming of M1 (Siebner et al., 2004; Hamada et al., 2008).
In the current study, we investigated whether priming the left-M1 intra- or inter-regionally with cTBS can induce more consistent, and robust neuroplastic effects. The dPMC and dorsolateral prefrontal cortex (DLPFC) ipsilateral to M1 were selected as they comprise a richly interconnected network which subserves motor control (Lu et al., 1994; Picard and Strick, 2001; Fang et al., 2005; Kantak et al., 2012). Four protocols were evaluated. For intra-regional priming, active cTBS was applied to the left M1 twice. For inter-regional priming of M1, cTBS was applied to the ipsilateral (left) dPMC, and ipsilateral (left) DLPFC. A control condition was included with sham stimulation applied to ipsilateral (left) M1. It was hypothesized that intra-regional priming of M1 would suppress corticospinal excitability (Goldsworthy et al., 2012, 2015). In accordance with the BCM model of homeostatic metaplasticity, it was anticipated that inter-regional priming of the ipsilateral dPMC, and DLPFC with cTBS would facilitate M1 excitability (Hamada et al., 2009; Pötter-Nerger et al., 2009).
Materials and Methods
Participants
Twenty right-handed participants (M = 26.45, SD = 3.07; range = 19–33; six males) attended four sessions. Participants had no self-reported history of psychiatric, or neurological illness. Participants were screened for contraindications to TMS according to standard exclusionary criteria (Rossi et al., 2009). This study was carried out in accordance with the recommendations of the National Statement of Ethical Conduct in Human Research and approved by the Deakin University Human Research Ethics Committee (DUHREC) with written informed consent from all subjects. All subjects gave written informed consent in accordance with the Declaration of Helsinki. The protocol was approved by the DUHREC. All participants were right-handed according to the Edinburgh Handedness Inventory (M = 83.71, SD = 21.35; range = 40–100; Oldfield, 1971).
Electromyography Recordings
Electromyography (EMG) was measured using the Trigno Wireless EMG system (Delsys, Natick, MA, USA). The electrode sensor was placed over the belly-tendon of the first dorsal interosseous (FDI) muscle of the right hand. EMG recordings (PowerLab 4/35, ADInstruments) were amplified (×1000), bandpass filtered (0.3 Hz–1 kHz), digitized (10 kHz) and epoched to the TMS pulse (−100 ms to 400 ms). Data was recorded, saved and processed offline (Labchart 7, ADInstruments).
Single and Paired-Pulse Transcranial Magnetic Stimulation
Single-pulse transcranial magnetic stimulation (spTMS) was administered using a Magstim 2002 unit (Magstim, UK) via a 70 mm figure 8 coil which generates a monophasic pulse with a 100 μm rise time of 1 ms (Magstim, 2011). The coil was held tangential to the scalp at a 45-degree angle to the midline, with the lead pointing in a postero-lateral direction. The presumed motor hand region location was probed in intervals of 10 mm over the left M1 to identify the location which produced the strongest motor evoked potential (MEP) response (i.e., the hand motor “hot spot”). The test stimulus was delivered at the % maximal stimulator output (%MSO) intensity which induced an average peak-to-peak MEP of approximately 1 mV across 10 trials. Changes in corticospinal excitability were quantified as peak-to-peak MEP amplitudes before the first (priming), and after the second (test) round of cTBS. The %MSO was left unchanged for spTMS. There were five measurement points; pre-intervention (baseline), and four post-intervention time points after the second test bout of cTBS (0, 10, 20 and 30 min). For each block, 10 TMS pulses were delivered manually at a rate of 4–6 s to avoid rhythmic delivery. Paired-pulse TMS (ppTMS) was used to investigate intra-cortical inhibitory, and facilitatory mechanisms but is not reported in this paper. For ppTMS, the conditioning and test pulses were delivered with a 70 mm figure 8 coil using the Magstim Bistim2 (Magstim, UK) connected via the Bistim Module.
Continuous Theta Burst Stimulation
cTBS was administered using a Magstim Rapid2 (Magstim, UK) via a 70 mm figure 8 air cooled coil which induces a biphasic pulse of 0.5 ms duration with 80 μs rise time (Magstim, 2007a). The coil was held tangential to the scalp at a 45-degree angle to the midline for all sites, and held in place by the researcher, supported by a mechanical stand. The cTBS protocol comprised three pulses delivered at 50 Hz repeated at 5 Hz for 40 s for a total of 600 pulses at 70% resting motor threshold (RMT; cTBS60070%RMT; Huang et al., 2005; Goldsworthy et al., 2012). RMT for cTBS was defined as a discernible MEP of peak-to-peak amplitude greater than 50 μV in three out of five trials and was established after baseline measurements with the Magstim Rapid2 using a non-air cooled figure 8 70 mm coil. Sham stimulation was delivered using an identical 70 mm figure 8 air cooled placebo coil which produces a similar discharge sound, but does not stimulate the cortex (Magstim, 2007b). The experimental design is illustrated in Figure 1.
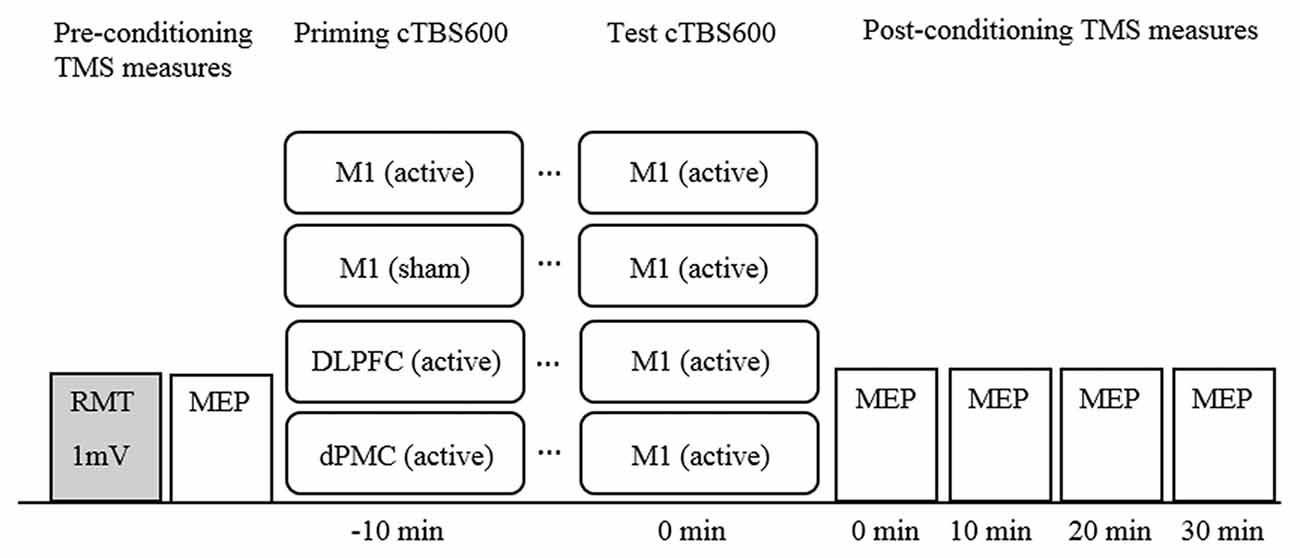
Figure 1. Timeline of experimental design. Note. cTBS600, continuous theta burst stimulation (600 pulses); DLPFC, dorsolateral prefrontal cortex; dPMC, dorsal premotor cortex; Ml, primary motor cortex; MEP, motor evoked potential; mV, millivolt; RMT, resting motor threshold; TMS, transcranial magnetic stimulation.
Experimental Conditions
Participants underwent all four experimental conditions with the order of conditions determined using a randomized computer-generated sequence. Participants attended sessions at approximately the same time of day for each session. Sessions were separated by a minimum of 5 days to avoid carry over effects (Bäumer et al., 2003). The basic paradigm comprised a conditioning-test protocol with an inter-train-interval of 10 min all applied to the left hemisphere (Figure 1). This interval was selected because it has been shown to induce robust neuroplastic effects (Goldsworthy et al., 2012), but also to maintain consistency across conditions. M1 was defined functionally as the FDI “motor hotspot”. The dPMC was defined as 2.5 cm anterior to the motor hot spot on the basis of neuroimaging (Picard and Strick, 2001), and previous rTMS studies (Rizzo et al., 2004). The DLPFC was defined as 5 cm anterior to the M1 hot spot (George et al., 1995; Loo et al., 1999).
Statistical Analysis
Only spTMS MEP data were analyzed. Outliers were defined as values exceeding absolute z-scores of 3.29. To reduce the influence of outliers, extreme values were replaced with the M + 3 SD (Field, 2009). Less than 1% of the data were transformed. Data were checked visually for normality using histograms. To better approximate normality and maintain positive values, log10 + 1 transformations were applied to raw spTMS MEP values (Tabachnick and Fidell, 2007). The primary analysis was a two-way repeated measures ANOVA with protocol (four levels: M1-M1, sham-M1, DLPFC-M1, dPMC-M1), and time (five levels: baseline, 0, 10, 20 and 30 min post-cTBS) as within-subjects factors. One-way repeated measures ANOVA (four levels: M1-M1, sham-M1, DLPFC-M1, dPMC-M1) were conducted to test for pre-conditioning differences in the %MSO 1 mV test stimulus, %MSO cTBS RMT, and baseline MEP values (transformed data). Mauchley’s test indicated that sphericity could be assumed for all analyses. Analyses were conducted offline (SPSS version 22 for Windows; SPSS Inc.). For ease of comparison, figures were generated using normalized MEP values by dividing spTMS MEP responses at each post-intervention time point (0, 10, 20 and 30 min) with pre-intervention mean baseline MEP responses. Normalized MEP values were not used for any parametric tests.
For categorical data analysis, nominal 20% post-measurement change from baseline levels were used to categorize participants as having an “expected” or “unexpected” response according to a priori directions of predicted change. For M1-M1 and sham-M1 conditions, participants with at least one post-intervention normalized MEP response ≤ 0.8, were classified as “expected”. For the DLPFC-M1 and dPMC-M1 conditions, participants with at least one post intervention response ≥1.2 were classified as “expected”. Cochrane’s Q analysis (exact p-value reported) was used to test whether the protocols differed in inducing patterns of “expected” vs. “unexpected” results. An alternative approach using the average of all post-measurement time points to categorize participants was performed and is included as Supplementary Material (refer to Data Sheet 1). Finally, individual response plots were generated for each participant across each measurement point, and protocol using normalized values.
Results
Participants completed each session without major complaints, or adverse reactions (refer to Supplementary Figure S1). Pre-conditioning values for the %MSO 1 mV test stimulus (F(3,57)= 1.472, p = 0.232, η2 = 0.072), %MSO cTBS RMT (F(3,57) = 1.728, p = 0.171, partial η2 = 0.083), and baseline MEP values (F(3,57) = 1.570, p = 0.207, partial η2 = 0.076) did not differ across conditions (Table 1).

Table 1. Average (SD) 1 mV test stimulus and resting motor threshold (RMT) for continuous theta burst stimulation (cTBS) expressed as a percentage of maximal stimulator output (%MSO) and mean (SD) raw pre-conditioning motor evoked potential (MEP) values across each protocol.
For the main analysis comparing the effect of all four protocols on MEP responses, the two-way repeated measures ANOVA revealed no main effect of time, F(4,76) = 0.683, p = 0.606, partial η2 = 0.035; protocol, F(3,57) = 0.235, p = 0.872, partial η2 = 0.012; or time*protocol interaction, F(12, 228) = 0.930, p = 0.518, partial η2 = 0.047. This indicates that M1 corticospinal excitability levels did not consistently differ between conditions (Figure 2). This was despite an increase in corticospinal excitability in the DLPFC-M1 condition from the second (10 min) post-measurement time points, and suppression in the M1-M1 condition at the first post-measurement time point.
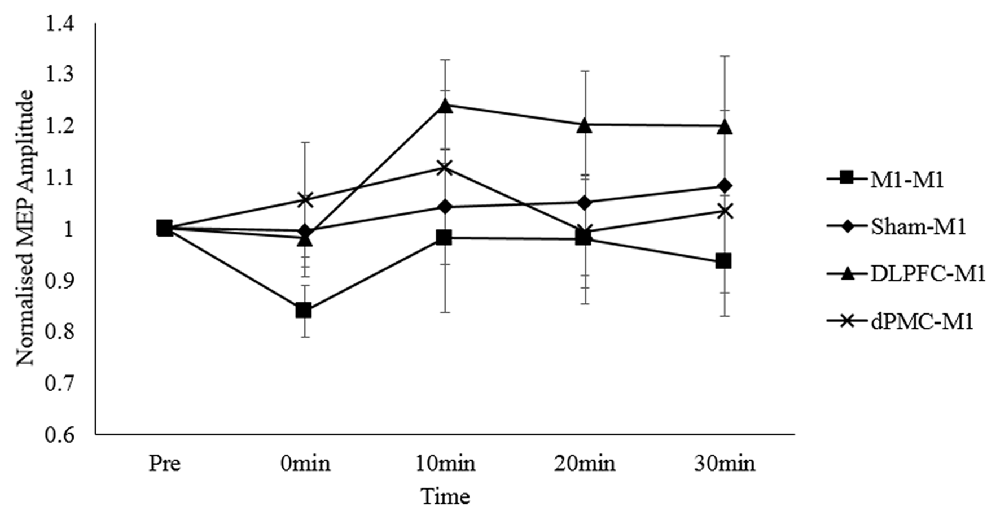
Figure 2. Normalized MEP amplitudes (standard errors) across each time point as a function of protocol. Note. DLPFC, dorsolateral prefrontal cortex; dPMC, dorsal premotor cortex; Ml, primary motor cortex.
Cochrane’s Q showed that the proportion of individuals with an “expected” vs. “unexpected” response did not differ across conditions, = 5.866, p = 0.136. This indicates that the protocols did not differ in inducing consistent and predicted inhibitory or facilitatory effects (Table 2).
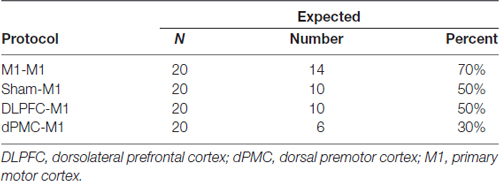
Table 2. Proportion of participants classified as having an expected or unexpected response as a function of protocol.
Normalized inter-individual values are presented in Figure 3. As observed, individual responses across each post-measurement point and protocol were highly variable.
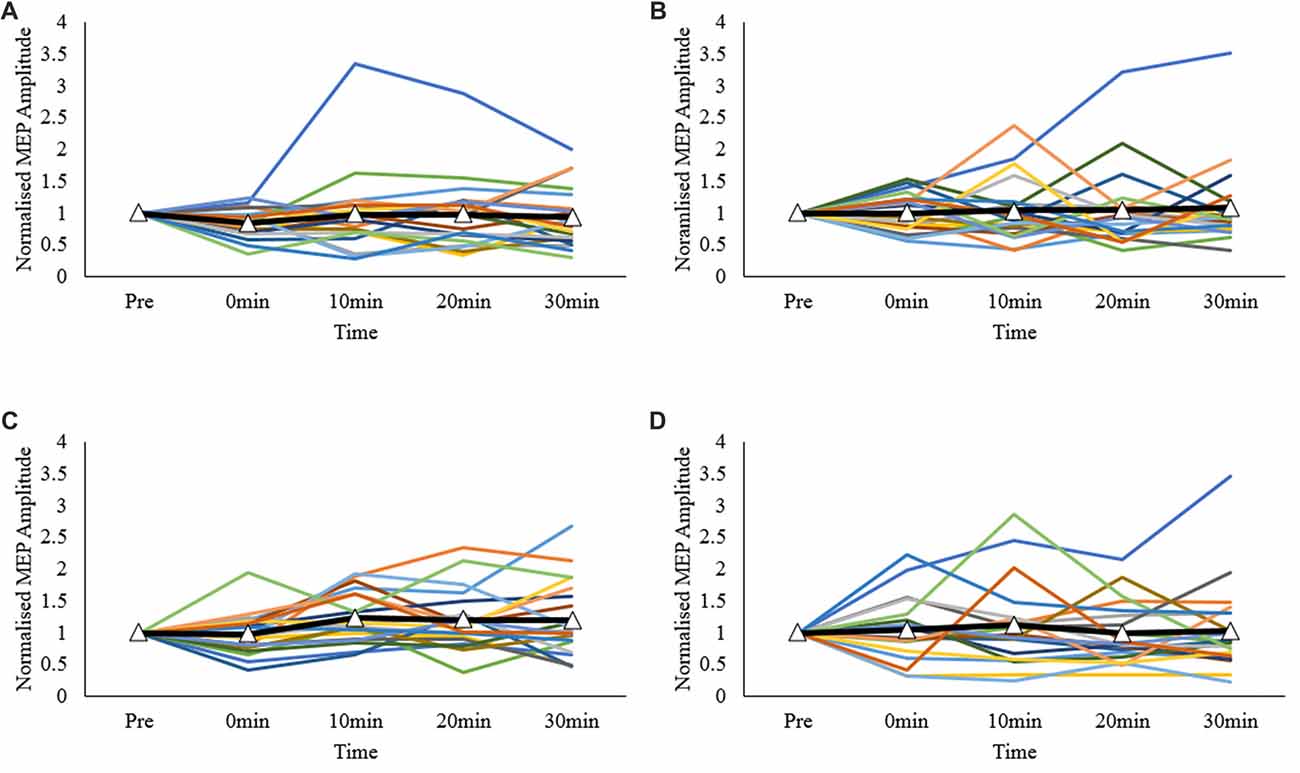
Figure 3. Individual MEP response plots expressed as normalized MEP amplitudes; (A) primary motor cortex (M1)-M1, (B) sham-M1, (C) dorsolateral prefrontal cortex-M1 (DLPFC-M1) and (D) dorsal premotor cortex-M1 (dPMC-M1) conditions. Bolded lines with triangle markers indicate the mean.
Discussion
The current study investigated whether intra- or inter-regional priming of the ipsilateral M1 with repeated cTBS60070%RMT trains can induce homogenous, and predictable neuroplastic effects on corticospinal excitability compared to a single round of cTBS60070%RMT. Overall, it was found that neither intra- nor inter-regional priming of left-M1 induced robust neuromodulation of M1 corticospinal excitability. For the categorical data, the proportion of individuals with an expected inhibitory or facilitatory response did not differ across conditions. Finally, individual response plots showed that participant responses to each protocol were highly variable.
cTBS of M1—Neurological Mechanisms
The overall null findings indicate that sham-M1 stimulation did not have significant inhibitory effects at the group level. Early studies reported that a single administration of cTBS80%Active motor threshold (AMT) can induce relatively strong suppression of M1 corticospinal excitability (Huang et al., 2005). These effects are described as “LTD-like”, and have been shown to be N-methyl-D-aspartate receptor dependent (Huang et al., 2007; Pell et al., 2011). More recently, studies with larger samples have shown that the effects of a single administration of cTBS60080%AMT can have both LTD-like and LTP-like effects (Hamada et al., 2012). Interestingly, individuals for whom TMS can more readily recruit late I-wave activity (when elicited with anterior-posteriorly induced currents) tend to show the expected suppressive effects, whilst individuals who show early I-wave recruitment tend to show facilitatory responses (Hamada et al., 2012). Future studies could examine whether differences in early- and late-I wave recruitment can be used to better predict “expected” and “unexpected” neuroplastic responses to cTBS60070%RMT.
Intra-Regional Priming of M1—Neurological Mechanisms
At least four studies have examined the effects of intra-regional priming of M1 with cTBS using different inter-train-intervals, and stimulation parameters with varying results. Gamboa et al. (2011) reported that two bouts of cTBS60080%AMT spaced by 20 min enhanced early suppression of M1, whilst Goldsworthy et al. (2012, 2015) showed that two rounds of cTBS60070% RMT spaced by 10 min induced robust suppression of corticospinal excitability lasting up to 2 h. In contrast, Gamboa et al. (2010, 2011) found that double application of cTBS60080% AMT attenuated the LTD response at short intervals (0 or 5 min), and Murakami et al. (2012) reported that sequential bouts of cTBS60080% AMT at longer intervals (15 min) induced facilitation. The differences in findings are likely due to a combination of factors including different cTBS parameters (e.g., the time interval between successive bouts, the use of AMT vs. RMT), and the activation of the target muscle required to determine AMT prior to conditioning (Gentner et al., 2008).
As a whole, these findings indicate that intra-regional priming of M1 can induce either homeostatic or non-homeostatic metaplastic effects, depending on the inter-train-interval. Intra-regional priming of M1 using paired trains of cTBS60070%RMT at 10 min appears to induce non-homesotatic metaplastic effects. The strong and long lasting suppression of M1 excitability for up to 2 h over and above the inhibitory effects following a single round of cTBS70%RMT (Goldsworthy et al., 2012, 2015) may be due to late phase-LTD consolidation as demonstrated in animal models (Nguyen et al., 1994; Karabanov et al., 2015). In contrast, paired trains of cTBS delivered at short (0 or 5 min) or long intervals (15 min) induces facilitatory effects (Gamboa et al., 2011; Murakami et al., 2012). The reversal of the expected inhibitory effects can be explained with the BCM theory of metaplasticity (Bienenstock et al., 1982), akin to those observed in the animal literature (Abraham and Bear, 1996). Although there was some evidence of suppression of M1 corticospinal excitability in the M1-M1 condition (particularly the first post-measurement time point), the analysis failed to reach significance. Future investigations could directly compare the effect of different inter-train-intervals using a repeated measures design to better control for individual, and methodological differences.
Inter-Regional Priming of M1—Neurological Mechanisms
Two studies examining the effects of inter-regional priming of M1 with inhibitory NIBS protocols have shown that the expected suppressive effects can be reversed in accordance with the predictions made by the BCM theory (Bienenstock et al., 1982). Pötter-Nerger et al. (2009) reported that priming the dPMC with low frequency rTMS1 Hz reversed the usual inhibitory effects of paired associative stimulation (PASN20–5 ms) applied over the ipsilateral (left) M1 into facilitation. Similarly, Hamada et al. (2009) reported that priming the SMA with low frequency QPS50 ms reversed the usual inhibitory effects of QPS30 ms applied over the left M1 into facilitation. Although visual inspection of Figure 2 shows facilitation of corticospinal excitability in the DLPFC-M1 condition, this failed to reach significance at the group level. Thus, we report that inter-regional priming of M1 with cTBS60070%RMT via the ipsilateral DLPFC, or dPMC does not induce consistent neuroplastic effects.
As mentioned, the reversal of expected neuroplastic effects in previous studies are in line with the predictions made by the BCM theory of metaplasticity (Bienenstock et al., 1982; Pötter-Nerger et al., 2009; Murakami et al., 2012). Although the precise neurological mechanisms that underpin these effects in humans are unknown (Hamada et al., 2009; Pötter-Nerger et al., 2009), because the SMA and dPMC both have connections with M1 (Picard and Strick, 2001; Dum and Strick, 2005; Kantak et al., 2012) the homeostatic-like effects reported in past studies may involve cortico-cortical pathways. A limitation of the current study was MEP responses were not collected during the inter-train-interval between each round of cTBS60070%RMT. Furthermore, the sham-M1 protocol (i.e., the control condition) failed to induce an inhibitory effect at the group level. Nonetheless, the lack of consistent effects at the group level across all conditions makes it difficult to draw strong conclusions regarding potential neurological mechanisms induced by inter-regional priming of M1 with cTBS60070%RMT. However, this does not preclude the use of other protocols (e.g., iTBS), localization methods (e.g., neuroimaging), or parameters (e.g., different inter-train-intervals) in future investigations.
Expected vs. Unexpected Responses
The categorical data analysis showed that the proportion of participants with an “expected” vs. “unexpected” response did not differ across conditions. The findings of the current study are largely consistent with the known inter-individual variability in responses following NIBS (Hamada et al., 2012). For example, both Hamada et al. (2012) and Goldsworthy et al. (2014) found that one round of cTBS60080% AMT applied to the left-M1 inducted the expected inhibitory response in only 25%–30% of participants. In the current study, inter-regional priming of the DLPFC (DLPFC-M1 condition) and dPMC (dPMC-M1 condition) only induced the expected facilitatory response in 50% and 30% of participants, respectively. Thus, we report similar variability even with inter-regional priming. Goldsworthy et al. (2014) have shown that cTBS60070% RMT induces inhibition in 70% of participants. In the current study, cTBS60070% RMT was used for both the conditioning and test bout. We found that M1-M1, and sham-M1 stimulation induced suppression in 70% and 50% of participants, respectively. The differences in reported findings between cTBS60080% AMT and cTBS60070% RMT may be due to the prior activation of the target muscle when establishing AMT (Gentner et al., 2008).
Inter- and Intra-Individual Responses
The overall null findings in the primary analysis is most likely driven by the high degree of intra- and inter-individual variability between protocols and across each measurement time point. Human responses to NIBS are known to be highly variable (Ridding and Ziemann, 2010; Hamada et al., 2012; Suppa et al., 2016). In one of the largest single studies to date (n = 57), Hamada et al. (2012) showed that a single application of cTBS, or intermittent TBS (iTBS) to the left-M1 resulted in highly variable neuroplastic responses. So much so, there were no overall differences at the group level. In the current study, it was also found that participant responses to intra- and inter-regional priming of left M1 using cTBS60070% RMT were highly variable. The factors which contribute to such variability remain to be elucidated but may include the prior activation history of a target region (Gentner et al., 2008), genetic factors (Cheeran et al., 2008), intrinsic fluctuations in corticospinal excitability (Kiers et al., 1993), individual differences in inter-neural networks (Hamada et al., 2012), and inherent variability in human responses to NIBS (Ridding and Ziemann, 2010).
Conclusion
The current investigation found that neither intra- nor inter-regional priming of the left M1 with cTBS60070%RMT induced consistent, and robust effects on corticospinal excitability. A high degree of inter-individual variability was observed regardless of whether the prime was applied inter- or intra-regionally. The neural mechanisms underpinning these findings are not clear. Potential mechanisms may include LTD-like mechanisms, or homeostatic and non-homeostatic metaplasticity. Further work is required to better understand the factors contributing to this variability. This in turn can be used to better inform research, and clinical parameters involving priming protocols.
Author Contributions
All authors named in this publication contributed equally to the design, implementation, and collection of data. Each author also contributed to the drafting, revision and preparation of the manuscript. All authors provided approval for final manuscript to be submitted for publication.
Funding
This work was wholly funded by Deakin University (Australia). PGE was supported by a Career Development Fellowship from the National Health and Medical Research Council (Australia; GNT1052073).
Conflict of Interest Statement
The authors declare that the research was conducted in the absence of any commercial or financial relationships that could be construed as a potential conflict of interest.
Supplementary Material
The Supplementary Material for this article can be found online at: https://www.frontiersin.org/articles/10.3389/fnhum.2018.00123/full#supplementary-material
References
Abraham, W. C., and Bear, M. F. (1996). Metaplasticity: the plasticity of synaptic plasticity. Trends Neurosci. 19, 126–130. doi: 10.1016/s0166-2236(96)80018-x
Bäumer, T., Lange, R., Liepert, J., Weiller, C., Siebner, H. R., Rothwell, J. C., et al. (2003). Repeated premotor rTMS leads to cumulative plastic changes of motor cortex excitability in humans. Neuroimage 20, 550–560. doi: 10.1016/s1053-8119(03)00310-0
Bienenstock, E. L., Cooper, L. N., and Munro, P. W. (1982). Theory for the development of neuron selectivity: orientation specificity and binocular interaction in visual cortex. J. Neurosci. 2, 32–48. doi: 10.1142/9789812795885_0006
Cheeran, B., Talelli, P., Mori, F., Koch, G., Suppa, A., Edwards, M., et al. (2008). A common polymorphism in the brain-derived neurotrophic factor gene (BDNF) modulates human cortical plasticity and the response to rTMS. J. Physiol. 586, 5717–5725. doi: 10.1113/jphysiol.2008.159905
Chung, S. W., Hill, A. T., Rogasch, N. C., Hoy, K. E., and Fitzgerald, P. B. (2016). Use of theta-burst stimulation in changing excitability of motor cortex: A systematic review and meta-analysis. Neurosci. Biobehav. Rev. 63, 43–64. doi: 10.1016/j.neubiorev.2016.01.008
Daskalakis, Z. J. (2005). Repetitive transcranial magnetic stimulation for the treatment of depression: To stimulate or not to stimulate? J. Psychiatry Neurosci. 30, 81–82. Available online at: http://link.galegroup.com/apps/doc/A129810816/AONE?u=deakin&sid=AONE&xid=d30fc5ec.
Dum, R. P., and Strick, P. L. (2005). Frontal lobe inputs to the digit representations of the motor areas on the lateral surface of the hemisphere. J. Neurosci. 25, 1375–1386. doi: 10.1523/jneurosci.3902-04.2005
Fang, P. C., Stepniewska, I., and Kaas, J. H. (2005). Ipsilateral cortical connections of motor, premotor, frontal eye and posterior parietal fields in a prosimian primate, Otolemur garnetti. J. Comp. Neurol. 490, 305–333. doi: 10.1002/cne.20665
Fitzgerald, P. B., Fountain, S., and Daskalakis, Z. J. (2006). A comprehensive review of the effects of rTMS on motor cortical excitability and inhibition. Clin. Neurophysiol. 117, 2584–2596. doi: 10.1016/j.clinph.2006.06.712
Gamboa, O. L., Antal, A., Laczo, B., Moliadze, V., Nitsche, M. A., and Paulus, W. (2011). Impact of repetitive theta burst stimulation on motor cortex excitability. Brain Stimul. 4, 145–151. doi: 10.1016/j.brs.2010.09.008
Gamboa, O. L., Antal, A., Moliadze, V., and Paulus, W. (2010). Simply longer is not better: reversal of theta burst after-effect with prolonged stimulation. Exp. Brain Res. 204, 181–187. doi: 10.1007/s00221-010-2293-4
Gentner, R., Wankerl, K., Reinsberger, C., Zeller, D., and Classen, J. (2008). Depression of human corticospinal excitability induced by magnetic theta-burst stimulation: Evidence of rapid polarity-reversing metaplasticity. Cereb. Cortex 18, 2046–2053. doi: 10.1093/cercor/bhm239
George, M. S., Wassermann, E. M., Williams, W. A., Callahan, A., Ketter, T. A., Basser, P., et al. (1995). Daily repetitive transcranial magnetic stimulation (rTMS) improves mood in depression. Neuroreport 6, 1853–1856. doi: 10.1097/00001756-199510020-00008
Goldsworthy, M. R., Müller-Dahlhaus, F., Ridding, M. C., and Ziemann, U. (2014). Inter-subject variability of LTD-like plasticity in human motor cortex: a matter of preceding motor activation. Brain Stimul. 7, 864–870. doi: 10.1016/j.brs.2014.08.004
Goldsworthy, M. R., Müller-Dahlhaus, F., Ridding, M. C., and Ziemann, U. (2015). Resistant against de-depression: LTD-like plasticity in the human motor cortex induced by spaced cTBS. Cereb. Cortex 25, 1724–1734. doi: 10.1093/cercor/bht353
Goldsworthy, M. R., Pitcher, J. B., and Ridding, M. C. (2012). The application of spaced theta burst protocols induces long-lasting neuroplastic changes in the human motor cortex. Eur. J. Neurosci. 35, 125–134. doi: 10.1111/j.1460-9568.2011.07924.x
Guse, B., Falkai, P., and Wobrock, T. (2010). Cognitive effects of high-frequency repetitive transcranial magnetic stimulation: a systematic review. J. Neural. Transm. 117, 105–122. doi: 10.1007/s00702-009-0333-7
Hamada, M., Hanajima, R., Terao, Y., Okabe, S., Nakatani-Enomoto, S., Furubayashi, T., et al. (2009). Primary motor cortical metaplasticity induced by priming over the supplementary motor area. J. Physiol. 587, 4845–4862. doi: 10.1113/jphysiol.2009.179101
Hamada, M., Murase, N., Hasan, A., Balaratnam, M., and Rothwell, J. C. (2012). The role of interneuron networks in driving human motor cortical plasticity. Cereb. Cortex 23, 1593–1605. doi: 10.1093/cercor/bhs147
Hamada, M., Terao, Y., Hanajima, R., Shirota, Y., Nakatani-Enomoto, S., Furubayashi, T., et al. (2008). Bidirectional long-term motor cortical plasticity and metaplasticity induced by quadripulse transcranial magnetic stimulation. J. Physiol. 586, 3927–3947. doi: 10.1113/jphysiol.2008.152793
Hebb, D. O. (1949). The Organization of Behavior: A Neuropsychological Theory. New York, NY: John Wiley & Sons, Inc.
Huang, Y.-Z., Chen, R.-S., Rothwell, J. C., and Wen, H.-Y. (2007). The after-effect of human theta burst stimulation is NMDA receptor dependent. Clin. Neurophysiol. 118, 1028–1032. doi: 10.1016/j.clinph.2007.01.021
Huang, Y. Z., Edwards, M. J., Rounis, E., Bhatia, K. P., and Rothwell, J. C. (2005). Theta burst stimulation of the human motor cortex. Neuron 45, 201–206. doi: 10.1016/j.neuron.2004.12.033
Jaafari, N., Rachid, F., Rotge, J.-Y., Polosan, M., El-Hage, W., Belin, D., et al. (2012). Safety and efficacy of repetitive transcranial magnetic stimulation in the treatment of obsessive-compulsive disorder: a review. World J. Biol. Psychiatry 13, 164–177. doi: 10.3109/15622975.2011.575177
Kantak, S. S., Stinear, J. W., Buch, E. R., and Cohen, L. G. (2012). Rewiring the brain: potential role of the premotor cortex in motor control, learning and recovery of function following brain injury. Neurorehabil. Neural. Repair. 26, 282–292. doi: 10.1177/1545968311420845
Karabanov, A., Ziemann, U., Hamada, M., George, M. S., Quartarone, A., Classen, J., et al. (2015). Consensus paper: probing homeostatic plasticity of human cortex with non-invasive transcranial brain stimulation. Brain Stimul. 8, 993–1006. doi: 10.1016/j.brs.2015.06.016
Kiers, L., Cros, D., Chiappa, K., and Fang, J. (1993). Variability of motor potentials evoked by transcranial magnetic stimulation. Electroencephalogr. Clin. Neurophysiol. 89, 415–423. doi: 10.1016/0168-5597(93)90115-6
Loo, C., Mitchell, P., Sachdev, P., McDarmont, B., Parker, G., and Gandevia, S. (1999). Double-blind controlled investigation of transcranial magnetic stimulation for the treatment of resistant major depression. Am. J. Psychiatry 156, 946–948. doi: 10.1176/ajp.156.6.946
Lu, M. T., Preston, J. B., and Strick, P. L. (1994). Interconnections between the prefrontal cortex and the premotor areas in the frontal lobe. J. Comp. Neurol. 341, 375–392. doi: 10.1002/cne.903410308
Magstim. (2007a). Magstim Double 70mm Air Film Coil-Operating Manual 3910-23-00. UK: The Magstim Company Ltd.
Magstim. (2007b). Magstim Double 70mm Air Film Placebo Coil-Operating Manual 3950-23-00. UK: The Magstim Company Ltd.
Magstim. (2011). Magstim Bistim2 Operating Manual (MOP02-EN Revision 1). UK: The Magstim Company Ltd.
Malenka, R. C., and Bear, M. F. (2004). LTP and LTD: an embarrassment of riches. Neuron 44, 5–21. doi: 10.1016/j.neuron.2004.09.012
Müller-Dahlhaus, F., and Ziemann, U. (2015). Metaplasticity in human cortex. Neuroscientist 21, 185–202. doi: 10.1177/1073858414526645
Murakami, T., Müller-Dahlhaus, F., Lu, M. K., and Ziemann, U. (2012). Homeostatic metaplasticity of corticospinal excitatory and intracortical inhibitory neural circuits in human motor cortex. J. Physiol. 590, 5765–5781. doi: 10.1113/jphysiol.2012.238519
Nguyen, P. V., Abel, T., and Kandel, E. R. (1994). Requirement of a critical period of transcription for induction of a late phase of LTP. Science 265, 1104–1107. doi: 10.1126/science.8066450
Oldfield, R. C. (1971). The assessment and analysis of handedness: the Edinburgh inventory. Neuropsychologia 9, 97–113. doi: 10.1016/0028-3932(71)90067-4
Pascual-Leone, A., Amedi, A., Fregni, F., and Merabet, L. B. (2005). The plastic human brain cortex. Annu. Rev. Neurosci. 28, 377–401. doi: 10.1146/annurev.neuro.27.070203.144216
Pell, G. S., Roth, Y., and Zangen, A. (2011). Modulation of cortical excitability induced by repetitive transcranial magnetic stimulation: influence of timing and geometrical parameters and underlying mechanisms. Prog. Neurobiol. 93, 59–98. doi: 10.1016/j.pneurobio.2010.10.003
Picard, N., and Strick, P. L. (2001). Imaging the premotor areas. Curr. Opin. Neurobiol. 11, 663–672. doi: 10.1016/s0959-4388(01)00266-5
Pötter-Nerger, M., Fischer, S., Mastroeni, C., Groppa, S., Deuschl, G., Volkmann, J., et al. (2009). Inducing homeostatic-like plasticity in human motor cortex through converging corticocortical inputs. J. Neurophysiol. 102, 3180–3190. doi: 10.1152/jn.91046.2008
Ridding, M., and Ziemann, U. (2010). Determinants of the induction of cortical plasticity by non-invasive brain stimulation in healthy subjects. J. Physiol. 588, 2291–2304. doi: 10.1113/jphysiol.2010.190314
Rizzo, V., Siebner, H. R., Modugno, N., Pesenti, A., Munchau, A., Gerschlager, W., et al. (2004). Shaping the excitability of human motor cortex with premotor rTMS. J. Physiol. 554, 483–495. doi: 10.1113/jphysiol.2003.048777
Rossi, S., Hallett, M., Rossini, P. M., and Pascual-Leone, A. (2009). Safety, ethical considerations and application guidelines for the use of transcranial magnetic stimulation in clinical practice and research. Clin. Neurophysiol. 120, 2008–2039. doi: 10.1016/j.clinph.2009.08.016
Siebner, H. R., Lang, N., Rizzo, V., Nitsche, M. A., Paulus, W., Lemon, R. N., et al. (2004). Preconditioning of low-frequency repetitive transcranial magnetic stimulation with transcranial direct current stimulation: evidence for homeostatic plasticity in the human motor cortex. J. Neurosci. 24, 3379–3385. doi: 10.1523/jneurosci.5316-03.2004
Suppa, A., Huang, Y.-Z., Funke, K., Ridding, M., Cheeran, B., Di Lazzaro, V., et al. (2016). Ten years of theta burst stimulation in humans: established knowledge, unknowns and prospects. Brain Stimul. 9, 323–335. doi: 10.1016/j.brs.2016.01.006
Keywords: corticospinal excitability, metaplasticity, motor cortex, neuroplasticity, theta burst stimulation, transcranial magnetic stimulation
Citation: Do M, Kirkovski M, Davies CB, Bekkali S, Byrne LK and Enticott PG (2018) Intra- and Inter-Regional Priming of Ipsilateral Human Primary Motor Cortex With Continuous Theta Burst Stimulation Does Not Induce Consistent Neuroplastic Effects. Front. Hum. Neurosci. 12:123. doi: 10.3389/fnhum.2018.00123
Received: 22 September 2017; Accepted: 13 March 2018;
Published: 29 March 2018.
Edited by:
Alessio Avenanti, Università degli Studi di Bologna, ItalyReviewed by:
Mitchell Ryan Goldsworthy, University of Adelaide, AustraliaGiovanni Pellegrino, IRCCS Fondazione Ospedale San Camillo, Italy
Copyright © 2018 Do, Kirkovski, Davies, Bekkali, Byrne and Enticott. This is an open-access article distributed under the terms of the Creative Commons Attribution License (CC BY). The use, distribution or reproduction in other forums is permitted, provided the original author(s) and the copyright owner are credited and that the original publication in this journal is cited, in accordance with accepted academic practice. No use, distribution or reproduction is permitted which does not comply with these terms.
*Correspondence: Michael Do, bS5kb0BkZWFraW4uZWR1LmF1