- 1Department of Biomedical Engineering, University of Wisconsin-Madison, Madison, WI, United States
- 2Department of Radiology, School of Medicine and Public Health, University of Wisconsin-Madison, Madison, WI, United States
- 3Medical Scientist Training Program, School of Medicine and Public Health, University of Wisconsin—Madison, Madison, WI, United States
- 4Neuroscience Training Program, University of Wisconsin—Madison, Madison, WI, United States
- 5Department of Neurology, University of Wisconsin—Madison, Madison, WI, United States
- 6Department of Psychology and Department of Psychiatry, University of Wisconsin—Madison, Madison, WI, United States
Following a stroke, the resulting lesion creates contralateral motor impairment and an interhemispheric imbalance involving hyperexcitability of the contralesional hemisphere. Neuronal reorganization may occur on both the ipsilesional and contralesional hemispheres during recovery to regain motor functionality and therefore bilateral activation for the hemiparetic side is often observed. Although ipsilesional hemispheric reorganization is traditionally thought to be most important for successful recovery, definitive conclusions into the role and importance of the contralesional motor cortex remain under debate. Through examining recent research in functional neuroimaging investigating motor cortex changes post-stroke, as well as brain-computer interface (BCI) and transcranial magnetic stimulation (TMS) therapies, this review attempts to clarify the contributions of each hemisphere toward recovery. Several functional magnetic resonance imaging studies suggest that continuation of contralesional hemisphere hyperexcitability correlates with lesser recovery, however a subset of well-recovered patients demonstrate contralesional motor activity and show decreased functional capability when the contralesional hemisphere is inhibited. BCI therapy may beneficially activate either the contralesional or ipsilesional hemisphere, depending on the study design, for chronic stroke patients who are otherwise at a functional plateau. Repetitive TMS used to excite the ipsilesional motor cortex or inhibit the contralesional hemisphere has shown promise in enhancing stroke patients' recovery.
Introduction
Stroke remains a leading cause of long-term disability (Hoyer and Celnik, 2011), affecting nearly 800,000 people annually within the United States (Mozaffarian et al., 2016), and causing almost 80% of patients to have persistent motor impairment following traditional therapy (Mayo et al., 1999). Imaging assessment of neural injury, function, and stroke subtype appear to have significant effects on stroke recovery (Burke Quinlan et al., 2015). Specifically, the initial degree of motor impairment (Byblow et al., 2015) and degree of injury to the corticospinal tract (Burke Quinlan et al., 2015; Feng et al., 2015; Doughty et al., 2016) have shown to be strong predictors of recovery, and several chronic stroke patients demonstrate functional recovery plateaus following standard rehabilitation (Young et al., 2014a).
Initial stroke recovery correlates to resolution of necrotic tissue, edema, and inflammation (Furlan et al., 1996; Stinear and Byblow, 2014), while later recovery relates mainly to disinhibition of redundant neural circuits, recruitment of functionally homologous pathways, and the creation of neural connections to overtake the previous functions of the damaged neurons (Rossini et al., 2007; Murphy and Corbett, 2009; Ackerley et al., 2011). Such neuronal processes may occur on the ipsilesional and contralesional hemispheres and are not completely understood (Hoyer and Celnik, 2011; Buetefisch, 2015); therefore, the relationship between the two motor cortex (M1) hemispheres post-stroke remains a topic of great interest (Baron et al., 2004; Hummel et al., 2008).
The contralateral M1 normally inhibits the ipsilateral hemisphere during motor performance tasks (Ocklenburg et al., 2015). However, patients with recent stroke commonly demonstrate increased M1 excitability on the contralesional hemisphere (equivalent to the ipsilateral hemisphere for healthy patients) for movements with the affected side (Chollet et al., 1991; Weiller et al., 1992; Shimizu et al., 2002; Butefisch et al., 2003, 2008; Murase et al., 2004; Tang et al., 2015). Therefore, stroke patients display an interhemispheric imbalance where the ipsilesional M1 no longer inhibits the contralesional hemisphere and the contralesional side appears to inhibit the ipsilesional (Baron et al., 2004), possibly through the transcallosal fibers (Murase et al., 2004) (Figure 1). The magnitude of such an imbalance appears to positively correlate with the degree of motor impairment (Murase et al., 2004), and the interhemispheric imbalance in other functional networks may also contribute toward other cortical functional disruptions including neglect (Tomaiuolo et al., 2010) and aphasia (Griffis et al., 2016).
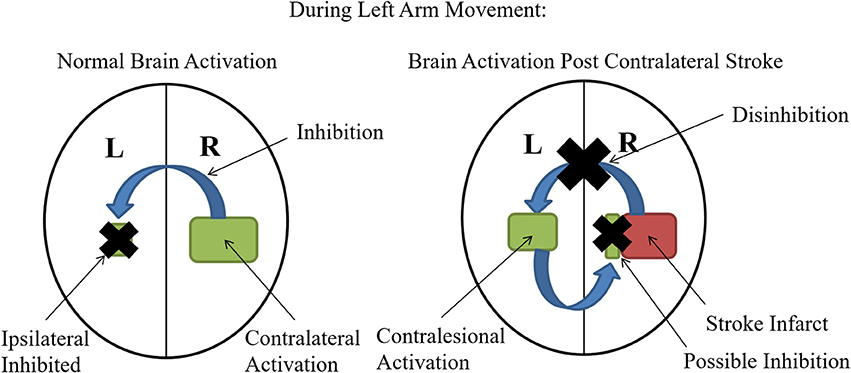
Figure 1. Interhemispheric Imbalance Post Stroke: Normally during unilateral motor performance tasks, activation of the contralateral hemisphere inhibits activation of the ipsilateral hemisphere (black X is showing inhibition of the ipsilateral side). However, when the contralateral M1 is impacted by stroke, this inhibition is lost (black X on the blue inhibitory arrow) and therefore the contralesional (analogous to ipsilateral for healthy controls) becomes more activated. Some studies suggest that this increased contralesional activation may also contribute to the interhemispheric imbalance by imposing increased inhibition on the ipsilesional (contralateral for healthy patients) hemisphere designated by the blue arrow and black X on the ipsilesional hemisphere (Murase et al., 2004).
Therapies that offer repeated action of the impaired arm, including mirror therapy (MT) (Rossiter et al., 2015), virtual-reality therapy (VRT) (Laver et al., 2015), constraint-induced movement therapy (CIMT) (Kwakkel et al., 2015), and brain-computer interface (BCI) therapy may reverse progressive down-regulation of sensorimotor activity (Lindberg et al., 2007) and induce neural plasticity (Johnson et al., 2004). However, most current rehabilitation methods that can target a specific M1 hemisphere, such as, transcranial magnetic stimulation (TMS), focus on ipsilesional hemispheric activation to facilitate motor recovery (Sanes et al., 1990; Hoyer and Celnik, 2011; Buetefisch, 2015). Although several studies demonstrate poorer motor recovery with increased contralesional hemispheric recruitment (Loubinoux et al., 2003; Fridman et al., 2004; Ward and Cohen, 2004; Calautti et al., 2007), some evidence suggests that contralesional recruitment may play an integral role in post-stroke motor recovery. According to Hoyer et al., the importance and role of contralesional M1 neural activity is probably determined by variables including time since stroke, degree of damage to the motor system, and complexity of the motor task (Hoyer and Celnik, 2011).
Brain-computer interfaces are a newer modality that use reward-based neuromodulatory training to induce use-dependent plasticity and facilitate functional recovery for stroke rehabilitation (Li et al., 2014; Young et al., 2014b; Soekadar et al., 2015; Mrachacz-Kersting et al., 2016). Unlike MT, VRT, CIMT, and TMS, BCI therapy uses signal detection of neural activity through an electroencephalogram (EEG) to provide specific and immediate visual feedback to the patient depending on their performance (Buch et al., 2008; Young et al., 2014a). This mini review attempts to broaden the scientific community's understanding of the roles of the M1 hemispheres in stroke rehabilitation by: (1) Analyzing current research on the role of the M1 hemispheres for spontaneous stroke recovery, and (2) Examining results from a therapy that relies upon specific M1 neuronal signals for feedback (BCI) as well as therapy that targets specific M1 hemispheres (TMS).
The Role of the Contralesional and Ipsilesional Hemispheres for Spontaneous Stroke Recovery
Most stroke patient brain activation studies find increased bilateral activation, related to elevated contralesional M1 activation, correlates to poorer motor recovery (Loubinoux et al., 2003; Baron et al., 2004). For example, increases in ipsilesional M1 activation, indicative of lesser bilateral activation, have significantly predicted larger treatment-induced behavioral gains for patients with lacunar infarcts (Burke Quinlan et al., 2015). Meanwhile, Ward et al.'s (2003a) cross-sectional study demonstrates a shift from bilateral activation to unilateral ipsilesional M1 activation in the more well-recovered patients during movement of the affected hand (Ward et al., 2003a) with other cross-sectional studies showing similar results (Chollet et al., 1991; Ward et al., 2003b). Longitudinal studies have also shown this trend with increased recovery correlated to increased unilateral development (Ward et al., 2003b). A limitation of many of these studies was significant mirror movements of the unaffected hand (Nelles et al., 1999, 2001; Carey et al., 2002; Feydy et al., 2002; Small et al., 2002). Such movements, indicative of poor motor outcome (Nelles et al., 1998; Kim et al., 2003), could have contributed to greater contralesional M1 activity (Buetefisch, 2015). Rodent stroke models that display decreased motor recovery when the non-affected limb is trained further suggests that increased contralesional M1 activation hinders motor recovery (Allred et al., 2005, 2010; Allred and Jones, 2008). In a recent systematic meta-analysis of studies in human stroke patients, Tang et al. demonstrated increased interhemispheric balance activation in patients within the sensorimotor and premotor cortices in well-recovered stroke patients (Tang et al., 2015). To facilitate optimal motor recovery, one might attempt to re-establish a normalized hemispheric balance between the two sides of the motor cortex given the evidence that activation for most well-recovered stroke patients shifts from a bilateral to an unilateral pattern (Hoyer and Celnik, 2011).
Increased ipsilateral M1 activation has been shown for tasks with higher accuracy or complexity demands in healthy subjects (Winstein et al., 1997; Hummel et al., 2003; Seidler et al., 2004; Verstynen et al., 2005; Buetefisch et al., 2014). Additionally, well-recovered stroke patients with chronic striatocapsular motor strokes (≥2 months post-stroke) show notable contralesional motor activity (Weiller et al., 1992), and Schaechter and Perdue demonstrated that increased activation of the contralesional cortical network in chronic stroke (≥6 months post-stroke) patients is associated with good motor recovery (Schaechter and Perdue, 2008). These findings suggest that uncrossed corticospinal tracts, which include roughly 10% of the corticospinal neuronal fibers, play a role in motor movement and possibly in recovery (Brus-Ramer et al., 2009). Touvykine et al. suggests that the contralesional premotor cortex may play a greater role in stroke recovery with larger lesions where damage on the ipsilesional side is more severe and where less ipsilesional neuronal recovery can develop (Touvykine et al., 2016). This indicates that activation of the contralesional hemisphere provides recruitment of additional neural resources due to the increased demands of the damaged ipsilesional motor system (Riecker et al., 2010). While some research suggests that increased activation of the contralesional M1 may detract from motor recovery (Calautti and Baron, 2003; Ward et al., 2003b), recent evidence suggests continued supportive functions of the contralesional hemisphere in a subset of chronic stroke patients (Butefisch et al., 2005; Lotze et al., 2006; Riecker et al., 2010). As most motor-impaired stroke patients initially demonstrate increased contralesional M1 activation (Murase et al., 2004), it is possible this activation offers increased motor function benefits (Lotze et al., 2006) within the subacute stage, but may interfere with continued recovery for some patients (Mansur et al., 2005; Fregni et al., 2006). Regardless, the contralesional M1 appears to support the function of the impaired limb in a subset of chronic stroke patients (Lotze et al., 2006), depending on factors including lesion location and size, but it may interfere in complete recovery of the limb in other subsets (Mansur et al., 2005; Fregni et al., 2006).
TMS can index interhemispheric interactions in patients >14 months post stroke (Borich et al., 2016) and has been used to help characterize the roles of the contralesional and ipsilesional hemispheres in spontaneous stroke recovery. Multiple studies utilizing TMS suggest that continuation of the increased activation of contralesional M1 associates with lesser clinical outcome (Turton et al., 1996; Netz et al., 1997; Feydy et al., 2002; Johansen-Berg et al., 2002). For instance, for 12 individuals with major arm paresis post-stroke, inhibitory TMS of the contralesional M1 significantly increased movement time in a paretic arm reaching task suggesting that patients with severe arm impairment rely heavily on the contralesional hemisphere (Mohapatra et al., 2016). Stinear et al. demonstrates that ipsilesional corticomotor excitability increased with time during recovery of 46 patients (44 with subcortical damage, and only two with motor cortex damage) during the first 6-months post-stroke (Stinear et al., 2015). However, interhemispheric inhibition of these patients was shown to be stable and symmetrical over time, with no decrease in contralesional corticomotor excitability (Stinear et al., 2015).
Other TMS studies suggest that contralesional hemispheric recruitment is a factor of well-recovered stroke patients. For instance, Lotze et al. demonstrated that rTMS applied to the contralesional hemisphere in well-recovered patients induced timing and accuracy deficits during production of a complex sequential motor task (Lotze et al., 2006). Furthermore, although TMS of only the ipsilesional hemisphere of well-recovered stroke patients, and not the contralesional, induced motor-evoked potentials in Nair et al., TMS did reveal greater transcallosal inhibition from the contralesional to the ipsilesional hemisphere than in the reverse direction (Nair et al., 2007). In Julkunen et al., TMS in conjunction with diffusion weighted imaging revealed that inter-hemispheric asymmetry in the M1 persists even years after stroke for well-recovered patients where clinical symptoms have normalized (Julkunen et al., 2016). These findings, along with a number of noninvasive brain stimulation studies that report null effects, suggest that the aim to strictly normalize the interhemispheric balance may be over-simplistic (Di Pino et al., 2014). Instead, other more complex models, such as, a bimodal balance-recovery model that links interhemispheric balancing and functional recovery to the amount of structural reserve spared by the lesion, may prove more accurate at determining optimal therapy for stroke patients (Di Pino et al., 2014).
The Role of the Contralesional and Ipsilesional Hemispheres with Newer Modality Therapy (BCI & TMS)
Some BCI paradigms have demonstrated motor improvements associated with recovery of the contralesional hemisphere. The BCI design in Song et al. and Young et al. demonstrated that increased contralesional hemispheric activation correlated to better performance on the Nine-Hole Peg Test (9-HPT), Action Research Arm Test (ARAT), and Stroke Impact Scale (SIS) (Song et al., 2014; Young et al., 2014a). Additionally, contralesional corticospinal tract (CST) fractional anisotropy (FA) correlated positively with improvements in the 9-HPT and increases in asymmetric FA correlated with the ARAT (Young et al., 2016). Similarly, Bundy et al.'s BCI, which focused on contralesional motor activation, found significant increases in the ARAT, grasp strength, Motricity Index, and the Canadian Occupational Performance Measure (Bundy et al., 2017). Young's, Song's, and Bundy's BCI paradigms suggested that axonal remodeling within, and increased motor fiber integrity of, the contralesional hemisphere correlates with individual motor gains for patients with upper-limb impairment (Song et al., 2014; Young et al., 2014a; Bundy et al., 2017).
In contrast, other BCI paradigms display motor improvements correlated to recovery of the ipsilesional hemisphere. Ramos-Murguialday et al.'s BCI [denoted as a brain-machine-interface (BMI)], saw significant motor gains associated with increased ipsilesional M1 recruitment with their experimental group as compared to control (Ramos-Murguialday et al., 2013). Additionally, only the experimental group demonstrated significantly improved modified upper limb Fugel-Meyer Motor Assessment (FMA) scores. Similarly, in Pichiorri et al., BCI training was associated both with increased ipsilesional hemisphere activation in response to motor imagery of the paralyzed limb and increased functional outcome in the FMA (Pichiorri et al., 2015).
Reasons for the differences found in the BCI studies probably relate to the BCI/BMI designs and the patient exclusion criteria. For instance, Young and Song recruited patients with a greater range of severity of motor impairments than Ramos (Ramos-Murguialday et al., 2013; Young et al., 2016). Additionally, Ramos' BMI paired orthoses movements with desynchronization of ipsilesional hemisphere brain oscillations (Ramos-Murguialday et al., 2013), Young and Song rewarded μ (8–12 Hz) and β (18–26 Hz) rhythms with functional electrical stimulation (FES), tongue display unit stimulation, and visual ball movement (Song et al., 2014; Young et al., 2014a), and Bundy used a moving hand exoskeleton that responded to contralesional motor signals (Bundy et al., 2017).
Overall, multiple BCI studies have found improved motor function for the severely impaired stroke patient correlated with contralesional M1 recruitment (Song et al., 2014; Young et al., 2014a; Bundy et al., 2017) as well as ipsilesional M1 recruitment (Ramos-Murguialday et al., 2013; Pichiorri et al., 2015). Specific BCI designs, as well as patient recruitment qualifications probably determine which hemisphere will activate more to begin motor function compensation development. Wide variation in the behavioral assessments used for outcome evaluation of motor function after stroke may also contribute to the heterogeneity in results making direct comparisons between study results challenging (Broetz et al., 2014). However, with the meta-analysis by Lohse et al. demonstrating that the trend of increased therapy correlating with increased improvement is not altered by stroke chronicity (Lohse et al., 2014), BCI appears to be a promising therapy for stroke patients who have reached a functional plateau with traditional therapy (Young et al., 2015).
TMS therapy can inhibit or excite targeted brain regions, and applications of this technique may encourage stroke rehabilitation (Hallett, 2000; Fregni et al., 2006) without deteriorating motor performance in the non-affected hand (Fregni et al., 2006; Vines et al., 2008; Hoyer and Celnik, 2011). One therapeutic utilization of TMS includes inhibitory, low-frequency, rTMS trains onto the contralesional motor region (Kobayashi et al., 2004). Numerous studies have already found improved task performance on the affected side following contralesional M1 inhibition via rTMS applied at a low-frequency (Demirtas-Tatlidede et al., 2015; Matsuura et al., 2015; Ludemann-Podubecka et al., 2016). Kobayashi et al. demonstrated that for healthy subjects, inhibitory 1-Hz rTMS over the non-performing M1 shows improvement of motor task performance of the ipsilateral hand, possibly by enhancing cortical excitability of the ipsilesional M1 through decreased interhemispheric inhibition from the stimulated (Kobayashi et al., 2004; Grefkes et al., 2010). Additionally, regional cerebral blood flow in the left M1 during right hand movement has been seen to increase during 1-Hz inhibitory rTMS of the right M1 (Conchou et al., 2009). For a study with eight mild-to-moderate stroke patients (<1 year post stroke), there was modest improvement in their motor function following an inhibitory rTMS applied to the contralesional hemisphere (Mansur et al., 2005). In a 2015 study, stroke patients with mild to moderate upper limb motor impairment were either subjected to 1-Hz contralesional M1 inhibitory rTMS or sham rTMS. Results demonstrated that a 1-Hz rTMS 15-day treatment plan showed significant motor dexterity improvement in dominant hemisphere strokes, but not those with strokes in the non-dominant hemispheres (Ludemann-Podubecka et al., 2015). In contrast Rose et al. found no significant improvement in restoration of upper extremity functional use for post 6-month stroke patients with a four-times-a-week program of inhibitory rTMS for 4 weeks (Rose et al., 2014). This study suggests that increased chronicity of stroke causes impairment of beneficial effects of rTMS in motor recovery (Rose et al., 2014).
Another method to regain interhemispheric balance and improve recovery is to excite the ipsilesional side with TMS (Talelli et al., 2007; Ameli et al., 2009). Kim et al. found that high-frequency rTMS to the motor cortex ipsilesionally gave improved finger sequence performance in the contralateral hand (Kim et al., 2004). Additionally, Khedr found that acute stroke patients with excitatory 3-Hz rTMS over the ipsilesional M1 for 10 days showed improved motor function (Khedr et al., 2005). Du et al. demonstrated that 3-Hz ipsilesional M1 rTMS showed greater motor improvements sustained for at least 3 months post treatment as compared to a control group with sham rTMS (Du et al., 2016). Brodie et al. confirmed that 5-Hz rTMS ipsilesionally enhances motor learning for chronic stroke (>6 months post) patients as compared to a sham control group over a serial tracking task (Brodie et al., 2014). Specifically the experimental group demonstrated significantly greater improvements in peak velocity, cumulative distance, and cutaneous somatosensation. The study concludes that when paired with motor practice, as both groups also went under tracking task training, 5-Hz excitatory rTMS over the ipsilateral M1 enhances motor learning in chronic stroke patients although no improvement in general upper extremity function was observed (Brodie et al., 2014).
A few studies have compared inhibiting the contralesional hemisphere to exciting the ipsilesional side through rTMS although the results remain inconclusive. Khedr et al. (2009) compared 1-Hz rTMS inhibition to 3-Hz rTMS ipsilesional M1 stimulation on simple motor tasks including keyboard tapping and a nine-hole pegboard task. This study found that the contralesional hemisphere 1-Hz inhibitory pulse sequence showed more motor improvement (Khedr et al., 2009). Similarly, another study compared 1 Hz inhibition contralesionally to 10-Hz excitatory ipsilesionally and a bilateral combination of both with the conclusion that only contralesional rTMS and bilateral showed significant improvement in motor training lasting 1 week (Takeuchi et al., 2009). Despite these findings, Emara et al. compared 1-Hz contralesional stimulation to 5-Hz ipsilesional hemisphere stimulation over 10 days and found that both group had significant motor function and disability scale improvement which lasted up to 12 weeks (Emara et al., 2010). Kim et al. also did not measure significant differences in motor function of the upper extremity between 1-Hz inhibitory and 20-Hz excitatory treatment for patients who suffered from their first subacute stroke within 4 weeks of the study (Kim et al., 2014). Finally, Mcdonnell and Stinear's meta-analysis found no clear evidence of interhemispheric inhibition or hyper-excitability of the unaffected hemisphere suggesting that exciting the ipsilesional M1 with TMS may be more beneficial than inhibiting the contralesional M1 (McDonnell and Stinear, 2017).
Conclusion
Major factors influencing unilateral recovery post-stroke appear to include chronicity, as well as lesion size and location. Increases in unilateral ipsilesional M1 activation for movement on the affected side appears to positively correlate with chronicity, especially for well-recovered patients (Ward et al., 2003b). However, increased contralesional M1 recruitment has been demonstrated for well-recovered patients with larger lesions (Touvykine et al., 2016). Overall, well-recovered patients display increased unilateral activation of the ipsilesional M1 with motor tasks as compared to ill-recovered patients, and animal models have suggested that motor learning of the non-affected side during recovery could limit rehabilitation of the ipsilesional hemisphere (Allred et al., 2005, 2010; Allred and Jones, 2008; Jones et al., 2013). However, some well-recovered patients display notable contralesional motor activity and the contralesional hemisphere appears to play a key role for at least a subset of stroke patients.
BCI training demonstrates potential in assisting with motor recovery for stroke sufferers who have reached a functional plateau following traditional rehabilitation. Evidence suggests that this therapy, even for chronic stroke sufferers, may induce neuromodulatory changes that enhance motor function. Both BCI designs that demonstrate development of the contralesional M1, as well as designs that increase ipsilesional M1 activity, appear to enhance motor recovery. It is currently unclear if either type of BCI design is superior to the other for all, or certain subsets of, patients.
Meanwhile, TMS is an effective and safe tool for research into this field and offers promising potential to be utilized for rehabilitation. TMS studies confirm that increased contralesional M1 activity as compared to the ipsilesional M1 is associated with lesser clinical outcome. Some TMS research suggests that for some well-recovered stroke patients contralesional corticomotor excitability does not decrease, but rather only the ipsilesional corticomotor excitability increases with recovery (Stinear et al., 2015) and often the interhemispheric asymmetry within M1 persists even years after stroke (Julkunen et al., 2016).
Both inhibition of the contralesional side and excitation of the ipsilesional side appear effective for motor recovery post-stroke. A few studies suggest that contralesional inhibition may be slightly more effective than ipsilesional M1 excitation although this is not seen throughout the literature. Initial degree of motor impairment and chronicity (Rose et al., 2014), as well as age (Kim et al., 2016), have been suggested to negatively correlate with the ability for rTMS to enhance the recovery process.
Current evidence favors ipsilesional M1 excitation and development to be the most important for stroke recovery over contralesional hemispheric recruitment. However, the contralesional side does appear to play a significant role for at least a subset of stroke patients and some research suggests that certain BCI paradigms targeting the contralesional hemisphere may be beneficial for motor recovery. It is important to note the numerous confounding variables that require more research to completely understand their effects including lesion location and size, and chronicity.
Author Contributions
KD wrote the manuscript, VN and VP reviewed and edited it, and provided key guidance.
Conflict of Interest Statement
VP has a pending US patent on the closed-loop neurofeedback used for BCI-facilitated intervention, application number 12/715090. The patent was filed jointly by VP and J. Williams.
The other authors declare that the research was conducted in the absence of any commercial or financial relationships that could be construed as a potential conflict of interest.
Acknowledgments
This work was supported by NIH grants RC1MH090912-01, T32GM008692, UL1TR000427, K23NS086852, T32EB011434, R01EB000856-06, and R01EB009103-01 and by the DARPA RCI Program (MTO) N66001-12-C-4025 and HIST Program (MTO) N66001-11-1-4013. Additional funding was also provided through a Coulter Translational Research Award, an American Heart Association Postdoctoral Fellow Research Award, the American Heart Association Grant 1T32EB011434-01A1, the Foundation of ASNR, UW Milwaukee-Madison Intercampus Grants, the UW Graduate School, and by Shapiro Foundation Grants.
References
Ackerley, S. J., Stinear, C. M., and Byblow, W. D (2011). Promoting use-dependent plasticity with externally-paced training. Clin. Neurophysiol. 122, 2462–2468. doi: 10.1016/j.clinph.2011.05.011
Allred, R. P., and Jones, T. A. (2008). Maladaptive effects of learning with the less-affected forelimb after focal cortical infarcts in rats. Exp. Neurol. 210, 172–181. doi: 10.1016/j.expneurol.2007.10.010
Allred, R. P., Cappellini, C. H., and Jones, T. A. (2010). The “good” limb makes the “bad” limb worse: experience-dependent interhemispheric disruption of functional outcome after cortical infarcts in rats. Behav. Neurosci. 124, 124–132. doi: 10.1037/a0018457
Allred, R. P., Maldonado, M. A., Hsu, J. E., and Jones, T. A. (2005). Training the “less-affected” forelimb after unilateral cortical infarcts interferes with functional recovery of the impaired forelimb in rats. Restor. Neurol. Neurosci. 23, 297–302.
Ameli, M., Grefkes, C., Kemper, F., Riegg, F. P., Rehme, A. K., Karbe, H., et al. (2009). Differential effects of high-frequency repetitive transcranial magnetic stimulation over ipsilesional primary motor cortex in cortical and subcortical middle cerebral artery stroke. Ann. Neurol. 66, 298–309. doi: 10.1002/ana.21725
Baron, J. C., Cohen, L. G., Cramer, S. C., Dobkin, B. H., Johansen-Berg, H., Loubinoux, I., et al. (2004). Neuroimaging in stroke recovery: a position paper from the First International Workshop on Neuroimaging and stroke recovery. Cerebrovasc. Dis. 18, 260–227. doi: 10.1159/000080293
Borich, M. R., Wheaton, L. A., Brodie, S. M., Lakhani, B., and Boyd, L. A. (2016). Evaluating interhemispheric cortical responses to transcranial magnetic stimulation in chronic stroke: a TMS-EEG investigation. Neurosci. Lett. 618, 25–30. doi: 10.1016/j.neulet.2016.02.047
Brodie, S. M., Meehan, S., Borich, M. R., and Boyd, L. A. (2014). 5 Hz repetitive transcranial magnetic stimulation over the ipsilesional sensory cortex enhances motor learning after stroke. Front. Hum. Neurosci. 8:143. doi: 10.3389/fnhum.2014.00143
Broetz, D., Del Grosso, N. A., Rea, M., Ramos-Murguialday, A., Soekadar, S. R., and Birbaumer, N. (2014). A new hand assessment instrument for severely affected stroke patients. NeuroRehabil. 34, 409–427. doi: 10.3233/NRE-141063
Brus-Ramer, M., Carmel, J. B., and Martin, J. H. (2009). Motor cortex bilateral motor representation depends on subcortical and interhemispheric interactions. J. Neurosci. 29, 6196–6206. doi: 10.1523/JNEUROSCI.5852-08.2009
Buch, E., Weber, C., Cohen, L. G., Braun, C., Dimyan, M. A., Ard, T., et al. (2008). Think to move: a neuromagnetic brain-computer interface (BCI) system for chronic stroke. Stroke 39, 910–997. doi: 10.1161/STROKEAHA.107.505313
Buetefisch, C. M. (2015). Role of the contralesional hemisphere in post-stroke recovery of upper extremity motor function. Front. Neurol. 6:214. doi: 10.3389/fneur.2015.00214
Buetefisch, C. M., Revill, K. P., Shuster, L., Hines, B., and Parsons, M. (2014). Motor demand-dependent activation of ipsilateral motor cortex. J. Neurophysiol. 112, 999–1009. doi: 10.1152/jn.00110.2014
Bundy, D. T., Souders, L., Baranyai, K., Leonard, L., Schalk, G., Coker, R., et al. (2017). Contralesional brain-computer interface control of a powered exoskeleton for motor recovery in chronic stroke survivors. Stroke 48, 1908–1915. doi: 10.1161/STROKEAHA.116.016304
Burke Quinlan, E., Dodakian, L., See, J., McKenzie, A., Le, V., Wojnowicz, M., et al. (2015). Neural function, injury, and stroke subtype predict treatment gains after stroke. Ann. Neurol, 77, 132–145. doi: 10.1002/ana.24309
Butefisch, C. M., Kleiser, R., Korber, B., Muller, K., Wittsack, H. J., Homberg, V., et al. (2005). Recruitment of contralesional motor cortex in stroke patients with recovery of hand function. Neurology 64, 1067–1069. doi: 10.1212/01.WNL.0000154603.48446.36
Butefisch, C. M., Netz, J., Wessling, M., Seitz, R. J., and Homberg, V. (2003). Remote changes in cortical excitability after stroke. Brain 126, 470–481. doi: 10.1093/brain/awg044
Butefisch, C. M., Wessling, M., Netz, J., Seitz, R. J., and Homberg, V. (2008). Relationship between interhemispheric inhibition and motor cortex excitability in subacute stroke patients. Neurorehabil. Neural Repair. 22, 4–21. doi: 10.1177/1545968307301769
Byblow, W. D., Stinear, C. M., Barber, P. A., Petoe, M. A., and Ackerley, S. J. (2015). Proportional recovery after stroke depends on corticomotor integrity. Ann. Neurol. 78, 848–859. doi: 10.1002/ana.24472
Calautti, C., and Baron, J. C. (2003). Functional neuroimaging studies of motor recovery after stroke in adults: a review. Stroke 34, 1553–1566. doi: 10.1161/01.STR.0000071761.36075.A6
Calautti, C., Naccarato, M., Jones, P. S., Sharma, N., Day, D. D., Carpenter, A. T., et al. (2007). The relationship between motor deficit and hemisphere activation balance after stroke: A 3T fMRI study. Neuroimage 34, 322-331. doi: 10.1016/j.neuroimage.2006.08.026
Carey, J. R., Kimberley, T. J., Lewis, S. M., Auerbach, E. J., Dorsey, L., Rundquist, P., et al. (2002). Analysis of fMRI and finger tracking training in subjects with chronic stroke. Brain 125, 773–788. doi: 10.1093/brain/awf091
Chollet, F., DiPiero, V., Wise, R. J., Brooks, D. J., Dolan, R. J., and Frackowiak, R. S. (1991). The functional anatomy of motor recovery after stroke in humans: a study with positron emission tomography. Ann. Neurol. 29, 63–71. doi: 10.1002/ana.410290112
Conchou, F., Loubinoux, I., Castel-Lacanal, E., Le Tinnier, A., Gerdelat-Mas, A., Faure-Marie, N., et al. (2009). Neural substrates of low-frequency repetitive transcranial magnetic stimulation during movement in healthy subjects and acute stroke patients. A PET study. Hum. Brain Mapp. 30, 2542–2557 doi: 10.1002/hbm.20690
Demirtas-Tatlidede, A., Alonso-Alonso, M., Shetty, R. P., Ronen, I., Pascual-Leone, A., and Fregni, F. (2015). Long-term effects of contralesional rTMS in severe stroke: safety, cortical excitability, and relationship with transcallosal motor fibers. Neurorehabilitation 36, 51–59. doi: 10.3233/NRE-141191
Di Pino, G., Pellegrino, G., Assenza, G., Capone, F., Ferreri, F., Formica, D., et al. (2014). Modulation of brain plasticity in stroke: a novel model for neurorehabilitation. Nat. Rev. Neurol. 10, 597–608. doi: 10.1038/nrneurol.2014.162
Doughty, C., Wang, J., Feng, W., Hackney, D., Pani, E., and Schlaug, G. (2016). Detection and predictive value of fractional anisotropy changes of the corticospinal tract in the acute phase of a stroke. Stroke 47, 1520–1526. doi: 10.1161/STROKEAHA.115.012088
Du, J., Tian, L., Liu, W., Hu, J., Xu, G., Ma, M., et al. (2016). Effects of repetitive transcranial magnetic stimulation on motor recovery and motor cortex excitability in patients with stroke: a randomized controlled trial. Eur. J. Neurol. 23, 1666–1672. doi: 10.1111/ene.13105
Emara, T. H., Moustafa, R. R., Elnahas, N. M., Elganzoury, A. M., Abdo, T. A., Mohamed, S. A., et al. (2010). Repetitive transcranial magnetic stimulation at 1Hz and 5Hz produces sustained improvement in motor function and disability after ischaemic stroke. Eur. J. Neurol. 17, 1203–1209. doi: 10.1111/j.1468-1331.2010.03000.x
Feng, W., Wang, J., Chhatbar, P. Y., Doughty, C., Landsittel, D., Lioutas, V. A., et al. (2015). Corticospinal tract lesion load: an imaging biomarker for stroke motor outcomes. Ann. Neurol. 78, 860–870. doi: 10.1002/ana.24510
Feydy, A., Carlier, R., Roby-Brami, A., Bussel, B., Cazalis, F., Pierot, L., et al. (2002). Longitudinal study of motor recovery after stroke: recruitment and focusing of brain activation. Stroke 33, 1610–1617. doi: 10.1161/01.STR.0000017100.68294.52
Fregni, F., Boggio, P. S., Valle, A. C., Rocha, R. R., Duarte, J., Ferreira, M. J., et al. (2006). A sham-controlled trial of a 5-day course of repetitive transcranial magnetic stimulation of the unaffected hemisphere in stroke patients. Stroke 37, 2115–2122. doi: 10.1161/01.STR.0000231390.58967.6b
Fridman, E. A., Hanakawa, T., Chung, M., Hummel, F., Leiguarda, R. C., and Cohen, L. G. (2004). Reorganization of the human ipsilesional premotor cortex after stroke. Brain 127, 747–758. doi: 10.1093/brain/awh082
Furlan, M., Marchal, G., Viader, F., Derlon, J. M., and Baron, J. C. (1996). Spontaneous neurological recovery after stroke and the fate of the ischemic penumbra. Ann. Neurol. 40, 216–226. doi: 10.1002/ana.410400213
Grefkes, C., Nowak, D. A., Wang, L. E., Dafotakis, M., Eickhoff, S. B., and Fink, G. R. (2010). Modulating cortical connectivity in stroke patients by rTMS assessed with fMRI and dynamic causal modeling Neuroimage 50, 233–242. doi: 10.1016/j.neuroimage.2009.12.029
Griffis, J. C., Nenert, R., Allendorfer, J. B., and Szaflarski, J. P. (2016). Interhemispheric plasticity following intermittent theta burst stimulation in chronic poststroke aphasia. Neural Plasticity, 2016:4796906. doi: 10.1155/2016/4796906
Hallett, M. (2000). Transcranial magnetic stimulation and the human brain. Nature 406, 147–150. doi: 10.1038/35018000
Hoyer, E. H., and Celnik, P. A. (2011). Understanding and enhancing motor recovery after stroke using transcranial magnetic stimulation. Restor. Neurol. Neurosci. 29, 395–409. doi: 10.3233/RNN-2011-061
Hummel, F. C., Celnik, P., Pascual-Leone, A., Fregni, F., Byblow, W. D., Buetefisch, C. M., et al. (2008). Controversy: noninvasive and invasive cortical stimulation show efficacy in treating stroke patients. Brain Stimul. 1, 370–382. doi: 10.1016/j.brs.2008.09.003
Hummel, F., Kirsammer, R., and Gerloff, C. (2003). Ipsilateral cortical activation during finger sequences of increasing complexity: representation of movement difficulty or memory load? Clin. Neurophysiol. 114, 605–613. doi: 10.1016/S1388-2457(02)00417-0
Johansen-Berg, H., Rushworth, M. F., Bogdanovic, M. D., Kischka, U., Wimalaratna, S., and Matthews, P. M. (2002). The role of ipsilateral premotor cortex in hand movement after stroke. Proc. Natl. Acad. Sci. U.S.A. 99, 14518–14523. doi: 10.1073/pnas.222536799
Johnson, M. J., Trickey, M., Brauer, E., and Feng, X. (2004). TheraDrive: a new stroke therapy concept for home-based, computer-assisted motivating rehabilitation. Conf. Proc. IEEE Eng. Med. Biol. Soc. 7, 4844–4487. doi: 10.1109/IEMBS.2004.1404340
Jones, T. A., Allred, R. P., Jefferson, S. C., Kerr, A. L., Woodie, D. A., Cheng, S. Y., et al. (2013). Motor system plasticity in stroke models: intrinsically use-dependent, unreliably useful. Stroke 44, S104–S106. doi: 10.1161/STROKEAHA.111.000037
Julkunen, P., Maatta, S., Saisanen, L., Kallioniemi, E., Kononen, M., Jakala, P., et al. (2016). Functional and structural cortical characteristics after restricted focal motor cortical infarction evaluated at chronic stage - Indications from a preliminary study. Clin. Neurophysiol. 127, 2775–2784. doi: 10.1016/j.clinph.2016.05.013
Khedr, E. M., Abdel-Fadeil, M. R., Farghali, A., and Qaid, M. (2009). Role of 1 and 3 Hz repetitive transcranial magnetic stimulation on motor function recovery after acute ischaemic stroke. Eur. J. Neurol. 16, 1323–1330. doi: 10.1111/j.1468-1331.2009.02746.x
Khedr, E. M., Ahmed, M. A., Fathy, N., and Rothwell, J. C. (2005). Therapeutic trial of repetitive transcranial magnetic stimulation after acute ischemic stroke. Neurology 65, 466–468. doi: 10.1212/01.wnl.0000173067.84247.36
Kim, C., Choi, H. E., Jung, H., Lee, B. J., Lee, K. H., and Lim, Y. J. (2014). Comparison of the Effects of 1 Hz and 20 Hz rTMS on motor recovery in subacute stroke patients. Ann. Rehabil. Med. 38, 585–591. doi: 10.5535/arm.2014.38.5.585
Kim, S. Y., Shin, S. B., Lee, S. J., Kim, T. U., and Hyun, J. K. (2016). Factors associated with upper extremity functional recovery following low-frequency repetitive transcranial magnetic stimulation in stroke patients. Ann. Rehabil. Med. 40, 373–382. doi: 10.5535/arm.2016.40.3.373
Kim, Y. H., Jang, S. H., Chang, Y., Byun, W. M., Son, S., and Ahn, S. H. (2003). Bilateral primary sensori-motor cortex activation of post-stroke mirror movements: an fMRI study. Neuroreport 14, 1329–1332. doi: 10.1097/00001756-200307180-00009
Kim, Y. H., Park, J. W., Ko, M. H., Jang, S. H., and Lee, P. K. (2004). Facilitative effect of high frequency subthreshold repetitive transcranial magnetic stimulation on complex sequential motor learning in humans. Neurosci. Lett. 367, 181–185. doi: 10.1016/j.neulet.2004.05.113
Kobayashi, M., Hutchinson, S., Theoret, H., Schlaug, G., and Pascual-Leone, A. (2004). Repetitive TMS of the motor cortex improves ipsilateral sequential simple finger movements, Neurology 62, 91–98. doi: 10.1212/WNL.62.1.91
Kwakkel, G., Veerbeek, J. M., van Wegen, E. E., and Wolf, S. L. (2015). Constraint-induced movement therapy after stroke. Lancet Neurol. 14, 224–234. doi: 10.1016/S1474-4422(14)70160-7
Laver, K. E., George, S., Thomas, S., Deutsch, J. E., and Crotty, M. (2015). Virtual reality for stroke rehabilitation. Cochrane Database Syst. Rev. 2:Cd008349. doi: 10.1002/14651858.CD008349.pub3
Li, M., Liu, Y., Wu, Y., Liu, S., Jia, J., and Zhang, L. (2014). Neurophysiological substrates of stroke patients with motor imagery-based Brain-Computer Interface training. Int. J. Neurosci. 124, 403–415. doi: 10.3109/00207454.2013.850082
Lindberg, P. G., Schmitz, C., Engardt, M., Forssberg, H., and Borg, J. (2007). Use-dependent up- and down-regulation of sensorimotor brain circuits in stroke patients. Neurorehabil. Neural Repair. 21, 315–326. doi: 10.1177/1545968306296965
Lohse, K. R., Lang, C. E., and Boyd, L. A. (2014). Is more better? Using metadata to explore dose-response relationships in stroke rehabilitation. Stroke 45, 2053–2058. doi: 10.1161/STROKEAHA.114.004695
Lotze, M., Markert, J., Sauseng, P., Hoppe, J., Plewnia, C., and Gerloff, C. (2006). The role of multiple contralesional motor areas for complex hand movements after internal capsular lesion. J. Neurosci. 26, 6096–6102. doi: 10.1523/JNEUROSCI.4564-05.2006
Loubinoux, I., Carel, C., Pariente, J., Dechaumont, S., Albucher, J. F., Marque, P., et al. (2003). Correlation between cerebral reorganization and motor recovery after subcortical infarcts, Neuroimage 20, 2166–2180. doi: 10.1016/j.neuroimage.2003.08.017
Ludemann-Podubecka, J., Bosl, K., and Nowak, D. A. (2016). Inhibition of the contralesional dorsal premotor cortex improves motor function of the affected hand following stroke. Eur. J. Neurol. 23, 823–830. doi: 10.1111/ene.12949
Ludemann-Podubecka, J., Bosl, K., Theilig, S., Wiederer, R., and Nowak, D. A. (2015). The effectiveness of 1 Hz rTMS over the primary motor area of the unaffected hemisphere to improve hand function after stroke depends on hemispheric dominance. Brain Stimul. 8, 823–830. doi: 10.1016/j.brs.2015.02.004
Mansur, C. G., Fregni, F., Boggio, P. S., Riberto, M., Gallucci-Neto, J., Santos, C. M., et al. (2005). A sham stimulation-controlled trial of rTMS of the unaffected hemisphere in stroke patients, Neurology 64, 1802–1804.
Matsuura, A., Onoda, K., Oguro, H., and Yamaguchi, S. (2015). Magnetic stimulation and movement-related cortical activity for acute stroke with hemiparesis. Eur. J. Neurol. 22, 1526–1532. doi: 10.1111/ene.12776
Mayo, N. E., Wood-Dauphinee, S., Ahmed, S., Gordon, C., Higgins, J., McEwen, S., et al. (1999). Disablement following stroke. Disabil. Rehabil. 21, 258–268. doi: 10.1080/096382899297684
McDonnell, M. N., and Stinear, C. M. (2017). TMS measures of motor cortex function after stroke: a meta-analysis. Brain Stimul. 10, 721–734. doi: 10.1016/j.brs.2017.03.008
Mohapatra, S., Harrington, R., Chan, E., Dromerick, A. W., Breceda, E. Y., and Harris-Love, M. (2016). Role of contralesional hemisphere in paretic arm reaching in patients with severe arm paresis due to stroke: a preliminary report. Neurosci. Lett. 617, 52–58. doi: 10.1016/j.neulet.2016.02.004
Mozaffarian, D., Benjamin, E. J., Go, A. S., Arnett, D. K., Blaha, M. J., Cushman, M., et al. (2016). Heart disease and stroke statistics-2016 update: a report from the american heart association. Circulation 133, e38–360. doi: 10.1161/CIR.0000000000000350
Mrachacz-Kersting, N., Jiang, N., Stevenson, A. J., Niazi, I. K., Kostic, V., Pavlovic, A., et al. (2016). Efficient neuroplasticity induction in chronic stroke patients by an associative brain-computer interface. J. Neurophysiol. 115, 1410–1421. doi: 10.1152/jn.00918.2015
Murase, N., Duque, J., Mazzocchio, R., and Cohen, L. G. (2004). Influence of interhemispheric interactions on motor function in chronic stroke. Ann. Neurol. 55, 400–449. doi: 10.1002/ana.10848
Murphy, T. H., and Corbett, D. (2009). Plasticity during stroke recovery: from synapse to behaviour. Nat. Rev. Neurosci. 10, 861–872. doi: 10.1038/nrn2735
Nair, D. G., Hutchinson, S., Fregni, F., Alexander, M., Pascual-Leone, A., and Schlaug, G. (2007). Imaging correlates of motor recovery from cerebral infarction and their physiological significance in well-recovered patients. Neuroimage 34, 253–263. doi: 10.1016/j.neuroimage.2006.09.010
Nelles, G., Cramer, S. C., Schaechter, J. D., Kaplan, J. D., and Finklestein, S. P. (1998). Quantitative assessment of mirror movements after stroke. Stroke 29, 1182–1187. doi: 10.1161/01.STR.29.6.1182
Nelles, G., Jentzen, W., Jueptner, M., Muller, S., and Diener, H. C. (2001). Arm training induced brain plasticity in stroke studied with serial positron emission tomography. Neuroimage 13, 1146–1154. doi: 10.1006/nimg.2001.0757
Nelles, G., Spiekramann, G., Jueptner, M., Leonhardt, G., Muller, S., Gerhard, H., et al. (1999). Evolution of functional reorganization in hemiplegic stroke: a serial positron emission tomographic activation study. Ann. Neurol. 46, 901–999. doi: 10.1002/1531-8249(199912)46:6<901::AID-ANA13>3.0.CO;2-7
Netz, J., Lammers, T., and Homberg, V. (1997). Reorganization of motor output in the non-affected hemisphere after stroke Brain 120 (Pt. 9), 1579–1586. doi: 10.1093/brain/120.9.1579
Ocklenburg, S., Ball, A., Wolf, C. C., Genc, E., and Gunturkun, O. (2015). Functional cerebral lateralization and interhemispheric interaction in patients with callosal agenesis, Neuropsychology 29, 806–815. doi: 10.1037/neu0000193
Pichiorri, F., Morone, G., Petti, M., Toppi, J., Pisotta, I., Molinari, M., et al. (2015). Brain-computer interface boosts motor imagery practice during stroke recovery. Ann. Neurol. 77, 851–865. doi: 10.1002/ana.24390
Ramos-Murguialday, A., Broetz, D., Rea, M., Läer, L., Yilmaz, Ö., Brasil, F. L., et al. (2013). Brain-machine-interface in chronic stroke rehabilitation: a controlled study. Ann. Neurol. 74, 100–108. doi: 10.1002/ana.23879
Riecker, A., Groschel, K., Ackermann, H., Schnaudigel, S., Kassubek, J., and Kastrup, A. (2010). The role of the unaffected hemisphere in motor recovery after stroke. Hum Brain Mapp. 31, 1017–1029. doi: 10.1002/hbm.20914
Rose, D. K., Patten, C., McGuirk, T. E., Lu, X., and Triggs, W. J. (2014). Does inhibitory repetitive transcranial magnetic stimulation augment functional task practice to improve arm recovery in chronic stroke? Stroke Res. Treat. 2014:305236. doi: 10.1155/2014/305236
Rossini, P. M., Altamura, C., Ferreri, F., Melgari, J. M., Tecchio, F., Tombini, M., et al. (2007). Neuroimaging experimental studies on brain plasticity in recovery from stroke. Eura Medicophys. 43, 241–254.
Rossiter, H. E., Borrelli, M. R., Borchert, R. J., Bradbury, D., and Ward, N. S. (2015). Cortical mechanisms of mirror therapy after stroke. Neurorehabil. Neural Repair. 29, 444–452. doi: 10.1177/1545968314554622
Sanes, J. N., Suner, S., and Donoghue, J. P. (1990). Dynamic organization of primary motor cortex output to target muscles in adult rats. I. Long-term patterns of reorganization following motor or mixed peripheral nerve lesions. Exp. Brain Res. 79, 479–491. doi: 10.1007/BF00229318
Schaechter, J. D., and Perdue, K. L. (2008). Enhanced cortical activation in the contralesional hemisphere of chronic stroke patients in response to motor skill challenge. Cereb. Cortex 18, 638–647. doi: 10.1093/cercor/bhm096
Seidler, R. D., Noll, D. C., and Thiers, G. (2004). Feedforward and feedback processes in motor control. Neuroimage 22, 1775–1783. doi: 10.1016/j.neuroimage.2004.05.003
Shimizu, T., Hosaki, A., Hino, T., Sato, M., Komori, T., Hirai, S., et al. (2002). Motor cortical disinhibition in the unaffected hemisphere after unilateral cortical stroke. Brain 125, 1896–1907. doi: 10.1093/brain/awf183
Small, S. L., Hlustik, P., Noll, D. C., Genovese, C., and Solodkin, A. (2002). Cerebellar hemispheric activation ipsilateral to the paretic hand correlates with functional recovery after stroke. Brain 125, 1544–1557. doi: 10.1093/brain/awf148
Soekadar, S. R., Birbaumer, N., Slutzky, M. W., and Cohen, L. G. (2015). Brain-machine interfaces in neurorehabilitation of stroke. Neurobiol. Dis. 83, 172–179. doi: 10.1016/j.nbd.2014.11.025
Song, J., Young, B. M., Nigogosyan, Z., Walton, L. M., Nair, V. A., Grogan, S. W., et al. (2014). Characterizing relationships of DTI, fMRI, and motor recovery in stroke rehabilitation utilizing brain-computer interface technology. Front. Neuroeng. 7:31. doi: 10.3389/fneng.2014.00031
Stinear, C. M., and Byblow, W. D. (2014). Predicting and accelerating motor recovery after stroke. Curr. Opin. Neurol. 27, 624–630. doi: 10.1097/WCO.0000000000000153
Stinear, C. M., Petoe, M. A., and Byblow, W. D. (2015). Primary motor cortex excitability during recovery after stroke: implications for neuromodulation. Brain Stimul. 8, 1183–1190. doi: 10.1016/j.brs.2015.06.015
Takeuchi, N., Tada, T., Toshima, M., Matsuo, Y., and Ikoma, K. (2009). Repetitive transcranial magnetic stimulation over bilateral hemispheres enhances motor function and training effect of paretic hand in patients after stroke. J. Rehabil. Med. 41, 1049–1054. doi: 10.2340/16501977-0454
Talelli, P., Greenwood, R. J., and Rothwell, J. C. (2007). Exploring theta burst stimulation as an intervention to improve motor recovery in chronic stroke. Clin. Neurophysiol. 118, 333–342. doi: 10.1016/j.clinph.2006.10.014
Tang, Q., Li, G., Liu, T., Wang, A., Feng, S., Liao, X., et al. (2015). Modulation of interhemispheric activation balance in motor-related areas of stroke patients with motor recovery: systematic review and meta-analysis of fMRI studies. Neurosci. Biobehav. Rev. 57, 392–400. doi: 10.1016/j.neubiorev.2015.09.003
Tomaiuolo, F., Voci, L., Bresci, M., Cozza, S., Posteraro, F., Oliva, M., et al. (2010). Selective visual neglect in right brain damaged patients with splenial interhemispheric disconnection. Exp. Brain Res. 206, 209–217. doi: 10.1007/s00221-010-2230-6
Touvykine, B., Mansoori, B. K., Jean-Charles, L., Deffeyes, J., Quessy, S., and Dancause, N. (2016). The effect of lesion size on the organization of the ipsilesional and contralesional motor cortex. Neurorehabil. Neural Repair. 30, 280–292. doi: 10.1177/1545968315585356
Turton, A., Wroe, S., Trepte, N., Fraser, C., and Lemon, R. N. (1996). Contralateral and ipsilateral EMG responses to transcranial magnetic stimulation during recovery of arm and hand function after stroke. Electroencephalogr. Clin. Neurophysiol. 101, 316–328. doi: 10.1016/0924-980X(96)95560-5
Verstynen, T., Diedrichsen, J., Albert, N., Aparicio, P., and Ivry, R. B. (2005). Ipsilateral motor cortex activity during unimanual hand movements relates to task complexity. J. Neurophysiol. 93, 1209–1222. doi: 10.1152/jn.00720.2004
Vines, B. W., Nair, D., and Schlaug, G. (2008). Modulating activity in the motor cortex affects performance for the two hands differently depending upon which hemisphere is stimulated. Eur. J. Neurosci. 28, 1667–1673. doi: 10.1111/j.1460-9568.2008.06459.x
Ward, N. S., and Cohen, L. G. (2004). Mechanisms underlying recovery of motor function after stroke. Arch Neurol. 61, 1844–1848. doi: 10.1001/archneur.61.12.1844
Ward, N. S., Brown, M. M., Thompson, A. J., and Frackowiak, R. S. (2003a). Neural correlates of outcome after stroke: a cross-sectional fMRI study. Brain 126, 1430–1448. doi: 10.1093/brain/awg145
Ward, N. S., Brown, M. M., Thompson, A. J., and Frackowiak, R. S. (2003b). Neural correlates of motor recovery after stroke: a longitudinal fMRI study. Brain 126, 2476–2496. doi: 10.1093/brain/awg245
Weiller, C., Chollet, F., Friston, K. J., Wise, R. J., and Frackowiak, R. S. (1992). Functional reorganization of the brain in recovery from striatocapsular infarction in man. Ann. Neurol. 31, 463–472. doi: 10.1002/ana.410310502
Winstein, C. J., Grafton, S. T., and Pohl, P. S. (1997). Motor task difficulty and brain activity: investigation of goal-directed reciprocal aiming using positron emission tomography. J. Neurophysiol. 77, 1581–1594.
Young, B. M., Nigogosyan, Z., Walton, L. M., Remsik, A., Song, J., Nair, V. A., et al. (2015). Dose-response relationships using brain-computer interface technology impact stroke rehabilitation. Front. Hum. Neurosci. 9:361. doi: 10.3389/fnhum.2015.00361
Young, B. M., Nigogosyan, Z., Walton, L. M., Song, J., Nair, V. A., Grogan, S. W., et al. (2014a). Changes in functional brain organization and behavioral correlations after rehabilitative therapy using a brain-computer interface. Front Neuroeng. 7:26. doi: 10.3389/fneng.2014.00026
Young, B. M., Stamm, J. M., Song, J., Remsik, A. B., Nair, V. A., Tyler, M. E., et al. (2016). Brain-computer interface training after stroke affects patterns of brain-behavior relationships in corticospinal motor fibers. Front. Hum. Neurosci. 10:457. doi: 10.3389/fnhum.2016.00457
Keywords: ipsilesional, contralesional, stroke, motor recovery, brain-computer interface, transcranial magnetic stimulation
Citation: Dodd KC, Nair VA and Prabhakaran V (2017) Role of the Contralesional vs. Ipsilesional Hemisphere in Stroke Recovery. Front. Hum. Neurosci. 11:469. doi: 10.3389/fnhum.2017.00469
Received: 08 May 2017; Accepted: 07 September 2017;
Published: 21 September 2017.
Edited by:
Mikhail Lebedev, Duke University, United StatesReviewed by:
Toshiki Tazoe, Tokyo Metropolitan Institute of Medical Science, JapanPavel Lindberg, Centre for Psychiatry and Neuroscience (INSERM), France
Filippo Brighina, University of Palermo, Italy
Copyright © 2017 Dodd, Nair and Prabhakaran. This is an open-access article distributed under the terms of the Creative Commons Attribution License (CC BY). The use, distribution or reproduction in other forums is permitted, provided the original author(s) or licensor are credited and that the original publication in this journal is cited, in accordance with accepted academic practice. No use, distribution or reproduction is permitted which does not comply with these terms.
*Correspondence: Vivek Prabhakaran, vprabhakaran@uwhealth.org