- 1Neural Engineering Laboratory, Department of Biomedical Engineering, City College of New York, City University of New York, New York, NY, USA
- 2Biomedical Engineering Department, Amirkabir University of Technology, Tehran, Iran
- 3Neurology Service Hospital de Cínicas de Porto Alegre, Department of Internal Medicina, Universidade Federal do Rio Grande do Sul (UFRGS), Porto Alegre, Brazil
- 4Hospital Moinhos de Vento, Porto Alegre, Brazil
- 5Laboratory of Neuroscience (LIM27), Department and Institute of Psychiatry, University of São Paulo, São Paulo, Brazil
- 6Faculdade de Ciências Médicas da Santa Casa de São Paulo, School of Medical Sciences, São Paulo, Brazil
- 7Departamento de Medicina - Fortaleza, Universidade de Fortaleza, Centro de Ciências da Saúde, Ceará, Brazil
- 8Laboratory of Neuromodulation, Physical Medicine and Rehabilitation Department, Spaulding Rehabilitation Hospital, Harvard Medical School, Boston, MA, USA
Background: Transcranial direct current stimulation (tDCS) is investigated to modulate neuronal function including cognitive neuroscience and neuropsychiatric therapies. While cases of human stimulation with rudimentary batteries date back more than 200 years, clinical trials with current controlled stimulation were published intermittently since the 1960s. The modern era of tDCS only started after 1998.
Objectives: To review methods and outcomes of tDCS studies from old literature (between 1960 and 1998) with intention of providing new insight for ongoing tDCS trials and development of tDCS protocols especially for the purpose of treatment.
Methods: Articles were identified through a search in PubMed and through the reference list from its selected articles. We included only non-invasive human studies that provided controlled direct current and were written in English, French, Spanish or Portuguese before the year of 1998, the date in which modern stimulation paradigms were implemented.
Results: Fifteen articles met our criteria. The majority were small-randomized controlled clinical trials that enrolled a mean of approximately 26 subjects (Phase II studies). Most of the studies (around 83%) assessed the role of tDCS in the treatment of psychiatric conditions, in which the main outcomes were measured by means of behavioral scales and clinical observation, but the diagnostic precision and the quality of outcome monitoring, including adverse events, were deficient by modern standards. Compared to modern tDCS dose, the stimulation intensities used (0.1–1 mA) were lower, however as the electrodes were typically smaller (e.g., 1.26 cm2), the average electrode current density (0.2 mA/cm2) was approximately 4× higher. The number of sessions ranged from one to 120 (median 14). Notably, the stimulation session durations of several minutes to 11 h (median 4.5 h) could markedly exceed modern tDCS protocols. Twelve studies out of 15 showed positive results. Only mild side effects were reported, with headache and skin alterations the most common.
Conclusion: Most of the studies identified were for psychiatric indications, especially in patients with depression and/or schizophrenia and majority indicated some positive results. Variability in outcome is noted across trials and within trials across subjects, but overall results were reported as encouraging, and consistent with modern efforts, given some responders and mild side effects. The significant difference with modern dose, low current with smaller electrode size and interestingly much longer stimulation duration may worth considering.
Introduction
Transcranial direct current stimulation (tDCS) consists of applying a weak direct current on the scalp, a portion of which crosses the skull (Datta et al., 2009) and induces cortical changes (Fregni and Pascual-Leone, 2007; Nitsche et al., 2008). The investigation of the application of electricity over the brain dates back to at least 200 years, when Giovanni Aldini (Zaghi et al., 2010) recommended galvanism for patients with deafness, amaurosis and “insanity”, reporting good results with this technique especially when used in patients with “melancholia”. Aldini also used tDCS in patients with symptoms of personality disorders and supposedly reported complete rehabilitation following transcranial administration of electric current (Parent, 2004).
These earliest studies used rudimentary batteries and so were constant voltage, where the resulting current depends on a variable body resistance. Over the 20th century, direct voltage continued to be used but most testing involved pulsed stimulation, starting with basic devices where a mechanical circuit that intermittently connected and broke the circuit between the battery and the subject and evolving to modern current control circuits including Cranial Electrotherapy Stimulation and its variants (Guleyupoglu et al., 2013). Interest in direct current stimulation (or tDCS) resurged with the studies of Priori et al. (1998) and Nitsche and Paulus (2000) that demonstrated weak direct current could change cortical response to Transcranial Magnetic Stimulation, thereby indicating that tDCS could change cortical “excitability”. Testing for clinical and cognitive modification soon followed (Fregni et al., 2005, 2006). Developments and challenges in tDCS research, including applications in the treatment of neuro-psychiatrics disease since 1998 have been reviewed in detailed elsewhere (Brunoni et al., 2012).
This historical note aims to explore earlier data on human trial using current controlled stimulation (tDCS) before 1998 with the goal of informing ongoing understanding and development of tDCS protocols. As expected, we found variability in the quality of trial design, data collection and reporting in these earlier studies. Nonetheless, many clinical findings are broadly consistent with modern efforts, including some encouraging results but also variability across subjects. We also describe a significant difference in dose with lower current, smaller electrodes and much longer durations (up to 11 h) than used in modern tDCS.
Methods
Literature Search
For our searching methodology, we included articles that: (a) investigated the clinical effects of transcranial direct current stimulation; (b) were published before 1998; (c) human studies; (d) written in English, Spanish, Portuguese or French; (e) controlled current for stimulation. We also excluded articles if they were reviews or meta-analysis, as well as studies that involved invasive procedures or other methods of electrical stimulation.
To identify relevant studies, we searched PubMed using the keywords (brain polarization), (transcranial direct current stimulation) and (electric stimulation therapy) along with (brain). We also searched the reference list of all selected articles to identify other relevant articles that we might have missed during the primary PubMed search. Initially, AP and PS conducted the search but, if there were any unresolved issue, FF was consulted. Most of the articles were not available online; therefore they were retrieved at Francis A. Countway Library of Medicine (Harvard, Cambridge, MA, USA).
The data was collected using a semi-structured form for each study. The following variables were extracted: (a) title; (b) year of publication; (c) Journal; (d) number of participants in the study; (e) their pre-existing condition; (f) medications; (g) intensity of the applied current; (h) duration of each session; (i) number of sessions; (j) total duration of stimulation; (k) position of the electrodes; (l) electrode size; (m) the strategy of stimulation; (n) clinical effects; (o) side effects; (p) trial design; (q) conclusion; and (r) main outcome. Some of these data were shown in Table 1. Because we only found 15 articles fulfilling the inclusion criteria, and included articles had with incomplete and variable reporting details, it was not prudent to conduct quantitative analysis.
Terminology
For the purpose of this study we combine typical terminology used in modern tDCS with literature with conventions in classic literature. tDCS always requires a positive (anode) and negative (cathode) electrode on the body. The term “active” indicates the electrode which is considered by the investigator to exert behavioral effects, presumably by modulating cortex under the electrodes, while “return” electrode indicate the counter polarity electrode which is presumed to have no or less consequential effect. The anode electrode is presumed to generate an excitatory influence, while the cathode a local inhibitory influence. This concept pervades historical to modern tDCS design, though modern neurophysiology, imaging and computational modeling suggest that how and which brain regions are modulated by tDCS is much more complex. One electrode must always be on the head. In modern literature, an electrode below the head is “extra-cephalic” and typically placed on the forearm. In older literature, “scalp-positive” or “scalp-negative” is used to indicate the use of an extra-cephalic electrode, typically placed on the hand or foot with the anode or cathode, respectively, on the head. For example, “scalp-positive” is comparable to “active anode electrode with extra-cephalic return”. For all the limitations in this terminology, here we respect nomenclature as used in the original reports. Electrode dimensions are assumed to refer to contact area between the electrolyte (sponge) and skin.
Results
Figure 1 displays the diagram of search strategy and its results. Table 1 indicates the final selected studies. Given these 15 articles, the oldest where current was controlled was written in 1964. The majority of articles were small studies with number of patients varying from 1 to 107 (mean, 26 subjects). Approximately half of the studies (8 out of 15) were randomized controlled trials, but there were also two single blind and five open the studies. Most of the studies involved patients with psychiatric disorders, mainly major depression and schizophrenia (Figure 2B). Only four studies were performed using exclusively healthy subjects. Eight out of 15 studies were performed in United Kingdom and United States (Figure 2B). Positive results were obtained in most of the analyzed studies (Table 1).
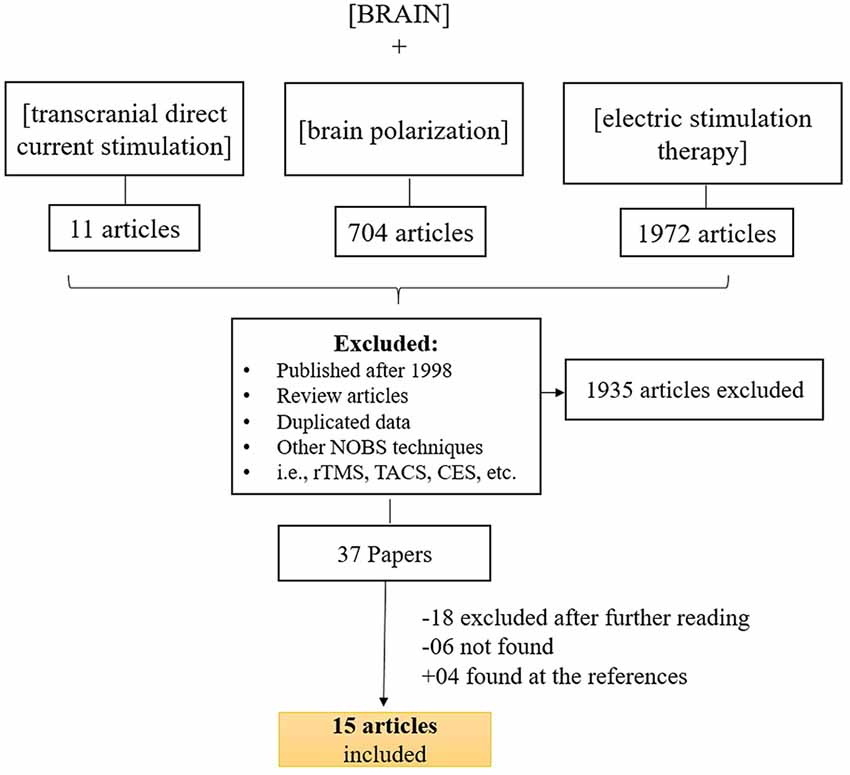
Figure 1. Search strategy for inclusion, exclusion criteria of this study. To identify relevant studies, we searched PubMed using the keywords (brain stimulation), (transcranial direct current stimulation) ad (electric stimulation therapy) along with (brain).
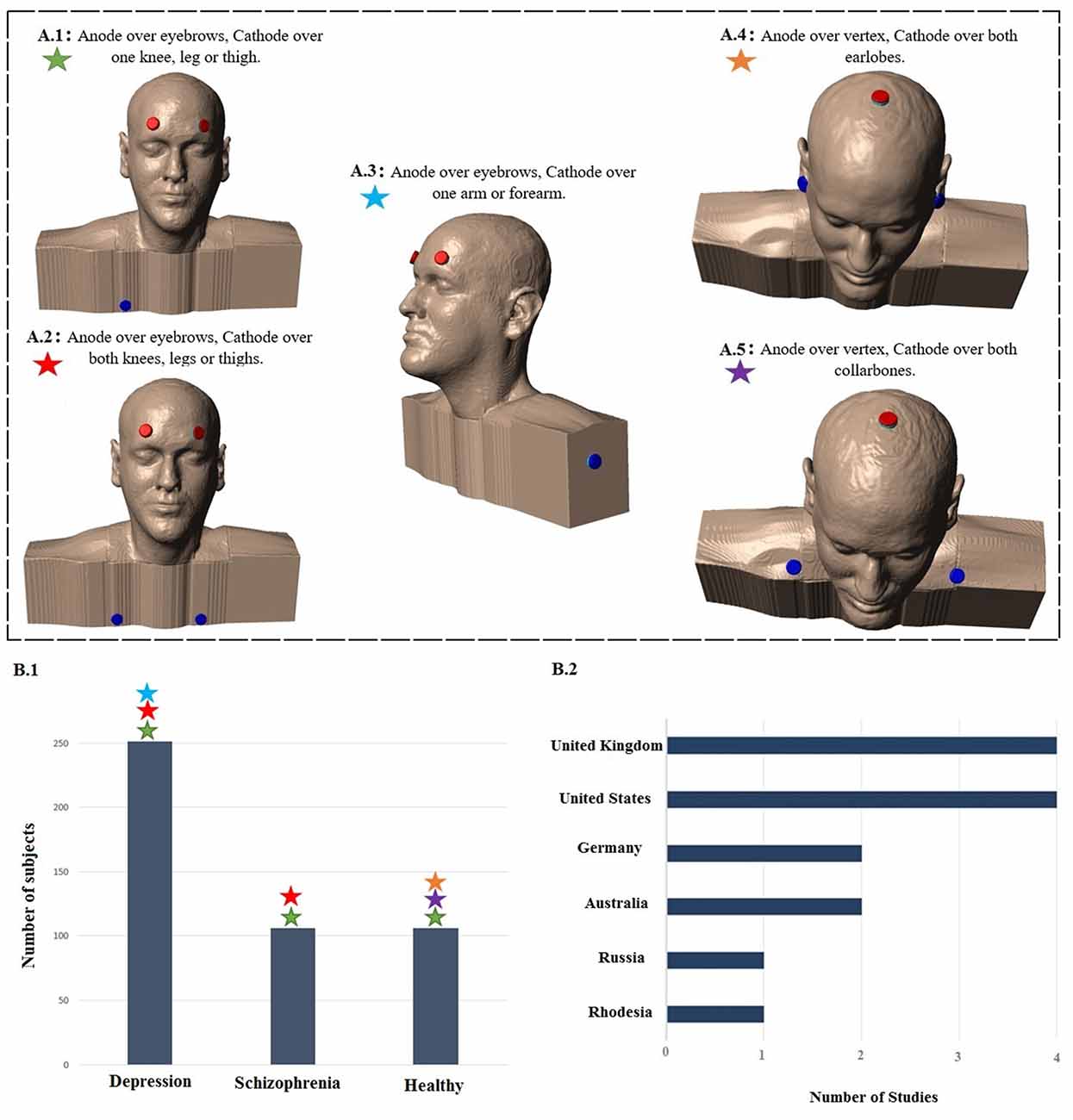
Figure 2. Summary of study parameters on human trials using transcranial direct current stimulation (tDCS) in old literature (from 1960 to 1998). Models of commonly used montages of tDCS in early studies (A); red: anode electrode(s), blue: cathode electrode(s). Total number of subjects in each group of patients participating in studies using aforementioned montages (B.1) and leading countries conducting tDCS studies in early stage with number of published articles (B.2).
tDCS Parameters
The intensity of electric current varied between studies. The median of most commonly used intensity was 0.33 mA for each anode; typically ranging from 0.1 mA (Redfearn et al., 1964) to 0.5 mA (Nias and Shapiro, 1974) for each anode. However, Lippold and Redfearn (1964) applied 3 mA in one single patient. The most common electrode montage was: active electrode(s) above eyebrow and reference electrode in extra-cephalic position (e.g., leg, hand; Figure 2A). The active electrodes were most commonly placed in the frontal—especially supraorbital—but also in occipital areas of the scalp and vertex. Apart from the leg and arm, other locations for the return electrode were also used such as the mastoid bone or collarbone. Historically, the approach of applying a stimulation over orbital fissures originated from two other failed trials conducted by Lippold and Redfearn (1964) and trial and error in electrode placement, current intensity and stimulation duration. They found that largest modification in mood and alertness would be produced when anode is placed over an orbital fissure and cathode at an extra-cephalic location (e.g., leg, thigh or arm). The essential differences between the two failed trials and the successful one was location of electrodes, lower applied current with longer duration of stimulation (Lippold and Redfearn, 1964) which was used in most of studies on depression afterwards.
Only 8 out of 15 studies specified the precise dimensions of the electrodes; in those ones the smallest active electrode was of 0.1 cm2 and the smallest reference electrode was of 0.2 cm2. The reference electrode area was often larger than the active ones, from approximately 30% (Lifshitz and Harper, 1968) to 50% bigger (Baker, 1970), but in some cases was the same (Elbert et al., 1981a). The use of a larger return electrode compared to active electrode is in line with modern conventions (Woods et al., 2016), though even the larger active and return electrodes are smaller than used in modern tDCS.
Most of studies employed several sessions of stimulation, with a median of 14 sessions. The quantity of session varied from one single to 120 sessions. The median of the total duration of stimulation was 30 h. Redfearn et al. (1964) conducted the longest study, with 960 h as the total time of stimulation.
The mean duration of session was 4.5 h (4 h and 30 min) with a maximum of 11 h (Redfearn et al., 1964) of electrical stimulation. Due to the long duration of stimulation in several studies, the devices were portable and patients were able to move around the hospital or go home (Lippold and Redfearn, 1964; Redfearn et al., 1964; Ramsay and Schlagenhauf, 1966; Baker, 1970). The regimen of sessions varied across articles—daily sessions or several days interval between sessions. In average, stimulation protocols consisted of applying 0.33 mA for 6 h per session that was continued up to 14 days.
In most included studies, stimulation apparatus was made of low voltage dry batteries in a pack with a potentiometer manually adjusted to produce a constant current. In a later study (Elbert et al., 1981b), an optocoupled system driven by the analog output provided constant current which had a ramp up period of 6 s to increase current from 0 mA to 0.25 mA. In all the studies, electrodes were metallic, either pure silver or silver chloride disks covered with saline soaked gauze or lint. Electrode contact and current was checked in pre-arranged times especially in studies with longer duration of stimulation.
Clinical and Side Effects
Twelve studies reported positive results. With the exception of Arfai et al. (1970), all other studies with melancholic or depressive patients showed some positive results using tDCS. The most common side effects reported were headache and skin sensitivity. Half of the studies did not mention any side effects.
Discussion
Across the limited historical use of tDCS between 1960 and 1998, there was little standardization of electrical parameters of stimuli. The lack of methodological rigidity on some parameters such as reference electrode position, number of sessions, the target area, current strength, electrode size and duration of each session might explain some contradictory findings between the studies. There was often limited information on subject inclusion and recruitment, in one case, not even the place of origin of the study was apparent (Herjanic and Moss-Herjanic, 1967).
The values of the current intensity used in the selected historical tDCS trials, from 0.1 mA to 0.5 mA (median 0.33 mA) for each anode(s), were overall lower than those ones used contemporarily in clinical trials, which vary between 1–3 mA (median 2 mA; Bikson et al., 2016). Potentially maximum current was constrained by hardware limitations (battery voltage), especially with the need for portability (small size and weight) and long duration operation (hours per session). Smaller electrodes were used in historical tDCS trials, but this may have marginal or no effects in resulting brain current density, compared to the linear loss with reduced current intensity (Miranda et al., 2009). Nitsche et al. (2008) demonstrated that, when stimulations durations are limited to several minutes, an intensity of 0.6 mA is required to induce a significant change in average cortical excitability detectable by TMS. Total stimulation charge was determined by the current and time. The neurophysiological consequence of lower-intensity stimulation but with longer period (e.g., hours) is unknown. In most cases included here the total charge applied (e.g., 4.5 h times 0.25 mA for each anode = 8100 mC1) was above that is used in modern tDCS (e.g., 2 mA for 20 min = 2400 mC). The side effect profile of the included historical trials, to the extent they were monitored and reported was mild.
Most of the studies placed the active electrode above the eyebrow and the reference one on the leg, or on the arm. This position of the active electrode approximates locations used in modern human trials. However, the “reference” electrode is now more commonly placed on the head; extra-cephalic “return” electrodes are sometimes used. Modern computational modeling studies suggest the use of extra-cephalic electrode produce significant current flow in deep and mid-brain structures (DaSilva et al., 2011). Indeed, Redfearn et al. (1964) suggested that highest current density in extra-cephalic stimulation could be in brainstem and supported it by evidence of respiratory depression caused by applying 3 mA cathodal stimulation in a normal subject.
Historical tDCS trials employed from 1 to 120 sessions with a median of 14 sessions, and a median of 4.5 h (20 min to 11 h) of electrical stimulation per session, resulting in a total duration of the trial with a median of 30 h (150 min to 960 h). Currently, it is known that stimulation duration of 20–30 min is more than enough to induce cortical excitability chances and consequently clinical improvements rather than hours of stimulation that would compromise patient’s compliance in clinical daily practice (imagine a patient using tDCS for hours at home).
The Use of Outcomes
The Hamilton Depression Rating Scale—HDRS (Hamilton, 1960), recognized as the gold standard in modern depression trials, although contemporary to the majority of early tDCS reviewed was not adopted. Rather early tDCS studies favored clinical outcomes and depression self-rating scales, more subjective and of difficult comparability. Only one study used the HDRS (Arfai et al., 1970). Other more objective measures used in depression trials were: laboratory changes (norepinephrine, serotonin, beta-endorphin and cholinesterase) and cardiac frequency. In the other conditions addressed, also subjective and objective outcomes assessment was conducted. Among the validated outcomes, the Benton Visual Retention Test (Benton, 1946) was used to evaluate the improvement in short-term memory in alcoholic patients. Tests of reaction to light stimuli were performed within a schizophrenic group of patients. Other studies took into account laboratory changes in hormone levels, self-report scales and several clinical outcomes such as remission of symptoms, improvement in terms of re-hospitalization and/or further treatment and medical evaluation.
Trial Design
The majority of the retrieved articles consisted of double blind controlled clinical trials, which is considered as the “gold standard” for intervention studies. On the other hand, some of them were inadequately reported, therefore making difficult to assess their quality. In a few of these studies, the blinding status was not clearly defined especially in those allocating patients with major depression. In fact, without an appropriate blinding, the results might be biased by a decrease of the placebo effect, as well as an increase of the number of false-positive results and over-estimate of the magnitude of an association. Another aspect to take into account is the high electrical density used that might have precluded blinding process. In most historical trials, the number of subjects was relativity small (indicating cautious interpretation of the results), this remains the case in modern tDCS pilot trials on new indications.
Adverse Effects
It is difficult to draw a reliable evaluation of the side effects from these works as the majority of articles did not post how many healthy subjects or patients were affected, and when multiple intensities were used did not correlate adverse events with intensity. There were no reports of subjects needing to terminate a session or receive medical care for injury. In contemporary tDCS trials, the most common side effects using standard protocols and montages—all transitory—are a mild tingling followed by itching and headache (Brunoni et al., 2011). Autonomic reactions are considered unlikely according to recent systematic review (Schestatsky et al., 2013). Historical studies lacked systematic questionnaire searching for adverse events, which might underestimate detection of occurrence.
Synopsis
In conclusion, we found 15 studies with semi-systematic approaches before the year of 1998, considered the time point of contemporary tDCS. For dosage, the use of multi-hour stimulation session, albeit with modestly reduced current intensity is a significant deviation from modern protocols. The use of supra-orbital active electrode(s) with an extra-cephalic return is another feature in these older studies, though rarely used in modern tDCS.
It is difficult to draw firm meta-conclusions from the analysis of the 15-included studies. This is due to lack of information regarding patient’s diagnosis and stimulation parameters as well as varied scientific rigor in design study. The most common type of patients addressed was from the psychiatric field. The occurrence of unusual adverse events i.e., papules, pustules and faint, might be related to longer duration of stimuli and higher density but also other conditions apart from the stimulation itself, such as stimulus-induced anxiety and unrelated events in patients.
Author Contributions
All authors contributed equally to this work.
Conflict of Interest Statement
MB has equity in Soterix Medical Inc. The City University of New York has patents on Brain Stimulation with MB as inventor.
The other authors declare that the research was conducted in the absence of any commercial or financial relationships that could be construed as a potential conflict of interest.
Acknowledgments
ARB is supported by the following grants: 2013 NARSAD Young Investigator from the Brain and Behavior Research Foundation (Grant Number 20493), 2013 FAPESP Young Researcher from the São Paulo State Foundation (Grant Number FAPESP 2012/20911-5) and National Council for Scientific and Technological Development (CNPq, Grant Number 470904). ARB is recipient of a research fellowship award from CNPq (303197). FF is supported by a NIH grant (R21 HD 079048-01 A1).
Footnotes
- ^ Mili-Coulomb (mC).
References
Arfai, E., Theano, G., Montagu, J. D., and Robin, A. A. (1970). A controlled study of polarization in depression. Br. J. Psychiatry 116, 433–434. doi: 10.1192/bjp.116.533.433
Baker, A. (1970). Brain stem polarization in the treatment of depression. S. Afr. Med. J. 44, 473–475.
Benton, A. L. (1946). A Visual Retention Test for Clinical Use. New York, NY: Psychological Corporation.
Bikson, M., Grossman, P., Thomas, C., Zannou, A. L., Jiang, J., Adnan, T., et al. (2016). Safety of transcranial direct current stimulation: evidence based update 2016. Brain Stimul. 9, 641–661. doi: 10.1016/j.brs.2016.06.004
Brunoni, A. R., Amadera, J., Berbel, B., Volz, M. S., Rizzerio, B. G., and Fregni, F. (2011). A systematic review on reporting and assessment of adverse effects associated with transcranial direct current stimulation. Int. J. Neuropsychopharmacol. 14, 1133–1145. doi: 10.1017/s1461145710001690
Brunoni, A. R., Nitsche, M. A., Bolognini, N., Bikson, M., Wagner, T., Merabet, L., et al. (2012). Clinical research with transcranial direct current stimulation (tDCS): challenges and future directions. Brain Stimul. 5, 175–195. doi: 10.1016/j.brs.2011.03.002
DaSilva, A. F., Volz, M. S., Bikson, M., and Fregni, F. (2011). Electrode positioning and montage in transcranial direct current stimulation. J. Vis. Exp. 51:e2744. doi: 10.3791/2744
Datta, A., Bansal, V., Diaz, J., Patel, J., Reato, D., and Bikson, M. (2009). Gyri-precise head model of transcranial direct current stimulation: improved spatial focality using a ring electrode versus conventional rectangular pad. Brain Stimul. 2, 201.e1–207.e1. doi: 10.1016/j.brs.2009.03.005
Elbert, T., Lutzenberger, W., Rockstroh, B., and Birbaumer, N. (1981a). The influence of low-level transcortical DC-currents on response speed in humans. Int. J. Neurosci. 14, 101–114. doi: 10.3109/00207458108985821
Elbert, T., Rockstroh, B., Lutzenberger, W., and Birbaumer, N. (1981b). The influence of low-level, event-related DC-currents during time estimation in humans. Int. J. Neurosci. 15, 103–106. doi: 10.3109/00207458108985850
Fregni, F., Boggio, P. S., Nitsche, M., Bermpohl, F., Antal, A., Feredoes, E., et al. (2005). Anodal transcranial direct current stimulation of prefrontal cortex enhances working memory. Exp. Brain Res. 166, 23–30. doi: 10.1007/s00221-005-2334-6
Fregni, F., Boggio, P. S., Nitsche, M. A., Rigonatti, S. P., and Pascual-Leone, A. (2006). Cognitive effects of repeated sessions of transcranial direct current stimulation in patients with depression. Depress. Anxiety 23, 482–484. doi: 10.1002/da.20201
Fregni, F., and Pascual-Leone, A. (2007). Technology insight: noninvasive brain stimulation in neurology—perspectives on the therapeutic potential of rTMS and tDCS. Nat. Clin. Pract. Neurol. 3, 383–393. doi: 10.1038/ncpneuro0530
Guleyupoglu, B., Schestatsky, P., Edwards, D., Fregni, F., and Bikson, M. (2013). Classification of methods in transcranial electrical stimulation (tES) and evolving strategy from historical approaches to contemporary innovations. J. Neurosci. Methods 219, 297–311. doi: 10.1016/j.jneumeth.2013.07.016
Hamilton, M. (1960). A rating scale for depression. J. Neurol. Neurosurg. Psychiatry 23, 56–62. doi: 10.1136/jnnp.23.1.56
Herjanic, M., and Moss-Herjanic, B. (1967). Clinical report on a new therapeutic technique: polarization. Can. Psychiatr. Assoc. J. 12, 423–424.
Lifshitz, K., and Harper, P. (1968). A trial of transcranial polarization in chronic schizophrenics. Br. J. Psychiatry 114, 635–637. doi: 10.1192/bjp.114.510.635
Lippold, O. C., and Redfearn, J. W. (1964). Mental changes resulting from the passage of small direct currents through the human brain. Br. J. Psychiatry 110, 768–772. doi: 10.1192/bjp.110.469.768
Miranda, P. C., Faria, P., and Hallett, M. (2009). What does the ratio of injected current to electrode area tell us about current density in the brain during tDCS? Clin. Neurophysiol. 120, 1183–1187. doi: 10.1016/j.clinph.2009.03.023
Nias, D. K., and Shapiro, M. B. (1974). The effects of small electrical currents upon depressive symptoms. Br. J. Psychiatry 125, 414–415. doi: 10.1192/bjp.125.4.414
Nitsche, M. A., Cohen, L. G., Wassermann, E. M., Priori, A., Lang, N., Antal, A., et al. (2008). Transcranial direct current stimulation: State of the art 2008. Brain Stimul. 1, 206–223. doi: 10.1016/j.brs.2008.06.004
Nitsche, M., and Paulus, W. (2000). Excitability changes induced in the human motor cortex by weak transcranial direct current stimulation. J. Physiol. 527, 633–639. doi: 10.1111/j.1469-7793.2000.t01-1-00633.x
Parent, A. (2004). Giovanni Aldini: from animal electricity to human brain stimulation. Can. J. Neurol. Sci. 31, 576–584. doi: 10.1017/s0317167100003851
Priori, A., Berardelli, A., Rona, S., Accornero, N., and Manfredi, M. (1998). Polarization of the human motor cortex through the scalp. Neuroreport 9, 2257–2260. doi: 10.1097/00001756-199807130-00020
Ramsay, J. C., and Schlagenhauf, G. (1966). Treatment of depression with low voltage direct current. South. Med. J. 59, 932–934. doi: 10.1097/00007611-196608000-00013
Redfearn, J. W., Lippold, O. C., and Costain, R. (1964). Preliminary account of the clinical effects of polarizing the brain in certain psychiatric disorders. Br. J. Psychiatry 110, 773–785. doi: 10.1192/bjp.110.469.773
Schestatsky, P., Simis, M., Freeman, R., Pascual-Leone, A., and Fregni, F. (2013). Non-invasive brain stimulation and the autonomic nervous system. Clin. Neurophysiol. 124, 1716–1728. doi: 10.1016/j.clinph.2013.03.020
Woods, A. J., Antal, A., Bikson, M., Boggio, P. S., Brunoni, A. R., Celnik, P., et al. (2016). A technical guide to tDCS, and related non-invasive brain stimulation tools. Clin. Neurophysiol. 127, 1031–1048. doi: 10.1016/j.clinph.2015.11.012
Keywords: tDCS, electric stimulation therapy, human, brain, review
Citation: Esmaeilpour Z, Schestatsky P, Bikson M, Brunoni AR, Pellegrinelli A, Piovesan FX, Santos MMSA, Menezes RB and Fregni F (2017) Notes on Human Trials of Transcranial Direct Current Stimulation between 1960 and 1998. Front. Hum. Neurosci. 11:71. doi: 10.3389/fnhum.2017.00071
Received: 08 July 2016; Accepted: 06 February 2017;
Published: 23 February 2017.
Edited by:
Aron K. Barbey, University of Illinois at Urbana–Champaign, USAReviewed by:
Filippo Brighina, University of Palermo, ItalyEmily Kappenman, San Diego State University, USA
Copyright © 2017 Esmaeilpour, Schestatsky, Bikson, Brunoni, Pellegrinelli, Piovesan, Santos, Menezes and Fregni. This is an open-access article distributed under the terms of the Creative Commons Attribution License (CC BY). The use, distribution and reproduction in other forums is permitted, provided the original author(s) or licensor are credited and that the original publication in this journal is cited, in accordance with accepted academic practice. No use, distribution or reproduction is permitted which does not comply with these terms.
*Correspondence: Felipe Fregni, fregni.felipe@mgh.harvard.edu