- 1Department of Radiology, First Affiliated Hospital, Guangxi University of Chinese Medicine, Nanning, China
- 2Department of Acupuncture, First Affiliated Hospital, Guangxi University of Chinese Medicine, Nanning, China
- 3Life Science Research Center, School of Life Science and Technology, Xidian University, Xi’an, China
- 4Department of Teaching, First Affiliated Hospital, Guangxi University of Chinese Medicine, Nanning, China
Background: Previous studies have revealed that the etiologies of premenstrual syndrome (PMS) refer to menstrual cycle related brain changes. However, its intrinsic neural mechanism is still unclear. The aim of the present study was to assess abnormal spontaneous brain activity and to explicate the intricate neural mechanism of PMS using resting state functional magnetic resonance imaging (RS-fMRI).
Materials and Methods: The data of 20 PMS patients (PMS group) and 21 healthy controls (HC group) were analyzed by regional homogeneity (ReHo) method during the late luteal phase of menstrual cycle. In addition, all the participants were asked to complete a daily record of severity of problems (DRSP) questionnaire.
Results: Compared with HC group, the results showed that PMS group had increased ReHo mainly in the bilateral precuneus, left inferior temporal cortex (ITC), right inferior frontal cortex (IFC) and left middle frontal cortex (MFC) and decreased ReHo in the right anterior cingulate cortex (ACC) at the luteal phase. Moreover, the PMS group had higher DRSP scores, and the DRSP scores positively correlated with ReHo in left MFC and negatively correlated with ReHo in the right ACC.
Conclusion: Our results suggest that abnormal spontaneous brain activity is found in PMS patients and the severity of symptom is specifically related to the left MFC and right ACC. The present findings may be beneficial to explicate the intricate neural mechanism of PMS.
Introduction
Premenstrual syndrome (PMS) refers to a series of cycling and relapsing physical, emotional, cognitive and behavioral symptoms that regularly recur during the late luteal phase of each menstrual cycle and relieve soon after the onset of menses (Tacani et al., 2015). It is estimated that more than 80% of women are affected by PMS (Halbreich, 2003). The symptoms of PMS are often mild, but many patients present gradually worsening symptoms within 10 years (Freeman, 2003), and approximately 3%–10% of PMS women suffer from severe syndrome associated with substantial distress or functional impairment which eventually reach the criteria for premenstrual dysphoric disorder (PMDD; Hamaideh et al., 2014; Ryu and Kim, 2015). PMS has a significant negative effect on woman’s quality of life, and disturbs family relationships, work, productivity, social activity and sexual relationships (Freeman, 2003; Halbreich et al., 2003). It is also an important risk factor for postpartum depression (Buttner et al., 2013). However, no characteristic symptoms and signs occur, nor is a recognizable physiological and anatomical factor identified in PMS. Thus, it is necessary to pay more attention to understanding the underlying mechanism of PMS.
Regarding the etiology mechanism of PMS, there exist a great argument on gonadal hormones (estrogen and progesterone), gene, psychosocial factors and certain central nervous system (CNS) pathways (Duvan et al., 2011; Rapkin and Akopians, 2012; Barth et al., 2015; Hantsoo and Epperson, 2015). The evidence from previous brain imaging studies has showed that PMS exists CNS dysfunctions. In the animal model, PMS is associated with dysregulation of hippocampus (Barth et al., 2014; Gao et al., 2014). As to the patients, the abnormalities of brain functional activity are also involved in PMS. Based on the single photon emission computed tomography (SPECT), the decreases of regional cerebral blood flow (rCBF) were reported to be located in the temporal lobes at luteal phase compared with the follicular phase in PMS patients (Buchpiguel et al., 2000). Liu Q. et al. (2015) found that compared with healthy subjects, women with PMS during luteal phase displayed decreased connectivity in the middle frontal gyrus and parahippocampal gyrus, and increased connectivity in the left medial/superior temporal gyri and precentral gyrus within default mode network (DMN). De Bondt et al. (2015) also indicated that there were relationships between the premenstrual-like symptoms and the increased functional connectivity (FC) of the posterior part of the DMN with the precuneus, middle frontal gyrus, the posterior cingulate and cuneus. The above-mentioned studies show that neural abnormalities are embedded in PMS. However, the underlying elements leading to CNS dysfunctions in PMS are not well understood. Substantial studies have demonstrated that psychological changes may be the pivotal factors resulting in the brain activity abnormalities (Andermann, 1960; Walker and McGlone, 2013; Ait-Belgnaoui et al., 2014). Given that PMS patients are tested with significant psychological changes in menstrual cycle, especially at the luteal phase (Liu Q. et al., 2015; Watanabe and Shirakawa, 2015). The efforts to investigate the correlates between psychological changes and neural abnormalities may extend our understanding of the neural mechanism of PMS. While the amounts of literature concerning psychological processes on brain activity of PMS are limited and the studies to explicate the intricate neural mechanism of PMS are still insufficient.
Resting state functional magnetic resonance imaging (RS-fMRI) is a useful tool to gather further insight into intricate functions of human brain (Bifone and Gozzi, 2011; Branco et al., 2016). Regional homogeneity (ReHo), a data-driven method, measures the similarity or synchronization of the time series of nearest neighboring voxels, and can detect intensity of regional spontaneous brain activity at the resting state (Zang et al., 2004). Combining the RS-fMRI and ReHo method, researchers have detected abnormal neural activity in the resting state of neuropsychiatric disorders (Yuan et al., 2008), such as Parkinson’s disease (Wu et al., 2009), depression (Guo et al., 2011) and schizophrenia (Liu H. et al., 2006). These findings have confirmed the measurement reliability and sensitivity of ReHo in the neuropsychiatric fields, which may be helpful to investigate the abnormal brain activity in PMS.
Thereby, the aim of this study was to investigate the spontaneous brain activity in the women with PMS and healthy controls (HC) at the late luteal phase by ReHo method. We hypothesized that there existed significant changes of the spontaneous brain activity in women with PMS compared with HC. We also hypothesized that the neuroimaging findings could be associated with the psychological changes in PMS.
Materials and Methods
Ethics Statement
All subjects were informed about the whole experiment procedure and signed a written informed consent form. This study was approved by the Medicine Ethics Committee of First Affiliated Hospital, Guangxi University of Chinese Medicine, Guangxi, China. All the research procedures of the present study were conducted in accordance with the Declaration of Helsinki.
Subjects
This study was performed on First Affiliated Hospital, Guangxi University of Chinese Medicine. Twenty-three patients (PMS-group) were recruited via advertisement in the Guangxi University of Chinese Medicine, Guangxi, China. To quantify premenstrual symptoms, all the patients were prospectively screened for 2 months and called for completing a daily record of severity of problems (DRSP) questionnaire decided by Dr. Endicott (Endicott et al., 2006; see Supplementary Material). Clinical diagnostic criteria for PMS were based on the recommendations and guidelines for PMS (Halbreich et al., 2007). Meanwhile, Diagnostic and Statistical Manual of Mental Disorders-5th Edition (DSM-5; American Psychiatric Association, 2013) was used to exclude patients from PMDD. All the patients were individually diagnosed by an experienced associated professor gynecologist. The inclusion criteria for PMS were met: (1) age ranged from 18 to 45 years old, being right-handed; (2) a regular menstrual cycle ranged from 24 to 35 days; (3) the premenstrual symptoms occurred up to 2 weeks before menses in most menstrual cycles; (4) symptoms remitted shortly following onset of menses and were absent during most of the mid-follicular phase of the menstrual cycle; (5) the symptoms were associated with impairment in daily functioning and/or relationships and/or caused suffering, such as emotional, behavioral and physical distress; (6) the menstrual-related cyclicity, occurrence during the late luteal phase of cycle (days −5 to −1) and absence during the middle follicular phase (days +6 to +10) were documented by repeated observations by the patients based on DRSP, and the mean luteal phase score was at least 30% greater than that of the follicular phase; and (7) the symptoms were not just an exacerbation or worsening of another mental or physical chronic disorders. The exclusion criteria for patients were as follows: (1) being currently pregnant or lactating; (2) having a history of thyroid disease, dysmenorrhea, gynecological inflammation, menopausal syndrome, hysterectomy or bilateral oophorectomy, mastopathy or cancer, or diabetes or any other structural diseases; (3) having psychiatric disorders by DSM-5 criteria, such as schizoaffective disorder, schizophrenia, organic mental disorder, delusional mental disorder, psychotic features coordinated or uncoordinated with mood or bipolar disorder; (4) treating with any steroid compound (including oral contraceptives and hormonal intrauterine devices), benzodiazepines, or other psychotropic drugs affecting PMS; (5) having any MRI contraindications; and (6) smoking or alcohol abuse.
Twenty-two age matched HC, right-handed women (HC group), with regular menstrual cycle of 24–35 days were recruited in this study. All the HC were free of psychiatric or neurological illness via assessment by medical history and physical examinations, and had no history of alcohol or drugs abuse. All the HC also underwent the same diagnostic screening tests.
Meanwhile, each subject was asked to complete an identical assessment protocol in the body mass index (BMI), women’s menstrual cycle, menophania, length of menstrual cycle, menstruation.
Experimental Paradigm
The PMS group and HC group were randomly arranged to receive fMRI examinations. Based on the females’ physical characteristics and hormone level, all the test dates were set at the late luteal phase, ranging from 1 to 5 days before menstruation. To confirm the relatively stable and low level of endogenous cortisol and estradiol, all of the scan tests were conducted between 20:00 and 22:00 pm (Bao et al., 2004). For menstrual cycle stage verification, we obtained prospective self-reports about when their menstruation started and combined this information with the primary gynecological examinations and B-ultrasonic wave results to arrange the test times. The subject then received a RS-fMRI scan for 6 min. During the scan, each subject was instructed to keep eyes closed, not to think about anything and to stay awake.
MRI Data Acquisition
MRI data were acquired using a 3.0 Tesla Siemens Magnetom Verio MRI System (Siemens Medical, Erlangen, Germany) at the Department of Radiology, First Affiliated Hospital, Guangxi University of Chinese Medicine, Nanning, Guangxi, China. To avoid head movement, each subject’s head was immobilized by foam pads in a standard 8-channel birdcage head coil. FMRI images were acquired with a single-shot gradient–recalled echo planar imaging (EPI) sequence with the parameters as following: repetition time (TR)/echo time (TE) = 2000 ms/30 ms, flip angle = 90°, field of view (FOV) = 240 mm × 240 mm, matrix size = 64 × 64, slice thickness = 5 mm and slices = 31. High resolution T1-weighted images were then obtained with a volumetric three-dimensional spoiled gradient recall sequence with the parameters as following: TR/TE = 1900 ms/2.22 ms, FOV = 250 mm × 250 mm, matrix size: 250 × 250, flip angle = 9°, slice thickness = 1 mm and 176 slices.
Image Preprocessing
Preprocessing was performed with SPM8 (SPM8)1. The first 10 volumes of each functional time series were removed to avoid the instability of the initial MRI signal. The remaining images were corrected for acquisition time delay between different slices and realigned to the first volume. The head motion parameters were calculated by estimating the translation in every direction and the angular rotation on each axis for every volume. If the translation was more than 1.5 mm in any cardinal direction and the rotation was more than 1.5° in each of the orthogonal x, y and z axes, the subject was discarded. The realigned functional images were then spatially normalized to the Montreal neurological institute space using the normalization parameters estimated by T1 structural image unified segmentation, re-sampled to 3 mm × 3 mm × 3 mm voxels. Several sources of spurious variance, such as the estimated motion parameters, average blood oxygenation level dependent (BOLD) signals in ventricular and white matter regions, were dislodged from the images. After removing the variance, linear drift was removed and temporal filter (0.01–0.08 Hz) was then performed on the time series of each voxel to reduce the effect of low-frequency drifts and high-frequency noise.
ReHo Analysis
The parameter of Kendall’s coefficient of concordance (KCC) was utilized to measure the similarity of time series of a given voxel to the ones of its 26 nearest voxels in a voxel-wise way based on the hypothesis that a voxel is temporally similar to the ones of its neighbors. Individual ReHo maps were created by computing KCC within a gray matter mask in a voxel-wise manner using REST Software2. When the center cube was on the edge of the gray matter mask, we only computed ReHo for a voxel if all of remaining nearest voxels were within the gray matter mask. For every subject, KCC map was normalized by dividing KCC in each voxel by the mean KCC of total gray matter. The KCC fMRI data were then spatially smoothed with a Gaussian kernel of 6 mm full-width at half-maximum.
Statistical Analysis
Demographic and clinical data were compared using two-sample t-test. The threshold level in all statistical analysis for significance criterion was determined at p < 0.05. Two sample t-test was then applied to examine different patterns of the spontaneous brain activity between the PMS patients and HC. All of the contrast threshold was set at p < 0.05 (false discovery rate (FDR) corrected). We applied correlation analysis to estimate the relationships between the influence of symptom severity of disease and the regions of interest (ROIs) showing the differences in PMS patients compared with HC. First, the 6 mm sphere around the peak voxels in the significant between-group clusters was formed as the ROIs. Pearson correlations between the mean ReHo of the ROIs and DRSP values were then estimated. The age, BMI, menstruation, menophania, length of menstrual cycle were considered as covariates of no interest in this study. Adjustment for multiple comparisons was made with the Bonferroni correction for correlation analysis (p < 0.05).
Results
Demographic and Clinical Results
In this study, three PMS patients and one HC were excluded from further data analysis because of distinct head movement. Finally, 20 women with PMS and 21 matched HC were included in our study. There were no significant differences in terms of age, BMI, menstruation (days), menophania (years), length of menstrual cycle (days) between the PMS group and HC-group (Table 1).
DRSP Result
The mean late luteal phase score of PMS group was 73.47 ± 7.84, it was the highest in groups. And the score variation rate from the middle follicular phase to the late luteal phase of PMS group was (46.24 ± 7.05)%. Each of the late luteal phase score in PMS group exceeded 50 and it was at least 30% larger than that of the follicular phase, which showed contrary to HC group (Table 2 and Figure 1).
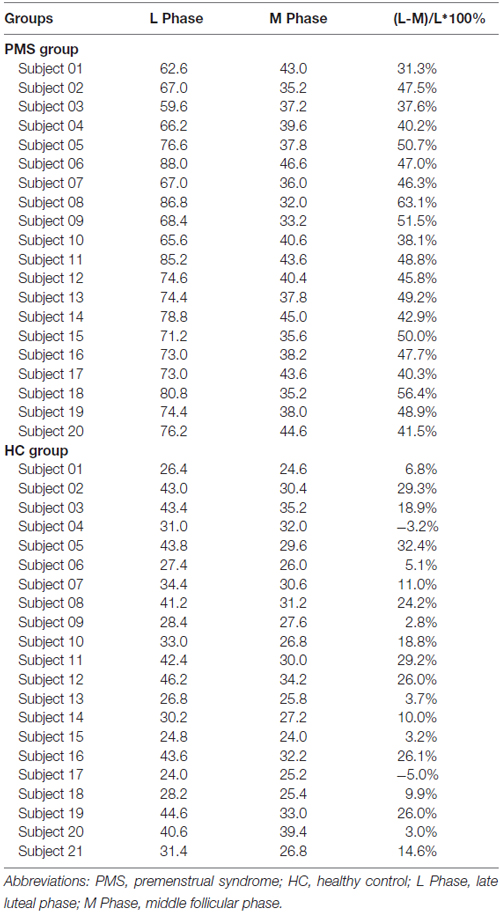
Table 2. The mean scores of late luteal phase and middle follicular phase and variation rate in PMS group and HC group.
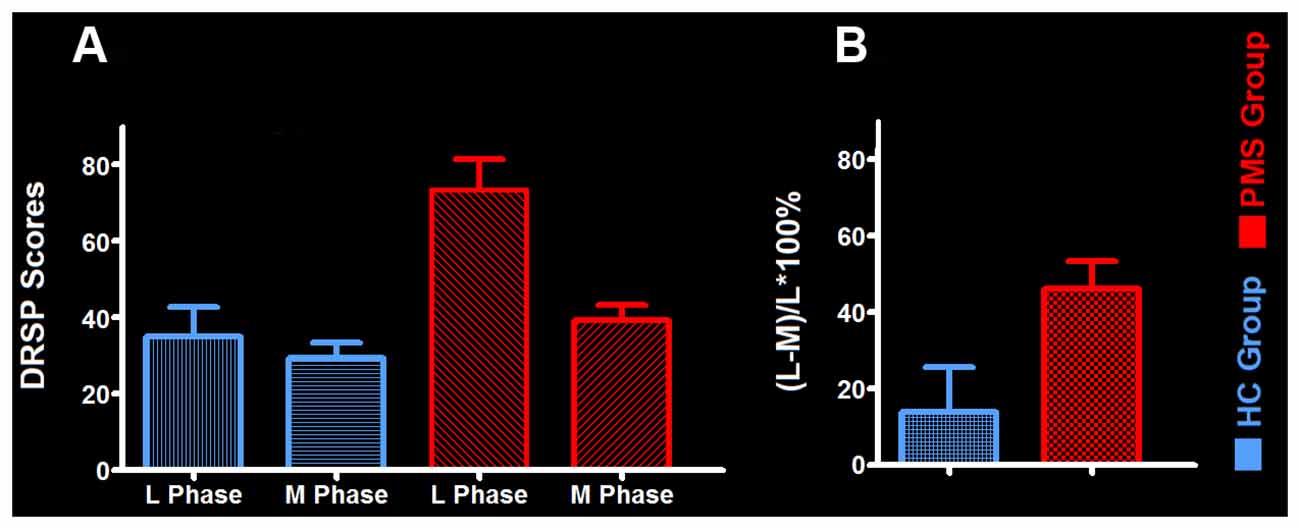
Figure 1. The mean daily record of severity of problems (DRSP) score and its variation rate between groups. (A) The mean DRSP score between PMS group and HC-group. (B) The variation rate of DRSP scores in PMS group and HC group. Abbreviations: PMS, premenstrual syndrome; HC, healthy control; L Phase, late luteal phase; M Phase, middle follicular phase.
Imaging Result
Compared with HC group, the results revealed that PMS group exhibited increased ReHo mainly in the bilateral precuneus, left inferior temporal cortex (ITC), right inferior frontal cortex (IFC) and left middle frontal cortex (MFC), as well as decreased ReHo in the right anterior cingulate cortex (ACC) at luteal phase (Figure 2).
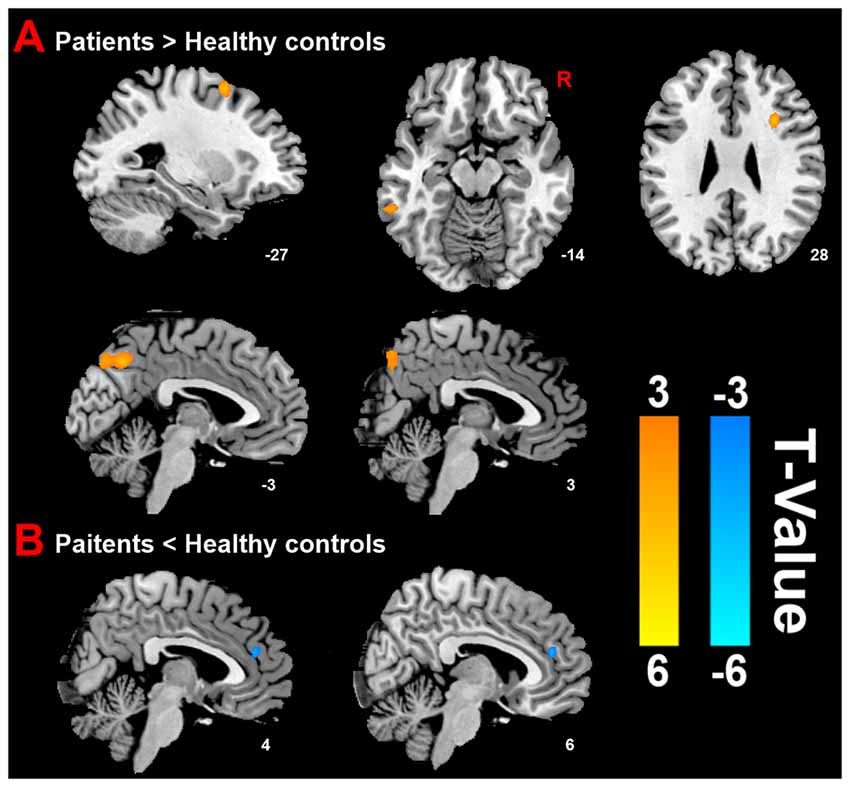
Figure 2. Distinct brain regions. (A) Increased regional homogeneity (ReHo) in brain regions between PMS patients and HC; (B) decreased ReHo in brain region between PMS patients and HC.
Correlation Analysis
Correlation analysis was applied to the ReHo and individual DRSP scores in the PMS group at luteal phase. The results indicated the DRSP scores in the PMS group positively correlated with the ReHo in the left MFC (r = 0.706, p = 0.001) and negatively correlated with the ReHo in the righ ACC (r = −0.656, p = 0.002; Figure 3).
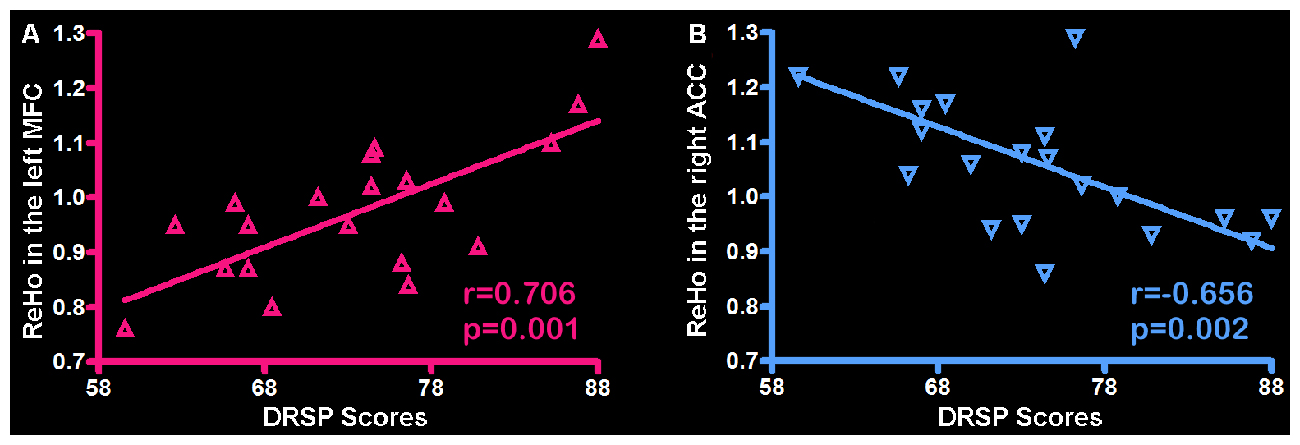
Figure 3. Correlation between the ReHo and individual DRSP scores in the PMS group. (A) The left MFC showed ReHo significant positive correlations with DRSP scores. (B) The right ACC showed ReHo significant negative correlations with DRSP scores. Each triangle represents the data from one subject. Abbreviation: DRSP, daily record of severity of problems; ReHo, Regional homogeneity; MFC, middle frontal cortex; ACC, anterior cingulate cortex.
Discussion
In present study, we adopted a RS-MRI strategy to investigate the spontaneous neural activity in PMS patients based on the ReHo method. Compared with HC, we observed altered patterns of spontaneous neural activity in PMS at luteal phase, mainly located in the bilateral precuneus, left ITC, right IFC, left MFC and right ACC. Moreover, in PMS group, the left MFC and right ACC were associated with the severity of clinical symptoms based on DRSP.
The DRSP is considered to be a preferred instrument for prospective assessment on premenstrual disorders, it can provide the sensitive, reliable and valid measures of the symptoms and functional impairment criteria for PMS (Endicott et al., 2006). The evidence from previous study has demonstrated that the higher score of DRSP means PMS patients being at more negative and stressful conditions (Watanabe and Shirakawa, 2015). While psychological changes are deemed to make important impacts on the brain activity. Based on our findings of the highest DRSP scores at the late luteal phase in PMS group, we infer that patients might feel more stress and negative mood at the late luteal phase, such significant psychological changes might eventually affect the brain activity of PMS patients.
The first major finding of the present study was the decreased ReHo in right ACC of PMS patients compared with HC. ACC is well known to be one of the most important higher-order brain structure exceedingly related to emotional and cognitive processing (Mayberg et al., 2002; Davis et al., 2005; Lavin et al., 2013). ACC is also a central component in cognitive and execution control system referred to detect incongruence between expectations and outcomes during decision-making processes (Vincent et al., 2008). When human brains are suffered from the conflict monitoring in the relationships of the structure to other functional networks with which they interact may have important consequences for attention, affect and/or emotion regulation, ACC shows its central monitored and adjustive functions in effectively allocating the resources to the center (Kerns et al., 2004; Petersen et al., 2014). A meta-analysis pointed out that ACC was also a special structure vulnerably involved in generating emotional responses or expressing negative emotion, it showed functional dysregulation when frequently confronted negative emotional stimuli including fear, anxiety, pain and emotional conflict, and patients with generalized anxiety and stress disorders were related to the weakened or impaired functionality of ACC (Etkin et al., 2011). As far as we know, PMS patients were cyclically suffered from negative stimuli in emotion, physic and behavior. Thereby, our finding of decreased ReHo in ACC indicates that there might exist functional abnormality or impairment in ACC. The researchers reported that the negative symptoms had been shown to have important effects on the activity of ACC and influenced the behavior of functional networks at rest during the menstrual cycle (Petersen et al., 2014). A RS-fMRI study on women brains found that the gray matter volume in the ACC was significantly associated with menstrual cycle (De Bondt et al., 2013). The aforementioned literature results revealed that ACC was a vulnerable and crucial region referred to functional abnormality at the late luteal phase, which was correlated with the clinical negative symptoms. Based on the findings of DRSP scores negative correlation with ReHo and the right ACC, it suggests that PMS patients might be affected by negative psychological stimuli, and then the ACC lose its higher-order cognitive, controlled and executive functions or central monitored and adjustive functions in allocating the resources to the center, which may partly contribute to underlying neural mechanism of PMS. Nevertheless, PMS is a special syndrome cyclically recurring during the late luteal phase but relieving soon after the onset of menses (Tacani et al., 2015). We speculate that patients may be at the periodic states from in-control to out-control. Thereby, besides the ACC, there might be existed other important structures being responsible for the baseline brain activity of PMS patients.
The precuneus represents a relevant cortical structure of the parietal lobes. It is a crucial node of the DMN involved in imagery, simulation visuospatial integration and self-awareness. It is also a core region that is responsible for baseline brain activity, and participates in functions such as intrinsic ongoing mental processes and fundamental cognitive social functions (Pereira-Pedro and Bruner, 2016). The precuneus is associated with interoceptive and emotional processing with widely distributed networks sharing connectivity with many brain regions in the frontal, temporal, occipital and parietal cortices (Tanaka and Kirino, 2016). It showed greater activation to incongruent stimuli than to congruent stimuli (Kitada et al., 2014). Halbreich et al. (2003) pointed out that the CNS of PMS patients were usually suffered from incongruent stimuli rooted in the gonadal hormone’s fluctuations. Here, based on the finding of activation in the precuneus revealed by the increased ReHo, we speculate that this phenomenon may be due to the effect of incongruent stimuli on patients’ brain. Meanwhile, the negative symptoms may affect the precuneus and certain brain regions, and then the precuneus enhances its core action on maintaining the baseline brain activity or strengthens its networks connectivity with other brain regions. However, as to the healthy female subjects, studies showed the contrary results with decreased activation in precuneus during the luteal phase. Kunisato et al. (2011) reported that the precuneus would be inclined to work at morbid state during luteal phase (Helmbold et al., 2016). Thereby, due to the activation of precuneus, its higher-order functions may be motivated, and then the physical, emotional, cognitive and behavioral symptoms of PMS would be cyclically relieved soon after luteal phase. This phenomenon may be partly contributed to the functional adjustment of the precuneus in PMS patients. However, the development of PMS might be not merely confined to one region, the neural foundation of PMS would be an intricate process and might be involved in multiple regions, networks or system. There may have other dysfunctions of brain regions or functional networks in PMS patients.
Besides the finding of increased ReHo in the precuneus, our study showed increased ReHo in left ITC of PMS patients at luteal phase. Temporal cortex generally plays a crucial role in auditory, language processing and memory. However, previous studies have indicated that temporal cortex also serves as a key region in patients with psychiatric disorders because of abnormal activity or structure was found in the subregions of temporal cortex (Kasai et al., 2003; Ma et al., 2012). Halbreich pointed out that PMS might be related to abnormal temporal-limbic circuitry which might be heredity or acquired in a very early age (Halbreich, 2003). A SPECT study showed the changes of rCBF in the temporal lobes on luteal phase compared with the follicular phase in PMS patients (Buchpiguel et al., 2000). Liu Q. et al. (2015) demonstrated that PMS females showed increased FC in temporal cortex at luteal phase. Our observations were consistent with previous findings with abnormal activation in temporal cortex. In present study, PMS patients showed abnormal reactivity in the left ITC and right ACC (a region of limbic system) at the luteal phase compared with HC. It should be pointed out that further analysis using FC method is needed for the temporal-limbic emotional circuitry reactivity between the left ITC and right ACC, which might be more important to investigate the potential neural mechanism behind the emotion related disorder in female with PMS.
The abnormal changes in right IFC and left MFC were other interesting findings in PMS patients. IFC and MFC are two important parts of prefrontal cortex, which are taken part in integration of cognitive, emotional behaviors by uniting emotional biasing signals or markers into decision making processing (Gusnard et al., 2001; Simpson et al., 2001). The emotional response inhibition of prefrontal cortex was sensitive to the variations of the menstrual cycle (Amin et al., 2006), the observation from this study showed that there was significantly raised activation in the prefrontal cortex when faced to emotional response inhibition during the luteal phase by comparing with the follicular phase. This prior finding was consistent with our results of increased ReHo in right IFC and left MFC. Prefrontal cortex was a special brain functional region which could significantly express some characteristic receptors contributed to the symptoms of PMS. Previous literature showed that prefrontal cortex enriched the CNS-related receptors concentration (Kugaya et al., 2003; Liu B. et al., 2015). These receptors have intrinsic influence on cognitive and emotional functions in the menstrual cycle (Bethea et al., 2000). It will further provide the neural base for explicating the right IFC and left MFC involved in PMS. Not coincidentally, as the sever form of PMS, PMDD patients were found similar abnormalities in prefrontal cortex. For example, Gingnell et al. (2013) showed that there was significantly increased reactivity in the prefrontal cortex when referred to negative emotional stimuli during the luteal phase. Moreover, PMDD patients had greater prefrontal activation than healthy subjects, and the abnormally increased activation of prefrontal cortex was correlated with the degree of the disease (Baller et al., 2013). Based on the finding of the DRSP scores positive correlation with ReHo in the left MFC, it could indicate that the left MFC might be another key region related to the degree symptom of PMS. Nevertheless, our observations were inconsistent with the findings of Liu Q. et al. (2015), the study demonstrated that the PMS patients had decreased activity in the MFC. We inferred that these incomplete coincidences with each other might be attributed to the different sample or analysis method, yet the MFC as an important region involving in the abnormal brain activity for the PMS patients was consensual. The increased ReHo in right IFC and left MFC suggests that the cortical emotional circuitry reactivity during negative stimuli is altered in PMS at the luteal phase, which might be part of the pathophysiology behind the emotional and cognitive symptoms or lack of emotional control reported by female with PMS. And the abnormalities within the right IFC and left MFC are speculated to hinder the processing of brain function and therefore to constitute a vicious cycle in the maintenance of clinical manifestations of PMS.
However, in holistic perspective, abnormal functional areas in PMS may go beyond the functions of single areas due to these areas usually interacting with each other to provide a more comprehensive brain system. In present study, we found the changed brain areas including the precuneus, ITC, ACC, IFC and MFC, which were referred to the default system functions. The DMN plays a vital role in self-referential activity, such as assessing characteristics of external and internal cues, planning the future, and remembering the past (Raichle and Snyder, 2007; Buckner et al., 2008). More importantly, theoretical models concerning the role of the default system in psychopathology had been well described in the previous literature (Zhao et al., 2007; Sheline et al., 2009; Messina et al., 2016). Given that psychological changes indeed occur in PMS, we conclude that the psychological changes might have some effects on modulating activity of DMN in PMS patients during the luteal phase. Our conclusion is in accordance with previous studies (De Bondt et al., 2015; Liu Q. et al., 2015), which observed the abnormal activity in the DMN for PMS patients. Thereby, the changed DMN would attach much more importance to the neural mechanism of PMS.
Our study was subject to some limitations that need to be taken into consideration: (1) the luteal phase was a key stage involved in PMS, PMS patients mainly referred to a series of symptoms in this phase. So, our study merely made an investigation on the abnormal brain activity of PMS patients at luteal phase by comparing with HC. The study did not investigate whether or not abnormal brain activity might exist among the luteal phase and follicular phase in PMS group and HC group, we would pay more concern on these topics in next stage. (2) Another limitation of the present study was the relatively limited sample size. Enlargement of the sample size would be the direction of our future study; and (3) The method of ReHo can not directly show the connectivity between brain regions, further analysis and application by diverse data analysis methods (e.g., FC) or multi modal functional magnetic resonance imaging may extend our understanding of the neural mechanism of PMS.
Conclusion
To summarize, we observed the changed patterns of spontaneous neural activity in PMS patients during the resting state, with increased ReHo in the bilateral precuneus, left ITC, right IFC, left MFC and decreased ReHo in the right ACC at luteal phase. Furthermore, our findings showed that the DRSP scores (an important index for assessing PMS severity and symptoms) in the PMS group positively correlated with ReHo in the left MFC and negatively correlated with the ReHo in the right ACC. The present findings may enhance our understanding of the neurobiological underpinnings of PMS.
Author Contributions
DD made substantial contributions to the overall conception of the work, designed the experiment, revised and handled the manuscript, accurately answered all the questions from the reviewers, ensured the integrity of the work and approved the final version to be published. HLiao made important contribution to the literature review, interpreted data for the work, drafted the manuscript, revised some critical structure and intellectual content, advised on the integrity of the work and approved the final version to be published. YP provided the whole designed theory of the study, made substantial contributions to the overall design and guideline of experiment, interpreted intellectual content, substantial work on drafting the manuscript and approved the final version to be published. PL conducted the data processing, interpreted intellectual content in disease, was accountable for some aspects of the work in ensuring that questions related to the accuracy of the work were appropriately resolved and approved the final version to be published. HLiu executed the diagnosis and assessment of patients, recruited subjects for this study, advised on the improvement of protocols, provided important intellectual content in experiment and approved the final version to be published. GD made important contribution to the design of MR scan protocols, carried out MRI operation, MRI data acquisition and storage and approved the final version to be published. YL conducted the data processing and analysis for the functional MRI data, interpreted the conceptions of data processing, wrote the procedure of data processing and approved the final version to be published. LT, JT, DW, SL and LL recruited subjects for this study, conducted the assessment the symptom, diagnosis and treatment of the patients, gave some important advices for the accuracy interpretation or description of conceptions related to the disease and approved the final version to be published.
Conflict of Interest Statement
The authors declare that the research was conducted in the absence of any commercial or financial relationships that could be construed as a potential conflict of interest.
Acknowledgments
The present study was supported by the Guangxi Natural Science Foundation (Grant No. 2011GXNSFA018176, 2016GXNSFAA380006); the National Natural Science Foundation of China (Grant No. 81471738, 81303060).
Abbreviations
ACC, Anterior cingulate cortex; BMI, Body mass index; BOLD, Blood oxygenation level dependent; CNS, Central nervous system; DMN, Default mode network; DSM-5, Diagnostic and Statistical Manual of Mental Disorders-5th Edition; DRSP, Daily rating of severity of problems; EPI, Echo planar imaging; FC, Functional connectivity; FDR, False discovery rate; FOV, Field of view; HC, Healthy controls; IFC, Inferior frontal cortex; ITC, Inferior temporal cortex; KCC, Kendall’s coefficient of concordance; MFC, Middle frontal cortex; PMDD, Premenstrual dysphoric disorder; PMS, Premenstrual syndrome; rCBF, regional cerebral blood flow; ReHo, Regional homogeneity; ROIs, Regions of interest; RS-fMRI, Resting state functional magnetic resonance imaging; SPECT, Single photon emission computed tomography; TE, Echo time; TR, Repetition time.
Footnotes
Supplementary Material
The Supplementary Material for this article can be found online at: http://journal.frontiersin.org/article/10.3389/fnhum.2017.00062/full#supplementary-material
References
Ait-Belgnaoui, A., Colom, A., Braniste, V., Ramalho, L., Marrot, A., Cartier, C., et al. (2014). Probiotic gut effect prevents the chronic psychological stress-induced brain activity abnormality in mice. Neurogastroenterol. Motil. 26, 510–520. doi: 10.1111/nmo.12295
American Psychiatric Association. (2013). Diagnostic and Statistical Manual of Mental Disorders: DSM-5TM. 5th Edn. Arlington, VA: American Psychiatric Publishing, Inc.
Amin, Z., Epperson, C. N., Constable, R. T., and Canli, T. (2006). Effects of estrogen variation on neural correlates of emotional response inhibition. Neuroimage 32, 457–464. doi: 10.1016/j.neuroimage.2006.03.013
Andermann, K. (1960). Cerebral abnormality and the electroencephalogram in relation to psychiatry. Part II. Interrelations of emotional factors with cerebral abnormality and electroencephalographic manifestations of psychological functions. Med. J. Aust. 47, 765–772.
Baller, E. B., Wei, S. M., Kohn, P. D., Rubinow, D. R., Alarcón, G., Schmidt, P. J., et al. (2013). Abnormalities of dorsolateral prefrontal function in women with premenstrual dysphoric disorder: a multimodal neuroimaging study. Am. J. Psychiatry 170, 305–314. doi: 10.1176/appi.ajp.2012.12030385
Bao, A.-M., Ji, Y.-F., Van Someren, E. J., Hofman, M. A., Liu, R.-Y., and Zhou, J.-N. (2004). Diurnal rhythms of free estradiol and cortisol during the normal menstrual cycle in women with major depression. Horm. Behav. 45, 93–102. doi: 10.1016/j.yhbeh.2003.09.004
Barth, A. M., Ferando, I., and Mody, I. (2014). Ovarian cycle-linked plasticity of δ-GABAA receptor subunits in hippocampal interneurons affects γ oscillations in vivo. Front. Cell. Neurosci. 8:222. doi: 10.3389/fncel.2014.00222
Barth, C., Villringer, A., and Sacher, J. (2015). Sex hormones affect neurotransmitters and shape the adult female brain during hormonal transition periods. Front. Neurosci. 9:37. doi: 10.3389/fnins.2015.00037
Bethea, C. L., Gundlah, C., and Mirkes, S. J. (2000). Ovarian steroid action in the serotonin neural system of macaques. Novartis Found. Symp. 230, 112–130; discussion 130–113. doi: 10.1002/0470870818.ch9
Bifone, A., and Gozzi, A. (2011). Functional and pharmacological MRI in understanding brain function at a systems level. Curr. Top. Behav. Neurosci. 7, 323–357. doi: 10.1007/7854_2010_103
De Bondt, T., Jacquemyn, Y., Van Hecke, W., Sijbers, J., Sunaert, S., and Parizel, P. M. (2013). Regional gray matter volume differences and sex-hormone correlations as a function of menstrual cycle phase and hormonal contraceptives use. Brain Res. 1530, 22–31. doi: 10.1016/j.brainres.2013.07.034
De Bondt, T., Smeets, D., Pullens, P., Van Hecke, W., Jacquemyn, Y., and Parizel, P. M. (2015). Stability of resting state networks in the female brain during hormonal changes and their relation to premenstrual symptoms. Brain Res. 1624, 275–285. doi: 10.1016/j.brainres.2015.07.045
Branco, P., Seixas, D., Deprez, S., Kovacs, S., Peeters, R., Castro, S. L., et al. (2016). Resting-state functional magnetic resonance imaging for language preoperative planning. Front. Hum. Neurosci. 10:11. doi: 10.3389/fnhum.2016.00011
Buchpiguel, C., Alavi, A., Crawford, D., Freeman, E., and Newberg, A. (2000). Changes in cerebral blood flow associated with premenstrual syndrome: a preliminary study. J. Psychosom. Obstet. Gynaecol. 21, 157–165. doi: 10.3109/01674820009075623
Buckner, R. L., Andrews-Hanna, J. R., and Schacter, D. L. (2008). The brain’s default network: anatomy, function, and relevance to disease. Ann. N. Y. Acad. Sci. 1124, 1–38. doi: 10.1196/annals.1440.011
Buttner, M. M., Mott, S. L., Pearlstein, T., Stuart, S., Zlotnick, C., and O’Hara, M. W. (2013). Examination of premenstrual symptoms as a risk factor for depression in postpartum women. Arch. Womens Ment. Health 16, 219–225. doi: 10.1007/s00737-012-0323-x
Davis, K. D., Taylor, K. S., Hutchison, W. D., Dostrovsky, J. O., McAndrews, M. P., Richter, E. O., et al. (2005). Human anterior cingulate cortex neurons encode cognitive and emotional demands. J. Neurosci. 25, 8402–8406. doi: 10.1523/JNEUROSCI.2315-05.2005
Duvan, C. I., Cumaoglu, A., Turhan, N. O., Karasu, C., and Kafali, H. (2011). Oxidant/antioxidant status in premenstrual syndrome. Arch. Gynecol. Obstet. 283, 299–304. doi: 10.1007/s00404-009-1347-y
Endicott, J., Nee, J., and Harrison, W. (2006). Daily record of severity of problems (DRSP): reliability and validity. Arch. Womens Ment. Health 9, 41–49. doi: 10.1007/s00737-005-0103-y
Etkin, A., Egner, T., and Kalisch, R. (2011). Emotional processing in anterior cingulate and medial prefrontal cortex. Trends Cogn. Sci. 15, 85–93. doi: 10.1016/j.tics.2010.11.004
Freeman, E. W. (2003). Premenstrual syndrome and premenstrual dysphoric disorder: definitions and diagnosis. Psychoneuroendocrinology 28, 25–37. doi: 10.1016/S0306-4530(03)00099-4
Gao, X., Sun, P., Qiao, M., Wei, S., Xue, L., and Zhang, H. (2014). Shu-Yu capsule, a traditional Chinese medicine formulation, attenuates premenstrual syndrome depression induced by chronic stress constraint. Mol. Med. Rep. 10, 2942–2948. doi: 10.3892/mmr.2014.2599
Gingnell, M., Bannbers, E., Wikström, J., Fredrikson, M., and Sundstrom-Poromaa, I. (2013). Premenstrual dysphoric disorder and prefrontal reactivity during anticipation of emotional stimuli. Eur. Neuropsychopharmacol. 23, 1474–1483. doi: 10.1016/j.euroneuro.2013.08.002
Guo, W.-B., Liu, F., Xue, Z.-M., Yu, Y., Ma, C.-Q., Tan, C.-L., et al. (2011). Abnormal neural activities in first-episode, treatment-naive, short-illness-duration and treatment-response patients with major depressive disorder: a resting-state fMRI study. J. Affect. Disord. 135, 326–331. doi: 10.1016/j.jad.2011.06.048
Gusnard, D. A., Akbudak, E., Shulman, G. L., and Raichle, M. E. (2001). Medial prefrontal cortex and self-referential mental activity: relation to a default mode of brain function. Proc. Natl. Acad. Sci. U S A 98, 4259–4264. doi: 10.1073/pnas.071043098
Halbreich, U. (2003). The etiology, biology, and evolving pathology of premenstrual syndromes. Psychoneuroendocrinology 28, 55–99. doi: 10.1016/s0306-4530(03)00097-0
Halbreich, U., Backstrom, T., Eriksson, E., O’brien, S., Calil, H., Ceskova, E., et al. (2007). Clinical diagnostic criteria for premenstrual syndrome and guidelines for their quantification for research studies. Gynecol. Endocrinol. 23, 123–130. doi: 10.1080/09513590601167969
Halbreich, U., Borenstein, J., Pearlstein, T., and Kahn, L. S. (2003). The prevalence, impairment, impact, and burden of premenstrual dysphoric disorder (PMS/PMDD). Psychoneuroendocrinology 28, 1–23. doi: 10.1016/s0306-4530(03)00098-2
Hamaideh, S. H., Al-Ashram, S. A., and Al-Modallal, H. (2014). Premenstrual syndrome and premenstrual dysphoric disorder among Jordanian women. J. Psychiatr. Ment. Health Nurs. 21, 60–68. doi: 10.1007/978-3-319-05870-2_18
Hantsoo, L., and Epperson, C. N. (2015). Premenstrual dysphoric disorder: epidemiology and treatment. Curr. Psychiatry Rep. 17:87. doi: 10.1007/s11920-015-0628-3
Helmbold, K., Zvyagintsev, M., Dahmen, B., Biskup, C. S., Bubenzer-Busch, S., Gaber, T. J., et al. (2016). Serotonergic modulation of resting state default mode network connectivity in healthy women. Amino Acids 48, 1109–1120. doi: 10.1007/s00726-015-2137-4
Kasai, K., Shenton, M. E., Salisbury, D. F., Hirayasu, Y., Lee, C. U., Ciszewski, A. A., et al. (2003). Progressive decrease of left superior temporal gyrus gray matter volume in patients with first-episode schizophrenia. Am. J. Psychiatry 160, 156–164. doi: 10.1176/appi.ajp.160.1.156
Kerns, J. G., Cohen, J. D., MacDonald, A. W. III, Cho, R. Y., Stenger, V. A., and Carter, C. S. (2004). Anterior cingulate conflict monitoring and adjustments in control. Science 303, 1023–1026. doi: 10.1126/science.1089910
Kitada, R., Sasaki, A. T., Okamoto, Y., Kochiyama, T., and Sadato, N. (2014). Role of the precuneus in the detection of incongruency between tactile and visual texture information: a functional MRI study. Neuropsychologia 64, 252–262. doi: 10.1016/j.neuropsychologia.2014.09.028
Kugaya, A., Epperson, C. N., Zoghbi, S., van Dyck, C. H., Hou, Y., Fujita, M., et al. (2003). Increase in prefrontal cortex serotonin 2A receptors following estrogen treatment in postmenopausal women. Am. J. Psychiatry 160, 1522–1524. doi: 10.1176/appi.ajp.160.8.1522
Kunisato, Y., Okamoto, Y., Okada, G., Aoyama, S., Demoto, Y., Munakata, A., et al. (2011). Modulation of default-mode network activity by acute tryptophan depletion is associated with mood change: a resting state functional magnetic resonance imaging study. Neurosci. Res. 69, 129–134. doi: 10.1016/j.neures.2010.11.005
Lavin, C., Melis, C., Mikulan, E., Gelormini, C., Huepe, D., and Ibañez, A. (2013). The anterior cingulate cortex: an integrative hub for human socially-driven interactions. Front. Neurosci. 7:64. doi: 10.3389/fnins.2013.00064
Liu, Q., Li, R., Zhou, R., Li, J., and Gu, Q. (2015). Abnormal resting-state connectivity at functional MRI in women with premenstrual syndrome. PLoS One 10:e0136029. doi: 10.1371/journal.pone.0136029
Liu, H., Liu, Z., Liang, M., Hao, Y., Tan, L., Kuang, F., et al. (2006). Decreased regional homogeneity in schizophrenia: a resting state functional magnetic resonance imaging study. Neuroreport 17, 19–22. doi: 10.1097/01.wnr.0000195666.22714.35
Liu, B., Wang, G., Gao, D., Gao, F., Zhao, B., Qiao, M., et al. (2015). Alterations of GABA and glutamate–glutamine levels in premenstrual dysphoric disorder: a 3T proton magnetic resonance spectroscopy study. Psychiatry Res. 231, 64–70. doi: 10.1016/j.pscychresns.2014.10.020
Ma, C., Ding, J., Li, J., Guo, W., Long, Z., Liu, F., et al. (2012). Resting-state functional connectivity bias of middle temporal gyrus and caudate with altered gray matter volume in major depression. PLoS One 7:e45263. doi: 10.1371/journal.pone.0045263
Mayberg, H. S., Silva, J. A., Brannan, S. K., Tekell, J. L., Mahurin, R. K., Mcginnis, S., et al. (2002). The functional neuroanatomy of the placebo effect. Am. J. Psychiatry 159, 728–737. doi: 10.1176/appi.ajp.159.5.728
Messina, I., Bianco, F., Cusinato, M., Calvo, V., and Sambin, M. (2016). Abnormal default system functioning in depression: implications for emotion regulation. Front. Psychol. 7:858. doi: 10.3389/fpsyg.2016.00858
Pereira-Pedro, A. S., and Bruner, E. (2016). Sulcal pattern, extension, and morphology of the precuneus in adult humans. Ann. Anat. 208, 85–93. doi: 10.1016/j.aanat.2016.05.001
Petersen, N., Kilpatrick, L. A., Goharzad, A., and Cahill, L. (2014). Oral contraceptive pill use and menstrual cycle phase are associated with altered resting state functional connectivity. Neuroimage 90, 24–32. doi: 10.1016/j.neuroimage.2013.12.016
Raichle, M. E., and Snyder, A. Z. (2007). A default mode of brain function: a brief history of an evolving idea. Neuroimage 37, 1083–1090; discussion 1097–1089. doi: 10.1016/j.neuroimage.2007.02.041
Rapkin, A. J., and Akopians, A. L. (2012). Pathophysiology of premenstrual syndrome and premenstrual dysphoric disorder. Menopause Int. 18, 52–59. doi: 10.1258/mi.2012.012014
Ryu, A., and Kim, T. H. (2015). Premenstrual syndrome: a mini review. Maturitas 82, 436–440. doi: 10.1016/j.maturitas.2015.08.010
Sheline, Y. I., Barch, D. M., Price, J. L., Rundle, M. M., Vaishnavi, S. N., Snyder, A. Z., et al. (2009). The default mode network and self-referential processes in depression. Proc. Natl. Acad. Sci. U S A 106, 1942–1947. doi: 10.1073/pnas.0812686106
Simpson, J. R. Jr., Snyder, A. Z., Gusnard, D. A., and Raichle, M. E. (2001). Emotion-induced changes in human medial prefrontal cortex: I. During cognitive task performance. Proc. Natl. Acad. Sci. U S A 98, 683–687. doi: 10.1073/pnas.98.2.683
Tacani, P. M., Ribeiro Dde, O., Barros Guimarães, B. E., Machado, A. F., and Tacani, R. E. (2015). Characterization of symptoms and edema distribution in premenstrual syndrome. Int. J. Womens Health 7, 297–303. doi: 10.2147/IJWH.S74251
Tanaka, S., and Kirino, E. (2016). Functional connectivity of the precuneus in female university students with long-term musical training. Front. Hum. Neurosci. 10:328. doi: 10.3389/fnhum.2016.00328
Vincent, J. L., Kahn, I., Snyder, A. Z., Raichle, M. E., and Buckner, R. L. (2008). Evidence for a frontoparietal control system revealed by intrinsic functional connectivity. J. Neurophysiol. 100, 3328–3342. doi: 10.1152/jn.90355.2008
Walker, S. C., and McGlone, F. P. (2013). The social brain: neurobiological basis of affiliative behaviours and psychological well-being. Neuropeptides 47, 379–393. doi: 10.1016/j.npep.2013.10.008
Watanabe, K., and Shirakawa, T. (2015). Characteristics of perceived stress and salivary levels of secretory Immunoglobulin A and cortisol in Japanese women with premenstrual syndrome. Nurs. Midwifery Stud. 4:e24795. doi: 10.17795/nmsjournal24795
Wu, T., Long, X., Zang, Y., Wang, L., Hallett, M., Li, K., et al. (2009). Regional homogeneity changes in patients with Parkinson’s disease. Hum. Brain Mapp. 30, 1502–1510. doi: 10.1002/hbm.20622
Yuan, Y., Zhang, Z., Bai, F., Yu, H., Shi, Y., Qian, Y., et al. (2008). Abnormal neural activity in the patients with remitted geriatric depression: a resting-state functional magnetic resonance imaging study. J. Affect. Disord. 111, 145–152. doi: 10.1016/j.jad.2008.02.016
Zang, Y., Jiang, T., Lu, Y., He, Y., and Tian, L. (2004). Regional homogeneity approach to fMRI data analysis. Neuroimage 22, 394–400. doi: 10.1016/j.neuroimage.2003.12.030
Keywords: premenstrual syndrome, resting state, fMRI, regional homogeneity, brain
Citation: Liao H, Pang Y, Liu P, Liu H, Duan G, Liu Y, Tang L, Tao J, Wen D, Li S, Liang L and Deng D (2017) Abnormal Spontaneous Brain Activity in Women with Premenstrual Syndrome Revealed by Regional Homogeneity. Front. Hum. Neurosci. 11:62. doi: 10.3389/fnhum.2017.00062
Received: 10 November 2016; Accepted: 30 January 2017;
Published: 13 February 2017.
Edited by:
Luis Manuel Colon-Perez, University of Florida, USAReviewed by:
Irene Messina, University of Padua, ItalyJiliang Fang, China Academy of Chinese Medical Sciences, China
Copyright © 2017 Liao, Pang, Liu, Liu, Duan, Liu, Tang, Tao, Wen, Li, Liang and Deng. This is an open-access article distributed under the terms of the Creative Commons Attribution License (CC BY). The use, distribution and reproduction in other forums is permitted, provided the original author(s) or licensor are credited and that the original publication in this journal is cited, in accordance with accepted academic practice. No use, distribution or reproduction is permitted which does not comply with these terms.
*Correspondence: Demao Deng, ZGVtYW9kZW5nQDE2My5jb20=
† These authors have contributed equally to this work.