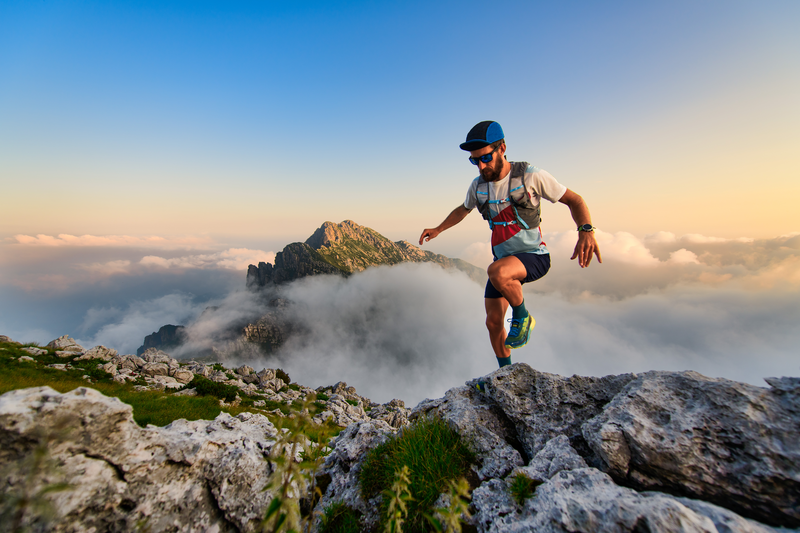
94% of researchers rate our articles as excellent or good
Learn more about the work of our research integrity team to safeguard the quality of each article we publish.
Find out more
ORIGINAL RESEARCH article
Front. Hum. Neurosci. , 11 January 2016
Sec. Cognitive Neuroscience
Volume 9 - 2015 | https://doi.org/10.3389/fnhum.2015.00709
This article is part of the Research Topic Online and Offline Modulators of Motor Learning View all 15 articles
Healthy sleep is essential in children’s cognitive, behavioral, and emotional development. However, remarkably little is known about the influence of sleep disorders on different memory processes in childhood. Such data could give us a deeper insight into the effect of sleep on the developing brain and memory functions and how the relationship between sleep and memory changes from childhood to adulthood. In the present study we examined the effect of sleep disorder on declarative and non-declarative memory consolidation by testing children with sleep-disordered breathing (SDB) which is characterized by disrupted sleep structure. We used a story recall task to measure declarative memory and Alternating Serial Reaction time (ASRT) task to assess non-declarative memory. This task enables us to measure two aspects of non-declarative memory, namely general motor skill learning and sequence-specific learning. There were two sessions: a learning phase and a testing phase, separated by a 12 h offline period with sleep. Our data showed that children with SDB exhibited a generally lower declarative memory performance both in the learning and testing phase; however, both the SDB and control groups exhibited retention of the previously recalled items after the offline period. Here we showed intact non-declarative consolidation in SDB group in both sequence-specific and general motor skill. These findings suggest that sleep disorders in childhood have a differential effect on different memory processes (online vs. offline) and give us insight into how sleep disturbances affects developing brain.
Healthy sleep is critical for children’s cognitive, behavioral, and emotional development. Unfortunately, sleep disturbances are common in childhood, including both primary (e.g., insomnia and sleep apnea) and secondary sleep disorders (other illnesses e.g., depression or bad/altered sleep hygiene results the sleep disorders; Anuntaseree et al., 2001; Rosen et al., 2003; Bixler et al., 2009). The prevalence of sleep disorders in childhood estimates vary from 0.7 to 13% (Brunetti et al., 2001; Bixler et al., 2009). Therefore clinical research and practice need to focus more on sleep disturbances in children. The current study focuses on the effect of childhood sleep-disordered breathing (SDB) on declarative and non-declarative memory consolidation.
Memory consolidation can be defined as a set of processes whereby the newly acquired and initially labile memory traces become more stable with the passage of time (Stickgold and Walker, 2007; Spencer, 2013; Urbain et al., 2013). Growing body of evidence indicates that sleep plays a crucial role in these consolidation mechanisms and leads to memory representation being more resistant to interference and forgetting (Dorfberger et al., 2007; Diekelmann et al., 2009; Rudoy et al., 2009; Diekelmann and Born, 2010; Diekelmann, 2014; Mednick et al., 2011; Born and Wilhelm, 2012; Schönauer et al., 2014, 2015).
The effect of sleep on declarative (e.g., remembering events or facts) and non-declarative/procedural memory (e.g., learning languages, learning to musical instruments and movement-based sports) domains is well explored in healthy adults (Fischer et al., 2002; Walker et al., 2002; Gais and Born, 2004; Gais et al., 2006; Song et al., 2007; Rickard et al., 2008; Nemeth et al., 2010), but only a few studies focused on children. These studies with typically developing children found that post-training sleep facilitates the consolidation of declarative memory processes (Gais et al., 2006; Backhaus et al., 2008; Wilhelm et al., 2008; Prehn-Kristensen et al., 2009) but the effect of sleep on non-declarative memory consolidation is still controversial. Some studies failed to find a facilitating effect of sleep on non-declarative memory consolidation (Wilhelm et al., 2008; Prehn-Kristensen et al., 2009), however some recent studies revealed that sleep impacts on non-declarative/procedural memory in children (Fischer et al., 2007; Wilhelm et al., 2012, 2013; Urbain et al., 2013). In contrast to these results, Fischer et al. (2007) demonstrated offline decrement after sleep in non-declarative memory in children compared to adults who showed offline improvement after sleep. In a recent study Borragán et al. (2015) clarified the picture by showing that sleep has a beneficial effect on the consolidation of motor skills but it has no influence on sequential skills. These results indicate that sleep-dependent non-declarative memory consolidation can depend on age (Fischer et al., 2007; Wilhelm et al., 2008) and the nature of the task (Wilhelm et al., 2008, 2012; Borragán et al., 2015). Less is known about how permanent sleep-disorder influences sleep-dependent consolidation of declarative and non-declarative memories in children.
In our study we examined children with SDB which is an ideal population to investigate the effects of sleep disorder on the consolidation of different memory systems. SDB is a spectrum disorder characterized by prolonged and intermittent partial (such as snoring) or complete upper airway obstruction (such as Obstructive sleep apnea, OSA) that disturbs normal ventilation and sleep pattern during sleep. Especially slow wave sleep and REM sleep are affected in SDB (Coleman, 2003; Li and Lee, 2009; Sinha and Guilleminault, 2010). OSA is the worst grade on this spectrum characterized by repetitive episodes of complete or partial upper airway obstruction during sleep resulting hypoxia and fragmented sleep (Banno and Kryger, 2007). The main cases of SDB in children is with adenotonsillar hypertrophy, but it also occurs with obesity, upper airway narrowing due to craniofacial or neuromuscular abnormalities or muscular coordination (Arens et al., 2001; Guimaraes et al., 2008; Katz and D’Ambrosio, 2008).
The neurocognitive consequences of SDB in children have not yet been fully evaluated. There is emerging evidence that cognitive deficits are most consistently apparent on tasks involving sustained attention and executive functions (Beebe and Gozal, 2002; Archbold et al., 2004; O’Brien et al., 2004b; Beebe, 2006). In addition, SDB is associated with deterioration of memory; for example, Gottlieb et al. (2004) revealed that children with SDB had significantly poorer performance on verbal (Narrative Memory) and visual memory tasks (Memory for Faces) compared to healthy participants. Kheirandish-Gozal et al. (2010) investigated the learning before sleep (acquisition) and delayed free recall performance after an overnight sleep (retention) in children with OSA compared with children without sleep disorder. They used pictorial-based memory task where the subjects required to learn and remember animal pictures. They found that both immediate (before sleep) and delayed recall performances (after sleep) were worse among OSA children compared to the control subjects. The authors suggested that this reduced performance may be caused by impaired ability to use adequate learning strategies which either leads to difficulties to learn new information or children with OSA suffer from impaired encoding or altered retrieval. In our recent study (Csábi et al., 2013), we investigated declarative and non-declarative memory performance in one learning session (without consolidation) and showed weaker declarative but intact non-declarative memory performance in children with SDB compared to the controls. These results suggest that the more attention-demanding declarative learning are more vulnerable to permanent sleep disorder than less attention demanding non-declarative learning.
The mechanisms causing these neuropsychological deficits have not been fully delineated. Previous studies suggest that the developing central nervous system in children may be relatively more vulnerable to the fragmented sleep and hypoxia, particularly the hippocampus and frontal lobe structures (Macey et al., 2002; Morrell et al., 2003; Bartlett et al., 2004; Halbower et al., 2006; Owens, 2009). Children with SDB can exhibit daytime behavioral regulation problems (such as inattention, hyperactivity, aggressiveness, social withdrawal) which might also imply frontal lobe dysfunction (Chervin and Archbold, 2001; Beebe and Gozal, 2002; Archbold et al., 2004; Archbold, 2006).
Previous studies examined memory encoding and consolidation before and after sleep in patients with sleep apnea in adults, and showed that declarative and some aspects of non-declarative memory performance is affected in patients with OSA (Kloepfer et al., 2009; Djonlagic et al., 2012; Csabi et al., 2014). Similarly to Borragán et al. (2015) we found dissociation in the effect of sleep (and/or sleep disorder) on offline changes of general motor skills and sequence-specific learning: adult OSA patients showed impaired consolidation of general motor but not on sequence-specific learning (Csabi et al., 2014). To our knowledge, the current study is the first to assess the effects of sleep disorder on declarative and non-declarative memory functions before and after a nighttime sleep in children. Based on previous studies, we hypothesized that SDB in childhood has an adverse effect on the consolidation of declarative memory while it has less influence on non-declarative memory consolidation. Within the later one we expect differences in the consolidation of motor and sequence-specific aspects of the offline changes.
Thirty two children participated in the experiment. Breathing events during sleep, Body Mass Index (BMI) and working memory (WM) measures of the SDB patients and healthy participants are listed in Table 1. All participants underwent an overnight polygraphy, which was performed with the Somnomedics Somnoscreen plus device (Randersacker, Germany) at the Sleep Disorders Laboratory of Heim Pál Children’s Hospital, Budapest, Hungary. Patients who met the International Classification of Sleep Disorders criteria’s (American Academy of Sleep Medicine, 2001) for SDB were included in the study. SDB was diagnosed by a board-certified sleep physician. The SDB group consisted of sixteen children with SDB (average age: 8.56 years [min: 6 to max: 11 years], SD: 2.31; 6 females/10 males) six of them with OSA and ten of them with primary snoring. The Apnea/Hypopnea (AHI) index of the OSA patients (M = 17.32, SD = 30.54, range 2–79) was significantly higher (all p’s < 0.01) than that of the snoring patients (M = 0.11, SD = 0.19, range 0–1) as well as the controls (M = 0.11, SD = 0.20, range 0–1). Similarly, the snore index of the snoring patients (M = 55.10, SD = 54.95, range 6–155) was significantly higher (all p’s < 0.03) than that of the OSA patients (M = 16.67, SD = 28.52, range 0–73) as well as the controls (M = 0.13, SD = 0.34, range 0–1). According to the literature, the neurobehavioral deficits is associated with snoring in children are similar to those found in children with OSA (Gozal and O’Brien, 2004; O’Brien et al., 2004a). Therefore we compared the performance of the SDB group to that of controls and did not intend to examine the OSA and snoring subgroups separately. All SDB patients were untreated prior to and during the experimental night in the sleep laboratory.
The control group consisted of sixteen healthy participants (average age: 8.75 years, SD: 1.44 [min: 6 to max: 15 years]; 8 females/8 males). The control and the patient groups were matched on age (t(30) = 0.28, p = 0.78) and gender ( = 0.51, p = 0.48) and parental education (mother education: t(12.54) < 0.001, p > 0.99; father education t(23) = 0.61, p = 0.55). They did not suffer from any developmental, psychiatric or neurological disorders, and were free of any sleeping disorders. Informed written parental consent and verbal assent of the children were provided, and participants did not receive any financial compensation for their participation. Ethics approval was obtained by the Ethics Committee at Heim Pal Children’s Hospital, Budapest.
Declarative memory performance was measured by “The War of the Ghosts” test (Bartlett, 1932; Bergman and Roediger, 1999). This is a story recall test, which is widely used to measure declarative memory for episodes (Bartlett, 1932; Bergman and Roediger, 1999; Andreano and Cahill, 2006, 2008; Schwabe and Wolf, 2009; Hardt et al., 2010). In this test children are asked to listen and repeat the story after various intervals (immediately or after a determinate interval). The story consisted of 36 sentences; based on the standardized scoring, each sentence is allocated 1 point for the verbatim recalled sentences and 0.5 points for partly correct responses (gist of the sentences; Bartlett, 1932; Gauld and Stephenson, 1967; Csábi et al., 2013).
We used a modified version of the original ASRT task in order to assess non-declarative/procedural learning performance. In the original version of this task, four open circles were displayed in the middle of the computer screen and subjects had to press the corresponding button when the circles were filled in with black (Howard and Howard, 1997). In our version, a dog’s head appeared in one of the four empty circles on the screen and participants had to press the corresponding button (Nemeth et al., 2010). The computer was equipped with a special keyboard with four marked keys (Y, C, B and M on a QWERTZ keyboard; thus, compared to the English keyboard layout, the location of the buttons Z and Y were switched), each corresponding to one of the horizontally aligned circles. Before beginning the task, detailed instructions were read to the participants. We emphasized that the aim was to try to respond as quickly and as correctly as possible. Session 1 (Learning Phase) consisted of 25 blocks, with 85 key presses in each block—the first five stimuli were random for practice purposes, then an eight-element alternating sequence (e.g., 2r1r4r3r, where numbers represent the four places on the screen, and r represents an event randomly selected from the four possible places) repeated ten times. This sequence structure is often described as non-adjacent second-order dependency (Remillard, 2008). Similarly to earlier studies (Nemeth et al., 2010), stimuli were presented 120 ms after the previous response (response-to-stimulus interval, RSI). Each block required about 1.5 min and the entire session took approximately 30–40 min. Between blocks, participants received feedback about their overall RT and accuracy on the screen and then rested 10–20 s before starting a new block. Session 2 (Testing Phase) consisted of 5 blocks; the number of key presses and the RSI were the same as in Session 1 and this Testing Phase took approximately 5–10 min to complete.
A different ASRT sequence was selected for each participant based on a permutation rule so that each of the six unique permutations of the four repeating events occurred. Consequently, six different sequences were used across participants.
As there is a fixed sequence in the ASRT task alternating with random stimuli (for instance 2r1r4r3r), some triplets or runs of three stimuli occur more frequently than others. For example, in the above illustration, triplets 2_1, 1_4, 4_3, and 3_2 would occur often because the third element could be derived from the sequence or could also be a random element. In contrast, 1_2 or 4_1 would occur less frequently because in this case the third element could only be random. Following previous studies (Howard and Howard, 1997; Song et al., 2007; Nemeth et al., 2010), we refer to the former as high-frequency triplets and the latter as low-frequency triplets. Out of the 64 possible triplets, the 16 high-frequency triplets occurred 62.5% of the time and the 48 low-frequency triplets occurred 37.5% of the time. Note that the final event of high-frequency triplets is therefore more predictable from the initial event compared to the low-frequency triplets.
Previous studies have shown that as people practice the ASRT task, they come to respond more quickly to the high- than low-frequency triplets, revealing sequence-specific learning (Howard and Howard, 1997; Howard et al., 2004; Song et al., 2007; Nemeth et al., 2010; Janacsek et al., 2012). In addition, general motor skill learning is revealed in the ASRT task by the overall speed-up due to practice, irrespective of the triplet types. Thus, using the ASRT task enables to measure both sequence-specific and general motor skill learning.
There were two sessions in the experiment. The declarative and non-declarative performance was assessed at 7–9 PM prior to sleep (Learning Phase/Session 1) and 7–9 AM after sleep (Testing Phase/Session 2), thus the average interval between the Learning and Testing Phase was 12 h. The order of the administration of declarative and non-declarative tasks was counterbalanced in order to minimize the interference between declarative and non-declarative tasks (see Brown and Robertson, 2007).
To facilitate data processing, the blocks of ASRT were organized into epochs of five blocks. The first epoch contained blocks 1–5, the second epoch contained blocks 6–10, etc. We calculated mean accuracy and median RT for correct responses only; separate for high- and low-frequency triplets and for each subject and each epoch. Note that for each response (n), we defined whether it was a high- or a low-frequency triplet by considering whether it was more or less predictable from the event n-2. For the analyses reported below, as in previous research (Howard and Howard, 1997; Song et al., 2007; Nemeth et al., 2010), two kinds of low frequency triplets were eliminated: repetitions (e.g., 222, 333) and trills (e.g., 212, 343). Repetitions and trills were low frequency for all participants and people often showed pre-existing response tendencies to them (Howard and Howard, 1997; Howard et al., 2004). By eliminating them we attempted to ensure that any high- vs. low-frequency differences are due to learning and not to pre-existing tendencies.
We conducted a mixed design ANOVA with SESSION (1–2) as a within-subject factor and GROUP (SDB vs. control) as a between-subject factor to assess offline changes in declarative memory performance. The main effect of GROUP was significant (F(1,29) = 6.155, = 0.175, p = 0.019), indicating weaker story recall performance in the SDB compared to the controls (6.267 vs. 10.406, respectively). This weaker performance of the SDB group compared to the control group was evident both in Session 1 (6.87 vs. 10.38; p = 0.03) and in Session 2 (5.67 vs. 10.44; p = 0.01; Figure 1).
Figure 1. Declarative memory performance in the evening and in the morning in the SDB and control groups. The dependent variable was the number of correctly recalled sentences. The overall declarative memory performance of the SDB group was significantly lower compared to the control group, but there were no offline changes in the memory performance in either group. Error bars indicate SEM.
The main effect of SESSION failed to reach significance (F(1,29) = 2.05, = 0.06, p = 0.16), suggesting no change in the performance during the offline period. Similarly, the SESSION × GROUP interaction was not significant either (F(1,29) = 2.53, = 0.08, p = 0.12), suggesting no differences in offline changes between the SDB and control groups.
A mixed design ANOVA was conducted on the 5 epochs of the data shown in Figure 2 with TRIPLET (2: high vs. low) and EPOCH (1–5) as within-subjects factors and GROUP (SDB vs. control) as a between-subjects factor.
Figure 2. Results of sequence-specific and general skill learning in the SDB (A) and control group (B) in Session 1 (Epoch 1–5) and Session 2 (Epoch 6) on accuracy measures. Both groups showed significant sequence-specific and general skill learning. There were no differences in learning and in offline changes between the groups; the pattern of learning was similar in the SDB and control groups. Error bars indicate SEM.
There was significant sequence-specific learning (indicated by the significant main effect of TRIPLET: F(1,30) = 61.26, = 0.67, p < 0.001), such that accuracy was greater on high- than on low-frequency triplets. SDB and control groups showed no differences in sequence-specific learning (TRIPLET × GROUP interaction: F(1,30) = 0.29, = 0.01, p = 0.59).
The main effect of EPOCH did not reach significance (F(4,120) = 2.58, = 0.07, p = 0.06), although accuracy decreased across epochs on a trend level. SDB and control groups performed at the same level (EPOCH × GROUP interaction: F(4,120) = 1.29, = 0.04, p = 0.28).
The TRIPLET × EPOCH interaction was significant (F(4,120) = 3.37, = 0.10, p = 0.01), but there were no significant differences between the groups (indicating by the TRIPLET × EPOCH × GROUP interaction F(4,120) = 0.41, = 0.01, p = 0.79; respectively), demonstrating that the pattern of learning was similar in the groups. The main effect of GROUP did not reach significance (F(1,30) = 3.91, = 0.11, p = 0.06), although the SDB group had lower accuracy on a trend level (SDB group: 88.6%, control group: 91.8%).
To investigate the offline changes of sequence-specific and general motor skill learning we compared the accuracy from the last epoch of Session 1 (Epoch 5) and the epoch of Session 2 (Epoch 6) in both groups. These variables were submitted to a mixed design ANOVA with TRIPLET (2: high- vs. low-frequency) and EPOCH (2: last epoch of Session 1 and epoch of Session 2) as within-subject factors, and GROUP (SDB vs. control) as a between-subject factor. The data is shown in Figure 2.
There was significant sequence-specific learning (indicating by the main effect of TRIPLET; F(1,30) = 95.40, = 0.76, p < 0.001), such that accuracy was greater on high- than on low-frequency triplets. It was similar in the SDB and control groups (indicated by the non-significant TRIPLET × GROUP interaction: F(1,30) = 0.04, = 0.002, p = 0.82).
There was a significant offline changes of general motor skills (indicating by the main effect of EPOCH; F(1,30) = 13.40, = 0.30, p = 0.01), thus accuracy increased from evening to morning. SDB and control groups performed at the same level (EPOCH × GROUP interaction: F(1,30) = 3.26, = 0.09, p = 0.08).
The TRIPLET × EPOCH and TRIPLET × EPOCH × GROUP interactions were not significant (F(1,30) = 0.20, = 0.01, p = 0.65; F(1,30) = 0.28, = 0.01, p = 0.59; respectively), indicating that the pattern of sequence-specific learning was similar in the groups. The main effect of GROUP was not significant (F(1,30) = 1.31, = 0.04, p = 0.26), reflecting that all groups responded with similar accuracy rates (SDB group: 88.8%, control group: 91.2%).
To investigate learning during Session 1, a mixed design ANOVA was conducted on the first 5 epochs of the data shown in Figure 3, with TRIPLET (2: high- vs. low-frequency) and EPOCH (5: 1–5) as within-subject factors, and GROUP (SDB vs. control) as a between-subject factor.
Figure 3. Results of sequence-specific and general skill learning in the SDB (A) and control (B) group in Session 1 (Epoch 1–5) and Session 2 (Epoch 6) on reaction time measures. Both groups showed significant sequence-specific and general skill learning. There were no differences in learning and in offline changes between the groups; the pattern of learning was similar in the SDB and control groups. Error bars indicate SEM.
Our data revealed significant sequence-specific learning (indicated by the significant main effect of TRIPLET: F(1,30) = 64.33, = 0.68, p < 0.001), such that RTs were faster on high- than on low-frequency triplets. SDB and control groups showed no differences in sequence-specific learning (TRIPLET × GROUP interaction: F(1,30) = 0.59, = 0.04, p = 0.44).
There was also significant general motor skill learning (shown by the significant main effect of EPOCH: F(4,120) = 54.80, = 0.64, p < 0.001), such that RTs deceased across epochs. SDB and control groups performed at the same level (EPOCH × GROUP interaction: F(4,120) = 0.95, = 0.03, p = 0.38).
The TRIPLET × EPOCH interaction was significant (F(4,120) = 5.26, = 0.14, p = 0.003), suggesting that sequence-specific knowledge increased during practice. The TRIPLET × EPOCH × GROUP interaction was not significant F(4,120) = 0.49, = 0.013, p = 0.67), indicating that the pattern of learning was similar in the groups. In overall RT both group performed at the same level (main effect of GROUP: F(1,30) = 1.37, = 0.04, p = 0.25).
To investigate the offline changes of sequence-specific and general motor skill learning we compared the RTs from the last epoch of Session 1 (Epoch 5) and the epoch of Session 2 (Epoch 6) in both groups. These variables were submitted to a mixed design ANOVA with TRIPLET (2: high- vs. low-frequency) and EPOCH (2: last epoch of Session 1 and epoch of Session 2) as within-subject factors, and GROUP (SDB vs. control) as a between-subject factor. The data is shown on Figure 3.
There was significant sequence-specific learning (indicating by the main effect of TRIPLET; F(1,30) = 125.76, = 0.80, p < 0.001), thus RTs were faster on high- than low-frequency triplets when analysing the two epochs together. The groups did not differ in overall sequence-specific learning (indicated by the non-significant TRIPLET × GROUP interaction: F(1,30) = 0.42, = 0.01, p = 0.51).
There was significant general motor skill learning during the offline period (demonstrated by the main effect of EPOCH: F(1,30) = 20.71, = 0.40, p < 0.001), such that RTs were faster in the morning compared to the evening. The SDB and control groups showed similar level of offline general motor skill learning (EPOCH × GROUP interaction: F(1,30) = 0.24, = 0.01, p = 0.62).
The TRIPLET × EPOCH and the TRIPLET × EPOCH × GROUP interactions were not significant (F(1,30) = 0.84, = 0.02, p = 0.36; F(1,30) = 2.18, = 0.06, p = 0.15, respectively), indicating that the SDB and the control group demonstrated no differences in the pattern of offline changes. There were no significant differences in the overall RTs between the SDB and control groups (main effect of GROUP: F(1,30) = 2.54, = 0.07, p = 0.12).
Our goal was to investigate the consolidation of declarative and non-declarative memory in children with SDB. We believe our study to be the first to investigate the offline changes of these two types of memory processes in children with sleep disorder. We found no group difference in the consolidation of declarative memory; the SDB group, however, showed generally weaker memory performance in both sessions. We used the ASRT task to measure non-declarative learning processes. This sequence learning task allowed us to differentiate between two components of learning: general motor skill learning and sequence-specific learning. We found that these two types of non-declarative learning and consolidation are intact in children with SDB.
Our results on online declarative memory performance are in line with previous studies that found weaker declarative performance in the SDB group in general (Blunden et al., 2000; Kaemingk et al., 2003; Gottlieb et al., 2004; Kennedy et al., 2004; Csábi et al., 2013). Gottlieb et al. (2004) found lower performance on verbal and visual memory tasks in children with SDB compared to healthy controls. The mechanism causing these neuropsychological deficits has not been fully explored. Results from previous studies suggest that sleep fragmentation and intermittent hypoxia could have negative influence on the development of the central nervous system resulting structural changes in brain circuits, particularly in the hippocampus and frontal lobe (Macey et al., 2002; Bartlett et al., 2004; Halbower et al., 2006; Owens, 2009). For example Bartlett et al. (2004) found that in the left hippocampal area, N-acetyl-containing/creatine-containing compounds was significantly increased in adult OSA patients using proton magnetic resonance spectroscopic imaging. In childhood OSA Halbower et al. (2006) showed also significant differences in the mean metabolite ratio N-acetyl in the left hippocampus and right frontal cortex compared to controls leading the conclusion that childhood OSA is associated with neuronal injury in the hippocampus and frontal cortex. It is important to note that we assessed only the breathing indices during sleep. Further investigations using polysomnography need to clarify the relationship between declarative memory functions and sleep stages or sleep deprivation in children with SDB.
In the case of the overnight consolidation of declarative memory, we failed to find differences between the SDB and control group. Although there was a general group difference in the overall performance, both groups showed intact consolidation. This result contradicts with the finding of Kheirandish-Gozal et al. (2010) who demonstrated decreased consolidation of declarative memory in children with OSA. The difference between the two studies might be explained by the type of materials to be remembered (verbal vs. nonverbal) and other task characteristics (e.g., number of repetitions). Another possible explanation might be that the SDB group in our study demonstrated a floor effect with no room to forget in the offline period. For example, compared to the healthy controls, sleep disturbances in the SDB group can lead to a greater fatigue effect, which can be more pronounced by the evening where the first session took place, and could lead to weaker memory performance in the SDB group. This explanation can be tested by controlling for circadian effects and comparing AM-PM vs. PM-AM designs. Future studies need to unravel how task characteristics and/or circadian factors affect sleep-related declarative memory consolidation in children.
In the case of non-declarative learning, we found similar performance between the SDB and control group in general motor skill and sequence-specific learning in the Learning Phase, both in accuracy and in RT. Our results are in line with our previous study in which the SDB group showed impaired declarative memory performance while the non-declarative learning remained intact compared to the healthy controls (Csábi et al., 2013). Nemeth et al. (2012) using the ASRT task also found intact non-declarative sequence learning in elderly adults with OSA. These results indicate that the relationship between online non-declarative memory formation and sleep is similar in children and adults with SDB. The performance difference between declarative and non-declarative tasks in session one can be explained by that the disrupted sleep pattern influences the more attention-demanding and cortical structure-guided explicit processes (story recall), while the less attention-demanding implicit processes (ASRT task) mediated by subcortical structures are preserved (Csábi et al., 2013).
In the overnight consolidation of non-declarative memory we found no differences in the offline changes of either general motor skill or sequence-specific learning between the two groups. We found offline improvement on general motor skill, while the sequence-specific learning remained on the same level and did not improved. To our knowledge, consolidation of non-declarative memory has not been tested in children with SDB yet. These results are in line with studies investigating the effect of sleep deprivation on non-declarative sequence learning in adults without sleep disorder (Genzel et al., 2009; Van Der Werf et al., 2011). There are a few studies investigating non-declarative memory consolidation in adults with OSA. For example, Kloepfer et al. (2009) found reduced overnight improvement on average RT performance in OSA patients using a very different task compared to ours (motor adaptation vs. sequence learning, respectively). Djonlagic et al. (2012) also examined adult OSA population and revealed that OSA and control groups showed almost identical performance in the initial training in the evening on a sequence learning task, but the control group exhibited significantly more overnight improvement. The authors suggest that this weaker offline performance was caused by sleep fragmentation in OSA. In our previous study with adult OSA patients, we revealed differences in the offline changes of general motor skill learning between the OSA and control group. The control group showed offline improvement on general motor skill learning from evening to morning, while the OSA group did not. In contrast, we did not find differences between the groups in offline changes in sequence-specific learning (Csabi et al., 2014). These results partly differ from our current findings and highlight the importance of developmental factors in the consolidation of non-declarative memory: sleep disordered breathing might affect the underlying neural network differently in childhood compared to adulthood.
It worth mentioning that our study have two important potential limitations. Firstly, the declarative and non-declarative tasks could be interfere to each other. For example Brown and Robertson (2007) found that declarative tasks can actually boost non-declarative learning. It is possible that our manipulation namely counterbalancing these two types of task is not enough to eliminate the interference. Secondly, it is possible that the actual story recall task is not sensitive enough to demonstrate sleep effect. Further studies need to clarify these issues by examining the declarative and non-declarative tasks separately in different experiments and using other type of declarative tasks as well.
In conclusion, our study found dissociation between the declarative and non-declarative processes in children with SDB. Similarly with Csábi et al. (2013) we found weaker declarative memory than non-declarative performance in the first Session (Learning Phase). Regarding the consolidation, we found intact consolidation in the case of declarative memory as well as sequence-specific and general motor skill aspects of non-declarative memory in SDB. These findings imply that actual and/or long-term disturbance of sleep has a differential effect on different memory processes (online vs. offline). Our findings underscore the importance of examining the effect of sleep disturbances on motor and cognitive functions in childhood. These studies can give us a deeper insight into the effect of sleep on the developing brain and memory functions and how the relationship between sleep and memory changes from childhood to adulthood. Since persistent sleep problems in childhood can lead not only to impaired cognitive functioning—consequently lower general intelligence and school performance—but also anxiety and depression disorders in adulthood (Gregory et al., 2005), these results can help us develop more sophisticated diagnostic tools, neuropsychological profile and more effective rehabilitation programs.
The authors declare that the research was conducted in the absence of any commercial or financial relationships that could be construed as a potential conflict of interest.
We are thankful to the nurses of the Sleep Center of Heim Pal Children’s Hospital for their work and assistance. This study was supported by Hungarian Brain Research Program: KTIA NAP Grant (ID: 13-2-2014-0020) and Janos Bolyai Research Fellowship of the Hungarian Academy of Sciences (KJ).
American Academy of Sleep Medicine. (2001). International Classification of Sleep Disorders, Revised: Diagnostic and Coding Manual. Chicago, IL: American Academy of Sleep Medicine.
Andreano, J. M., and Cahill, L. (2006). Glucocorticoid release and memory consolidation in men and woman. Psychol. Sci. 17, 466–470. doi: 10.1111/j.1467-9280.2006.01729.x
Andreano, J. M., and Cahill, L. (2008). Menstrual cycle modulation of the relationship between cortisol and long-term memory. Psychoneuroendocrinology 33, 874–882. doi: 10.1016/j.psyneuen.2008.03.009
Anuntaseree, W., Rookkapan, K., Kuasirikul, S., and Thonsuksai, P. (2001). Snoring and obstructive sleep apnea in Thai school-aged children: prevalence and predisposing factors. Pediatr. Pulmonol. 32, 222–227. doi: 10.1002/ppul.1112
Archbold, K. H. (2006). Sleep disorders and attention-deficit hyperactivity disorder in children: a missing differential diagnosis. J. Am. Psychiatr. Nurses Assoc. 12, 216–224. doi: 10.1177/1078390306295087
Archbold, K. H., Giordani, B., Ruzicka, D. L., and Chervin, R. D. (2004). Cognitive executive dysfunction in children with mild sleep-disordered breathing. Biol. Res. Nurs. 5, 168–176. doi: 10.1177/1099800403260261
Arens, R., McDonough, J. M., Costarino, A. T., Mahboubi, S., Tayag-Kier, C. E., Maislin, G., et al. (2001). Magnetic resonance imaging of the upper airway structure of children with obstructive sleep apnea syndrome. Am. J. Respir. Crit. Care Med. 164, 698–703. doi: 10.1164/ajrccm.164.4.2101127
Backhaus, J., Hoeckesfeld, R., Hohagen, J. B., Born, J., and Junghanns, K. (2008). Immediate as well as delayed post learning sleep but not wakefulness enhances declarative memory consolidation in children. Neurobiol. Learn. Mem. 89, 76–80. doi: 10.1016/j.nlm.2007.08.010
Banno, K., and Kryger, H. M. (2007). Sleep apnea: clinical investigations in humans. Sleep Med. 8, 400–426. doi: 10.1016/j.sleep.2007.03.003
Bartlett, F. C. (1932). Remembering: An Experimental and Social Study. Cambridge: Cambridge University.
Bartlett, D. J., Rae, C., Thompson, C. H., Byth, K., Joffe, D. A., Enright, T., et al. (2004). Hippocampal area metabolites relate to severity and cognitive function in obstructive sleep apnea. Sleep Med. 5, 593–596. doi: 10.1016/j.sleep.2004.08.004
Beebe, D. W. (2006). Neurobehavioral morbidity associated with disordered breathing during sleep in children: a comprehensive review. Sleep 29, 1115–1134.
Beebe, D. W., and Gozal, D. (2002). Obstructive sleep apnea and the prefrontal cortex: towards a comprehensive model linking nocturnal upper airway obstruction to daytime cognitive and behavioral deficits. J. Sleep Res. 11, 1–16. doi: 10.1046/j.1365-2869.2002.00289.x
Bergman, E. T., and Roediger, H. L. (1999). Can Bartlett’s repeated reproduction experiments be replicated? Mem. Cognit. 27, 937–947. doi: 10.3758/bf03201224
Bixler, E. O., Vgontzas, A. N., Lin, H. M., Liao, D., Calhoun, S., Vela-Bueno, A., et al. (2009). Sleep disorder breathing in children in a general population sample: prevalence and risk factors. Sleep 32, 731–736.
Blunden, S., Lushington, K., Kennedy, D., Martin, J., and Dawson, D. (2000). Behavior and neurocognitive performance in children aged 5–10 years who snore compared to controls. J. Clin. Exp. Neuropsychol. 22, 554–568. doi: 10.1076/1380-3395(200010)22:5;1-9;ft554
Born, J., and Wilhelm, I. (2012). System consolidation of memory during sleep. Psychol. Res. 76, 192–203. doi: 10.1007/s00426-011-0335-6
Borragán, G., Urbain, C., Schmitz, R., Mary, A., and Peigneux, P. (2015). Sleep and memory consolidation: motor performance and proactive interference effects in sequence learning. Brain Cogn. 95, 54–61. doi: 10.1016/j.bandc.2015.01.011
Brown, R. M., and Robertson, E. M. (2007). Off-line processing: reciprocal interactions between declarative and procedural memories. J. Neurosci. 27, 10468–10475. doi: 10.1523/jneurosci.2799-07.2007
Brunetti, L., Rana, S., Lospalutti, M. L., Pietrafesa, A., Francavilla, R., Fanelli, M., et al. (2001). Prevalence of obstructive sleep apnea syndrome in a cohort of 1,207 children on southern Italy. Chest 120, 1930–1935. doi: 10.1378/chest.120.6.1930
Case, R., Kurland, D. M., and Goldberg, J. (1982). Operational efficiency and the growth of short-term memory span. J. Exp. Child. Psychol. 33, 386–404. doi: 10.1016/0022-0965(82)90054-6
Chervin, R. D., and Archbold, K. H. (2001). Hyperactivity and polisomnographic findings in children evaluated for sleep disordered breathing. Sleep 24, 313–320.
Coleman, J. (2003). “Pathophysiology of snoring and obstructive sleep apnea: airway dynamics,” in Snoring and Obstructive Sleep Apnea, eds D. N. F. Fairbanks, S. A. Mickelson, and B. T. Woodson (Philadelphia, PA: Williams and Wilkins Co.), 19–24.
Csábi, E., Benedek, P., Janacsek, K., Katona, G., and Nemeth, D. (2013). Sleep-disorder in childhood impaired declarative but not non-declarative forms of learning. J. Clin. Exp. Neuropsychol. 35, 677–685. doi: 10.1080/13803395.2013.815693
Csabi, E., Varszegi-Schulz, M., Janacsek, K., Malacek, N., and Nemeth, D. (2014). The consolidation of implicit sequence memory in obstructive sleep apnea. PLoS One 9:e109010. doi: 10.1371/journal.pone.0109010
Daneman, M., and Blennerhassett, A. (1984). How to assess the listening comprehension skills of prereaders. J. Educ. Psychol. 76, 1372–1381. doi: 10.1037//0022-0663.76.6.1372
Diekelmann, S. (2014). Sleep for cognitive enhancement. Front. Syst. Neurosci. 8:46. doi: 10.3389/fnsys.2014.00046
Diekelmann, S., and Born, J. (2010). The memory function of sleep. Nat. Rev. Neurosci. 11, 114–126. doi: 10.1038/nrn2762
Diekelmann, S., Wilhelm, I., and Born, J. (2009). The whats and whens of sleep-dependent memory consolidation. Sleep Med. Rev. 13, 309–321. doi: 10.1016/j.smrv.2008.08.002
Djonlagic, I., Saboisky, J., Carusona, A., Stickgold, R., and Malhotra, A. (2012). Increased sleep fragmentation leads to impaired offline consolidation of motor memories in humans. PLoS One 7:e34106. doi: 10.1371/journal.pone.0034106
Dorfberger, S., Adi-Japha, E., and Karni, A. (2007). Reduced susceptibility to interference in the consolidation of motor memory before adolescence. PLoS One 2:e240. doi: 10.1371/journal.pone.0000240
Fischer, S., Hallschmid, M., Elsner, A. L., and Born, J. (2002). Sleep forms memory for finger skills. Proc. Natl. Acad. Sci. U S A 99, 11987–11991. doi: 10.1073/pnas.182178199
Fischer, S., Wilhelm, I., and Born, J. (2007). Developmental differences in sleep’s role for implicit off-line learning: comparing children with adults. J. Cogn. Neurosci. 19, 214–227. doi: 10.1162/jocn.2007.19.2.214
Gais, S., and Born, J. (2004). Declarative memory consolidation: mechanisms acting during human sleep. Learn. Mem. 11, 679–685. doi: 10.1101/lm.80504
Gais, S., Lucas, B., and Born, J. (2006). Sleep after learning aids memory recall. Learn. Mem. 13, 259–262. doi: 10.1101/lm.132106
Gauld, A., and Stephenson, G. M. (1967). Some experiments relating to Bartlett’s theory of remembering. Br. J. Psychol. 58, 39–49. doi: 10.1111/j.2044-8295.1967.tb01054.x
Genzel, L., Dresler, M., Wehrle, R., Grözinger, M., and Steiger, A. (2009). Slow wave sleep and REM sleep awakenings do not affect sleep dependent consolidation memory consolidation. Sleep 32, 302–310.
Gottlieb, D. J., Chase, C., Vezina, R. M., Heeren, T. C., Corwin, M. J., Auerbach, S. H., et al. (2004). Sleep-disordered breathing symptoms are associated with poorer cognitive function in 5-year-old children. J. Pediatr. 145, 458–464. doi: 10.1016/j.jpeds.2004.05.039
Gozal, D., and O’Brien, L. M. (2004). Snoring and obstructive sleep apnea in children: why should we treat? Paediatr. Respir. Rev. 5, S371–S376. doi: 10.1016/s1526-0542(04)90066-8
Gregory, A. M., Caspi, A., Eley, T. C., Moffit, T. E., O’ Connor, T. G., and Poulton, R. (2005). Prospective longitudinal association between persistent sleep problems in childhood and anxiety and depression disorders in adulthood. J. Abnorm. Child Psychol. 33, 157–166. doi: 10.1007/s10802-005-1824-0
Guimaraes, C. V. A., Kalra, M., Donelly, L. F., Shott, S. R., Fitz, K., Singla, A., et al. (2008). The frequency of lingual tonsil enlargement in obese children. AJR Am. J. Roentgenol. 190, 973–975. doi: 10.2214/AJR.07.3020
Halbower, A. C., Degaonkar, M., Barker, P. B., Earley, C. J., Marcus, C. L., Smith, P. L., et al. (2006). Childhood obstructive sleep apnea associates with neuropsychological deficits and neuronal brain injury. PLoS Med. 3:e301. doi: 10.1371/journal.pmed.0030301
Hardt, O., Einarsson, E. Ö., and Nader, K. (2010). A bridge over troubled water: reconsolidation as a link between cognitive and neuroscientific memory research traditions. Annu. Rev. Psychol. 61, 141–167. doi: 10.1146/annurev.psych.093008.100455
Howard, J. H., and Howard, D. V. (1997). Age differences in implicit learning of higher order dependencies in serial patterns. Psychol. Aging 12, 634–656. doi: 10.1037/0882-7974.12.4.634
Howard, D. V., Howard, J. H., Jr., Japikse, K., DiYanni, C., Thompson, A., and Somberg, R. (2004). Implicit sequence learning: effects of level of structure, adult age and extended practice. Psychol. Aging 19, 79–92. doi: 10.1037/0882-7974.19.1.79
Janacsek, K., Fiser, J., and Nemeth, D. (2012). The best time to acquire new skills: age-related differences in implicit sequence learning across the human lifespan. Dev. Sci. 15, 496–505. doi: 10.1111/j.1467-7687.2012.01150.x
Kaemingk, K. L., Pavogel, A. E., Goodwin, J. L., Mulvaney, S. A., Martinez, F., Enright, P. L., et al. (2003). Learning in children and sleep disorder breathing: finding of the tucson Children’s Assessment of Sleep Apnea (tuCASA) prospective cohort study. J. Int. Neuropsychol. Soc. 9, 1016–1026. doi: 10.1017/s1355617703970056
Katz, E. S., and D’Ambrosio, C. M. (2008). Pathophysiology of pediatric obstructive sleep apnoea. Proc. Am. Thorac. Soc. 5, 253–262. doi: 10.1513/pats.200707-111MG.
Kennedy, J. D., Blunden, S., Hirte, C., Parsons, D. W., Martin, A. J., and Crowe, E. (2004). Reduced neurocognition in children who snore. Pediatr. Pulmonol. 37, 330–337. doi: 10.1002/ppul.10453
Kheirandish-Gozal, L., De Jong, M. R., Spruyt, K., Chamuleau, S. A. J., and Gozal, D. (2010). Obstructive sleep apnoea is associated with impaired pictorial memory task acquisition and retention in children. Eur. Res. J. 36, 164–169. doi: 10.1183/09031936.00114209
Kloepfer, C., Riemann, D., Nofzinger, E. A., Feige, B., Untereiner, J., O’Hara, R., et al. (2009). Memory before and after sleep in patient with moderate obstuctive sleep apnea. J. Clin. Sleep Med. 5, 540–548.
Li, H. Y., and Lee, L. A. (2009). Sleep-disorders breathing in children. Chang Gung Med. J. 32, 247–257.
Macey, P. M., Henderson, L. A., Macey, K. E., Alger, J. R., Frysinger, R. C., Woo, M. A., et al. (2002). Brain morphology associated with obstructive sleep apnea. Am. J. Respir. Crit. Care Med. 166, 1382–1387. doi: 10.1164/rccm.200201-050OC
Mednick, S. C., Cai, D. J., Schuman, T., Anagnostaras, S., and Wixted, J. T. (2011). An opportunistic theory of cellular and systems consolidation. Trends Neurosci. 34, 504–514. doi: 10.1016/j.tins.2011.06.003
Morrell, M. J., McRobbie, D. W., Quest, R. A., Cummin, A. R., Ghiassi, R., and Corfield, D. R. (2003). Changes in brain morphology associated with obstructive sleep apnea. Sleep Med. 4, 451–454. doi: 10.1016/s1389-9457(03)00159-x
Nemeth, D., Csábi, E., Janacsek, K., Várszegi, M., and Mari, Z. (2012). Intact probabilistic sequence learning in obstructive sleep apnea. J. Sleep Res. 21, 396–401. doi: 10.1111/j.1365-2869.2011.00983.x
Nemeth, D., Janacsek, K., Londe, Z., Ullman, M. T., Howard, D., and Howard, J., Jr. (2010). Sleep has no critical role in implicit motor sequence learning in young and old adults. Exp. Brain Res. 201, 351–358. doi: 10.1007/s00221-009-2024-x
O’Brien, L. M., Mervis, C. B., Holbrook, C. R., Bruner, J. L., Klaus, C. J., Rutherford, J., et al. (2004a). Neurobehavioral implications of habitual snoring in children. Pediatrics 114, 44–49. doi: 10.1542/peds.114.1.44
O’Brien, L. M., Mervis, C. B., Holbrook, C. R., Bruner, J. L., Smith, N. H., McNally, N., et al. (2004b). Neurobehavioral correlates of sleep-disordered breathing in children. J. Sleep Res. 13, 165–172. doi: 10.1111/j.1365-2869.2004.00395.x
Owens, J. A. (2009). Neurocognitive and behavioral impact of sleep-disordered breathing in children. Pediatr. Pulmonol. 44, 417–422. doi: 10.1002/ppul.20981
Prehn-Kristensen, A., Göder, R., Chirobeja, S., Breßmann, I., Ferstl, R., and Baving, L. (2009). Sleep in children enhances preferentially emotional declarative but not procedural memories. J. Exp. Child Psychol. 104, 132–139. doi: 10.1016/j.jecp.2009.01.005
Remillard, G. (2008). Implicit learning of second-, third- and fourth-order adjacent and nonadjacent sequential dependencies. Q. J. Exp. Psychol. (Hove) 61, 400–424. doi: 10.1080/17470210701210999
Rickard, T. C., Cai, D. J., Rieth, C. A., Jones, J., and Ard, M. C. (2008). Sleep does not enhance motor sequence learning. J. Exp. Psychol. Learn. Mem. Cogn. 34, 834–842. doi: 10.1037/0278-7393.34.4.834
Rosen, C. L., Larkin, E. K., Kirchner, H. L., Emancipator, J. L., Bivins, S. F., Surovec, S. A., et al. (2003). Prevalence and risk factors for sleep.disordered breathing in 8- to 11-year old children: association with race and prematurity. J. Pediatr. 142, 383–389. doi: 10.1067/mpd.2003.28
Rudoy, J. D., Voss, J. L., Westerberg, C. E., and Paller, K. (2009). Strenghtening individual memories by reactivating them during sleep. Science 326:1079. doi: 10.1126/science.1179013
Schönauer, M., Geisler, T., and Gais, S. (2014). Strenghtening procedural memories by reactivation in sleep. J. Cogn. Sci. 26, 143–153. doi: 10.1162/jocn_a_00471
Schönauer, M., Grätsch, M., and Gais, S. (2015). Evidence for two distinct sleep-related long-term memory consolidation. Cortex 63, 68–78. doi: 10.1016/j.cortex.2014.08.005
Schwabe, L., and Wolf, O. T. (2009). New episodic learning interferes with the reconsolidation of autobiographical memories. PLoS One 4:e7519. doi: 10.1371/journal.pone.0007519
Sinha, D., and Guilleminault, C. (2010). Sleep disordered breathing in children. Int. J. Med. Res. 131, 311–320.
Song, S., Howard, J. H., Jr., and Howard, D. V. (2007). Sleep does not benefit probabilistic motor sequence learning. J. Neurosci. 27, 12475–12483. doi: 10.1523/jneurosci.2062-07.2007
Spencer, R. M. C. (2013). Neurophysiological basis of sleep’s function on memory and cognition. ISRN Physiol. 2013:619379. doi: 10.1007/978-1-4419-7436-5_2
Stickgold, R., and Walker, M. P. (2007). Sleep-dependent memory consolidation and reconsolidation. Sleep Med. 8, 331–343. doi: 10.1016/j.sleep.2007.03.011
Urbain, C., Galer, S., Van Bogaert, P., and Peigneux, P. (2013). Pathophysiology of sleep dependent memory consolidation processes in children. Int. J. Psychophysiol. 89, 273–283. doi: 10.1016/j.ijpsycho.2013.06.022
Van Der Werf, Y., Altena, E., Vis, J., Koene, T., and Van Someren, E. (2011). Reduction of nocturnal slow wave activity affects daytime vigilance lapses and memory encoding but not reaction time or implicit learning. Prog. Brain Res. 193, 245–255. doi: 10.1016/b978-0-444-53839-0.00016-8
Walker, M. P., Brakefield, T., Morgan, A., Hobson, J. A., and Stickgold, R. (2002). Practice make sleep perfect: sleep-dependent motor skill learning. Neuron 35, 205–211. doi: 10.1016/S0896-6273(02)00746-8
Wilhelm, I., Diekelmann, S., and Born, J. (2008). Sleep in children improves memory performance on declarative but not procedural tasks. Learn. Mem. 15, 373–377. doi: 10.1101/lm.803708
Wilhelm, I., Metzkow-Mészáros, M., Knapp, S., and Born, J. (2012). Sleep dependent consolidation of procedural motor memories in children and adults: the pre-sleep level of performance matters. Dev. Sci. 15, 506–515. doi: 10.1111/j.1467-7687.2012.01146.x
Keywords: sleep deprivation, memory consolidation, declarative memory, skill learning, sequence learning, sleep-disordered breathing (SDB), implicit learning
Citation: Csábi E, Benedek P, Janacsek K, Zavecz Z, Katona G and Nemeth D (2016) Declarative and Non-declarative Memory Consolidation in Children with Sleep Disorder. Front. Hum. Neurosci. 9:709. doi: 10.3389/fnhum.2015.00709
Received: 4 September 2015; Accepted: 18 December 2015;
Published: 11 January 2016.
Edited by:
Genevieve Albouy, KU Leuven, BelgiumReviewed by:
Charline Urbain, The Hospital for Sick Children, CanadaCopyright © 2016 Csábi, Benedek, Janacsek, Zavecz, Katona and Nemeth. This is an open-access article distributed under the terms of the Creative Commons Attribution License (CC BY). The use, distribution and reproduction in other forums is permitted, provided the original author(s) or licensor are credited and that the original publication in this journal is cited, in accordance with accepted academic practice. No use, distribution or reproduction is permitted which does not comply with these terms.
*Correspondence: Dezso Nemeth, bmVtZXRoZEBnbWFpbC5jb20=
† These authors have contributed equally to this work.
Disclaimer: All claims expressed in this article are solely those of the authors and do not necessarily represent those of their affiliated organizations, or those of the publisher, the editors and the reviewers. Any product that may be evaluated in this article or claim that may be made by its manufacturer is not guaranteed or endorsed by the publisher.
Research integrity at Frontiers
Learn more about the work of our research integrity team to safeguard the quality of each article we publish.