- 1Division of Functional and Restorative Neurosurgery and Division of Translational Neurosurgery, Department of Neurosurgery, Eberhard Karls University Tuebingen, Tuebingen, Germany
- 2Neuroprosthetics Research Group, Werner Reichardt Center for Integrative Neuroscience, Eberhard Karls University Tuebingen, Tuebingen, Germany
- 3Department of Empirical Inference, Max Planck Institute for Intelligent Systems, Tuebingen, Germany
- 4Department of Medical Physics, University Medical Center Utrecht, Utrecht University, Utrecht, Netherlands
- 5Department of Computer Engineering, Wilhelm-Schickard Institute for Computer Science, Eberhard Karls University Tuebingen, Tuebingen, Germany
- 6Institute of Medical Psychology and Behavioral Neurobiology, Eberhard Karls University Tuebingen, Tuebingen, Germany
- 7Institute of Psychology, University of Wuerzburg, Wuerzburg, Germany
- 8Research Group Human Media Interaction, Department of Electrical Engineering, Mathematics and Computer Science, University of Twente, Enschede, Netherlands
- 9Health and Quality of life Unit, Fatronik-Tecnalia, San Sebastian, Spain
- 10Istituto di Ricovero e Cura a Carattere Scientifico, IRCCS Ospedale San Camillo, Venezia, Italy
Electroencephalography (EEG) often fails to assess both the level (i.e., arousal) and the content (i.e., awareness) of pathologically altered consciousness in patients without motor responsiveness. This might be related to a decline of awareness, to episodes of low arousal and disturbed sleep patterns, and/or to distorting and attenuating effects of the skull and intermediate tissue on the recorded brain signals. Novel approaches are required to overcome these limitations. We introduced epidural electrocorticography (ECoG) for monitoring of cortical physiology in a late-stage amytrophic lateral sclerosis patient in completely locked-in state (CLIS). Despite long-term application for a period of six months, no implant-related complications occurred. Recordings from the left frontal cortex were sufficient to identify three arousal states. Spectral analysis of the intrinsic oscillatory activity enabled us to extract state-dependent dominant frequencies at <4, ~7 and ~20 Hz, representing sleep-like periods, and phases of low and elevated arousal, respectively. In the absence of other biomarkers, ECoG proved to be a reliable tool for monitoring circadian rhythmicity, i.e., avoiding interference with the patient when he was sleeping and exploiting time windows of responsiveness. Moreover, the effects of interventions addressing the patient’s arousal, e.g., amantadine medication, could be evaluated objectively on the basis of physiological markers, even in the absence of behavioral parameters. Epidural ECoG constitutes a feasible trade-off between surgical risk and quality of recorded brain signals to gain information on the patient’s present level of arousal. This approach enables us to optimize the timing of interactions and medical interventions, all of which should take place when the patient is in a phase of high arousal. Furthermore, avoiding low-responsiveness periods will facilitate measures to implement alternative communication pathways involving brain-computer interfaces (BCI).
Introduction
Assessing both the level (i.e., arousal) and the content (i.e., awareness) of pathologically altered consciousness in clinical environments is limited to evaluating patients’ motor responsiveness (Laureys et al., 2009). Neurodegenerative diseases or injuries to the central nerve system may paralyze the affected patients to such a degree that they lose any remaining ability to communicate by volitional muscle control, thereby impeding the assessment of the different dimensions of consciousness (Laureys et al., 2004).
In the case of amyotrophic lateral sclerosis (ALS), this disconnection from the environment progresses slowly. In the late stage of the disease, in which the patients are no longer able to move their body or to speak, this condition spans a transition from the locked-in state (LIS), with very limited remnants of voluntary movements such as muscle twitches or eye movements, to the completely locked-in state (CLIS), with the loss of all motor control (Kübler and Birbaumer, 2008).
This is paralleled by a decline of other physiological measures for communication, including sphincter and facial electromyography as well as oculography (Murguialday et al., 2011). Similarly, body signals mediated by the parasympathetic and sympathetic nervous system show significant abnormalities (Pinelli et al., 1995) such as decreased heart rate variation (Pisano et al., 1995), alterations of the excretory function of the salivary glands (Giess et al., 2000), disturbances of the gastrointestinal tract (Toepfer et al., 1997, 1999), and alterations of the skin responses (Masur et al., 1995).
In addition, when examining CLIS patients with electroencephalography (EEG), Kotchoubey et al. (2003) reported a large variability of event-related responses (ERP) including a complete loss of ERP. Even when provided with EEG-based brain-computer interfaces (BCIs), ALS patients are unable to retain communication once they enter CLIS. This loss of the ability to communicate might be related to the disease itself and may reflect an irreversible decline of awareness, in which case it would be unavoidable (Kübler and Birbaumer, 2008). On the other hand, such a lack of communication could also be due to methodological and technical problems that could be overcome by alternative BCI approaches (Bensch et al., 2014). Recently, a metabolic BCI based on near-infrared spectroscopy has been introduced as a promising tool for communication in CLIS (Gallegos-Ayala et al., 2014).
However, locked-in patients are known to suffer from disturbed sleep patterns with increased fragmentation during the course of the disease (Ferguson et al., 1995; Soekadar et al., 2013). These fluctuations might inherently limit the success of novel BCI approaches in the affected patients. Detecting episodes of low arousal may therefore be essential in optimizing the timing of communication attempts towards phases of higher arousal. At the same time, distorting and attenuating effects of the skull and intermediate tissue on the neural signals inherent to the classical EEG approach might be surmounted by signal detection closer to the brain (Buzsáki et al., 2012; Bensch et al., 2014). Recently, we have introduced epidural ECoG as a tool to assess attention and cognitive function in LIS (Bensch et al., 2014). However, this technique applied event-related brain-potentials at specific time points to track the long-term transition from the locked-in to the completely locked in-state, thereby not allowing a close-meshed monitoring of the patient’s current state of arousal (Bensch et al., 2014).
Thus, a novel methodology for continuous monitoring is still required to gain information on the patient’s present level of arousal and to overcome the limitations of current approaches so as to interact with patients without motor responsiveness.
Methods
Clinical Case
The patient described in this manuscript was a well-informed 40-year-old male, late-stage ALS patient, who had been diagnosed with this disease 10 years before hospitalization and who already had a 7-year history of artificial ventilation. Being fully aware of the long-term consequences of ALS with respect to the loss of all ability to communicate in later stages of this progressive disease, he was determined to retain this ability for as long as possible. Although he was already in a late stage of this disease when he contacted us, he was still able to communicate reliably via muscle twitches. During the period of several months in which we had the opportunity to examine him on a daily basis, his status deteriorated continuously, i.e., a transition from LIS to CLIS took place. While he was initially able to gain some control of an EEG-based BCI, his BCI performance dropped to random over the course of several weeks.
Before entering this stage of his disease, the patient and his legal representative had given informed consent to implantation of an ECoG grid, both on first contact before hospitalization and during his stay in hospital before surgery. The purpose of this implantation was to monitor the patient’s arousal in the absence of other biomarkers and to develop alternative communication pathways for him with ECoG-based BCI technology. The results of the BCI communication study do not constitute part of the present report. This study was conducted in accordance with the Declaration of Helsinki and with the guidelines of the local ethics committee of the University of Tuebingen (Medical Faculty).
Electrocorticography
The observation at our institution covered a period of 6 months after ECoG grid implantation before the patient died due to a general deterioration of his medical condition unrelated to the implantation. There was no post-mortem examination, e.g., of the implant, due to the wish of the legal representative of the patient. During the observation period, the patient lost all volitional motor control for communication and entered the CLIS. For further details on this transition see Murguialday et al. (2011) and Bensch et al. (2014). Epidural ECoG recording (BrainAmp amplifier from Brain Products GmbH, Munich, Germany) was performed with a custom-made grid and two electrode strips, resulting in a total of 128 contacts (Ad-Tech Medical Instrument Corporation, Wisconsin, USA) covering parts of the left frontal, temporal and parietal cortex (Figure 1) which were externalized through subcutaneous extension wires in the ipsilateral subclavicular region. Coregistration of preoperative magnetic resonance imaging and postoperative computed tomography imaging allowed determining electrode positions with respect to the brain.
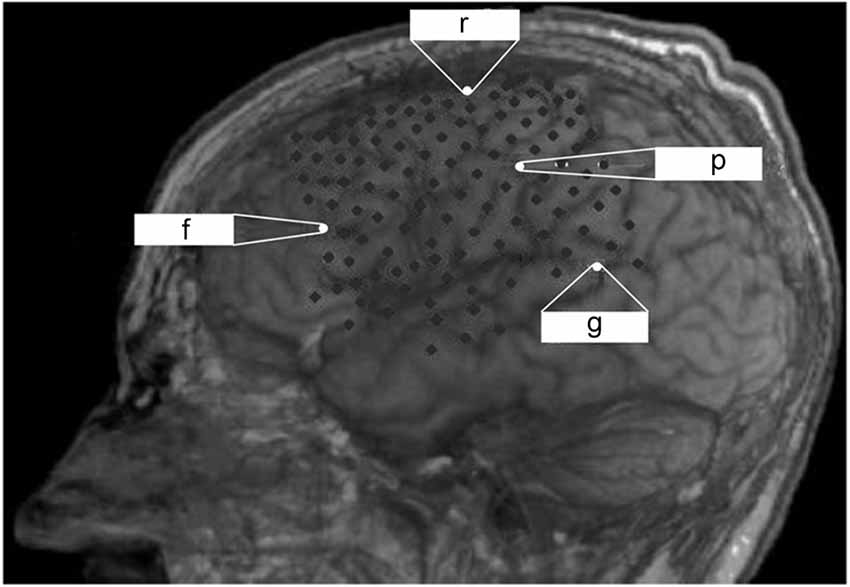
Figure 1. Lateral projections of implanted ECoG recording with a grid and two electrode strips resulting in a total of 128 contacts that cover a large part of the left hemisphere. Reference (r), ground (g) and recording channels on the frontal (f) and parietal (p) cortex for monitoring are indicated.
The data reported here was collected continuously over 24 h a day for 30 subsequent days. The ECoG signals were sampled at 500 Hz and data analysis (Matlab, The Mathworks™) was carried out after selecting a frontal and a parietal channel from the ECoG grid based on the signal-to-noise ratio (Figure 1). We intended to cover two different neurofunctional systems with their respective dominant frequencies (7 Hz vs. 15–17 Hz) for long-term monitoring. Moreover, previous studies have shown a significant increase in left frontal cortex glucose metabolism to correlate with improved cognitive function following amantadine medication in patients with chronic traumatic brain injury (Kraus et al., 2005). We performed a spectral analysis on the signals from these two channels, which remained constant throughout the measurements. We began by downsampling the ECoG data to 60 Hz after proper low-pass filtering to reduce the data set size. We then divided the data into 30-s epochs, estimated an autoregressive (AR) model of the order of 7 in each epoch, and derived the power spectrum from the AR coefficients in each epoch as described in Nielsen (1992). We extracted the dominant frequency over time as the frequency within the 2–30 Hz band with maximum spectral power per epoch and per channel. We then classified each 30-s epoch as a slow-wave epoch, if the dominant frequency in both channels was lower than 4 Hz and as a non-slow-wave epoch otherwise. We derived a slow-wave on/off curve by treating each consecutive epoch as a time sample and by assigning a value of 1 to slow-wave epochs and a value of 0 to non-slow-wave epochs. We then calculated the autocorrelation function of this slow-wave on/off curve to detect periodic components. We also calculated an auto-correlation function on the simultaneously recorded temperature and the heartbeat rate to detect rhythmicity.
Results
Clinical Characteristics
Epidural ECoG was feasible and safe for monitoring cortical physiology in a late-stage ALS patient in CLIS. Despite long-term application for a period of 6 months, no implant-related complications arose; in particular, there was no infection at the site of externalization of the connection wires through the skin. Although a multi-channel device was implanted to maximize signal recording for BCI application, two recording channels (one frontal and one parietal, see Figure 1) were sufficient for longitudinal monitoring of arousal, indicating that less extended implants are feasible for this purpose in future cases.
ECoG Characteristics
The frontal channel showed activity with a dominant frequency of 7 Hz, whereas the parietal channel showed an increased activity between 15 and 17 Hz. At times, the ECoG slowed down and manifested high-amplitude waves in the delta and theta range, with spindle-like activity allowing for bedside visual analysis (Figure 2). Three months after implantation we started daily amantadine medication (30 mg/day). At this time point the patient had no control over eye movements for more than 1 month, i.e., he was in the CLIS. Four days after the start of amantadine administration, the dominant frequency increased from 7 to 20 Hz (frontal channel) and from 17 to 20 Hz (parietal channel). Eleven days after the start of the amantadine administration, the patient could reliably communicate for about 30 min by means of eye movements, during which he answered negatively to the question whether he felt sorry about the surgery and replied positively to the question whether he was happy to have tried the operation. He denied wanting to die and confirmed wanting to live. After this last communication he was permanently in CLIS and was no longer able to respond to our questions.
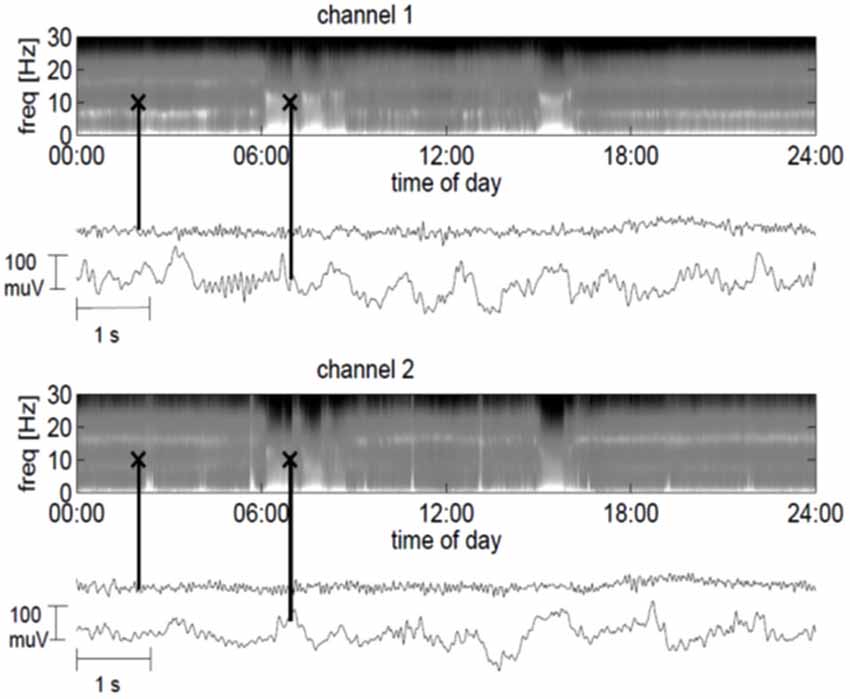
Figure 2. 24-hour power spectra derived from the ECoG for the frontal channel 1 (top plot) and the parietal channel 2 (lower plot), where bright colors indicate strong spectral power. In addition, 10-s ECoG traces are indicated by black crosses in the spectrograms. The markers at 2:00 am in the spectrograms correspond to the traces directly below the spectrogram and depict the ECoG in a minimally slow state (channel 1) or normal state (channel 2). The markers at 7:00 am in the spectrograms correspond to the lower traces and show the ECoG in a slow wave period.
Arousal and Circadian Rhythm
We discovered that the ECoG slow-wave pattern with a frequency below 4 Hz was readily reversible by tactile stimulation, for example by repositioning the patient. This suggested that this slow-wave pattern represented phases of sleep or very low arousal similar to those in EEG recordings in healthy subjects. The slow-wave patterns were somewhat irregular. A slow wave period frequently began around midnight, although slow-wave periods were also frequently recorded during the day (Figure 3). Slow-wave periods were occasionally absent for more than 24 h. Despite these irregularities, the spectrum of the slow-wave on/off curve revealed a dominant periodicity of about 24 h (Figure 4). By contrast, neither the body temperature nor the heart rate curve revealed a circadian rhythmicity.
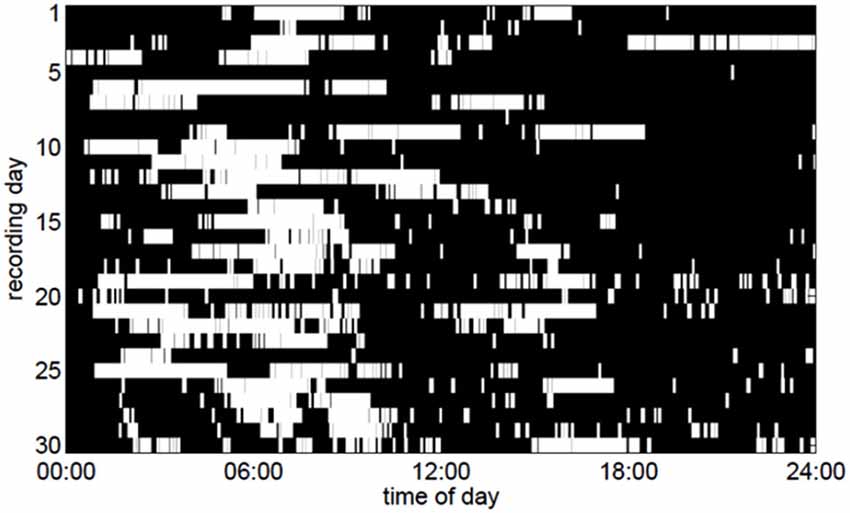
Figure 3. Periods of slow-wave activity (white pixels) in the ECoG as a function of the time of day over a period of 30 days. To make the figure clearer, only slow-wave periods lasting for at least 5 min are depicted here.
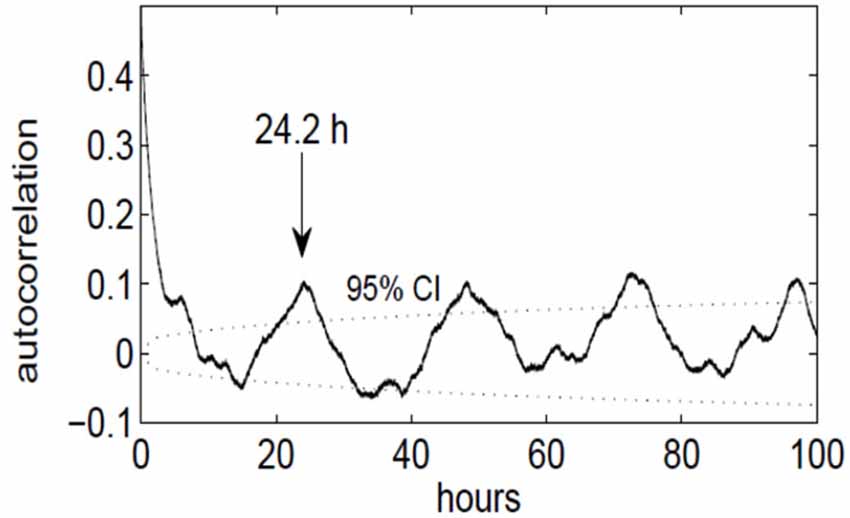
Figure 4. Autocorrelation function of the slow-wave on/off curve, indicating a periodicity of approximately 24 h. The dotted lines denote the upper and lower 95% confidence limit (p = 0.05), obtained by computing the effective sample size and the large-lag error by Bartlett’s formula.
Discussion
In this study, we have demonstrated that ECoG is a safe and robust tool for continuous, long-term monitoring of intrinsic oscillatory activity in a completely locked-in patient in the real-world clinical setting of an intensive care unit.
Although it is difficult to draw general conclusions from a single case, this observation supports previous findings in non-human primates demonstrating long-term stability of ECoG-based decoding of task-related oscillatory activity over several months (Chao et al., 2010). In their experiments, Chao et al. (2010) used the classical subdural ECoG recording technique. This technique is also applied in the majority of clinical applications, e.g., for the identification of seizure foci (McKhann et al., 2000; Kuruvilla and Flink, 2003; Luther et al., 2011) or eloquent cortex (Leuthardt et al., 2007; Miller et al., 2007, 2011). Although these subdural ECoG grids in patients are usually implanted for a short period of several days to 1–2 weeks only, they have been associated with significant complications. These complications increased as the number of grid electrodes increased, in particular >60 electrodes, and the longer the monitoring lasted, especially after a period of 10 days (Hamer et al., 2002). In their study, Hamer et al. (2002) also showed that infection, which occurred in 24 of 187 patients (12.2%), was the most relevant complication. These data can be intuitively explained by the fact that penetrating the dura through wires leaves a pathway for infections during the time of monitoring.
In the last few years, placing ECoG grids in the epidural space has been proposed to be a less invasive alternative to subdural grids, with most studies reporting on the decoding of task-related oscillatory activity in rodents (Uejima et al., 2009; Slutzky et al., 2010, 2011) or non-human primates (Rouse and Moran, 2009; Shimoda et al., 2012; Lee et al., 2013; Marathe and Taylor, 2013; Rouse et al., 2013; Williams et al., 2013). A few human case studies have also shown the feasibility of epidural recordings for decoding task-related oscillations during short recording sessions (Leuthardt et al., 2006; Gomez-Rodriguez et al., 2010; Gharabaghi et al., 2014a,b).
However, to date, no long-term recording of epidural ECoG has been reported, albeit recent experimental studies indicate signal feature detection of comparable fidelity for this modality as opposed to subdural ECoG (Slutzky et al., 2010; Torres Valderrama et al., 2010). Slutzky et al. (2010) used finite element modeling to compare the spatial resolution of epidurally recorded signals with those recorded from subdural locations. They came to the conclusion that epidural recordings yield signals of a quality similar to that of subdural recordings. Due to their reduced invasiveness, the former thus provide a more attractive source of control signals for BCIs. Moreover, Torres Valderrama et al. (2010) have reported measurements of brain signal attenuation by the human dura in vivo and also used signal detection theory to examine how the presence of the dura between the sources and the recording electrodes affects signal power features. It is worth mentioning that they observed no detrimental effects on signal feature detection due to the dura for noise levels typical of clinical brain signal recording equipment.
Epidural ECoG could therefore provide both a powerful—in terms of signal quality—and safe—in terms of surgical risk—evolution of the traditional subdural ECoG which itself is already known to be a good balance between the more invasive brain-penetrating approaches, i.e., intraparenchymatous micro-arrays, and the less invasive scalp EEGs (Moran, 2010; Schalk, 2010). The advantages of ECoG over EEG due to higher spatial resolution, i.e., millimeters vs. centimeters (Freeman et al., 2000; Slutzky et al., 2010), broader bandwidth, i.e., 0–500 Hz vs. 0–40 Hz (Staba et al., 2002), higher signal amplitude, i.e., 50–100 µV maximum vs. 10–20 µV, greater signal-to-noise ratio (Ball et al., 2009), and less vulnerability to artifacts such as EMG (Freeman et al., 2003), render this recording technique a powerful tool for complex clinical cases. Using simultaneous EEG/ECoG recordings in both experimental and clinical epilepsy, D’Ambrosio et al. (2009) have convincingly demonstrated that epidural and subdural ECoG signals recorded in rodents and humans, respectively, can capture short and focal epileptiform events that were undetectable by EEG despite being typically associated with subtle behavioral correlates that are easily overlooked. This renders the ECoG approach particularly suitable for ALS, where a progressive deterioration of clinical and physiological parameters, including oscillatory brain activity, takes place (Kotchoubey et al., 2003; Murguialday et al., 2011).
Following ECoG implantation in the reported patient, we made a concerted effort to analyze and apply the signals acquired with the implant. No EEG recordings were therefore available for a direct comparison between EEG and ECoG recordings. In view of the fact that the patient had a progressively deteriorating, neurodegenerative disease, a comparison of the earlier, preoperative EEG signals and the later ECoG signals after implantation was not feasible either, particularly since the patient experienced a transition from LIS to CLIS over time. Nonetheless, as we know from the literature, even mildly to moderately affected ALS patients have lower amplitudes and longer latencies of late ERP components than healthy controls (Gil et al., 1995; Münte et al., 1998; Westphal et al., 1998; Vieregge et al., 1999; Hanagasi et al., 2002; Paulus et al., 2002). For the CLIS, Kotchoubey et al. (2003) showed a large variability of the responses, including a complete loss of ERP when recording with EEG. In this context, by using ECoG recordings, we were at least able to rule out signal-distorting effects of the skull and intermediate tissue on the brain signals.
Despite long-term application of the ECoG grid for a period of 6 months—which is, to our knowledge, the longest period for human use reported in literature—no implant-related complications arose; most importantly, no infection occurred. We attributed this to the epidural implantation technique, and propose that this approach is more suited for monitoring chronic diseases than the subdural technique which can lead to considerably more complications the longer the device remains implanted (Hamer et al., 2002). The most obvious limitation of the present approach, however, was the necessity to externalize the connecting wires through the skin. Despite the long-term period of skin penetration, no complications related to this externalization were observed. This might be at least partly explained by the fact that we deliberately chose a region for externalization that was far enough away from the implantation site but still easily accessible for nursing, i.e., at the subclavicular region and not at the head, as is usually the case when the connecting wires of ECoG grids are externalized. Nonetheless, externalizing wires for long-term recording must be regarded as a temporary solution only. Together with industrial partners, we are therefore currently working on the development of implantable devices for clinical use which allow for wireless transmission of intracranial multichannel signals for long-term monitoring purposes. Although similar projects have already been reported (Anderson and Harrison, 2010; Guillory et al., 2011; Hirata et al., 2011; Charvet et al., 2012), no appropriate devices are currently available for clinical use.
A further limitation of the current technique was the large size of the grid and the number of electrodes implanted which necessitated a craniotomy, and hence increased invasiveness. This was based on the rationale to cover frontal (language-related), sensorimotor and temporal cortical areas for exploring different neurofunctional systems to implement BCI based communication in CLIS. Since this goal had not been realized in any other case, yet, our aim was to provide access to all relevant target structures for this purpose.
The essential precondition for any kind of BCI based communication was to identify the time periods best suited for BCI trials. This task was nontrivial since ALS patients were known to have frequent episodes of low arousal, disturbed and fragmented sleep patterns (Ferguson et al., 1995; Laureys et al., 2005; Soekadar et al., 2013) or even severe insomnia (Barthlen and Lange, 2000). Since rapid eye movement and muscle tone information was absent in the CLIS, only a coarse sleep assessment based on oscillatory recordings could be performed. Moreover, in LIS and CLIS, the operational electrophysiological definition of wakefulness and sleep was problematic because oscillations recorded might no longer have reflected the same mechanisms as in normal physiological sleep. In healthy individuals decreased arousal during sleep is accompanied by well-defined EEG changes such as slow-wave patterns (Rechtschaffen and Kales, 1968). In pathological conditions, the same slow waves do not necessarily indicate deep non-rapid eye movement or slow-wave sleep as is the case in normal sleeping individuals (Cologan et al., 2010). Sleep stage criteria in LIS and CLIS remained undefined. However, the detection of a circadian rhythmicity indicates the preservation of an—although disturbed—sleep-wake cycle, thereby allowing to differentiate the respective arousal states.
In our study, epidural recordings from one contact in the region of the dorso-lateral, left frontal cortex was sufficient to differentiate between three arousal states. Spectral analysis of the intrinsic oscillatory activity enabled us to extract state-dependent dominant frequencies at below 4 Hz, around 7 Hz and around 20 Hz, respectively. Slow wave patterns below 4 Hz commenced around midnight, although they also often occurred during the day and were unpredictable with regard to their frequency and duration. Despite these irregularities, the spectrum of the slow-wave curve revealed a dominant periodicity of about 24 h, indicating that they represented phases of sleep or very low arousal, which are common in LIS (Laureys et al., 2005). These phases were readily reversible, e.g., when the patient experienced tactile stimulation during positioning, resulting in a dominant frequency of around 7 Hz. Time periods with this dominant frequency occurred often in the afternoon and evening, and probably represented awake states. Since ECoG recordings have revealed that beta oscillations (13–30 Hz) extend far beyond primary sensorimotor regions towards the middle frontal gyrus and the pars opercularis of the frontal cortex (Groppe et al., 2013) and as TMS evoked EEG responses in the same region present a natural frequency of >20 Hz (Rosanova et al., 2009), the slowed dominant frequency (around 7 Hz) in our patient—which is often described in LISs (Patterson and Grabois, 1986)—might thus indicate decreased arousal despite the awake state in this patient and is probably related to the disease. This interpretation is supported by the increase of the dominant frequency to 20 Hz following several days of repetitive amantadine medication. This increase towards the natural frequency of the frontal cortex in our patient indicates increased arousal and is in line with earlier results in studies on the influence of amantadine in patients with chronic traumatic brain injury (Kraus et al., 2005). They showed a significant increase in left frontal cortex glucose metabolism correlated with improved cognitive function following amantadine medication. Similarly, in our patient the increase of the intrinsic oscillatory pattern was paralleled by a new episode of reliable communication by means of eye movements.
In the absence of other biomarkers, epidural ECoG proved to be a reliable tool for monitoring circadian rhythmicity in CLIS, i.e., avoiding interference with the patient when he was sleeping and exploiting time windows of responsiveness. This enabled nursing staff and therapists to adopt interventions in accordance with the patient’s individual arousal (i.e., schedule them in the afternoons and evenings). Moreover, we demonstrated that sufficient arousal monitoring could be acquired from one frontal electrode contact, indicating that future implants may require only a relatively small burr hole, thus reducing surgical risks in this vulnerable patient group even further. This methodology might also present a useful tool for monitoring the arousal in other conditions with pathologically altered consciousness whenever the patients’ motor responsiveness is compromised.
Conclusions
We implemented a novel technique for continuous, long-term monitoring of arousal in a chronic disease without further physiological or behavioral parameters. The epidural ECoG approach presented here constitutes a feasible trade-off between surgical risk and quality of brain signals. The information provided is essential for both the social and the medical environment in dealing with expectations and planning interventions that aim to implement alternative communication pathways such as BCIs in conditions of missing motor responsiveness.
Conflict of Interest Statement
The authors declare that the research was conducted in the absence of any commercial or financial relationships that could be construed as a potential conflict of interest.
Acknowledgments
Alireza Gharabaghi was supported by grants from the German Research Council [DFG GH 94/2-1, DFG EC 307], and from the Federal Ministry for Education and Research [BFNT 01GQ0761, BMBF 16SV3783, BMBF 03160064B, BMBF V4UKF014].
References
Anderson, G. S., and Harrison, R. R. (2010). “Wireless integrated circuit for the acquisition of electrocorticogram signals,” in Circuits and Systems (ISCAS), Proceedings of 2010 IEEE International Symposium (Paris, France), 2952–2955.
Ball, T., Kern, M., Mutschler, I., Aertsen, A., and Schulze-Bonhage, A. (2009). Signal quality of simultaneously recorded invasive and non-invasive EEG. Neuroimage 46, 708–716. doi: 10.1016/j.neuroimage.2009.02.028
Pubmed Abstract | Pubmed Full Text | CrossRef Full Text | Google Scholar
Barthlen, G. M., and Lange, D. J. (2000). Unexpectedly severe sleep and respiratory pathology in patients with amyotrophic lateral sclerosis. Eur. J. Neurol. 7, 299–302. doi: 10.1046/j.1468-1331.2000.00044.x
Pubmed Abstract | Pubmed Full Text | CrossRef Full Text | Google Scholar
Bensch, M., Martens, S., Halder, S., Hill, J., Nijboer, F., Ramos, A., et al. (2014). Assessing attention and cognitive function in completely locked-in state with event-related brain potentials and epidural electrocorticography. J. Neural Eng. 11:026006. doi: 10.1088/1741-2560/11/2/026006
Pubmed Abstract | Pubmed Full Text | CrossRef Full Text | Google Scholar
Buzsáki, G., Anastassiou, C. A., and Koch, C. (2012). The origin of extracellular fields and currents—EEG, ECoG, LFP and spikes. Nat. Rev. Neurosci. 13, 407–420. doi: 10.1038/nrn3241
Pubmed Abstract | Pubmed Full Text | CrossRef Full Text | Google Scholar
Chao, Z. C., Nagasaka, Y., and Fujii, N. (2010). Long-term asynchronous decoding of arm motion using electrocorticographic signals in monkeys. Front. Neuroeng. 3:3. doi: 10.3389/fneng.2010.00003
Pubmed Abstract | Pubmed Full Text | CrossRef Full Text | Google Scholar
Charvet, G., Foerster, M., Chatalic, G., Michea, A., Porcherot, J., Bonnet, S., et al. (2012). A wireless 64-channel ECoG recording electronic for implantable monitoring and BCI applications: WIMAGINE. Conf. Proc. IEEE Eng. Med. Biol. Soc. 2012, 783–786. doi: 10.1109/EMBC.2012.6346048
Pubmed Abstract | Pubmed Full Text | CrossRef Full Text | Google Scholar
Cologan, V., Schabus, M., Ledoux, D., Moonen, G., Maquet, P., and Laureys, S. (2010). Sleep in disorders of consciousness. Sleep Med. Rev. 14, 97–105. doi: 10.1016/j.smrv.2009.04.003
Pubmed Abstract | Pubmed Full Text | CrossRef Full Text | Google Scholar
D’Ambrosio, R., Hakimian, S., Stewart, T., Verley, D. R., Fender, J. S., Eastman, C. L., et al. (2009). Functional definition of seizure provides new insight into post-traumatic epileptogenesis. Brain 132, 2805–2821. doi: 10.1093/brain/awp217
Pubmed Abstract | Pubmed Full Text | CrossRef Full Text | Google Scholar
Ferguson, K. A., Strong, M. J., Ahmad, D., and George, C. F. (1995). Sleep and breathing in amyotrophic lateral sclerosis. Sleep 18, 514.
Freeman, W. J., Holmes, M. D., Burke, B. C., and Vanhatalo, S. (2003). Spatial spectra of scalp EEG and EMG from awake humans. Clin. Neurophysiol. 114, 1053–1068. doi: 10.1016/s1388-2457(03)00045-2
Pubmed Abstract | Pubmed Full Text | CrossRef Full Text | Google Scholar
Freeman, W. J., Rogers, L. J., Holmes, M. D., and Silbergeld, D. L. (2000). Spatial spectral analysis of human electrocorticograms including the alpha and gamma bands. J. Neurosci. Methods 95, 111–121. doi: 10.1016/s0165-0270(99)00160-0
Pubmed Abstract | Pubmed Full Text | CrossRef Full Text | Google Scholar
Gallegos-Ayala, G., Furdea, A., Takano, K., Ruf, C. A., Flor, H., and Birbaumer, N. (2014). Brain communication in a completely locked-in patient using bedside near-infrared spectroscopy. Neurology 82, 1930–1932. doi: 10.1212/WNL.0000000000000449
Pubmed Abstract | Pubmed Full Text | CrossRef Full Text | Google Scholar
Gharabaghi, A., Naros, G., Walter, A., Grimm, F., Schuermeyer, M., Roth, A., et al. (2014a). From assistance towards restoration with epidural brain-computer interfacing. Restor. Neurol. Neurosci. 32, 517–525. doi: 10.3233/RNN-140387
Pubmed Abstract | Pubmed Full Text | CrossRef Full Text | Google Scholar
Gharabaghi, A., Naros, G., Walter, A., Roth, A., Bogdan, M., Rosenstiel, W., et al. (2014b). Epidural electrocorticography of phantom hand movement following long-term upper-limb amputation. Front. Hum. Neurosci. 8:285. doi: 10.3389/fnhum.2014.00285
Pubmed Abstract | Pubmed Full Text | CrossRef Full Text | Google Scholar
Giess, R., Naumann, M., Werner, E., Riemann, R., Beck, M., Puls, I., et al. (2000). Injections of botulinum toxin A into the salivary glands improve sialorrhoea in amyotrophic lateral sclerosis. J. Neurol. Neurosurg. Psychiatry 69, 121–123. doi: 10.1136/jnnp.69.1.121
Pubmed Abstract | Pubmed Full Text | CrossRef Full Text | Google Scholar
Gil, R., Neau, J. P., Dary-Auriol, M., Agbo, C., Tantot, A. M., and Ingrand, P. (1995). Event-related auditory evoked potentials and amyotrophic lateral sclerosis. Arch. Neurol. 52, 890–896. doi: 10.1001/archneur.1995.00540330068017
Pubmed Abstract | Pubmed Full Text | CrossRef Full Text | Google Scholar
Gomez-Rodriguez, M., Grosse-Wentrup, M., Peters, J., Naros, G., Hill, J., Scholkopf, B., et al. (2010). “Epidural ECoG online decoding of arm movement intention in hemiparesis,” in Brain Decoding: Pattern Recognition Challenges in Neuroimaging (WBD), 2010 First Workshop (Istanbul), 36–39.
Groppe, D. M., Bickel, S., Keller, C. J., Jain, S. K., Hwang, S. T., Harden, C., et al. (2013). Dominant frequencies of resting human brain activity as measured by the electrocorticogram. Neuroimage 79, 223–233. doi: 10.1016/j.neuroimage.2013.04.044
Pubmed Abstract | Pubmed Full Text | CrossRef Full Text | Google Scholar
Guillory, K. S., Askin, R. E., Smith, C. F., Mcdonnall, D., Hiatt, S., and Wilder, A. M. (2011). “Wireless electrocortigraph (ECoG) recording system,” in Neural Engineering (NER), 2011 5th International IEEE/EMBS Conference (Cancun), 196–197.
Hamer, H. M., Morris, H. H., Mascha, E. J., Karafa, M. T., Bingaman, W. E., Bej, M. D., et al. (2002). Complications of invasive video-EEG monitoring with subdural grid electrodes. Neurology 58, 97–103. doi: 10.1212/wnl.58.1.97
Pubmed Abstract | Pubmed Full Text | CrossRef Full Text | Google Scholar
Hanagasi, H. A., Gurvit, I. H., Ermutlu, N., Kaptanoglu, G., Karamursel, S., Idrisoglu, H. A., et al. (2002). Cognitive impairment in amyotrophic lateral sclerosis: evidence from neuropsychological investigation and event-related potentials. Brain Res. Cogn. Brain Res. 14, 234–244. doi: 10.1016/s0926-6410(02)00110-6
Pubmed Abstract | Pubmed Full Text | CrossRef Full Text | Google Scholar
Hirata, M., Matsushita, K., Suzuki, T., Yoshida, T., Morris, S., Yanagisawa, T., et al. (2011). A Fully-Implantable wireless system for human brain-machine interfaces using brain surface electrodes: W-HERBS. IEICE Trans. Commun. 94, 2448–2453. doi: 10.1587/transcom.e94.b.2448
Kotchoubey, B., Lang, S., Winter, S., and Birbaumer, N. (2003). Cognitive processing in completely paralyzed patients with amyotrophic lateral sclerosis. Eur. J. Neurol. 10, 551–558. doi: 10.1046/j.1468-1331.2003.00647.x
Pubmed Abstract | Pubmed Full Text | CrossRef Full Text | Google Scholar
Kraus, M. F., Smith, G. S., Butters, M., Donnell, A. J., Dixon, E., Yilong, C., et al. (2005). Effects of the dopaminergic agent and NMDA receptor antagonist amantadine on cognitive function, cerebral glucose metabolism and D2 receptor availability in chronic traumatic brain injury: a study using positron emission tomography (PET). Brain Inj. 19, 471–479. doi: 10.1080/02699050400025059
Pubmed Abstract | Pubmed Full Text | CrossRef Full Text | Google Scholar
Kübler, A., and Birbaumer, N. (2008). Brain-computer interfaces and communication in paralysis: extinction of goal directed thinking in completely paralysed patients? Clin. Neurophysiol. 119, 2658–2666. doi: 10.1016/j.clinph.2008.06.019
Pubmed Abstract | Pubmed Full Text | CrossRef Full Text | Google Scholar
Kuruvilla, A., and Flink, R. (2003). Intraoperative electrocorticography in epilepsy surgery: useful or not? Seizure 12, 577–584. doi: 10.1016/s1059-1311(03)00095-5
Pubmed Abstract | Pubmed Full Text | CrossRef Full Text | Google Scholar
Laureys, S., Boly, M., Moonen, G., and Maquet, P. (2009). “Coma,” in Encyclopedia of Neuroscience, ed L. Squire (Oxford: Academic Press), 1133–1142.
Laureys, S., Owen, A. M., and Schiff, N. D. (2004). Brain function in coma, vegetative state and related disorders. Lancet Neurol. 3, 537–546. doi: 10.1016/s1474-4422(04)00852-x
Pubmed Abstract | Pubmed Full Text | CrossRef Full Text | Google Scholar
Laureys, S., Pellas, F., Van Eeckhout, P., Ghorbel, S., Schnakers, C., Perrin, F., et al. (2005). The locked-in syndrome: what is it like to be conscious but paralyzed and voiceless? Prog. Brain Res. 150, 495–511. doi: 10.1016/s0079-6123(05)50034-7
Pubmed Abstract | Pubmed Full Text | CrossRef Full Text | Google Scholar
Lee, J., Choi, H., Kim, T., Lee, H., Kim, I. Y., Jang, D. P., et al. (2013). “The effectiveness of epidural ECoG on brain computer interface in primate,” in Brain-Computer Interface (BCI), 2013 International Winter Workshop (Gangwo), 107–108.
Leuthardt, E. C., Miller, K., Anderson, N. R., Schalk, G., Dowling, J., Miller, J., et al. (2007). Electrocorticographic frequency alteration mapping: a clinical technique for mapping the motor cortex. Neurosurgery 60, 260–270; discussion 270–271. doi: 10.1227/01.neu.0000255413.70807.6e
Pubmed Abstract | Pubmed Full Text | CrossRef Full Text | Google Scholar
Leuthardt, E. C., Miller, K. J., Schalk, G., Rao, R. P., and Ojemann, J. G. (2006). Electrocorticography-based brain computer interface—the Seattle experience. IEEE Trans. Neural Syst. Rehabil. Eng. 14, 194–198. doi: 10.1109/tnsre.2006.875536
Pubmed Abstract | Pubmed Full Text | CrossRef Full Text | Google Scholar
Luther, N., Rubens, E., Sethi, N., Kandula, P., Labar, D. R., Harden, C., et al. (2011). The value of intraoperative electrocorticography in surgical decision making for temporal lobe epilepsy with normal MRI. Epilepsia 52, 941–948. doi: 10.1111/j.1528-1167.2011.03061.x
Pubmed Abstract | Pubmed Full Text | CrossRef Full Text | Google Scholar
Marathe, A. R., and Taylor, D. M. (2013). Decoding continuous limb movements from high-density epidural electrode arrays using custom spatial filters. J. Neural Eng. 10:036015. doi: 10.1088/1741-2560/10/3/036015
Pubmed Abstract | Pubmed Full Text | CrossRef Full Text | Google Scholar
Masur, H., Schulte-Oversohl, U., Papke, K., Oberwittler, C., and Vollmer, J. (1995). Sympathetic skin response in patients with amyotrophic lateral sclerosis. Funct. Neurol. 10, 131–135.
McKhann, G. M. 2nd, Schoenfeld-Mcneill, J., Born, D. E., Haglund, M. M., and Ojemann, G. A. (2000). Intraoperative hippocampal electrocorticography to predict the extent of hippocampal resection in temporal lobe epilepsy surgery. J. Neurosurg. 93, 44–52. doi: 10.3171/jns.2000.93.1.0044
Pubmed Abstract | Pubmed Full Text | CrossRef Full Text | Google Scholar
Miller, K. J., Abel, T. J., Hebb, A. O., and Ojemann, J. G. (2011). Rapid online language mapping with electrocorticography. J. Neurosurg. Pediatr. 7, 482–490. doi: 10.3171/2011.2.PEDS1156
Pubmed Abstract | Pubmed Full Text | CrossRef Full Text | Google Scholar
Miller, K. J., Dennijs, M., Shenoy, P., Miller, J. W., Rao, R. P., and Ojemann, J. G. (2007). Real-time functional brain mapping using electrocorticography. Neuroimage 37, 504–507. doi: 10.1016/j.neuroimage.2007.05.029
Pubmed Abstract | Pubmed Full Text | CrossRef Full Text | Google Scholar
Moran, D. (2010). Evolution of brain-computer interface: action potentials, local field potentials and electrocorticograms. Curr. Opin. Neurobiol. 20, 741–745. doi: 10.1016/j.conb.2010.09.010
Pubmed Abstract | Pubmed Full Text | CrossRef Full Text | Google Scholar
Münte, T. F., Tröger, M. C., Nusser, I., Wieringa, B. M., Johannes, S., Matzke, M., et al. (1998). Alteration of early components of the visual evoked potential in amyotrophic lateral sclerosis. J. Neurol. 245, 206–210. doi: 10.1007/s004150050206
Pubmed Abstract | Pubmed Full Text | CrossRef Full Text | Google Scholar
Murguialday, A. R., Hill, J., Bensch, M., Martens, S., Halder, S., Nijboer, F., et al. (2011). Transition from the locked in to the completely locked-in state: a physiological analysis. Clin. Neurophysiol. 122, 925–933. doi: 10.1016/j.clinph.2010.08.019
Pubmed Abstract | Pubmed Full Text | CrossRef Full Text | Google Scholar
Patterson, J. R., and Grabois, M. (1986). Locked-in syndrome: a review of 139 cases. Stroke 17, 758–764. doi: 10.1161/01.str.17.4.758
Pubmed Abstract | Pubmed Full Text | CrossRef Full Text | Google Scholar
Paulus, K. S., Magnano, I., Piras, M. R., Solinas, M. A., Solinas, G., Sau, G. F., et al. (2002). Visual and auditory event-related potentials in sporadic amyotrophic lateral sclerosis. Clin. Neurophysiol. 113, 853–861. doi: 10.1016/s1388-2457(02)00082-2
Pubmed Abstract | Pubmed Full Text | CrossRef Full Text | Google Scholar
Pinelli, P., Pisano, F., and Miscio, G. (1995). The possible role of a secondary pathogenetic factor in amyotrophic lateral sclerosis. Adv. Neurol. 68, 29–40.
Pisano, F., Miscio, G., Mazzuero, G., Lanfranchi, P., Colombo, R., and Pinelli, P. (1995). Decreased heart rate variability in amyotrophic lateral sclerosis. Muscle Nerve 18, 1225–1231. doi: 10.1002/mus.880181103
Pubmed Abstract | Pubmed Full Text | CrossRef Full Text | Google Scholar
Rechtschaffen, A., and Kales, A. (1968). A Manual of Standardized Terminology, Techniques and Scoring System for Sleep Stages of Human Subjects 1968. Bethesda, MD: U.S. Dept. of Health, Education and Welfare.
Rosanova, M., Casali, A., Bellina, V., Resta, F., Mariotti, M., and Massimini, M. (2009). Natural frequencies of human corticothalamic circuits. J. Neurosci. 29, 7679–7685. doi: 10.1523/JNEUROSCI.0445-09.2009
Pubmed Abstract | Pubmed Full Text | CrossRef Full Text | Google Scholar
Rouse, A. G., and Moran, D. W. (2009). Neural adaptation of epidural electrocorticographic (EECoG) signals during closed-loop brain computer interface (BCI) tasks. Conf. Proc. IEEE Eng. Med. Biol. Soc. 2009, 5514–5517. doi: 10.1109/IEMBS.2009.5333180
Pubmed Abstract | Pubmed Full Text | CrossRef Full Text | Google Scholar
Rouse, A. G., Williams, J. J., Wheeler, J. J., and Moran, D. W. (2013). Cortical adaptation to a chronic micro-electrocorticographic brain computer interface. J. Neurosci. 33, 1326–1330. doi: 10.1523/JNEUROSCI.0271-12.2013
Pubmed Abstract | Pubmed Full Text | CrossRef Full Text | Google Scholar
Schalk, G. (2010). Can Electrocorticography (ECoG) support robust and powerful brain-computer interfaces? Front. Neuroeng. 3:9. doi: 10.3389/fneng.2010.00009
Pubmed Abstract | Pubmed Full Text | CrossRef Full Text | Google Scholar
Shimoda, K., Nagasaka, Y., Chao, Z. C., and Fujii, N. (2012). Decoding continuous three-dimensional hand trajectories from epidural electrocorticographic signals in Japanese macaques. J. Neural Eng. 9:036015. doi: 10.1088/1741-2560/9/3/036015
Pubmed Abstract | Pubmed Full Text | CrossRef Full Text | Google Scholar
Slutzky, M. W., Jordan, L. R., Krieg, T., Chen, M., Mogul, D. J., and Miller, L. E. (2010). Optimal spacing of surface electrode arrays for brain-machine interface applications. J. Neural Eng. 7:26004. doi: 10.1088/1741-2560/7/2/026004
Pubmed Abstract | Pubmed Full Text | CrossRef Full Text | Google Scholar
Slutzky, M. W., Jordan, L. R., Lindberg, E. W., Lindsay, K. E., and Miller, L. E. (2011). Decoding the rat forelimb movement direction from epidural and intracortical field potentials. J. Neural Eng. 8:036013. doi: 10.1088/1741-2560/8/3/036013
Pubmed Abstract | Pubmed Full Text | CrossRef Full Text | Google Scholar
Soekadar, S. R., Born, J., Birbaumer, N., Bensch, M., Halder, S., Murguialday, A. R., et al. (2013). Fragmentation of slow wave sleep after onset of complete locked-in state. J. Clin. Sleep Med. 9, 951–953. doi: 10.5664/jcsm.3002
Pubmed Abstract | Pubmed Full Text | CrossRef Full Text | Google Scholar
Staba, R. J., Wilson, C. L., Bragin, A., Fried, I., and Engel, J. Jr. (2002). Quantitative analysis of high-frequency oscillations (80–500 Hz) recorded in human epileptic hippocampus and entorhinal cortex. J. Neurophysiol. 88, 1743–1752.
Toepfer, M., Folwaczny, C., Lochmüller, H., Schroeder, M., Riepl, R. L., Pongratz, D., et al. (1999). Noninvasive (13)C-octanoic acid breath test shows delayed gastric emptying in patients with amyotrophic lateral sclerosis. Digestion 60, 567–571. doi: 10.1159/000007708
Pubmed Abstract | Pubmed Full Text | CrossRef Full Text | Google Scholar
Toepfer, M., Schroeder, M., Klauser, A., Lochmüller, H., Hirschmann, M., Riepl, R. L., et al. (1997). Delayed colonic transit times in amyotrophic lateral sclerosis assessed with radio-opaque markers. Eur. J. Med. Res. 2, 473–476.
Torres Valderrama, A., Oostenveld, R., Vansteensel, M. J., Huiskamp, G. M., and Ramsey, N. F. (2010). Gain of the human dura in vivo and its effects on invasive brain signal feature detection. J. Neurosci. Methods 187, 270–279. doi: 10.1016/j.jneumeth.2010.01.019
Pubmed Abstract | Pubmed Full Text | CrossRef Full Text | Google Scholar
Uejima, T., Kita, K., Fujii, T., Kato, R., Takita, M., and Yokoi, H. (2009). Motion classification using epidural electrodes for low-invasive brain-machine interface. Conf. Proc. IEEE Eng. Med. Biol. Soc. 2009, 6469–6472. doi: 10.1109/IEMBS.2009.5333547
Pubmed Abstract | Pubmed Full Text | CrossRef Full Text | Google Scholar
Vieregge, P., Wauschkuhn, B., Heberlein, I., Hagenah, J., and Verleger, R. (1999). Selective attention is impaired in amyotrophic lateral sclerosis—a study of event-related EEG potentials. Brain Res. Cogn. Brain Res. 8, 27–35. doi: 10.1016/s0926-6410(99)00004-x
Pubmed Abstract | Pubmed Full Text | CrossRef Full Text | Google Scholar
Westphal, K. P., Heinemann, H. A., Grözinger, B., Kotchoubey, B. J., Diekmann, V., Becker, W., et al. (1998). Bereitschaftspotential in amyotrophic lateral sclerosis (ALS): lower amplitudes in patients with hyperreflexia (spasticity). Acta Neurol. Scand. 98, 15–21. doi: 10.1111/j.1600-0404.1998.tb07372.x
Pubmed Abstract | Pubmed Full Text | CrossRef Full Text | Google Scholar
Williams, J. J., Rouse, A. G., Thongpang, S., Williams, J. C., and Moran, D. W. (2013). Differentiating closed-loop cortical intention from rest: building an asynchronous electrocorticographic BCI. J. Neural Eng. 10:046001. doi: 10.1088/1741-2560/10/4/046001
Pubmed Abstract | Pubmed Full Text | CrossRef Full Text | Google Scholar
Keywords: electrocorticography, epidural recording, locked-in state, coma, consciousness, brain-computer interface, neuroprosthetic devices
Citation: Martens S, Bensch M, Halder S, Hill J, Nijboer F, Ramos-Murguialday A, Schoelkopf B, Birbaumer N and Gharabaghi A (2014) Epidural electrocorticography for monitoring of arousal in locked-in state. Front. Hum. Neurosci. 8:861. doi: 10.3389/fnhum.2014.00861
Received: 30 July 2014; Accepted: 06 October 2014;
Published online: 21 October 2014.
Edited by:
Marta Olivetti, Sapienza University of Rome, ItalyReviewed by:
Maurizio Mattia, Istituto Superiore di Sanità, ItalyPierre Megevand, Hofstra North Shore-LIJ School of Medicine, USA
Copyright © 2014 Martens, Bensch, Halder, Hill, Nijboer, Ramos-Murguialday, Schoelkopf, Birbaumer and Gharabaghi. This is an open-access article distributed under the terms of the Creative Commons Attribution License (CC BY). The use, distribution and reproduction in other forums is permitted, provided the original author(s) or licensor are credited and that the original publication in this journal is cited, in accordance with accepted academic practice. No use, distribution or reproduction is permitted which does not comply with these terms.
*Correspondence: Alireza Gharabaghi, Division of Functional and Restorative Neurosurgery and Division of Translational Neurosurgery, Department of Neurosurgery, Eberhard Karls University Tuebingen, Otfried-Mueller-Str. 45, 72076 Tuebingen, Germany e-mail: alireza.gharabaghi@uni-tuebingen.de