- Institute of Medical Psychology and Medical Sociology, University Hospital of RWTH Aachen University, Aachen, Germany
Alcohol dependence is a serious condition characterized by persistent desires to drink and unsuccessful efforts to control alcohol consumption despite the knowledge of dysfunction through the usage. The study at hand examined the influence of an alcohol exposure on inhibitory processes. Research provides evidence that trying to resist the temptation to drink exerts self-control, a limited resource which is used during all acts of inhibition. In line with this, studies demonstrate an impaired ability to regulate an already initiated response in alcohol-dependent and healthy subjects when confronted with alcohol-related stimuli. The related neuronal correlates in alcohol-dependent patients remain to be elucidated. The inhibition performance of 11 male alcohol-dependent patients during an alcohol exposure was compared with the task performance during a control condition. Behavioral data and neural brain activation during task performance were acquired by means of functional magnetic resonance imaging. The alcohol cue exposure led to subjectively stronger urges to drink which was accompanied by differential neural activation in amygdala and hippocampus. Moreover, the results revealed typical neural activation during inhibition performance across both conditions. Anyhow, we could not detect any behavioral deficits and only subtle neural differences between induction conditions during the performance of the inhibition task within the inferior frontal cortex. The results suggest that although the sample reports a subjectively stronger urge to drink after the alcohol cue exposure this effect was not strong enough to significantly impair task performance. Coherently, we discover only subtle differential brain activation between conditions during the inhibition task. In opposition to findings in literature our data do not reveal that an exposure to alcohol-related cues and thereby elicited cue reactivity results in impaired inhibition abilities.
Introduction
Alcohol dependence is a condition characterizing the concerned person by a “persisting substance use despite clear evidence of overtly harmful consequences” according to the International Classification of Diseases, 10th edition (World Health Organization, 1992). Recent research proposes alcohol dependence could result from an imbalance between increased automatic (e.g., cognitive biases/reactions toward emotionally laden stimuli) and decreased controlled processes (e.g., executive control required for response inhibition; Wiers et al., 2007; Noel et al., 2010). According to a model on the development of alcohol dependence repeated alcohol consumption during adolescence leads to a sensitized appetitive system triggering automatic (drinking) behavior and an underdeveloped regulatory executive system (Wiers et al., 2007). An imbalance of these systems impacts on an outlasting global loss of willpower by affecting reactive mechanisms on direct incentives and reflective mechanisms which moderate impulsive behavior (Noel et al., 2010). Hence alcohol may trigger automatic attentional, memory, and associated emotional systems (bottom-up) which modulate (top-down) goal driven attentional resources needed to reflectively regulate ongoing voluntary behavior. This could explain why patients with alcohol dependence keep up consumption or relapse despite their knowledge about the severe consequences.
Alcohol craving is another central criterion for the diagnosis of alcohol dependence and is described as “a strong desire or sense of compulsion to take alcohol” (World Health Organization, 1992). Craving is thought to contribute to relapse in alcohol-dependent patients through loss of self-control, an ability strongly related to willpower (Rankin et al., 1983; Modell et al., 1992; Anton et al., 1995; Littleton, 1995). Longitudinal studies investigating therapeutic processes and outcomes in alcohol-dependent patients support this relationship (O’Malley et al., 1992; Volpicelli et al., 1992; Paille et al., 1995).
One mechanism that is thought to explain the existence of craving symptoms is based on classical conditioning. The theory postulates that exposure to cues that have been regularly associated with alcohol consumption can elicit conditioned urges to drink alcohol (i.e., craving; Anton, 1999). Alcohol-dependent patients who are trying not to drink must, therefore, expend great effort to overcome such conditioned responses when they are confronted with alcohol-related cues (Brown, 1998; Everitt and Robbins, 2005). In other words, they need much self-control to resist the temptation to drink. The ability to self-control seems, however, to be a limited resource, which must be used during all acts of inhibition (Muraven et al., 1998; Muraven and Baumeister, 2000; Muraven and Shmueli, 2006). Thus, according to the “resource depletion model” exerting self-control during alcohol craving can reduce drinkers’ ability to exert self-control in other realms. Also, according to Tiffany and co-workers’ “cognitive processing model of craving” mental processes that are effortful are triggered by situations in which craving is induced and hence may interfere with other cognitive tasks (Tiffany, 1999; Tiffany and Conklin, 2000). The researchers assume that, contrary to classically conditioned responses, some craving induced physiological changes reflect reactions to cognitive demands of certain situations, i.e., representing, if anything, secondarily conditioned effects. Thus, craving effects are not restricted to conditioned responses, a view which offers to investigate data applying broader cognitive psycho-physiological models. The model states that performing complex behavior will be guided by automatic and non-automatic processes. Craving is related to the activation of non-automated processes (Tiffany, 1990). It is elicited in situations when attempting to overcome impediments to automated consumption or to avoid the execution of an automatic drug use sequence. The cognitive substrate associated with craving is reflected in behavior, self-report, and autonomic responses visible in alcoholics who try to stay abstinent and those who do not give up consumption. Above from this, being viewed as a non-automated process craving is capacity limiting, hence hindering successful operation of other cognitively demanding processes (Tiffany and Conklin, 2000). As a consequence the ability to resist the urge to drink alcohol and automated drug use is diminished.
There is an ever-growing body of evidence underpinning this limited resource model and the cognitive model of craving by demonstrating that inhibitory performance is impaired when self-control has to be shown. In studies conducted by Muraven and Shmueli (2006) and by Gauggel et al. (2010) craving-related cue reactivity was elicited via a cue exposure paradigm within laboratory settings. In both studies, cue reactivity was induced by letting the participants take a smell of their favorite alcoholic beverage in contrast to exposing the subjects to the smell of a glass of water. Muraven and Shmueli (2006) investigated a sample of 160 social drinkers whereas Gauggel et al. (2010) studied 20 detoxified patients with alcohol dependence. Both studies used the well-established stop-signal paradigm (SSP; Logan, 1994), a task requiring the ability to cancel an already initiated motor response. Results from both studies support the resource depletion model by demonstrating that cue exposure leads to impairment in subsequent self-control tasks such as the SSP. Importantly, the effect size was much larger in the study by Gauggel et al. (2010) than in Muraven and Shmueli’s (2006) study, suggesting that detoxified alcohol-dependent patients have even greater inhibitory deficits than social drinkers after exposure to alcohol.
The models and results discussed above underline the importance of exploring inhibitory processing during cue exposure among alcohol-dependent patients. There is ample evidence on impairment of various domains of functioning associated with alcohol dependence. Cognitive deficits and impairments in emotional realms have been widely discussed to be intermingled in both development of alcohol dependence and probability of relapse. Nonetheless differences between investigations and findings maintain the discussion concerning underlying processes and changes leading to alcohol dependence and relapse. Studies differ with respect to characteristics displayed by the investigated patients (e.g., age, onset and duration of illness, comorbidities) or progress related factors (e.g., treatment duration, number of detoxifications). Thus, the study at hand was initiated to further investigate the imbalance between attention consuming reactions to salient stimuli and the functioning of regulatory executive systems in patients with alcohol dependence who display a prolonged consumption history.
Moreover, the neuronal correlates of inhibitory processes in alcohol-dependent patients to whom craving is experimentally induced through cue exposure have not yet been intensively investigated. Therefore, the present study will address neuronal mechanisms and correlates involved in inhibitory processes after alcohol cue exposure.
With regard to brain mechanism associated with drug use and craving there is ample evidence supporting the role of the limbic system (Rodriguez de and Navarro, 1998; Miller and Goldsmith, 2001; Heinz et al., 2010). Even so, brain regions intermingled in cue reactivity and reported as being most relevant may slightly differ across individual studies due to differing imaging techniques, stimuli, and populations. For example, confronting subjects with alcohol-related stimuli like pictures, words, or odors associated with beverages has been related to activation in limbic areas such as the anterior cingulate cortex (ACC), amygdala, hippocampus, and thalamus (George et al., 2001; Schneider et al., 2001; Tapert et al., 2003, 2004; Vollstädt-Klein et al., 2011). It has been proposed that different limbic circuits are important in several specific aspects of alcohol-related reward-signal processing (Rodriguez de and Navarro, 1998). Amygdala and hippocampus are thought to be involved in the remembrance and encoding of significant affective stimuli, the appraisal of the acute emotional state as well as the initiation of responses associated with drug exposure whereas aspects of perceptual and attentional nature are predominantly undertaken and coordinated by prefrontal and cingulate cortices (Rodriguez de and Navarro, 1998; Vollstädt-Klein et al., 2011).
The neural source of inhibitory motor control has been widely studied in the past years by revealing the neural correlates during the performance of the SSP. The inferior frontal cortex (IFC), the pre-supplementary motor area (pre-SMA), and the basal ganglia are discussed as key areas for the “inhibitory control network” in animal, lesion, and fMRI studies (Rubia et al., 1999, 2001, 2003; Aron et al., 2003; Rieger et al., 2003; Gauggel et al., 2004; Aron and Poldrack, 2006; Boecker et al., 2011). Aron et al. (2003) found lesion volume affecting the right IFC (rIFC) to be highly correlated with inhibition performance while Chambers et al. (2006) found that a temporary deactivation of the pars opercularis in the rIFC via transcranial magnetic stimulation (TMS) impairs the ability to inhibit an already initiated action. The role of pre-SMA as a part of the dorsomedial frontal cortex and its relation to the rIFC is still unclear. There is emerging evidence from animal and human studies describing the pre-SMA as “negative motor area” (Aron et al., 2007; Aron, 2011) which generates control signals for specific actions rather than controlling whether or not a movement is made (Scangos and Stuphorn, 2010) while the rIFC is thought to be responsible for the implementation of inhibitory control (Aron et al., 2007). Evidence for an involvement of the basal ganglia in inhibitory processes comes from studies with patients suffering from Parkinson’s disease (Gauggel et al., 2004; van den Wildenberg et al., 2006) and lesion studies with rats where inhibitory processes were requested (Eagle et al., 2008).
Altogether, the present study aims at further extending our knowledge about the impact of cue exposure on self-control and response inhibition by investigating the performance of detoxified patients with alcohol dependence in the SSP during alcohol cue exposure as compared to a neutral exposure condition. Cue exposure was implemented by presenting the smell of the participants’ favorite alcoholic beverage. The smell of orange juice was applied as a neutral olfactory control stimulation in order to improve potential limitations of prior investigations (Muraven and Shmueli, 2006; Gauggel et al., 2010).
We expected (1) that participants would report greater subjective craving in the alcohol cue exposure compared to the alcohol–neutral exposure. We further hypothesized that (2) alcohol cue exposure would result in less available self-control for the execution of the SSP which would be indicated in longer reaction time needed to inhibit an initiated response compared to the control condition. (3) During the alcohol cue exposure we expected more metabolic activity in limbic areas (especially thalamus, amygdala, hippocampus, ACC) compared to the alcohol–neutral condition. Finally, we hypothesized that (4) differences in the IFC, which is deemed important for response inhibition as measured with the SSP, would be found between the alcohol cue exposure condition and the alcohol–neutral condition.
Materials and Methods
Participants
Fourteen male alcohol-dependent patients, who fulfilled ICD-10 criteria for alcohol dependence (F10.21), were recruited from a collaborating psychiatric hospital in Aachen (Germany) to participate in the present study. All participants were undergoing inpatient treatment for alcohol dependence and were abstinent for at least 1 week. Hence, none of the patients was medicated to reduce withdrawal symptoms. After excluding 3 participants due to technical problems within the scanner environment (e.g., malfunctioning interlinkage between scanner and button or headphone devices), 11 participants (mean age = 44, SD = 10, range 25–54 years) remained in the analyses. Means and SDs of the participants’ characteristics are presented in Table 1.
All participants gave informed consent and were paid for their participation. The present study was approved by the Ethical Committee of the Medical Faculty of the University Hospital Aachen (EK 096/08) and was conducted according to the Declaration of Helsinki (World Medical Association, 1999). Any patient who met a standard exclusion criterion for MRI investigation (e.g., metallic implants that obscure or interfere with MRI) could not participate in the present study.
Design
The design of the present study was a within subject design, in which we compared an alcohol condition (alcohol cue exposure) with a control condition (alcohol–neutral exposure) on the dependent variables [reported subjective craving, stop-signal reaction time (SSRT) and error rate of the SSP]. The order of conditions was randomized, so that half of the participants received the alcohol exposure before the alcohol–neutral exposure and vice versa.
Procedure
Pretest
One week prior to the experiment volunteers that met the inclusion criteria were informed about the study contents and procedures. Moreover, participants filled out a volunteers sheet including demographic questions (e.g., age, education), a patient form to assess individual drinking habits and treatment history, the Edinburgh Handedness Inventory (Oldfield, 1971) to ensure only right handed patients would participate and a questionnaire assessing individual drug history (“Fragebogen zur differenzierten Drogenanamnese,” FDDA; Grüsser et al., 2004). Here, we were especially interested in participants’ average alcohol consumption and their age of first and regular consumption (see Table 1). The FDDA documents the consumption onset and duration of other drug use as well (see Table 1). Except tobacco and cannabis, which nine respectively two patients consumed regularly no other drug was frequently used in our sample. In addition, participants were asked about their favorite alcohol and about situations in which they usually and never drink alcohol. This information served as guideline for the induction of conditions during the experiment. Therefore, detailed descriptions of the respective situations (including, e.g., sounds, smells, other persons, surroundings) and accompanying subjective feelings, thoughts, and body sensations were documented.
Training phase
Prior to performing the SSP in the fMRI scanner environment, participants performed two training sessions of 5 min each to become familiar with the task (Figure 1). The SSP consists of circles or triangles (onset stimuli) to which participants have to respond. The paradigm contains three trial types (Go-trials, Stop-trials, and Null-events) each lasting for 3000–3500 ms. A fixation cross is presented in the middle of a gray screen for 1500 ms before each trial. In case of a Null-event, the fixation cross is presented instead of an onset stimulus. In the Go-trials participants perform a simple discrimination task on the two different onset stimuli. They are asked to respond to triangles by pressing a response button with the index finger and to respond to circles by pressing another response button with the middle finger of the right hand. In case of a Stop-trial a Stop-Signal (1000-Hz tone) is presented after the onset stimulus for 500 ms and participants are instructed not to press any button, thus to inhibit their initiated response to the stimulus. Importantly, the delay from onset stimulus to the presentation of the Stop-Signal varies (stimulus onset asynchrony, SOA) according to the staircase-tracking algorithm (Kaernbach, 1991). The SOA is adapted to the participants’ responses, in a way that an inhibition rate of 50% is attained. At the beginning, the SOA is set to 250 ms. If a response can be inhibited successfully the SOA is enhanced by 50 ms in the next Stop-trial. If the response can not be inhibited, the SOA is decreased by 50 ms in the next Stop-trial. The SSRT, which is the time participants needed to inhibit an initiated response, is calculated by means of the difference between the mean reaction time on correctly answered Go-trials and the mean Stop-SOA. This measure indicates participants’ rate of inhibition controlling for their speed of responses to Go-trials. This difference is important, because people who react slower can inhibit a response more easily than people who react faster on the same SOAs.
Training session 1 aimed at making participants familiar with the discrimination task and therefore consisted of 33 Go-trials and 3 Null-events only. In order to practice the inhibition task, training session 2 consisted of 90 trials (56 Go-trials, 24 Stop-trials, and 10 Null-events). Participants were instructed to react as fast and accurately as possible to the stimuli and not to wait for the Stop-Signal, but to try to inhibit their response whenever the Stop-Signal appeared. They were also informed that they could not always be successful, because the Stop-Signals were adapted according to an algorithm which leads to a success rate of 50%.
At the end of the training phase participants were asked to remember the circumstances under which they would always or never drink alcohol discussed the week before in order to make the individual situations accessible during the standardized induction of the two conditions in the scanner environment. The experimenter repeated the scripts for both conditions in detail in order to facilitate elicitation of accompanying thoughts, feelings, and bodily sensations.
Exposure of alcohol and control conditions
In both alcohol and control condition all participants listened to the same standardized auditory scripts while lying in the scanner environment.
Within the alcohol condition (A), cue reactivity was induced by instructing participants to remember and imagine the situation in which they usually drink alcohol before listening to the standardized script within the scanner environment. In the alcohol condition the script referred to the imagination of the typical place in which the participant would be drinking alcohol including the typical smell and sounds of this place as well as to the imagination of the bodily sensations in this situation and the rising urge to drink alcohol. In addition to the auditory instructions, the smell of participants’ favorite alcohol was presented by placing a saturated cloth in the conditioner of the fMRI scanner. This smell of their favorite alcohol was presented every 5 min for 30 s throughout the whole experiment.
In the control condition (N), participants were instructed to imagine the situation, in which they never drink alcohol before listening to a standardized auditory script containing aspects of the place in which this situation would occur as the typical sound and smell. Moreover, it was referred to the bodily sensations in a comfortable situation and the patients were instructed to concentrate on the associated feelings. This time, the smell of oranges was presented to the participants, which again was offered every 5 min for 30 s throughout the whole measurement.
Course of MRI investigation
The subsequent fMRI investigation comprised the accomplishment of two SSPs of about 20 min each intermediated by an acquisition of the brain structure for about 10 min, and a 20-min break. The functioning of the buttons required for the SSP was tested prior to the task execution. Both SSPs comprised 340 trials [210 Go-trials (70%), 90 Stop-trials (30%), and 40 Null-events].
Imminently before the task was performed either the A or N were induced as described above. To assess the individual cue reactivity, two items of the Alcohol Craving Questionnaire (ACQ-Now; Singleton, 1996) were answered while lying in the scanner, once, immediately after the respective induction and another time after the SSP. The statements (“I want to drink so bad I can almost taste it.” and “I would feel less restless if I drank alcohol.”) had to be rated on a seven-point Likert scale (−3 = strongly disagree to 3 = strongly agree). Higher scores indicate stronger substance craving.
Finally, at the day of the MRI investigation, patients filled out the German version of the Brief Symptom Inventory (BSI, Derogatis and Melisaratos, 1983; Derogatis, 1993; Franke, 2000) in order to acquire the occurrence of comorbidities and symptopathology other than alcohol dependence in the sample. The BSI is a self-report assessment of a patient’s symptoms on nine primary dimensions and their intensity at a specific point in time. The BSI provides t-distributed (M = 50, SD = 10) norm values for the nine dimensions and moreover a global severity index (GSI) can be calculated which allows to quantify the patient’s over all severity-of-illness. Table 2 provides the information gained from this assessment in our patient sample.
MR Technical Parameters
Structural and functional MR measurements were acquired at the University hospital of the RWTH Aachen using a 3-T Magneton TRIO TIM MR scanner (Siemens, Erlangen, Germany) with a standard CP head coil. For functional imaging, “Akzent_bold” gradient-echo echoplanar T2*-weighted images (EPI) were acquired [time repetition (TR) = 2400 ms, time echo (TE) = 30 ms, flip angle (FA) = 90°, field of view (FoV) = 220 mm, voxel size (VS) = 2.5 mm × 2.5 mm × 2.5 mm, basis resolution (BR) = 88 mm × 88 mm, slice thickness (ST) = 2.5 mm, 42 axial slices, interleaved slice acquisition].
Anatomical images were acquired using a T1-weighted 3D magnetization-prepared, rapid acquisition gradient echo (MP-RAGE) pulse sequence (TR = 2300 ms, TE = 2.98 ms, time inversion = 900 ms, FA = 9°, VS = 1 mm × 1 mm × 1.2 mm, BR = 256 mm × 256 mm, ST = 1.2 mm, 160 sagittal slices).
The SSP as well as the ACQ statements were presented by means of the software “presentation” on a head mounted display inside the fMRI scanner. Possible visual defects were adjusted with appropriate lenses. The volume of the Stop-Signal was adjusted for every participant, so that each participant was able to hear the tone in a comfortable manner inside the scanner.
Statistical Analyses
Behavioral Data
Numerical data were analyzed using the software package PASW Statistics 18 (SPSS Inc, 2009) applying analyses of variance (ANOVA) with a within subject factor “condition” (A, N) and parameters of the SSP (RTs, errors, SSRTs in correct Stop- and Go-trials) and the two ACQ rating scores (pre and post SSP) as dependent measures. Moreover, in order to investigate whether the ratings in the ACQ stayed stable across time in the induction conditions, which would indicate that induction effects hold throughout SSP, paired T-tests were calculated between the ACQ ratings pre and post SSP for both conditions.
fMRI data
Functional data were analyzed with SPM5 (Wellcome Trust Centre for Neuroimaging, London, UK; http://www.fil.ion.ucl.ac.uk/spm) implemented in MATLAB 7 (The Mathworks Inc., Natick, MA, USA). Data were realigned, normalized into standard stereotactic space (Talairach coordinates), and smoothed with a Gaussian Kernel of 8 mm (full width half maximum). For each participant and each condition (A and N) the following events were modeled with a canonical hemodynamic response function (HRF): correctly responded Go-trials, successfully inhibited Stop-trials, incorrect Go-trials, and Stop-trials that were responded to.
For the Go-trials the onsets of the events were set to the time of the presentation of the respective onset stimulus. For the Stop-trials the event onsets were modeled at the time of the Stop-Signal due to the high variability of the SOA, hence ensuring a good coverage of activation related to individual response inhibition. Finally, for each subject contrast images were calculated and submitted to a second-level random effects analysis with a within subject factor “condition” referring to A and N assuming measures to be dependent, and a factor “trial type” referring to the contrasts of the respective Go- and Stop-trials assuming that these are independent measures.
As there is a lot of evidence on the relevant brain regions recruited for Go- and Stop-trials during the performance of the SSP (Rubia et al., 2001; Aron and Poldrack, 2006; Boecker et al., 2011) as well as regions intermingled in craving-associated processes (Miller and Goldsmith, 2001) we conducted specific region of interest (ROI) analyses to test whether the same brain areas would be involved during task performance in the participating subjects in the present investigation as reflected in similar activation patterns.
As reported in Aron and Poldrack (2006) the IFC (BA 47), the sub-thalamic nucleus (STN), the globus pallidus (GP; lateral, medial), and the motor cortex (MC; BA 4, 6) were chosen in the present study as relevant regions for inhibitory processes within the SSP as assessed with the Stop-trials. Based on the assumptions of the same authors the prefrontal cortex (PFC; BA 8, 9, 10, 11, 44, 45, 46, 47), the striatum (S), the GP (lateral, medial), the thalamus (TH), and the MC (BA 4, 6) were selected as areas relevant to response selection, i.e., regions that should be recruited during the Go-trials (Aron and Poldrack, 2006). In order to test whether these typical brain regions would be recruited during the SSP task performance we firstly analyzed the data looking at the total of Stop- and Go-trials across conditions (Stop_A&N; Go_A&N). Hereafter, we calculated the differential contrasts for successful Stop- and Go-trials between the conditions (Stop_A > N; Stop_N > A; Go_A > N, Go_N > A). In all analyses a conservative threshold of p < 0.05, corrected for multiple comparisons (FWE) and an extend threshold of >1 voxel were applied.
Moreover, to survey our third hypothesis the left and right limbic lobe as reported in Miller and Goldsmith (2001) served as ROI for the subsequent analyses. Here we chose to inspect the differential activation between the conditions (A > N; N > A) applying conjunction analyses (with p < 0.05, uncorrected on voxel level) including all Stop- and Go-trials in order to investigate activation associated with cue reactivity across the complete duration of task performance.
Altogether, all second level contrasts were calculated within these specific ROIs (described above) as defined by the aal-coordinates (Maldjian et al., 2003). Anatomical labeling provided in the tables was performed with help of the aal-coordinates provided by the WFU-Pickatlas (Maldjian et al., 2003).
Results
Behavioral Data
Exposure of alcohol and control conditions
The ANOVA showed a significant effect for the within subject factor “condition” [F(4) = 4.3; p < 0.05; ]. Figure 2 shows that patients indicate a significantly stronger urge to drink alcohol in the A condition as compared to the N condition in both items of the ACQ (all p < 0.05) before and after the SSP. Table 3 summarizes means, SDs, and p-values. Importantly, the results from a subsequent paired T-test showed that the answers before and after task performance within the conditions did not differ indicating that the specific exposure conditions remained stable over time (values of the paired T-tests are shown in Table 3).
Stop-signal paradigm
The ANOVA showed no significant effect for the within subject factor “condition” [F(9) = 1.3; p = 0.52; ]. Table 4 summarizes means, SDs, and p-values. There were neither significant error- nor significant RT differences in the correct Go-trials and the correctly responded Stop-trials (i.e., trials in which participants pressed the correct button despite the Stop-Signal) between the induction conditions (A and N). Importantly, the SSRT as well did not differ between conditions.
As the occurrence of other symptoms like anxiousness and depressive mood is frequent in patients with alcohol dependence we re-examined our results by including the BSI scores of the depression and anxiety dimensions and the GSI score as covariates in our analysis. This analysis served to look for effects of cue exposure conditions on inhibition performance while keeping the influences of other psychopathology constant. When introducing the BSI scores as covariates F-statistics reveal no significant effect of the factor condition on the SSRT [F(1,7) = 5.4; p = 0.053; ]. Hence including the covariates into the model did not change the over all pattern of results. Moreover, this analysis revealed that neither the BSI Depression score [F(1,7) = 0.00; p = 0.9; ], nor the BSI Anxiety score [F(1,7) = 0.33; p = 0.6; ] nor the BSI GSI [F(1,7) = 0.11; p = 0.7; ] had any significant effect on the inhibition performance (SSRT).
fMRI Data
Exposure of alcohol and control conditions
For A > N the conjunction analysis revealed significant differential activation in the left hippocampus (peak voxel at: x = −30, y = −14, z = −11) and the left amygdala (peak voxel at: x = −26, y = −7, z = −18) whereas the inverse contrast (N > A) showed the maximum activation in the posterior cingulate cortex (peak voxel at: x = −4, y = −24, z = 27; p < 0.05, uncorrected on voxel level, extend threshold >1 voxel). Table 5 summarizes all significant coordinates of the conjunction analyses.
Stop-signal paradigm: Stop_A&N, Go_A&N
Across both conditions the ROI analyses revealed the maximum activation within the IFG (BA 13; peak voxel at: x = 30, y = 20, z = 6) during the correct inhibited Stop-trials while the maximum activation was located in the precentral gyrus (BA 4) during the correct responded Go-trials (peak voxel at: x = −46, y = −13, z = 52; p < 0.05, FWE corrected, extend threshold >1 voxel). Table 6 summarizes all significant suprathreshold maxima. Figure 3 displays the distribution of activations for the respective contrasts.
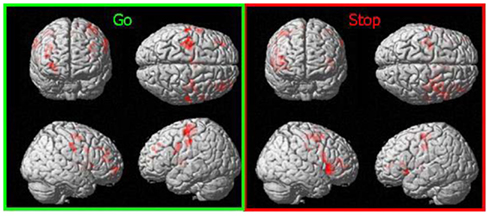
Figure 3. Activation during correct responded Go-trials and successfully inhibited Stop-trials across conditions; SPM5; ROI; p < 0.05, corrected for multiple comparisons (FWE).
Stop-signal paradigm: Go_A > N, Go_N > A, Stop_A > N, Stop_N > A
The differential contrasts between the conditions revealed neither for the correct responded Go-trials nor for the successfully inhibited Stop-trials any significant suprathreshold activation (p < 0.05, FWE corrected, extend threshold >1 voxel).
However, in order to account for the small behavioral effects of the induction as reported by the patients and to unravel even very small differential activation between the conditions in the Stop-trials, we also compared data between conditions for the Stop-trials on a more lenient threshold (p < 0.05 uncorrected with an extent threshold of >1 voxels). Applying this threshold revealed most significant differential activation within the IFG (BA 9) for the contrast A > N and within the GP for the inverse contrast (N > A). Table 7 summarizes all remaining suprathreshold activations and Figure 4 displays the distribution of activations for the respective post hoc contrasts.
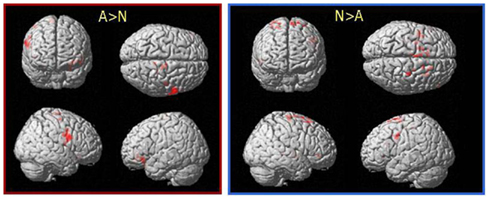
Figure 4. Differential activation between conditions during successfully inhibited Stop-trials; SPM5; ROI; p < 0.05, uncorrected on voxel level.
Discussion
The resource depletion model (Muraven and Baumeister, 2000) and the cognitive processing model of craving (Tiffany and Conklin, 2000) both postulate that effortful mental processes interfere with other cognitive tasks (e.g., response inhibition) when triggered by situations in which craving is induced and self-control is demanded. Based on these assumptions we examined the effect of an alcohol cue exposure on the ability of detoxified alcohol-dependent patients to inhibit their ongoing responses in a SSP and the associated neural activation.
The finding that alcohol-dependent patients report a stronger urge to drink alcohol when confronted with alcohol-related cues is in line with results reported in previous studies (Schneider et al., 2001; Muraven and Shmueli, 2006; Gauggel et al., 2010). Moreover, looking at the elicited neural activation during the alcohol exposure indicates that typical brain regions were triggered. Amygdala and hippocampus are both key structures within the limbic system, which in turn has been reported to orchestrate stress responses and reward-related aspects within drug abuse and craving processes by incorporating the anatomical requirements for successful accomplishment of emotional as well as motivational tasks (Rodriguez de and Navarro, 1998; Miller and Goldsmith, 2001; Heinz et al., 2010).
Cue reactivity is a learned response that connects a substance and the typical surroundings and context under which consumption takes place. On the neural level amygdala and hippocampus are recruited to correctly remember such situations and the emotions that are associated with the circumstances of drug consumption and experience (Schneider et al., 2001; Goldstein and Volkow, 2002; Weiss, 2005; Heinz et al., 2010). Our results are in accordance with the above assumptions underpinning that the participants were vividly reminded of the respective situation and that alcohol cue exposure involved conditioned emotional responses as mediated by the amygdala and hippocampus.
The finding that the neutral control condition was predominantly associated with elicited activation within the posterior cingulate cortex and not with activation of the amygdala or hippocampus further strengthened the discovery that solely the alcohol cue exposure elicited conditioned emotionally laden cue reactivity responses. While Goldstein and Volkow (2002) found the ACC to be associated with higher order motivational functions and attention processes, such as context dependent tracking, modulating, and updating certain values as a function of the expectation and ability to control and suppress behavior, the general heterogeneity of the functional associations of the cingulate cortex is long known (Vogt et al., 1992). For example, anatomically the anterior cingulate is reciprocally connected to the amygdala whereas the posterior cingulate cortex is not. Researchers describe the role of the ACC in intoxication and craving, and its deactivation during withdrawal but rarely embed the posterior part, a region involved in functions considered to be untypical for the limbic system, in the discussion (Goldstein and Volkow, 2002). More generally, the ACC has been described as a region serving executive functions whenever behavioral and neuroendocrine responses need to be controlled while it is suggested that the posterior part is predominantly involved in assessing context and memory rather than initiating behavioral processes (Vogt et al., 1992). Although the parts of the cingulate cortex certainly are not independent, one might speculate, that the activation found in our investigation during the alcohol–neutral exposure indeed reflects recruitment of environmental or context factors, namely such information that represent situations in which the patients would never drink alcohol, but that this condition did not evoke emotionally laden cue reactivity responses similar to the alcohol exposure.
In the study at hand we hypothesized according to the resource depletion model and according to assumptions made by the cognitive processing model of craving (Tiffany, 1999; Muraven and Baumeister, 2000; Tiffany and Conklin, 2000), that the conducted alcohol cue exposure should have resulted in diminished performances in the SSP in patients with alcohol dependence. On the neural level we therefore expected that albeit typical brain regions should be activated during task performance across conditions, a finding strengthening the premise that the paradigm effectively operated, differential neural activation should become evident during the Stop-trials predominantly within the IFC between the two exposure contexts.
On the behavioral level, we could not replicate the findings by Muraven and Shmueli (2006) or Gauggel et al. (2010) who found that, when confronted with alcohol-smell, the performance in the SSP in social drinkers and alcohol-dependent patients is impaired. The participating patient sample in our study did not show any differences between the performances during the alcohol cue exposure and the control condition. RTs, error rates, and most importantly SSRTs did neither differ in the Go- nor in the Stop-trials between conditions. Even when controlling for the influence of typical comorbidities in alcohol dependence, like depression and anxiety, the over all pattern of results did not change. Moreover, these findings were accompanied by only subtle differential neural activation in the postulated regions between the two exposure conditions, although across conditions the characteristic neural activation during task performance was elicited (Aron et al., 2003; Aron and Poldrack, 2006; Boecker et al., 2011). Even though the study was designed on the basis of successful experiments which suggested the postulated direction of results and although we believe we could improve the induction of conditions there might be several reasons for the discrepant findings.
First, it could be that the alcohol-dependent subjects could neither perform the task during the alcohol cue exposure nor the neutral condition. When comparing our behavioral results in both conditions with findings by Aron et al. (2003) our participants show longer RTs compared to a healthy sample of a similar age and similar RTs as patients with lesions of the right frontal lobe. Hence, it could be that the patients in our study display a floor effect, i.e., generally slow RTs under both conditions, which impeded an additional slowing of responses through our alcohol cue exposure. However, this argument remains speculative as no healthy control group was included in the study and other research, including our own investigations, found similar RTs in healthy and alcohol-dependent samples (Gauggel et al., 2010; Boecker et al., 2011). Hence, as shown in our previous investigation (Gauggel et al., 2010) we expected the differences between conditions to be strong enough to become evident in a within subject design – a presumption we could not substantiate with our investigation.
A second explanation why the RTs and SSRTs in the Stop-trials between alcohol and neutral cue exposure did not differ could be that the urge to drink alcohol has no influence on performance at all. Although this is a weak assumption as most research, including our own (Gauggel et al., 2010), found stable evidence for a close connection between craving-related processes and impaired (inhibition) performance (Noel et al., 2001, 2007; Fillmore, 2003; Kamarajan et al., 2005), there are studies reporting divergent findings (Bradizza et al., 1995; Townshend and Duka, 2007). For example Bradizza et al. (1995), trying to test Tiffany’s predictions that urges to drink alcohol would interfere with performance on cognitive demanding tasks, could not provide support for Tiffany’s assumptions. Moreover, Townshend and Duka (2007) found an avoidance of alcohol-related stimuli in alcohol-dependent inpatients in comparison to social drinkers. However, according to incentive salience theories (Robinson and Berridge, 1993) attentional orientation toward alcohol-related cues is an important conditioned response mediating drug-seeking in alcoholic subjects. Moreover, alcohol-relevant cues can increase attention toward alcohol-related stimuli interfering with the processing of other ongoing tasks (Cox et al., 1999). The authors interpreted these findings as evidence that patients become increasingly aware of their inability to control their drinking behavior during therapy leading to an attention withdrawal when confronted with alcohol-related stimuli rather than an attention bias toward the drug-related cues (Townshend and Duka, 2007). This assumption was further supported by the results found in the assessment of craving with a questionnaire providing scores on four factors of alcohol craving. Here, the patients rated their perceived “loss of control over drinking” higher and “mild desires and intentions to drink” lower compared to social drinkers (Townshend and Duka, 2007). Outgoing from the above assumptions one might speculate that our patients actively detracted their attention from the wish to consume alcohol hence leaving the performance unaffected between the conditions. This would go in line with the over all relatively low ratings on the urge to drink alcohol in the ACQ. The SSP is moreover a task which is quite attention consuming and might have facilitated a successful distraction from other processes.
Finally it is possible that the craving intensity or the urge to drink alcohol as elicited with our induction procedure was simply not strong enough to have a significant impact on task performance and thereby lead to different RT and SSRTs between the conditions. After all we do observe differences between the exposure conditions as reflected in a higher self-reported desire to drink in the items of the ACQ after alcohol cue exposure as well as in differential neural activation between the two conditions in brain areas relevant to craving-related processes. Moreover, when applying a more lenient threshold on the neural data for the SSP we find differential activation in the hypothesized brain network between conditions.
During alcohol cue exposure the Stop-trials revealed maximum differential activation within the rIFC which is known to play the key role in the ability to perform tasks where inhibition of ongoing responses is required. Numerous studies on healthy subjects and patients support this assumption (Rubia et al., 2001, 2003; Aron et al., 2003, 2004; Rieger et al., 2003; Aron and Poldrack, 2006; Chambers et al., 2006; Boecker et al., 2011).
The alcohol–neutral exposure on the contrary elicited maximum differential activation within the GP during the Stop-trials. This region is also known to be intermingled in inhibitory processes (Aron and Poldrack, 2006) a fact which was expected as we assumed the patients to try to inhibit their responses under both conditions. Looking at the neuroanatomical connections between regions involved in inhibitory processes initially the STN receives excitatory input from the frontal lobes (IFC; BA4, 6, 8; Mink, 1996; Aron and Poldrack, 2006). The GP as part of the basal ganglia receives excitatory output from its connection to the STN and further sends output projections to thalamus and brainstem (Mink, 1996). Interestingly, applying the more lenient threshold we also find differential activation of the thalamus during the alcohol–neutral condition and not during the alcohol cue exposure. It seems that looking at the neural network of motor response inhibition STN, GP, and thalamus are triggered subsequently after the IFC (Mink, 1996; Aron and Poldrack, 2006). This would support the view that in our patients, when confronted with alcohol-related cues, neuronal the inhibitory processes are affected at a very early level of processing compared to the control condition. One might speculate that the patients put more effort into successful response inhibition under alcohol cue exposure from the very beginning resulting in enhanced activation of the IFC to compensate possible impairments in subsequent mechanisms.
Functionally, it has been shown that a stimulation of the STN improves the SSRT in patients and that lesions of this region led to impaired performance (slower SSRTs; van den Wildenberg et al., 2006; Eagle et al., 2008). Moreover in a study by Aron and Poldrack (2006) the GP was activated during successfully inhibited Stop-trials but not during Stop-trials that were responded to. Both results argue for our finding that the neutral exposure condition during which we expected the patients to be more successful in inhibiting ongoing responses elicited a maximum of activation in the GP, i.e., in parts of the basal ganglia intermingled in successful inhibitory motor responses.
A review on the role of the basal ganglia in motor responses states that the basal ganglia broadly inhibit competing motor mechanisms thereby allowing actions to proceed without interference (Mink, 1996). When intended movement is generated, as for example through the presentation of an onset stimulus in the SSP, motor areas in the cerebral cortex send a signal to the STN leading to an excitation of the GP and a subsequent inhibition of motor pattern generators for competing motions. Moreover, it is described that depending on the movement and involved mechanisms, the number of concurring mechanisms may increase leading to progressive slowing of the actions (Mink, 1996). Hence when during the inhibition of an already initiated response resources are required through processes triggered by an alcohol cue exposure (adding even more competing mechanisms to the desired movement) this should have an impact on the RTs and SSRTs in the SSP and be neuronally reflected by aberrant activation of areas related to the basal ganglia as the GP. As we could not detect any behavioral deficits in our alcohol-dependent sample the subtle neural differences between induction conditions during the performance of the SSP are only coherent.
Altogether the above assumptions remain speculative. The study at hand suffers from some general limitations which could have led to the subtle results in comparison to other studies as the small sample size of patients with alcohol dependence, the absence of a healthy control group and general difficulties in the assessment of subjectively reported craving.
The chosen sample – as in most other investigations – certainly represents a specific population of patients with alcohol dependence showing a characteristic state of personality, severity, and duration of illness. Moreover, the state of detoxification might come along with social desirable responses on questions concerning the triggered urge to drink.
Another general limitation of the study is the absence of a healthy control group which could have helped to explain the diverging RT findings between the investigations of the SSP discussed above. Note however that the study by Gauggel et al. (2010) showed differences in RT between exposure conditions in the SSP in patients with alcohol dependence. This led to our assumption that the exposure conditions would affect task performance and that the effect of an alcohol cue exposure would be strong enough to cause this difference in patients with alcohol dependence rendering a control group unnecessary. A different stimulus selection and study surrounding might have caused that we could not replicate the findings of the preceding study. During the SSP the first investigation presented word stimuli whereas the study at hand asked the participants to respond to symbols instead. Additionally, the specific experimental setting in our investigation (i.e., lying in the scanner environment in comparison to sitting in front of a computer screen) could have contributed to an enhanced arousal or elicited alertness hence interfering with effective exposure procedures and task performances.
Finally, concerning common difficulties in the assessment of subjectively reported craving, it would be interesting to replicate the experiment including more physiological measures (e.g., measurements of heart beat, saliva, and skin conductance responses). This would strengthen findings gained from the subjective reports specifying the urge to drink that was elicited through a cue exposure.
All in all this study investigated the ability of alcohol-dependent patients to inhibit already initiated responses when confronted with alcohol-related cues. Moreover the neuronal correlates during task performance under cue exposure were examined. The subjectively stronger urge to drink was accompanied by activation of limbic brain regions during the alcohol cue exposure compared to the control condition. Moreover, during performance of the SSP typical brain regions were recruited across exposure conditions. The results hint to the direction that alcohol-dependent patients participating in the investigation at hand are able to compensate impairments in inhibitory control induced by an alcohol cue exposure.
Conflict of Interest Statement
The authors declare that the research was conducted in the absence of any commercial or financial relationships that could be construed as a potential conflict of interest.
References
Anton, R. F. (1999). What is craving? Models and implications for treatment. Alcohol Res. Health 23, 165–173.
Anton, R. F., Moak, D. H., and Latham, P. (1995). The Obsessive Compulsive Drinking Scale: a self-rated instrument for the quantification of thoughts about alcohol and drinking behavior. Alcohol. Clin. Exp. Res. 19, 92–99.
Aron, A. R. (2011). From reactive to proactive and selective control: developing a richer model for stopping inappropriate responses. Biol. Psychiatry 69, 55–68.
Aron, A. R., Behrens, T. E., Smith, S., Frank, M. J., and Poldrack, R. A. (2007). Triangulating a cognitive control network using diffusion-weighted magnetic resonance imaging (MRI) and functional MRI. J. Neurosci. 27, 3743–3752.
Aron, A. R., Fletcher, P. C., Bullmore, E. T., Sahakian, B. J., and Robbins, T. W. (2003). Stop-signal inhibition disrupted by damage to right inferior frontal gyrus in humans. Nat. Neurosci. 6, 115–116.
Aron, A. R., and Poldrack, R. A. (2006). Cortical and subcortical contributions to stop signal response inhibition: role of the subthalamic nucleus. J. Neurosci. 26, 2424–2433.
Aron, A. R., Robbins, T. W., and Poldrack, R. A. (2004). Inhibition and the right inferior frontal cortex. Trends Cogn. Sci. (Regul. Ed.) 8, 170–177.
Boecker, M., Drueke, B., Vorhold, V., Knops, A., Philippen, B., and Gauggel, S. (2011). When response inhibition is followed by response reengagement: an event-related fMRI study. Hum. Brain Mapp. 32, 94–106.
Bradizza, C. M., Lisman, S. A., and Payne, D. G. (1995). A test of Tiffany’s cognitive model of drug urges and drug-use behavior. Alcohol. Clin. Exp. Res. 19, 1043–1047.
Brown, J. M. (1998). “Self-regulation and the addictive behaviors,” in Treating Addictive Behaviors, eds W. R. Miller, and N. Heather (New York: Plenum Press), 61–73.
Chambers, C. D., Bellgrove, M. A., Stokes, M. G., Henderson, T. R., Garavan, H., Robertson, I. H., Morris, A. P., and Mattingley, J. B. (2006). Executive “brake failure” following deactivation of human frontal lobe. J. Cogn. Neurosci. 18, 444–455.
Cox, W. M., Yeates, G. N., and Regan, C. M. (1999). Effects of alcohol cues on cognitive processing in heavy and light drinkers. Drug Alcohol Depend. 55, 85–89.
Derogatis, L. R. (1993). The Brief Symptom Inventory (BSI): Administration, Scoring and Procedures Manual, 3rd Edn. Minneapolis, MN: National Computer System.
Derogatis, L. R., and Melisaratos, N. (1983). The Brief Symptom Inventory: an introductory report. Psychol. Med. 13, 595–605.
Eagle, D. M., Baunez, C., Hutcheson, D. M., Lehmann, O., Shah, A. P., and Robbins, T. W. (2008). Stop-signal reaction-time task performance: role of prefrontal cortex and subthalamic nucleus. Cereb. Cortex 18, 178–188.
Everitt, B. J., and Robbins, T. W. (2005). Neural systems of reinforcement for drug addiction: from actions to habits to compulsion. Nat. Neurosci. 8, 1481–1489.
Fillmore, M. T. (2003). Drug abuse as a problem of impaired control: current approaches and findings. Behav. Cogn. Neurosci. Rev. 2, 179–197.
Franke, G. H. (2000). Brief Symptom Inventory von L. R. Derogatis (Kurzform der SCL-90-R), Deutsche Version. Göttingen: Beltz Test GmbH.
Gauggel, S., Heusinger, A., Forkmann, T., Boecker, M., Lindenmeyer, J., Cox, W. M., and Staedtgen, M. (2010). Effects of alcohol cue exposure on response inhibition in detoxified alcohol-dependent patients. Alcohol. Clin. Exp. Res. 34, 1584–1589.
Gauggel, S., Rieger, M., and Feghoff, T. A. (2004). Inhibition of ongoing responses in patients with Parkinson’s disease. J. Neurol. Neurosurg. Psychiatr. 75, 539–544.
George, M. S., Anton, R. F., Bloomer, C., Teneback, C., Drobes, D. J., Lorberbaum, J. P., Nahas, Z., and Vincent, D. J. (2001). Activation of prefrontal cortex and anterior thalamus in alcoholic subjects on exposure to alcohol-specific cues. Arch. Gen. Psychiatry 58, 345–352.
Goldstein, R. Z., and Volkow, N. D. (2002). Drug addiction and its underlying neurobiological basis: neuroimaging evidence for the involvement of the frontal cortex. Am. J. Psychiatry 159, 1642–1652.
Grüsser, S. M., Wöfling, K., Düffert, S., Mörsen, C., and Flor, H. (2004). Psychometric properties and initial validation of the questionnaire on differentiated assessment of addiction. Psychother. Psychosom. Med. Psychol. 54, 405–412.
Heinz, A., Beck, A., Mir, J., Grüsser, S. M., Grace, A. A., and Wrase, J. (2010). “Alcohol craving and relapse prediction. Imaging studies,” in Advances in the Neuroscience of Addiction, Chap. 4. eds C. M. Kuhn, and G. F. Kroob1 (Boca Raton, FL: CRC Press), 137–162.
Kaernbach, C. (1991). Simple adaptive testing with the weighted up-down method. Percept. Psychophys. 49, 227–229.
Kamarajan, C., Porjesz, B., Jones, K. A., Choi, K., Chorlian, D. B., Padmanabhapillai, A., Rangaswamy, M., Stimus, A. T., and Begleiter, H. (2005). Alcoholism is a disinhibitory disorder: neurophysiological evidence from a Go/No-Go task. Biol. Psychol. 69, 353–373.
Logan, G. D. (1994). “On the ability to inhibit thought and action: a users’ guide to the stop-signal paradigm,” in Inhibitory Processes in Attention, Memory and Language, eds D. Dagenbach, and T. H. Carr (San Diego: Academic Press), 189–239.
Maldjian, J. A., Laurienti, P. J., Kraft, R. A., and Burdette, J. H. (2003). An automated method for neuroanatomic and cytoarchitectonic atlas-based interrogation of fMRI data sets. Neuroimage 19, 1233–1239.
Miller, N. S., and Goldsmith, R. J. (2001). Craving for alcohol and drugs in animals and humans: biology and behavior. J. Addict. Dis. 20, 87–104.
Mink, J. W. (1996). The basal ganglia: focused selection and inhibition of competing motor programs. Prog. Neurobiol. 50, 381–425.
Modell, J. G., Glaser, F. B., Cyr, L., and Mountz, J. M. (1992). Obsessive and compulsive characteristics of craving for alcohol in alcohol abuse and dependence. Alcohol. Clin. Exp. Res. 16, 272–274.
Muraven, M., and Baumeister, R. F. (2000). Self-regulation and depletion of limited resources: does self-control resemble a muscle? Psychol. Bull. 126, 247–259.
Muraven, M., and Shmueli, D. (2006). The self-control costs of fighting the temptation to drink. Psychol. Addict. Behav. 20, 154–160.
Muraven, M., Tice, D. M., and Baumeister, R. F. (1998). Self-control as limited resource: regulatory depletion patterns. J. Pers. Soc. Psychol. 74, 774–789.
Noel, X., Bechara, A., Brevers, D., Verbanck, P., and Campanella, S. (2010). Alcoholism and the loss of willpower: a neurocognitive perspective. J. Psychophysiol. 24, 240–248.
Noel, X., Paternot, J., Van der Linden, M., Sferrazza, R., Verhas, M., Hanak, C., Kornreich, C., Martin, P., De Mol, J., Pelc, I., and Verbanck, P. (2001). Correlation between inhibition, working memory and delimited frontal area blood flow measure by 99mTc-Bicisate SPECT in alcohol-dependent patients. Alcohol Alcohol. 36, 556–563.
Noel, X., Van der Linden, M., d’Acremont, M., Bechara, A., Dan, B., Hanak, C., and Verbanck, P. (2007). Alcohol cues increase cognitive impulsivity in individuals with alcoholism. Psychopharmacology (Berl.) 192, 291–298.
Oldfield, R. C. (1971). The assessment and analysis of handedness: the Edinburgh inventory. Neuropsychologia 9, 97–113.
O’Malley, S. S., Jaffe, A. J., Chang, G., Schottenfeld, R. S., Meyer, R. E., and Rounsaville, B. (1992). Naltrexone and coping skills therapy for alcohol dependence. A controlled study. Arch. Gen. Psychiatry 49, 881–887.
Paille, F. M., Guelfi, J. D., Perkins, A. C., Royer, R. J., Steru, L., and Parot, P. (1995). Double-blind randomized multicentre trial of acamprosate in maintaining abstinence from alcohol. Alcohol Alcohol. 30, 239–247.
Rankin, H., Hodgson, R., and Stockwell, T. (1983). Cue exposure and response prevention with alcoholics: a controlled trial. Behav. Res. Ther. 21, 435–446.
Rieger, M., Gauggel, S., and Burmeister, K. (2003). Inhibition of ongoing responses following frontal, nonfrontal, and basal ganglia lesions. Neuropsychology 17, 272–282.
Robinson, T. E., and Berridge, K. C. (1993). The neural basis of drug craving: an incentive-sensitization theory of addiction. Brain Res. Brain Res. Rev. 18, 247–291.
Rodriguez de, F. F., and Navarro, M. (1998). Role of the limbic system in dependence on drugs. Ann. Med. 30, 397–405.
Rubia, K., Overmeyer, S., Taylor, E., Brammer, M., Williams, S. C., Simmons, A., and Bullmore, E. T. (1999). Hypofrontality in attention deficit hyperactivity disorder during higher-order motor control: a study with functional MRI. Am. J. Psychiatry 156, 891–896.
Rubia, K., Russell, T., Overmeyer, S., Brammer, M. J., Bullmore, E. T., Sharma, T., Simmons, A., Williams, S. C., Giampietro, V., Andrew, C. M., and Taylor, E. (2001). Mapping motor inhibition: conjunctive brain activations across different versions of go/no-go and stop tasks. Neuroimage 13, 250–261.
Rubia, K., Smith, A. B., Brammer, M. J., and Taylor, E. (2003). Right inferior prefrontal cortex mediates response inhibition while mesial prefrontal cortex is responsible for error detection. Neuroimage 20, 351–358.
Scangos, K. W., and Stuphorn, V. (2010). Medial frontal cortex motivates but does not control movement initiation in the countermanding task. J. Neurosci. 30, 1968–1982.
Schneider, F., Habel, U., Wagner, M., Franke, P., Salloum, J. B., Shah, N. J., Toni, I., Sulzbach, C., Honig, K., Maier, W., Gaebel, W., and Zilles, K. (2001). Subcortical correlates of craving in recently abstinent alcoholic patients. Am. J. Psychiatry 158, 1075–1083.
SPSS Inc. (2009). PASW Statistics 18.0.0 [Computer Software]. Chicago, IL. Available at: www.spss.com
Tapert, S. F., Brown, G. G., Baratta, M. V., and Brown, S. A. (2004). fMRI BOLD response to alcohol stimuli in alcohol dependent young women. Addict. Behav. 29, 33–50.
Tapert, S. F., Cheung, E. H., Brown, G. G., Frank, L. R., Paulus, M. P., Schweinsburg, A. D., Meloy, M. J., and Brown, S. A. (2003). Neural response to alcohol stimuli in adolescents with alcohol use disorder. Arch. Gen. Psychiatry 60, 727–735.
Tiffany, S. T. (1990). A cognitive model of drug urges and drug-use behavior: role of automatic and nonautomatic processes. Psychol. Rev. 97, 147–168.
Tiffany, S. T., and Conklin, C. A. (2000). A cognitive processing model of alcohol craving and compulsive alcohol use. Addiction 95(Suppl. 2), 145–153.
Townshend, J. M., and Duka, T. (2007). Avoidance of alcohol-related stimuli in alcohol-dependent inpatients. Alcohol. Clin. Exp. Res. 31, 1349–1357.
van den Wildenberg, W. P., van Boxtel, G. J., van der Molen, M. W., Bosch, D. A., Speelman, J. D., and Brunia, C. H. (2006). Stimulation of the subthalamic region facilitates the selection and inhibition of motor responses in Parkinson’s disease. J. Cogn. Neurosci. 18, 626–636.
Vogt, B. A., Finch, D. M., and Olson, C. R. (1992). Functional heterogeneity in cingulate cortex: the anterior executive and posterior evaluative regions. Cereb. Cortex 2, 435–443.
Vollstädt-Klein, S., Loeber, S., Richter, A., Kirsch, M., Bach, P., von der Goltz, C., Hermann, D., Mann, K., and Kiefer, F. (2011). Validating incentive salience with functional magnetic resonance imaging: association between mesolimbic cue reactivity and attentional bias in alcohol-dependent patients. Addict. Biol. doi: 10.1111/j.1369-1600.2011.00352.x. [Epub ahead of print].
Volpicelli, J. R., Alterman, A. I., Hayashida, M., and O’Brien, C. P. (1992). Naltrexone in the treatment of alcohol dependence. Arch. Gen. Psychiatry 49, 876–880.
Weiss, F. (2005). Neurobiology of craving, conditioned reward and relapse. Curr. Opin. Pharmacol. 5, 9–19.
Wiers, R. W., Bartholow, B. D., van den Wildenberg, E., Thush, C., Engels, R. C., Sher, K. J., Grenard, J., Ames, S. L., and Stacy, A. W. (2007). Automatic and controlled processes and the development of addictive behaviors in adolescents: a review and a model. Pharmacol. Biochem. Behav. 86, 263–283.
World Health Organization. (1992). The ICD-10 Classification of Mental and Behavioral Disorders: Clinical Descriptions and Diagnostic Guidelines. Geneva: World Health Organization.
Keywords: cue exposure, inhibition, inferior frontal cortex, alcohol dependence
Citation: Mainz V, Drüke B, Boecker M, Kessel R, Gauggel S and Forkmann T (2012) Influence of cue exposure on inhibitory control and brain activation in patients with alcohol dependence. Front. Hum. Neurosci. 6:92. doi: 10.3389/fnhum.2012.00092
Received: 16 January 2012; Accepted: 30 March 2012;
Published online: 01 May 2012.
Edited by:
Patrizia Thoma, Ruhr-University Bochum, GermanyReviewed by:
Pierre Maurage, University of Louvain, BelgiumXavier Noel, Fonds de la Recherche Scientifique, Belgium
Copyright: © 2012 Mainz, Drüke, Boecker, Kessel, Gauggel and Forkmann. This is an open-access article distributed under the terms of the Creative Commons Attribution Non Commercial License, which permits non-commercial use, distribution, and reproduction in other forums, provided the original authors and source are credited.
*Correspondence: Verena Mainz, Institute of Medical Psychology and Medical Sociology, University Hospital of RWTH Aachen University, Pauwelsstr. 19, 52074 Aachen, Germany. e-mail:dm1haW56QHVrYWFjaGVuLmRl