- 1 Division of Mental Health and Addiction, Oslo University Hospital, Oslo, Norway
- 2 Institute of Clinical Medicine, University of Oslo, Oslo, Norway
- 3 Department of Psychiatry and Psychotherapy, Charité Universitätsmedizin, Berlin, Germany
- 4 Section of Neuroradiology, Department of Radiology and Nuclear Medicine, Oslo University Hospital, Oslo, Norway
- 5 Institute of Psychology, University of Oslo, Oslo, Norway
This study investigated the effect of arousal on short-term relational memory and its underlying cortical network. Seventeen healthy participants performed a picture by location, short-term relational memory task using emotional pictures. Functional magnetic resonance imaging was used to measure the blood-oxygenation-level dependent signal relative to task. Subjects’ own ratings of the pictures were used to obtain subjective arousal ratings. Subjective arousal was found to have a dose-dependent effect on activations in the prefrontal cortex, amygdala, hippocampus, and in higher order visual areas. Serial position analyses showed that high arousal trials produced a stronger primacy and recency effect than low arousal trials. The results indicate that short-term relational memory may be facilitated by arousal and that this may be modulated by a dose–response function in arousal-driven neuronal regions.
Introduction
Arousal is important for experiencing emotion (Russell and Barrett, 1999), and can be defined as giving intensity or individual strength to the emotion experienced (Hamann, 2003). High emotional arousal is suggested to facilitate encoding, consolidation, and retrieval of memory (Kensinger, 2009), with high arousal events being better remembered than neutral events (Brown and Kulik, 1977; Hamann, 2001). Although the effects of arousal on the neuronal system are complex, it is suggested that one effect of high arousal on memory are through activation of amygdala which then in turn modulates other neuronal regions for enhanced memory processing (LeDoux, 1995; McGaugh et al., 2002).
However, the effect of arousal has been debated with regard to combining different components of information to relational representations in memory (e.g., where you were when you heard the news). This is mainly due to divergent findings that indicate that high arousal can both facilitate (D’Argembeau and Van Der Linden, 2004; Hadley and MacKay, 2006; Kensinger et al., 2007; Mather and Nesmith, 2008) and impede (Mather et al., 2006; Mitchell et al., 2006; Cook et al., 2007) relational memory. Relational memory refers to the processing and retrieval of the relationship among components in memory (e.g., item and context; Prince et al., 2005; Davachi, 2006; Shimamura and Wickens, 2009). The neural substrates underlying relational memory are assumed to be the lateral prefrontal cortex (PFC; Blumenfeld et al., 2011), hippocampus (Konkel et al., 2008), and the superior parietal cortex (SPC; Wendelken et al., 2008). Arousal could affect the processing of relational memory through a neuronal emotional system, such as the amygdala (Hadley and MacKay, 2006). Amygdala may modulate hippocampus (McGaugh, 2002), and to this end may facilitate the processing of relational representations suggested to be supported by hippocampus. In addition, amygdala may also affect other cortical and subcortical regions (McGaugh et al., 2002), for instance by facilitating regions processing sensory and perceptual information, such as the fusiform gyrus (Barrett et al., 2007). High arousal could then facilitate processing of characteristics associated with an event by recruitment of higher order visual areas. In two functional magnetic resonance imaging (fMRI) studies (Mather et al., 2006; Mitchell et al., 2006), high arousal was associated with increased activity in the fusiform gyrus and the middle occipital gyrus during encoding of an object and its associated context in a short-term relational memory paradigm. In these studies however, relational memory were impeded by high arousal.
Easterbrook (1959) differentiated between the use of central and peripheral cues during high arousal in perception and attention. He proposed that as arousal increases, the utilization of peripheral cues (i.e., irrelevant cues) decreases. With respect to relational memory, irrelevant contextual information may not be adequately processed leaving a reduced relational memory for high arousing events (Kensinger, 2009). Recently, more specific hypotheses have been proposed to the underlying mechanisms of arousal and relational binding during encoding. Mather (2007) suggested that high arousal facilitates within-object binding at the cost of between-object binding. This could explain the enhanced effect of arousal on item-memory, but also why arousal may disrupt relational memory. Kensinger (2007, 2009) proposed that it is what is perceived as intrinsic details of an event that is facilitated by arousal, but not details perceived to be extrinsic. Thus, if the context of the high arousing object is important for the affective relevance, it is more likely to be perceived as intrinsic and then relational memory may be facilitated. Finally, MacKay and colleagues(MacKay and Ahmetzanov, 2005; Hadley and MacKay, 2006) suggested that high arousing objects elicit a binding mechanism that couples the source of the arousal (e.g., object) to the context it occurs subsequently facilitating relational memory.
Previous research and hypotheses regarding the role of arousal on relational memory has focused on the encoding phase and the relational binding of the different components. To what extent the same neuronal network reactivates during successful recognition are not known. It is suggested that central structures in relational memory such as hippocampus, activates both during formation and reactivates during recognition of established bindings of relational representations (Ranganath, 2010). The present study sought to investigate the neuronal processes underlying recognition phase of relational memory over a short retention interval during different levels of subjective arousal. Of particular interest were whether there was a dose-dependent response with arousal in these neuronal regions. In order to measure this, a picture by location paradigm based on Mather et al. (2006) was used.
Materials and Methods
Participants
Twenty-three healthy participants [age: mean (m) = 28, SD ± 7 years, 38% female] were recruited from the University of Oslo community via posters and received payment for their participation. All participants gave their informed consent according to the guidelines of the Norwegian Regional Ethics Committee. Participants were interviewed for MRI contra-indications, as well as current and previous somatic and psychiatric health. Exclusion criteria was a current or previous, serious somatic, or psychiatric illness. Participants were given a detailed description of the experiment and allowed to practice on a different stimuli-set until they were comfortable with the task outside the scanner. Data from two participants was discarded due to technical errors in registration of behavioral data. One participant’s data was discarded due to a technical error during one of the fMRI sessions.
Task
A trial started with the text “Ready,” presented for 500 ms. In the encoding epoch, four pictures with different locations on the screen were presented sequentially for 900 ms each. Subjects were instructed to remember each individual picture and the location on the screen. After the encoding epoch, a fixation cross was presented for 900 ms, followed by a screen with the word “test” for 500 ms. The test consisted of one of the pictures studied during encoding presented in either an old or new location. The task was to determine whether a given picture were associated with a given location. The memory test lasted for a maximum of 3000 ms (see Figure 1 for an illustration of the trial structure). The inter-trial interval was jittered by an average of 4000 ms (range 3000–5000 ms). The screen was divided into eight different locations from which the pictures (460 × 280 pixels) could emerge. The serial position of the pictures and the location on the screen were pseudo-randomized and counterbalanced. All the pictures and positions used during encoding were used as test probes for the duration of the experiment. All stimuli were presented onto a black screen with the text in magenta. The experiment was divided into two sessions. The stimuli were presented and controlled using E-prime software (Psychology Software Tools, Inc; Pittsburgh, PA, USA) and presented in the scanner using VisualSystem (NordicNeuroLab, Bergen, Norway).
Stimuli
Picture stimuli consisted of 96 pictures from the International Affective Picture System (IAPS; Lang et al., 1999). Pictures were selected on the basis of IAPS arousal and valence mean ratings to create three conditions of various levels of arousal (high, medium, and low arousal). The low arousal condition consisted of pictures with neutral valence ratings. The high and medium arousal condition consisted of pictures of high and medium negative valence ratings. There were 32 pictures in each condition. For each condition, the 32 pictures were randomly assigned to a picture-set containing four pictures. Thus, each condition had eight picture-sets. These picture-sets were replicated four times throughout the experiment. Each picture was presented in every serial position. The presentation of stimuli was randomized. The subjects rated the stimuli on valence and arousal after the experiment using a 10 point anchored scale.
MRI Data Acquisition
Participants were scanned at the Oslo University Hospital, Ullevål using a 3T GE Signa HDxt scanner (General Electric Medical Systems, Milwaukee, WI, USA) with an eight-channel head coil. Cushions were placed around the subject’s head to prevent motion. First, a high-resolution anatomical image was acquired using a T1-weighted GE fast spoiled gradient echo (FSPGR) Bravo (TR = 10.9 s; TE = 4.6 ms; FA = 13.0°; 236 axial slices 3D acquisition; 1.2 mm thick; 240 mm × 240 mm in plane resolution, 352 × 224 matrix). The functional runs were acquired with a T2*-weighted echo-planar imaging sequence sensitive to blood-oxygenation-level dependent (BOLD) contrast (TR = 2 s; TE = 25 ms; FA = 78°). Each volume consisted of 36 slices acquired approximately parallel to the AC–PC plane (interleaved acquisition; 3.5 mm thick with a 0.5 mm gap; 4 mm ×4 mm in plane resolution; 64 × 64 matrix).
Preparation of Behavioral Data
The subjects’ ratings of the stimuli were normalized to a Z-distribution and compared with IAPS normative valence material (Lang et al., 1999). If a subject’s rating of a picture’s valence diverged by more than two SD from IAPS norms, it was classified as a deviant rating. If a subject had more than 30% deviant ratings of the stimuli on the valence dimension the subject was classified as an outlier, as information from these subjects was evaluated to be less reliable (e.g., this could be pictures that were highly negative and the subjects rated them as positive). Three subjects were categorized as outliers and removed from further analysis.
The stimuli were divided into three conditions with an equal amount of trials based on the subject’s own arousal ratings (33 percentile). The conditions were: (1) high arousal [arousal: (m ± SD) = 8.02 ± 1.47; valence: 2.44 ± 1.75]; (2) medium arousal [arousal: 4.47 ± 1.65; valence: 4.23 ± 1.77]; (3) low arousal [arousal: 1.42 ± 0.83; valence: 5.36 ± 1.42]. Response times above three SD from an individual’s mean were removed from behavioral analyses. Accuracy is expressed as percent correct.
Analysis of fMRI Data
Spatial and temporal pre-processing was conducted using SPM5 (http://www.fil.ion.ucl.ac.uk/spm/). All functional runs were investigated for artifacts and extreme variance, and functional images were slice-time corrected and realigned to the first image in the series. No subject moved more than 3 mm in any direction. The anatomical image were spatially normalized to the Montreal Neurological Institute (MNI) template (Evans et al., 1993). The functional images were then normalized to the parameters established for the anatomical image. The functional images were re-sampled to 3 mm isotropic voxels and smoothed using an 8 mm full width half maximum kernel.
Blood-oxygenation-level dependent responses were specified as stick functions convolved with a synthetic hemodynamic response function. For each session the model included regressors for trials with high, medium, and low arousal. For each level of arousal both the start of encoding and the retrieval epoch were specified, while analysis was carried out for the retrieval epoch correct trials only. In addition, the model included regressors for erroneous trials and the six movement regressors obtained from the realignment. Second level random effects repeated measures general linear model (GLM) was conducted on contrast images from individual data on high arousal, medium arousal, and low arousal. Analyses for the main effect and linearity were performed using voxelwise statistics [the threshold was set to p false discovery rate corrected (FDR) < 0.001 and cluster size greater than 10 contiguous voxels]. One sample t-tests were then conducted using voxelwise statistics on individual contrast images for the contrast [high arousal > low arousal] with a threshold set to pFDR = 0.05 and cluster size greater than 10 contiguous voxels. A priori regions of interest were based on functional areas previously defined at the ventrolateral prefrontal cortex (vlPFC) [−40 20 −2], the dorsolateral PFC (dlPFC) [−42 28 24], and the posterior precuneus [−6 −70 60; 12 −74 54] (Lundstrom et al., 2005). The fusiform gyrus and the middle occipital gyrus were defined from Mather et al. (2006) at [41 −51 −13] and [45 −70 9], respectively. To define areas in the opposite hemisphere, the sign was switched for the x coordinate. The regions were defined by spheres with a radius of 10 mm centered on the coordinates. The bilateral hippocampus and amygdala were analyzed with masks created from the SPM anatomy toolbox (Amunts et al., 2005). Regions of interest analyses were performed using small volume correction (SVC) thresholded at pFDR < 0.05. In order to control for valence partial correlations were performed between the calculated local percent signal change in peak voxels and each subject’s mean arousal per condition while controlling for mean valence.
Results
Behavioral Results
A GLM repeated measure revealed no significant difference in response times among conditions [high arousal: (m ± SD) 1191 ± 153 ms; medium arousal: 1190 ± 146 ms; low arousal: 1208 ± 179 ms; F(2,32) = 0.38, p = n.s.]. Furthermore, there were no significant differences for accuracy for arousal [high arousal: 83 ± 7%; medium arousal: 82 ± 10%; low arousal: 81 ± 9%; F(2,32) = 1.192, p = n.s.].
Stimuli to be remembered presented in a sequential fashion are known to generate serial position effects, with a facilitation of memory for items present early (primacy effect) and late (recency effect) in a sequence. As this may be due to the distinctiveness of these items, we performed further analyses to investigate whether arousal (also affecting distinctiveness) could interact with the presentation of the stimuli. A three (arousal) by four (serial position) repeated measures analysis was conducted. This yielded a significant interaction effect for accuracy [F(6,96) = 2.70, p < 0.05; see Table 1 and Figure 2 for an overview]. To evaluate the serial position effect we performed a GLM repeated measure for each level of arousal and position. Accuracy yielded a significant serial position effect for high arousal [F(3,48) = 12.55, p < 0.001], medium arousal [F(3,48) = 8.08, p < 0.001], and low arousal [F(3,48) = 4.45, p < 0.01]. All levels of arousal yielded a recency effect for correct responses. The low arousal trials showed a steady increase in accuracy from the first to the last serial position. The high arousal trials showed an enhanced recency effect, but also a primacy effect. A paired sample t-test showed that difference between high and low arousal were significant for the first [t(16) = 2.88, p < 0.05] and final serial position [t(16) = 3.16, p < 0.01].
fMRI Results
General linear model repeated measures for correct > error across all conditions in the regions of interest were performed to rule out that the activations were not just an effect of viewing high arousal pictures (see Table 2 for an overview). Activations in amygdala and hippocampus were obtained for correct trials compared to errors independent of arousal condition. Error analyses for the different arousal conditions were not possible due to too few observations.
General linear model repeated measures analysis using a whole brain search showed significant effects related to change in arousal level (see Table 3). Test for linearity revealed that several regions showed significant dose-dependent relationships between arousal and BOLD-response (see Table 4 and Figure 3). Post hoc t-tests in the regions of interest (Table 5) showed that the bilateral fusiform gyrus, bilateral middle occipital gyrus, right posterior precuneus, bilateral amygdala, bilateral hippocampus, and bilateral vlPFC demonstrated a stronger activation in high arousal trials as compared to low arousal trials. No significant activations in dlPFC were obtained.
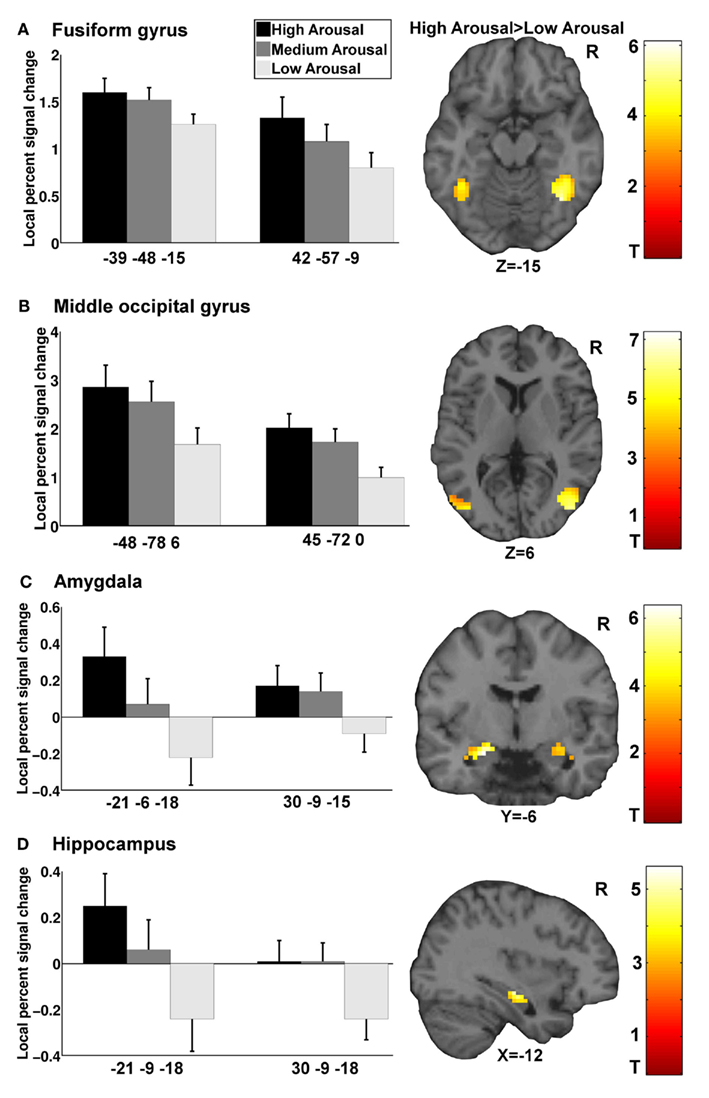
Figure 3. Functional magnetic resonance imaging results for arousal-driven regions of interest. (A–D) displays from left to right dose-dependent modulation of arousal (bargraphs represent local percent signal change extracted from peak voxels in GLM test for linearity) and activation pattern for high arousal compared to low arousal (images are explicitly masked and t-value > 3 for illustrative purposes).
Partial Correlations
There were significant correlations between local percent signal change and arousal in the bilateral amygdala (left: r = 0.30, p < 0.05; right = 0.35, p < 0.05), right hippocampus (r = 0.32, p < 0.05), left fusiform gyrus (r = 0.29, p < 0.05), right middle occipital gyrus (r = 0.31, p < 0.05), and posterior precuneus (r = 0.37, p < 0.01) after controlling for valence. To test whether the regions that did not show associations between the signal change and level of arousal could be better explained by valence, we performed the same analysis with these regions while controlling for arousal level. The results showed that there were no significant correlations between the signal change and valence when controlling for arousal.
Follow-Up Analyses
To investigate whether there were serial positions effects that paralleled the behavioral results for high and low arousal trials in the functional data, we performed a GLM repeated measure on extracted averaged signal change from the regions of interest. None of the regions of interest showed alterations in BOLD-response that paralleled the behavioral findings.
Analyses of the Encoding Phase
For the analyses of the encoding phase a separate model were specified with duration of encoding as a block. A one sample t-test comparing high arousal > low arousal for the encoding phase revealed significant activations for both a whole brain search (pFDR < 0.05, cluster size > 10) and within some of our regions of interest (SVC pFDR < 0.05; see Table 6). The region of interest analyses displayed significant activations in bilateral fusiform gyrus, bilateral middle occipital gyrus, and bilateral amygdala.
Discussion
The present study shows that level of subjective experienced emotional arousal modulates higher order visual areas, lateral PFC, amygdala, and hippocampus in a dose–response manner. Amygdala and hippocampus appeared to mediate short-term relational memory performance. Relational memory performance was facilitated, with both a primacy and a recency effect for high arousal trials. These results do indicate that high subjective arousal facilitates short-term relational memory, but depends on the order of the encoding of the stimuli.
The effect of arousal on relational memory is especially interesting due to the observed variability in performance across previous studies. The theoretical discussion has centered on to what extent associated information are adequately processed during high arousal, and to what extent this information is perceived as relevant or central for the task. In the current paradigm, where the subjects were informed that both picture and location were relevant, there was a facilitation of relational memory in high arousal trials. The present results may in addition emphasize some methodological issues. Arousal is an important aspect of experiencing emotion, and provides individual strength and intensity to the experienced emotion (Hamann, 2003). Research using pictures and words as stimuli to manipulate arousal has to some extent used population or group based averaged ratings of arousal. Although this has several benefits, it may mask the individual experience of arousal. One could argue that using subjective ratings of arousal is beneficial to elucidate the behavioral effects and the neuronal underpinnings of arousal.
There was no main effect of arousal for the behavioral measures. One reason for this may be that the different arousal conditions were randomly presented at a relatively short inter-trial interval within the experiment. Previous research has shown that presentation of high arousal stimuli may interfere with memory for stimuli in temporal proximity (Hurlemann et al., 2005). Thus, one cannot rule out the possibility that presenting the different arousal conditions in separate blocks could provide different results. However, in a similar paradigm as the present experiment, Mather and Nesmith (2008) found enhanced picture by location memory for high arousal trials independent of whether presentation were in blocks of arousal or randomly distributed throughout the experiment. The obtained behavioral effect of arousal on short-term relational memory in the present study depended on the order of the encoding of the stimuli. Laboratory studies of memory often use sequential presentation of the stimuli to be remembered. Memory for information presented in the beginning and at the end of sequential presentations is often facilitated, which is referred to as the primacy- and recency-effect (serial position effects; Murdock, 1962). These effects are suggested to be due to the distinctiveness of the serial position in which the stimuli occurs (Neath, 1993). For visual short-term memory in general, the primacy effect is not as reliable produced, while the recency effect may be critically dependent on the distinctiveness of the stimuli (Hay et al., 2007). Our data fits well with these effects, where trials with low arousal demonstrate a recency effect and no primacy effect. More importantly, trials with high arousal show a primacy effect and an increased recency effect compared to low arousal trials. The obtained effects for high arousal indicate that these trials have additional distinctiveness, facilitating relational memory. The obtained serial position effects emphasize not only the importance of experimental control, but also that these effects may be to the very core of explaining how high arousal could affect memory processes. Analyses for serial position effects in our regions of interest did not show alterations in the BOLD-response that paralleled the behavioral findings which showed that memory performance depended on the order of the encoding of the stimuli. The analyses of the encoding phase showed an increased BOLD-response for the high arousal trials compared to low arousal trials in bilateral amygdala, fusiform gyrus, and middle occipital gyrus. The finding that arousal modulates hippocampus during retrieval, does however suggest a partial retrieval explanation. The error analysis does support this as correct trials compared to erroneous trials displayed activity in hippocampus and amygdala. Due to the short interval between the encoding and the retrieval phase, identifying how arousal affects encoding and retrieval are difficult and to this end represents a limitation to the current study. For instance, it is difficult to dissect whether the obtained activation of amygdala during retrieval is purely sustained activity from the encoding phase or if it supports memory processes during retrieval in conjunction with hippocampus.
While arousal describes the intensity of an emotion, a second dimension, valence, to a greater extent describes the quality of the emotion (e.g., positive, neutral, or negative emotion). Although arousal and valence are highly correlated and difficult to disentangle, they may impact memory and cognition differently (Hamann, 2003; Kensinger, 2009). So, to isolate the effect of arousal on cognitive processes, it may be important to control for valence. The present study used statistical techniques to this end, and highlighted some arousal-driven neuronal regions composed by amygdala, hippocampus, and higher order visual areas. These regions could then be important in understanding how arousal affects relational memory.
The present findings partly replicate Mather et al. (2006) and Mitchell et al. (2006), insofar as high arousal trials are associated with activation in the fusiform gyrus and the middle occipital gyrus. The activations in the fusiform gyrus obtained in the present study can be interpreted as a modulation of attention and object processing by arousal. In keeping with the above finding, the present results also indicate a significant role for processing of contextual information, with activation of precuneus observed for high arousal trials compared to low arousal trials. The SPC is important for spatial processing, but several studies indicate it may play a more general role in processing relations between components (Lundstrom et al., 2005; Cavanna and Trimble, 2006). Wendelken et al. (2008) suggest the dlPFC and the SPC compose a fronto-parietal network involved in short-term relational memory. In their model the dlPFC manipulates and organizes the relational information, while the SPC serves as a substrate to which the spatial relations are held and manipulated. Our results do not indicate that activity in the dlPFC is modulated by arousal, but the activation of SPC (precuneus) could indicate that high arousal trials recruits increased spatial processing.
The amygdala has traditionally been implicated in the processing of fear, and more recently has been found to be related to other facets of emotion (e.g., disgust and positive emotion) as well (Zald, 2003). It has been proposed that amygdala might serve a more general cognitive function such as relevance detection (Davis and Whalen, 2001; Sander et al., 2003; Ousdal et al., 2008), processing novel stimuli, and signaling importance (Weierich et al., 2010). The experience of arousal does encapsulate relevance and importance, and might explain the relationship between the amygdala and arousal. The present results indicate that both amygdala and hippocampus shows a dose-dependent response to increasing levels of arousal. Activation of amygdala may facilitate normal memory processes in a dose-dependent manner through modulation of the hippocampus (Hamann, 2001; McGaugh, 2002; Sander et al., 2003; Kensinger, 2004; Lupien et al., 2007). One function of hippocampus is suggested to support the binding of different components in memory such as an object and where this object was located (Ranganath, 2010). Furthermore, the amygdala may also modulate other cortical and subcortical regions (Barrett et al., 2007; Weierich et al., 2010); high levels of arousal activate the amygdala, which then modulates the neural sensitivity of perceptual regions such as the fusiform gyrus (Barrett et al., 2007). The activations of the fusiform gyrus and inferior temporal gyrus in conjunction with the middle occipital gyrus and precuneus could make perceptual details for both picture and location more available, rendering accurate relational memory decisions easier during high arousal. This is in accordance with the original source memory framework which suggested that perceptual details are important in deciding on the source of a memory trace (Johnson et al., 1993).
In conclusion, the present study indicates that the recognition of pictures and their respective locations after short retention intervals is facilitated by arousal. This advantage appears to be due to some arousal-driven regions composed of the amygdala, hippocampus, and higher order visual processing areas. Recruitment of these regions may enhance the processing of object properties, their spatial relations, and the combination of these components.
Conflict of Interest Statement
The authors declare that the research was conducted in the absence of any commercial or financial relationships that could be construed as a potential conflict of interest.
Acknowledgments
This study was supported by a grant from the South-Eastern Norway Regional Health Authority. The authors thank Greg Reckless for help with preparation of the manuscript.
References
Amunts, K., Kedo, O., Kindler, M., Pieperhoff, P., Mohlberg, H., Shah, N. J., Habel, U., Schneider, F., and Zilles, K. (2005). Cytoarchitectonic mapping of the human amygdala, hippocampal region and entorhinal cortex: intersubject variability and probability maps. Anat. Embryol. (Berl.) 210, 343–352.
Barrett, L. F., Bliss-Moreau, E., Duncan, S. L., Rauch, S. L., and Wright, C. I. (2007). The amygdala and the experience of affect. Soc. Cogn. Affect. Neurosci. 2, 73–83.
Blumenfeld, R. S., Parks, C. M., Yonelinas, A. P., and Ranganath, C. (2011). Putting the pieces together: the role of dorsolateral prefrontal cortex in relational memory encoding. J. Cogn. Neurosci. 23, 257–265.
Cavanna, A. E., and Trimble, M. R. (2006). The precuneus: a review of its functional anatomy and behavioural correlates. Brain 129, 564–583.
Cook, G. I., Hicks, J. L., and Marsh, R. L. (2007). Source monitoring is not always enhanced for valenced material. Mem. Cognit. 35, 222–230.
D’Argembeau, A., and Van Der Linden, M. (2004). Influence of affective meaning on memory for contextual information. Emotion 4, 173–188.
Davachi, L. (2006). Item, context and relational episodic encoding in humans. Curr. Opin. Neurobiol. 16, 693–700.
Easterbrook, J. A. (1959). The effect of emotion on cue utilization and the organization of behavior. Psychol. Rev. 66, 183–201.
Evans, A. C., Collins, D. L., Mills, S. R., Brown, E. D., Kelly, R. L., and Peters, T. M. (1993). “3d statistical neuroanatomical models from 305 MRI volumes,” in Proceedings of the Institute of Electrical and Electronics Engineering-Nuclear Science Symposium and Medical Imaging, London, 3, 1813–1817.
Hadley, C. B., and MacKay, D. G. (2006). Does emotion help or hinder immediate memory? Arousal versus priority-binding mechanisms. J. Exp. Psychol. Learn. Mem. Cogn. 32, 79–88.
Hamann, S. (2001). Cognitive and neural mechanisms of emotional memory. Trends Cogn. Sci. (Regul. Ed.) 5, 394–400.
Hay, D. C., Smyth, M. M., Hitch, G. J., and Horton, N. J. (2007). Serial position effects in short-term visual memory: a SIMPLE explanation? Mem. Cognit. 35, 176–190.
Hurlemann, R., Hawellek, B., Matusch, A., Kolsch, H., Wollersen, H., Madea, B., Vogeley, K., Maier, W., and Dolan, R. J. (2005). Noradrenergic modulation of emotion-induced forgetting and remembering. J. Neurosci. 25, 6343–6349.
Johnson, M. K., Hashtroudi, S., and Lindsay, D. S. (1993). Source monitoring. Psychol. Bull. 114, 3–28.
Kensinger, E. A. (2004). Remembering emotional experiences: the contribution of valence and arousal. Rev. Neurosci. 15, 241–251.
Kensinger, E. A. (2007). Negative emotion enhances memory accuracy: behavioral and neuroimaging evidence. Curr. Dir. Psychol. Sci. 16, 213–218.
Kensinger, E. A., O’Brien, J. L., Swanberg, K., Garoff-Eaton, R. J., and Schacter, D. L. (2007). The effects of emotional content on reality-monitoring performance in young and older adults. Psychol. Aging 22, 752–764.
Konkel, A., Warren, D. E., Duff, M. C., Tranel, D. N., and Cohen, N. J. (2008). Hippocampal amnesia impairs all manner of relational memory. Front. Hum. Neurosci. 2:15. doi:10.3389/neuro.09.015.2008
Lang, P. J., Bradley, M. M., and Cuthbert, B. N. (1999). The International Affective Picture System (IAPS): Technical Manual and Affective Ratings. Gainesville, FL: University of Florida.
Lundstrom, B. N., Ingvar, M., and Petersson, K. M. (2005). The role of precuneus and left inferior frontal cortex during source memory episodic retrieval. Neuroimage 27, 824–834.
Lupien, S. J., Maheu, F., Tu, M., Fiocco, A., and Schramek, T. E. (2007). The effects of stress and stress hormones on human cognition: implications for the field of brain and cognition. Brain Cogn. 65, 209–237.
MacKay, D. G., and Ahmetzanov, M. V. (2005). Emotion, memory, and attention in the taboo Stroop paradigm. Psychol. Sci. 16, 25–32.
Mather, M. (2007). Emotional arousal and memory binding: an object-based framework. Pers. Psychol. Sci. 2, 33–52.
Mather, M., Mitchell, K. J., Raye, C. L., Novak, D. L., Greene, E. J., and Johnson, M. K. (2006). Emotional arousal can impair feature binding in working memory. J. Cogn. Neurosci. 18, 614–625.
Mather, M., and Nesmith, K. (2008). Arousal-enhanced location memory for pictures. J. Mem. Lang. 58, 449–464.
McGaugh, J. L. (2002). Memory consolidation and the amygdala: a systems perspective. Trends Neurosci. 25, 456–461.
McGaugh, J. L., Mcintyre, C. K., and Power, A. E. (2002). Amygdala modulation of memory consolidation: interaction with other brain systems. Neurobiol. Learn. Mem. 78, 539–552.
Mitchell, K. J., Mather, M., Johnson, M. K., Raye, C. L., and Greene, E. J. (2006). A functional magnetic resonance imaging investigation of short-term source and item memory for negative pictures. Neuroreport 17, 1543–1547.
Neath, I. (1993). Distinctiveness and serial position effects in recognition. Mem. Cognit. 21, 689–698.
Ousdal, O. T., Jensen, J., Server, A., Hariri, A. R., Nakstad, P. H., and Andreassen, O. A. (2008). The human amygdala is involved in general behavioral relevance detection: evidence from an event-related functional magnetic resonance imaging Go-NoGo task. Neuroscience 156, 450–455.
Prince, S. E., Daselaar, S. M., and Cabeza, R. (2005). Neural correlates of relational memory: successful encoding and retrieval of semantic and perceptual associations. J. Neurosci. 25, 1203–1210.
Ranganath, C. (2010). A unified framework for the functional organization of the medial temporal lobes and the phenomenology of episodic memory. Hippocampus 20, 1263–1290.
Russell, J. A., and Barrett, L. F. (1999). Core affect, prototypical emotional episodes, and other things called emotion: dissecting the elephant. J. Pers. Soc. Psychol. 76, 805–819.
Sander, D., Grafman, J., and Zalla, T. (2003). The human amygdala: an evolved system for relevance detection. Rev. Neurosci. 14, 303–316.
Shimamura, A. P., and Wickens, T. D. (2009). Superadditive memory strength for item and source recognition: the role of hierarchical relational binding in the medial temporal lobe. Psychol. Rev. 116, 1–19.
Weierich, M. R., Wright, C. I., Negreira, A., Dickerson, B. C., and Barrett, L. F. (2010). Novelty as a dimension in the affective brain. Neuroimage 49, 2871–2878.
Wendelken, C., Bunge, S. A., and Carter, C. S. (2008). Maintaining structured information: an investigation into functions of parietal and lateral prefrontal cortices. Neuropsychologia 46, 665–678.
Keywords: amygdala, arousal, fMRI, hippocampus, relational memory, serial position effect, short-term memory
Citation: Thoresen C, Jensen J, Sigvartsen NPB, Bolstad I, Server A, Nakstad PH, Andreassen OA and Endestad T (2012) Arousal modulates activity in the medial temporal lobe during a short-term relational memory task. Front. Hum. Neurosci. 5:177. doi: 10.3389/fnhum.2011.00177
Received: 12 June 2011; Paper pending published: 04 September 2011;
Accepted: 14 December 2011; Published online: 03 January 2012.
Edited by:
Tor Wager, Columbia University, USAReviewed by:
Bradford C. Dickerson, Harvard Medical School, USATom Johnstone, University of Reading, UK
Copyright: © 2012 Thoresen, Jensen, Sigvartsen, Bolstad, Server, Nakstad, Andreassen and Endestad. This is an open-access article distributed under the terms of the Creative Commons Attribution Non Commercial License, which permits non-commercial use, distribution, and reproduction in other forums, provided the original authors and source are credited.
*Correspondence: Christian Thoresen, Research Fellow, Oslo University Hospital, Kirkeveien 166, Bygg 49, 0407 Oslo, Norway. e-mail: christian.thoresen@ous-hf.no