- 1 Neurobiology Department, Institute of Life Sciences, Interdisciplinary Center for Neural Computation, Hebrew University, Jerusalem, Israel
- 2 Edmond and Lily Safra Brain Research Center, Hebrew University, Jerusalem, Israel
Binocular rivalry (BR) occurs when the brain cannot fuse percepts from the two eyes because they are different. We review results relating to an ongoing controversy regarding the cortical site of the BR mechanism. Some BR qualities suggest it is low-level: (1) BR, as its name implies, is usually between eyes and only low-levels have access to utrocular information. (2) All input to one eye is suppressed: blurring doesn’t stimulate accommodation; pupilary constrictions are reduced; probe detection is reduced. (3) Rivalry is affected by low-level attributes, contrast, spatial frequency, brightness, motion. (4) There is limited priming due to suppressed words or pictures. On the other hand, recent studies favor a high-level mechanism: (1) Rivalry occurs between patterns, not eyes, as in patchwork rivalry or a swapping paradigm. (2) Attention affects alternations. (3) Context affects dominance. There is conflicting evidence from physiological studies (single cell and fMRI) regarding cortical level(s) of conscious perception. We discuss the possibility of multiple BR sites and theoretical considerations that rule out this solution. We present new data regarding the locus of the BR switch by manipulating stimulus semantic content or high-level characteristics. Since these variations are represented at higher cortical levels, their affecting rivalry supports high-level BR intervention. In Experiment I, we measure rivalry when one eye views words and the other non-words and find significantly longer dominance durations for non-words. In Experiment II, we find longer dominance times for line drawings of simple, structurally impossible figures than for similar, possible objects. In Experiment III, we test the influence of idiomatic context on rivalry between words. Results show that generally words within their idiomatic context have longer mean dominance durations. We conclude that BR has high-level cortical influences, and may be controlled by a high-level mechanism.
Introduction and Review of Conflicting Evidence Regarding Site of Binocular Rivalry
At any given moment our brains are busy with many tasks, including: receiving sensory information, regulating autonomous behavior, planning voluntary movements, and building memory of objects and events. Only some of these functions involve consciousness, and we are unaware of most brain activity.
Binocular rivalry (BR) has the unique property that, while physical stimuli remain constant, subjective perception changes. While two images are presented to the visual system and both are (at least partially) processed, the observer is only aware of one. Thus, BR is considered “perhaps the present most important experimental approach to finding the neural correlate of consciousness” (NCC; Crick and Koch, 1998). BR has been reviewed in more general contexts (Tong, 2003; Blake and Wilson, 2011).
Levels of Perceptual Representation
It is well known that there is a hierarchy of visual information processing (Hubel and Wiesel, 1962). Information from the eye enters, via the thalamus, low cortical levels (V1, V2, etc.) where simple features (such as lines of a specific orientation and location) are represented. Feed-forward processing leads to categorical representations, without details, at high cortical levels. Recent work by Hochstein and Ahissar (2002) suggests that there may be a reverse hierarchy of explicit visual perception. Visual information first travels in the bottom-up direction, because input from lower levels is necessary to construct a global representation, but this process may be implicit, and unavailable to consciousness. It was suggested that conscious perception begins with high-level categorical representations of the global scene. According to this reverse hierarchy theory (RHT), only later do we become aware of scene details, by reverse hierarchy return to lower level smaller receptive fields.
Binocular Rivalry
Binocular rivalry occurs when the brain cannot fuse the images seen by the two eyes because they are two completely different pictures or contain elements that differ in one or more features or attributes such as: color, orientation, size, velocity of motion, direction of motion, or depth (Blake and Logothetis, 2002). After an initial 150–200 ms, during which perception is of the two views, superimposed (Wolfe, 1996), perception alternates between two percepts. This alternation presumably reflects competition between the images for conscious perception (Crick, 1996; Logothetis, 1998). Alternations continue about every 2–3 s, as long as both stimuli are continuously viewed. Usually the two percepts correspond to the stimuli in each eye separately. A histogram of the time intervals (t) that one of the stimuli is dominant is well fit by the gamma distribution (Fox and Hermann, 1967; Papathomas et al., 1999; but see Rubin and Hupe, 2005) with two parameters λ, r:

To obtain fluctuation of entire pictures (exclusive rather than piecemeal rivalry), the stimuli must subtend no more than about 1 square degree for foveal targets (Blake et al., 1992; Kovács et al., 1996). One eye’s view may be dominant more often or for longer periods than that of the other, as occurs for figures that are brighter, in higher contrast, moving, or of higher spatial frequency (Levelt, 1965; Blake and Logothetis, 2002). It has been suggested that strengthening one stimulus shortens its suppression durations without affecting its dominance times (Levelt, 1965; Fox and Rasche, 1969). Finally, there is a long-standing debate (dating back to Helmholtz and Hering) concerning the impact of voluntary attention or cognitive salience on dominance times (see Ooi and He, 1999; Meng and Tong, 2004; Chong et al., 2005; Paffen et al., 2006; see also Toppino, 2003; van Ee et al., 2005).
Locus of Binocular Rivalry
Related to the issue of voluntary control, there is ongoing controversy regarding the low or high-level site of the BR mechanism. Supporting evidence for low-level mechanisms, include the following:
1. The fact that BR is usually a competition between monocular images suggests that the competition takes place at low cortical levels at or before the site of the transition from monocular to binocular representation; higher level cortical areas do not generally have utrocular information.
2. Basic stimulus attributes that are represented at low cortical levels affect rivalry (see above; Levelt, 1965).
3. Suppression operates to “non-selectively weaken all inputs to the suppressed eye,” which is “sufficient to compromise, but not abolish, visual performance” (Blake and Logothetis, 2002). Suppression “erases” or “blocks” processing of stimuli to that eye (perhaps similar to “early selection” in attention; Broadbent, 1958; Deutsch and Deutsch, 1963; Johnston and Heinz, 1979). Together with suppressing one eye’s image, detection of a probe to that eye is also reduced; blurring the picture does not stimulate the accommodation reflex; pupilary constrictions (in response to light flashes) are reduced in amplitude; and high-level adaptation effects such as the global motion after effect are reduced, though the tilt and local motion after effects remain (Lehmkuhle and Fox, 1975; Wade and Wenderoth, 1978; O’Shea and Crassini, 1981; Blake et al., 2006).
4. There is no visual priming due to suppressed words or pictures (Zimba and Blake, 1983), but priming with a certain direction of motion during suppression can bias the direction seen in a following ambiguous apparent motion sequence (Blake et al., 1998; see also Hock et al., 1996).
5. Semantic content has not been found to influence probe detection during rivalry suggesting that rivalry occurs before semantic content is extracted (Blake, 1988). However, it was recently found that a word related to a previously binocularly primed word “comes out” of suppression faster (in a flash-suppression paradigm; Costello et al., 2009). See also the new results presented later in this paper.
On the other hand, based on recent research, it seems that there are also high-level effects in BR:
1. Attention. Voluntary attention to one stimulus may increase its relative prominence, but not “save” it altogether from being suppressed and pop-out cues to one eye (initiating involuntary attention) can force a stimulus out of suppression (Ooi and He, 1999; Chong et al., 2005).
2. Patchwork rivalry. Kovács et al. (1996; see also Alais et al., 2000) used novel stimuli where two pictures were divided between the two eyes so that in order to see a coherent picture, information from the two eyes had to be integrated. Indeed the most prevalent percept was of coherent pictures, not of pictures deriving from one eye, suggesting that rivalry is mainly a high-level effect where competition is between integral percepts. Lee and Blake (1999) reject this conclusion, claiming that even low-level eye rivalry can be or patchy (as generally found for large stimuli; Blake et al., 1992). Kovács et al. (1996; see also Papathomas et al., 1999) would presumably respond that the choice of pieces which form a coherent picture would have to be high-level, proving at least a top-down influence on the rivalry mechanism. In a recent paper, Lee and Blake (2004) again challenge this interpretation, showing that swapping the dominant image to the other eye causes the percept to change; indicating that it was the eye that was dominant in that patch and not the percept. In addition, a simulation of independent regions of eye rivalry creates a dominance pattern that is similar to patchwork rivalry, demonstrating that patchwork rivalry can be explained without rejecting eye-based rivalry. Nevertheless, these authors do not deny a top-down mechanism may play a role in choosing the interocular grouping.
3. Context. When one of the rivaling stimuli is embedded in a congruent (high-level) context, its dominant periods are shorter (Carter et al., 2004) or longer (without a concomitant shortening of the suppression periods; Blake and Logothetis, 2002; Sobel and Blake, 2002).
4. Global effects. Even for large targets where piecemeal rivalry occurs, there are also periods of exclusive dominance (significantly more than chance), where the whole percept is monocular (Blake et al., 1992). Similarly, when small identical rival targets are distributed throughout the visual field they tend to fluctuate together.
5. Meaningful Content. A cognitively more salient stimulus, i.e., one with more “meaningful content,” remains dominant for longer periods (Walker, 1978; e.g., an upright face: Engel, 1956 see also Zhou et al., 2010; an emotional face: Alpers and Gerdes, 2007; Bannerman et al., 2008; a recognizable figure: Yu and Blake, 1992; a familiar figure: Lo Sciuto and Hartley, 1963; Goryo, 1969; Jiang et al., 2007). The implication of a high-level mechanism has been questioned, however, because the methods used for these studies were subjective, with a bias that could have affected the response rather than the percept, and because a low-level configuration detection mechanism could have affected both low-level rivalry and high-level meaning comprehension (Yu and Blake, 1992; Blake, 2000).
6. Swapping. When gratings were rapidly swapped between the eyes (at 1–3 Hz), observers report normal BR alternation rates (0.3–0.5 Hz), indicating that the pattern, not the eye, was dominant (pattern rivalry; Logothetis et al., 1996; van Boxtel et al., 2008). Following this finding, Wilson (2003) models rivalry as having both low-level and high-level mechanisms. However, Lee and Blake (1999) suggest that rapid swapping may interfere with “normal” rivalry mechanisms. More recent studies show that there actually is some rapid eye-related alternation; with the percentage of time that pattern rivalry is perceived as opposed to eye rivalry depending on the coherence of the stimuli (Bonneh et al., 2001; Silver and Logothetis, 2007).
7. Partial Rivalry. In an amazingly prescient paper, Treisman (1962) found that when each eye was shown a different colored circle (red/green) that was displaced relative to a surrounding circle, observers experienced BR between the two colored circles, in depth. The disparity information was taken into account even while the stimulus was suppressed. Superimposed orthogonal drifting gratings are perceived as a single plaid surface moving in the direction of the vector sum of the two movements. But when the two gratings are presented one to each eye, rivalry ensues. For patches small enough to ensure exclusive rivalry (0.8°), only one grating is perceived at any moment but it moves in the combined direction (Andrews and Blakemore, 1999). For larger patches, too, when one experiences piecemeal rivalry, the mosaic moves coherently, and again in the combined direction (Andrews and Blakemore, 2002). Thus, the suppressed (non-perceived) grating contributes to the perceived direction of motion (see also Alais and Parker, 2006). One may conclude that different neurons mediate rivalry of different features (motion, contour, color).
8. Rubin (2003) suggested that alternations between percepts of ambiguous figures have similar characteristics to BR alternations; (see also Carter and Pettigrew, 2003; van Ee, 2005; van Boxtel et al., 2008). Since ambiguous figure alternations are essentially between high-level interpretations, this similarity suggests that BR, too, may depend on a high-level mechanism.
Physiological Investigations
Physiological studies have also been used to find the site of rivalry alternation and the neural loci where activity corresponds to the conscious percept rather than to the presence of a physical stimulus. These investigators were often seeking hints at the site of the NCC but their results may tell us where to look for the alternation mechanism. These studies used a number of methodologies, including the following:
1. VEP. Using temporal tagging/labeling for two images presented to the two eyes, Brown and Norcia (1997) found correspondence between activity in the occipital cortex and perceptual changes. But this recording cannot separate between different visual areas in the brain. Studies using MEG found extended network responses modulated, but not extinguished by rivalry (Tononi et al., 1998).
2. Single-cell recordings. Leopold and Logothetis(1996; see summary Logothetis, 1998) studied alert monkeys as they viewed rivalry stimuli passively, or reported percept fluctuations. The dominance pattern was similar to that in humans. No evidence was found for any inhibition in the Lateral Geniculate Nucleus. Some of the neurons in striate cortex (20%) and early extrastriate cortex (V4, MT; 40%) showed activity modulations that corresponded to the perceptual changes, but not to the all-or-none extent experienced perceptually. In inferior temporal (IT) cortex, most of the neurons (90%) were active only when their preferred stimulus was consciously perceived – i.e., as reported by the monkey. Activity usually ceased when the preferred stimulus was suppressed, though some neurons acted in the opposite manner. Thus, area IT seems to be located at or beyond the point where the rivalry conflict is resolved.
3. Functional imaging. fMRI activity was measured in early visual cortex while subjects viewed rivaling gratings of different contrasts (Polonsky et al., 2000). Activity in V1 increased when the higher contrast was perceived and decreased when the lower contrast was perceived. These fluctuations were 55% as large as when the gratings physically alternated. The same results were found for V2, V3, V3a, and V4v. Tong et al. (1998) used pictures of a face and a house, presented respectively to each of the two eyes, and found modulations of brain activity in the Fusiform Face Area and Posterior Parietal Area that coincided with the fluctuations in the reported percept and were equal in strength to those reported when the stimuli were physically alternating, suggesting rivalry is resolved at or before these areas. It is difficult to resolve whether there is a build-up of rivalry along the hierarchy or a high-level mechanism whose results are fed back. More conclusively, Tong and Engel (2001) cleverly measured responses in the blind spot which produces a monocular region of human V1 and found fMRI modulations during rivalry which were as large as those evoked by physically alternating stimuli, suggesting that rivalry might be resolved by early interocular competition. However, supporting the above single-cell results, Brouwer et al. (2009) recently found intraparietal sulcus (IPS) sensitivity to the degree of perceptual incongruence of an ambiguous image, perhaps suggesting that this area may play a role in signaling the need to reinterpret ambiguous depth cues in the visual scene, initiating bistable perception. Similarly, Kanai et al. (2010) recently found that individual BR differences may depend on their different superior parietal lobe activations.
4. TMS. Related to the above finding, Zaretskaya et al. (2010) used TMS to individually disrupt processing in areas where fMRI activity correlated with rivalry alternations. They found that TMS over right IPS prolonged periods of stable percepts and that the more lateralized the IPS blood oxygen level-dependent signal, the more lateralized were the TMS effects. This suggests a causal, destabilizing, and individually lateralized effect of normal IPS function on perceptual continuity in rivalry, consistent with an IPS role in selection, related to its role in attention.
5. Localized brain damage. While much has been learned in the past from studies of deficits following localized brain damage, this avenue has not been utilized extensively for the study of BR. One exception is the study by Valle-Inclán and Gallego (2006) of a patient who had most of the prefrontal cortex disconnected from the rest of the brain due to a bilateral frontal leukotomy. Results indicated that prefrontal cortex is unnecessary for perceptual alternations during BR.
Summary
Thus, debate is still open regarding the locus of BR. A recently suggested and commonly held “solution” to these opposing views is that both high- and low-level mechanisms control rivalry, with local stimuli inducing alternations at lower cortical sites and complex configurations inducing high-level perceptual interpretations at high cortical areas (Rubin, 2003; Wilson, 2003, 2005; Alais and Blake, 2005; Rubin and Hupe, 2005; Tong et al., 2006). However, there may be an essential problem with this solution. What determines if the low-level mechanism initiates rivalry? If it always does, why don’t we see its impact in those cases that suggest a high-level mechanism (patchwork rivalry, swapping, etc.)? And why do high-level features (such as context) affect rivalry? On the other hand, rapid access of partial information to high-level areas before processing in early stages has been completed, may explain such phenomena (see e.g., Rousselet et al., 2002). In addition, RHT (see above; Hochstein and Ahissar, 2002) predicts that high-level mechanisms guide and feedback to low cortical levels, so that (albeit pushing this argument to its extreme), high-level attention (see Chong et al., 2005) may affect even a low-level BR mechanism, or, alternatively – and more likely – a high-level rivalry mechanism may have effects at lower levels.
Since complex images and scenes are represented as such at higher cortical levels, we investigate in the following sections the impact of varying high-level characteristics of the two eyes’ views, and propose that if these variations affect rivalry, it would support high-level intervention in BR, i.e., a high-level mechanism or at least a top-down effect in rivalry. We study BR with words and compare dominance durations for words and non-words. The only difference between these stimuli is high-level. Thus, any difference in rivalry pattern between words and non-words would indicate a high-level mechanism.
Dominance Durations when Words Compete with Non-Words
Introduction and Methods
We study rivalry between words and non-words (i.e., strings of letters without meaning, or pronounceable nonsense words) to investigate whether high-level semantic meaning affects the time spent with each type of input.
Subjects were 11 university students (age 19–35, mean 24), naïve to the purpose of the experiment, remunerated for participation, tested for normal or corrected-to-normal vision in both eyes, with good stereovision and no strabismus.
Stimuli were superimposed semitransparent 4° × 4° diagonal texts, one in green and one in red, viewed through red/green filter glasses so that the stimuli were seen as equally salient black and each eye saw one text, as demonstrated in Figure 1. A black frame and black fixation cross, seen by both eyes, promoted fusion. Viewing distance was 57 cm. Texts were in Hebrew, the main language of the subjects. In our presentations, as is general in Hebrew, vowels are assumed and not explicitly written, and non-words are usually pronounceable. Words (44 in all) were common (average 0.2/thousand) short (3.2 letters), nouns (59%), verbs (11%), and simple adjectives (25%) with few cases of polysemy (9%) or homography (11%). Non-words were created using the same letters as the words. Since Hebrew words depend on a 3-letter root, changing letter order creates a totally new – and usually unrecognizable – non-word. Words were in print font and non-words in script (or vice versa), allowing responses without explicit reference to text meaningfulness. Stimuli were counterbalanced between print and script font and between red and green color. Words (and non-words) were not repeated within trials.
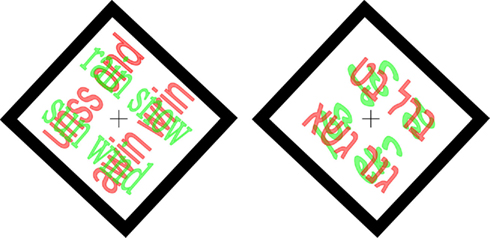
Figure 1. Examples of word-non-word rivaling stimuli. Left English text (rain snow sun wind vs. unss ond awin wrin – pronounceable non-words); Right Hebrew text – as actually used in Experiment 1, since subjects were Hebrew speakers; text in print vs. script font.
Subjects were asked to maintain fixation throughout the trial, and initiated trials by pressing the space-bar. There were 24 trials/session, each of 90 s during which the stimulus did not change. Subjects reported perceptual changes by briefly pressing one of three keys: PRINT (the “p” key), SCRIPT (“i”), or MIXED (“o”; subjects were instructed to indicate PRINT or SCRIPT as long as the stimulus was predominantly such).
Results
The mean dominance time for non-words was longer than for words, 3.03 ± 0.08 vs. 2.58 ± 0.06 s; p < 0.0001 (unpaired two-tailed t-test); dominance times for print and script were not significantly different: 2.78 ± 0.06 vs. 2.83 ± 0.07 s; p = 0.6, as demonstrated in Figure 2. Furthermore, for each subject, the mean dominance time was longer for non-words than for words (or in two cases nearly identical), as shown in the scatter plot of Figure 3 comparing average dominance times for each of the 11 subjects. Nearly all points lie above the diagonal of equality. Across-subject mean is 4.5 ± 1 vs. 3.7 ± 0.9 s; p < 0.02 (paired two-tailed t-test). Comparing these word-non-word competition data with dominance times for words presented to both eyes and for non-words presented to both eyes, we found an indication for competition leading to shortening of dominance times for words, rather than lengthening for non-words.
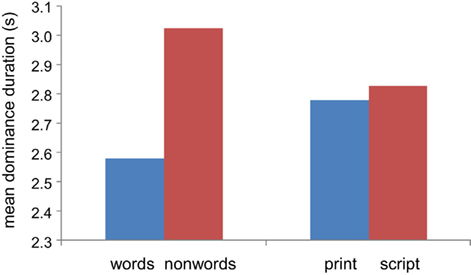
Figure 2. Dominance durations. Mean dominance duration for non-words is greater than for words; no difference between print and script font. Pooled results for all subjects.
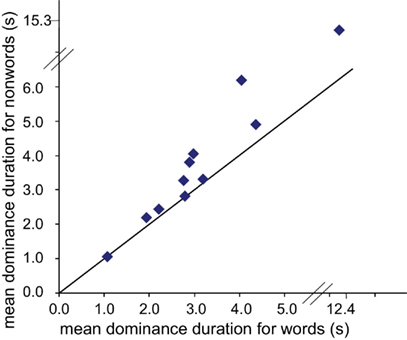
Figure 3. Scatter plot showing mean dominance durations of non-words vs. words for each subject. All points are on or above the equivalence line: mean dominance durations for non-words were longer than for words. Note one observer had considerably longer dominance durations, plotted on an extended scale.
Dominance Durations when Possible Figures Compete with Impossible Figures
Introduction and Methods
Having found a difference between dominance times for non-words vs. words, we asked if this difference might be more general, extending to other images beyond those of written words, in particular real, structurally possible figures compared with impossible figures. This would be consistent with previous findings that displeasing stimuli have an advantage over pleasing images (Smets, 1975).
Methods were as above, with two quite similar line drawings superimposed, one possible and one impossible, one in red and one in green, as demonstrated in Figure 4. Trials lasted 60 s, 20/session, 17 subjects (age 20–36; mean 25) reported their percept by pressing one of two keys (e.g., “j” for triangle pointing up; “f” for pointing down). We used two kinds of pictures: “simple” including: forks (possible and impossible), triangles (possible 3-D triangle and Penrose triangle; Draper, 1978), and squares (possible 3-D vs. Penrose-like square); and “complex” including Esher-type drawings (Thro, 1983) and similar possible objects: houses, windowpanes, wheels, and cubes. In each trial, both pictures were either simple or complex.
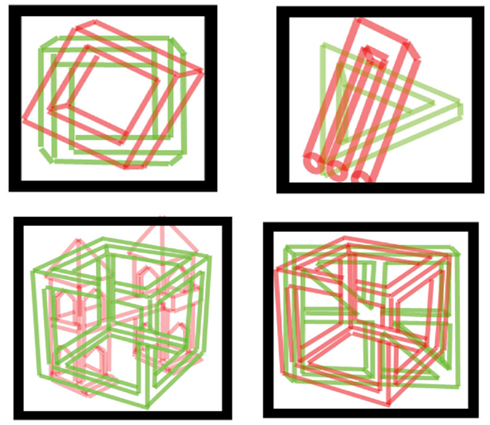
Figure 4. Examples of stimuli used for possible vs. impossible figures. Simple and complex figures (top and bottom row, respectively) were used. Subjects viewed pictures through red/green filtered glasses.
Results
For simple figures, impossible figures were dominant for longer times. Mean dominance durations for impossible and possible figures were 2.8 ± 0.2 vs. 2.5 ± 0.2 s; p < 0.02 (paired two-tail t-test), as shown in Figures 5 and 6. On the other hand, for complex figures, dominance times were considerably longer, with no difference between figures: 3.4 ± 0.3 vs. 3.4 ± 0.4 s; p = 0.93. These results are confirmed in the scatter plots of Figure 6, where the points for the simple impossible figures fall above the diagonal line of equivalence, but not the points for the complex figures.
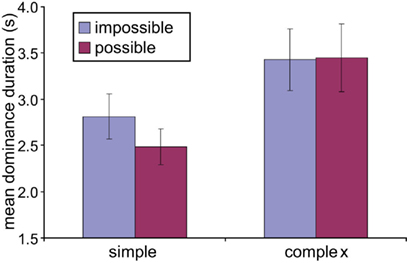
Figure 5. Dominance durations – possible vs. impossible figures. Across-subject mean dominance durations for possible and impossible figures for the different classes of images. Simple impossible figures have longer dominance durations than possible figures, with no difference for complex figures.
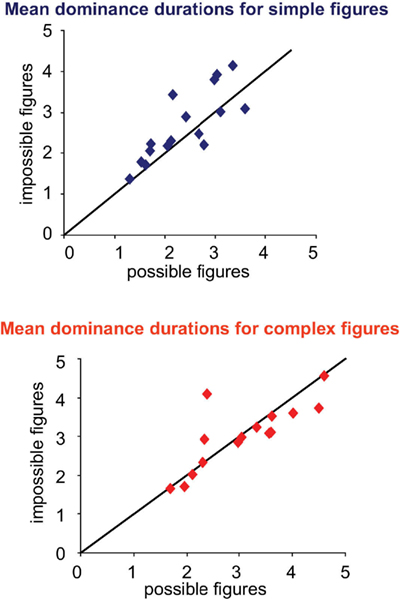
Figure 6. Mean dominance duration scatter plots by subject for simple (top) and complex (bottom) figures. For simple figures, most points are above the equality line indicating longer dominance durations for impossible figures. For complex figures, points are mostly close to the equality line.
A possible explanation for this difference is that with simple figures, impossible ones are more intriguing, but for complex figures, even the possible figures were complicated enough that they were as interesting as the impossible figures, and/or their dominance times reached some ceiling of dominance time. Previous studies showed that processing structurally possible and impossible figures takes place at high-level cortical regions (Schacter et al., 1995) and that only these levels may be used for differentiating percepts of possible vs. impossible objects.
Effect of Idiomatic Context on Dominance Duration
Introduction and Methods
Having found that semantic content affects BR dominance, we tested semantic context. Will words imbedded in a congruent context be more salient than words incongruent with that context, increasing the dominance times? Perhaps while nonsense words attract more – longer – attention due to the variety of ways they may be read (so that subjects may be experimenting with them (consciously or unconsciously) trying to figure out a meaningful way to pronounce them, words out-of-context are easily read and their nonsense context easily rejected, so that perhaps no extra time will be spent with incongruent texts. Context effects have been tested for low-level stimuli (e.g., Sobel and Blake, 2002; Carter et al., 2004), but what about high-level context? What constitutes congruent context for words? We used well known short (Hebrew) idioms.
Stimuli were constructed so that with colored stimuli and glasses (as above) one eye viewed a well known short idiom and the other a modified idiom where one word was replaced with a different word (chosen to fit grammatically and to have an equal frequency in Hebrew; see http://word-freq.mscc.huji.ac.il/index.html). For example, idiom: Mipnay seiva takum (translation: Rise for the elderly); modified idiom: Mipnay hakara takum (translation: Rise for the frost); (Similar to idiom: a stitch in time saves nine; modified “idiom”: a stitch in time waits nine).
Stimulus size was 3.5 × 3.5 cm viewed at 76 cm. See example in Figure 7. Trials lasted 60 s. Subjects tracked their percept by pressing one of three keys, “print,” “script,” or “mixed.” They were asked to adopt a constant criterion and, press “print” or “script” even if the percept was not exclusively so.
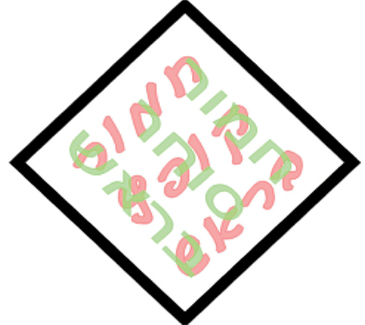
Figure 7. Example of stimulus for Experiment 3. Note that the idiom is in script font, the non-idiom in print.
Results
Real idioms were dominant for longer periods than modified texts. Words in-context had greater predominance than words not-in-context. Figure 8 shows mean dominance durations for individual subjects, and across-subject means for the two conditions. For almost all observers, mean dominance duration in-context was longer than when not-in-context; mean in-context 5.3 ± 0.5 s; out-of-context 4.7 ± 0.4 s; p < 0.01, paired t-test.
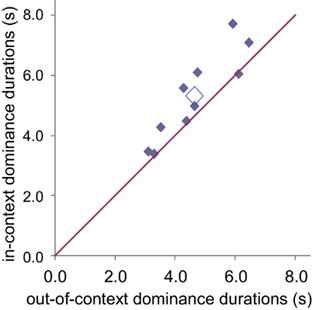
Figure 8. Mean dominance durations of in-context vs. out-of-context idiom texts, for each subject (filled diamonds) and across-subject means (open diamond). In-context dominance duration is generally longer than out-of-context duration.
General Discussion
We found a series of high-level effects in BR. The only difference between the stimuli presented to the two eyes was their cognitive semantic content. Nevertheless, there was a significant difference in the dominance periods of the two images.
In the context of the issue of the low or high cortical level of the mechanism controlling BR, these stimuli differed only in semantic content so that a low-level mechanism would treat them equally, while, at higher cortical levels, one could be preferred. In each pair, one stimulus was a group of possible words and the other impossible words composed of the same letters (Experiment 1), one was a structurally possible figure and the other impossible (Experiment 2), or one was a proper idiom and the other an idiom with a non-appropriate word interjected (Experiment 3). If the mechanism controlling BR were low-level, there should be no difference between non-words and words, between possible and impossible figures, or between words in- or out-of-context and we should find balanced rivalry (as with horizontal vs. vertical gratings). On the other hand, a high-level mechanism would be expected to distinguish between the two, and favor the more “interesting” image.
In fact, an effect in either direction would have indicated a high-level effect. We actually expected words to predominate over non-words and objects over non-objects, due to their being more familiar, containing meaning, and perhaps a “better unit” object or word in the Gestalt sense. Surprisingly, the results indicate otherwise. Non-words and impossible objects seem to be more interesting and attract longer scrutiny (see also Bonneh et al., 2001; Mudrik et al., 2011). Importantly, these results indicate that high-level interest overpowers more simple and direct familiarity.
Previous studies that attempted to differentiate between low- and high-level rivalry mechanisms (reviewed in Introduction and Review of Conflicting Evidence Regarding Site of Binocular Rivalry) used binocular effects such as patchwork images (Kovács et al., 1996) or flicker and switch stimuli (Logothetis et al., 1996) to demonstrate non-eye-related percepts and high-level image-related percepts. Others looked for manipulations that influence rivalry dominance times, such as contrast or context. Contrast would seem to indicate a low-level mechanism, context a high-level one. We now add a new approach, using high-level differences between stimuli and their impact on rivalry dominance times to demonstrate that these, too, depend on high-level effects.
The indication of a shortening of the dominance times for words when competing with non-words coincides with Levelt’s famous second law that the more salient stimulus has longer dominance times because of the shortening of its suppression times. While extension of non-word dominance durations could derive from an attentional effect, shortening of non-word suppression periods more definitively suggests a high-level BR control mechanism1. Similar results were found by Ooi and He (1999) who manipulated attention during BR and by van Ee et al. (2007) who found a dominance dependence on simultaneous auditory stimuli. In addition, Sobel and Blake (2002) and Carter et al. (2004) found that the context surrounding simple rivalrous stimuli affects their dominance phases. The results of these authors, demonstrating an attentional or contextual involvement in rivalry, also support high-level control of dominance times. Our own new results, taken together with those of these prior studies, provide substantial support for the conclusion that rivalry is controlled or at least affected by high-level mechanisms.
Though we may not have solved the entire mystery of which part of the brain is responsible for the switching in BR, we have demonstrated a new set of stimuli for which one may be easily convinced that it is a high-level mechanism that decides which stimulus is dominant. This finding is consistent with RHT (Hochstein and Ahissar, 2002), which suggests that high cortical level effects are the first to enter conscious perception. Thus, a switching mechanism located here will have rapid effects on perception and then gradually travel down the hierarchy – in reverse order – affecting lower cortical level responses as well.
Conflict of Interest Statement
The authors declare that the research was conducted in the absence of any commercial or financial relationships that could be construed as a potential conflict of interest.
Footnote
- ^We thank an anonymous reviewer for suggesting this line of reasoning.
References
Alais, D., O’Shea, R. P., Mesana-Alais, C., and Wilson, G. (2000). On binocular alternation. Perception 29, 1437–1445.
Alais, D., and Parker, A. (2006). Independent binocular rivalry processes for motion and form. Neuron 52, 911–920.
Alpers, G. W., and Gerdes, A. B. M. (2007). Here is looking at you: emotional faces predominate in binocular rivalry. Emotion 3, 495–506.
Andrews, T. J., and Blakemore, C. (1999). Form and motion have access to consciousness. Nat. Neurosci. 2, 405–406.
Andrews, T. J., and Blakemore, C. (2002). Integration of motion information during binocular rivalry. Vision Res. 42, 301–309.
Bannerman, R. L., Milders, M., De Gelder, B., and Sahraie, A. (2008). Influence of emotional facial expressions on binocular rivalry. Ophthalmic Physiol. Opt. 28, 317–326.
Blake, R. (1988). Dichoptic reading: the role of meaning in binocular rivalry. Percept. Psychophys. 44, 133–141.
Blake, R. (2000). A primer on binocular rivalry, including current controversies. Brain Mind 2, 5–38.
Blake, R., O’Shea, R. P., and Mueller, T. J. (1992). Spatial zones of binocular rivalry in central and peripheral vision. Vis. Neurosci. 8, 469–478.
Blake, R., Tadin, D., Sobel, K., Raissian, T., and Chong, S. C. (2006). Strength of visual adaptation depends on visual awareness. Proc. Natl. Acad. Sci. U.S.A. 103, 4783–4788.
Blake, R., Yu, K., Lokey, M., and Norman, H. (1998). Binocular rivalry and motion perception. J. Cogn. Neurosci. 10, 46–60.
Bonneh, Y., Sagi, D., and Karni, A. (2001). A transition between eye and object rivalry determined by stimulus coherence. Vision Res. 41, 981–989.
Brouwer, G. J., Tong, F., Hagoort, P., and van Ee, R. (2009). Perceptual incongruence influences bistability and cortical activation. PLoS ONE 4, e5056. doi:10.1371/journal.pone.0005056
Brown, R. J., and Norcia, A. M. (1997). A method for investigating binocular rivalry in real time with the steady-state VEP. Vision Res. 37, 2401–2408.
Carter, O. L., Campbell, T. G., Liu, G. B., and Wallis, G. (2004). Contradictory influence of context on predominance during binocular rivalry. Clin. Exp. Optom. 87, 153–162.
Carter, O. L., and Pettigrew, J. D. (2003). A common oscillator for perceptual rivalries? Perception 32, 295–305.
Chong, S. C., Tadin, D., and Blake, R. (2005). Endogenous attention prolongs dominance durations in binocular rivalry. J. Vis. 5, 1004–1012.
Costello, P., Jiang, Y., Baartman, B., McGlennen, K., and He, S. (2009). Semantic and subword priming during binocular suppression. Conscious. Cogn. 18, 375–382.
Deutsch, J. A., and Deutsch, D. (1963). Attention: some theoretical considerations. Psychol. Rev. 70, 80–90.
Fox, R., and Hermann, J. (1967). Stochastic properties of binocular rivalry alternations. Percept. Psychophys. 2, 432–436.
Fox, R., and Rasche, F. (1969). Binocular rivalry and reciprocal inhibition. Percept. Psychophys. 5, 215–217.
Goryo, K. (1969). The effect of past experience upon the binocular rivalry. Jpn. Psychol. Res. 11, 46–53.
Hochstein, S., and Ahissar, M. (2002). View from the top: hierarchies and reverse hierarchies in the visual system. Neuron 36, 791–804.
Hock, H. S., Schöner, G., and Hochstein, S. (1996). Perceptual stability and the selective adaptation of perceived and unperceived motion directions. Vision Res. 36, 3311–3323.
Hubel, D. H., and Wiesel, T. N. (1962). Receptive fields, binocular interaction and functional architecture in the cat’s visual cortex. J. Physiol. 160, 106–154.
Jiang, Y., Costello, P., and He, S. (2007). Processing of invisible stimuli: advantage of upright faces and recognizable words in overcoming interocular suppression. Psychol. Sci. 18, 349–355.
Johnston, W. A., and Heinz, S. P. (1979). Depth of nontarget processing in an attention task. J. Exp. Psychol. Hum. Percept. Perform. 5, 168–175.
Kanai, R., Bahrami, B., and Rees, G. (2010). Human parietal cortex structure predicts individual differences in perceptual rivalry. Curr. Biol. 20, 1626–1630.
Kovács, I., Papathomas, T. V., Yang, M., and Feher, A. (1996). When the brain changes its mind: interocular grouping during binocular rivalry. Proc. Natl. Acad. Sci. U.S.A. 93, 15508–15511.
Lee, S. H., and Blake, R. (2004). A fresh look at interocular grouping during binocular rivalry. Vision Res. 44, 983–991.
Lehmkuhle, S., and Fox, R. (1975). Effect of binocular rivalry suppression on the motion after effect. Vision Res. 15, 855–859.
Leopold, D. A., and Logothetis, N. K. (1996). Activity changes in early visual cortex reflect monkeys’ percepts during binocular rivalry. Nature 379, 549–553.
Levelt, W. J. M. (1965). On Binocular Rivalry Soesterberg, The Netherlands: Institute for Perception RVOTNO.
Lo Sciuto, L. A., and Hartley, E. L. (1963). Religious affiliation and open-mindedness in binocular resolution. Percept. Mot. Skills 17, 427–430.
Logothetis, N. K. (1998). Single units and conscious vision. Philos. Trans. R. Soc. Lond. B Biol. Sci. 353, 1801–1818.
Logothetis, N. K., Leopold, D. A., and Sheinberg, D. L. (1996). What is rivaling during binocular rivalry? Nature 380, 621–624.
Meng, M., and Tong, F. (2004). Can attention selectively bias bistable perception? Differences between binocular rivalry and ambiguous figures. J. Vis. 4, 539–551.
Mudrik, L., Deouell, L. Y., and Lamy, D. (2011). Scene congruency biases binocular rivalry. Conscious. Cogn. 20, 756–767.
Ooi, T. L., and He, Z. J. (1999). Binocular rivalry and visual awareness: the role of attention. Perception 28, 551–574.
O’Shea, R. P., and Crassini, B. (1981). Interocular transfer of the motion after-effect is not reduced by binocular rivalry. Vision Res. 21, 801–804.
Paffen, C. L., Alais, D., and Verstraten, F. A. (2006). Attention speeds binocular rivalry. Psychol. Sci. 17, 752–756.
Papathomas, T. V., Kovacs, I., Feher, A., and Julezs, B. (1999). “Visual dilemmas: competition between eyes and between percepts in binocular rivalry,” in What is Cognitive Science? eds E. Lepore and Z. Pylyshyn (Malden, MA: Blackwell Publishers), 263–294.
Polonsky, A., Blake, R., Braun, J., and Heeger, D. J. (2000). Neuronal activity in human primary cortex correlates with perception during binocular rivalry. Nat. Neurosci. 3, 1153–1159.
Rousselet, G. A., Fabre-Thorpe, M., and Thorpe, S. J. (2002). Parallel processing in high-level categorization of natural images. Nat. Neurosci. 5, 629–630.
Rubin, N., and Hupe, J. M. (2005). “Dynamics of perceptual bistability: plaids and binocular rivalry compared,” in Binocular Rivalry, eds D. Alais and R. Blake (Cambridge, MA: MIT Press), 138–154.
Schacter, D. L., Reiman, E., Uecker, A., Polster, M. R., Yun, L. S., and Cooper, L. A. (1995). Brain regions associated with retrieval of structurally coherent visual information. Nature 376, 587–590.
Silver, M. A., and Logothetis, N. K. (2007). Temporal frequency and contrast tagging bias the type of competition in interocular switch rivalry. Vision Res. 47, 532–543.
Smets, G. (1975). Pleasingness vs. interestingness of visual stimuli with controlled complexity: their relationship to looking time as a function of exposure time. Percept. Mot. Skills 40, 3–7.
Sobel, K. V., and Blake, R. (2002). How context influences predominance during binocular rivalry. Perception 31, 813–824.
Tong, F., and Engel, S. A. (2001). Interocular rivalry revealed in the human cortical blind-spot representation. Nature 411, 195–199.
Tong, F., Meng, M., and Blake, R. (2006). Neural bases of binocular rivalry. Trends Cogn. Sci. (Regul. Ed.) 10, 502–511.
Tong, F., Nakayama, K., Vaughan, J. T., and Kanwisher, N. (1998). Binocular rivalry and visual awareness in human extrastriate cortex. Neuron 21, 753–759.
Tononi, G., Srinivasan, R., Russell, D. P., and Edelman, G. M. (1998). Investigating neural correlates of conscious perception by frequency-tagged neuromagnetic responses. Proc. Natl. Acad. Sci. U.S.A. 95, 3198–3203.
Toppino, T. C. (2003). Reversible-figure perception: mechanisms of intentional control. Percept. Psychophys. 65, 1285–1295.
Treisman, A. (1962). Binocular rivalry and stereoscopic depth perception. Q. J. Exp. Psychol. 14, 23–37.
Valle-Inclán, F., and Gallego, E. (2006). Bilateral frontal leucotomy does not alter perceptual alternation during binocular rivalry. Prog. Brain Res. 155, 235–239.
van Boxtel, J. J., Knapen, T., Erkelens, C. J., and van Ee, R. (2008). Removal of monocular interactions equates rivalry behavior for monocular, binocular, and stimulus rivalries. J. Vis. 8, 13.1–13.17.
van Ee, R. (2005). Dynamics of perceptual bi-stability for stereoscopic slant rivalry and a comparison with grating, house-face, and Necker cube rivalry. Vision Res. 45, 29–40.
van Ee, R., Parker, A. L., van Boxtel, J. J., and Alais, D. M. (2007). Voluntary control over visual rivalry can be enhanced by sound that is consistent with scene interpretation. Perception 36, S57.
van Ee, R., van Dam, L. C., and Brouwer, G. J. (2005). Voluntary control and the dynamics of perceptual bi-stability. Vision Res. 45, 41–55.
Wade, N. J., and Wenderoth, P. (1978). The influence of colour and contour rivalry on the magnitude of the tilt after effect. Vision Res. 18, 827–835.
Wilson, H. R. (2003). Computational evidence for a rivalry hierarchy. Proc. Natl. Acad. Sci. U.S.A. 100, 14499–144503.
Wilson, H. R. (2005). “Rivalry and perceptual oscillations: a dynamical synthesis,” in Binocular Rivalry, eds D. Alais and R. Blake (Cambridge, MA: MIT Press), 317–335.
Yu, K., and Blake, R. (1992). Do recognizable figures enjoy an advantage in binocular rivalry. J. Exp. Psychol. Hum. Percept. Perform. 18, 1158–1173.
Zaretskaya, N., Thielscher, A., Logothetis, N. K., and Bartels, A. (2010). Disrupting parietal function prolongs dominance durations in binocular rivalry. Curr. Biol. 20, 2106–2111.
Zhou, G., Zhanga, L., Liub, J., Yangc, J., and Qu, Z. (2010). Specificity of face processing without awareness. Conscious. Cogn. 19, 408–412.
Keywords: binocular rivalry, cortical level, consciousness, perception, binocular, monocular, utrocular, semantics
Citation: Wolf M and Hochstein S (2011) High-level binocular rivalry effects. Front. Hum. Neurosci. 5:129. doi: 10.3389/fnhum.2011.00129
Received: 29 June 2011; Accepted: 17 October 2011;
Published online: 01 December 2011.
Edited by:
Alexander Maier, Vanderbilt University, USAReviewed by:
David Alais, University of Sydney, AustraliaLionel Naccache, Hôpital de la Pitié-Salpétrière, France
Chien-Te Wu, National Taiwan University College of Medicine, Taiwan
Yoram S. Bonneh, University of Haifa, Israel
Copyright: © 2011 Wolf and Hochstein. This is an open-access article subject to a non-exclusive license between the authors and Frontiers Media SA, which permits use, distribution and reproduction in other forums, provided the original authors and source are credited and other Frontiers conditions are complied with.
*Correspondence: Michal Wolf and Shaul Hochstein, Neurobiology Department, Institute for Life Sciences, Interdisciplinary Center for Neural Computation and Edmond and Lily Safra Brain Research Center, Hebrew University, Jerusalem 91904, Israel. e-mail:bWljaGFsLndvbGZAbWFpbC5odWppLmFjLmls;c2hhdWxAdm1zLmh1amkuYWMuaWw=