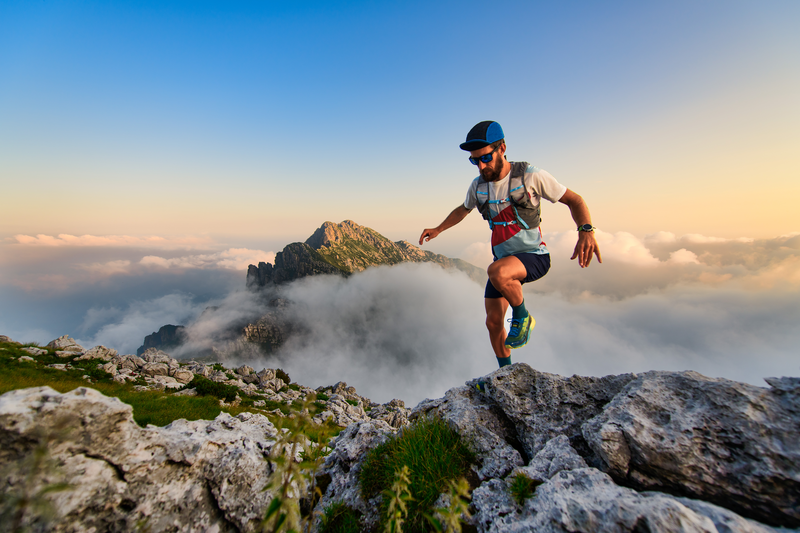
94% of researchers rate our articles as excellent or good
Learn more about the work of our research integrity team to safeguard the quality of each article we publish.
Find out more
ORIGINAL RESEARCH article
Front. Hortic. , 18 July 2024
Sec. Controlled Environment Horticulture
Volume 3 - 2024 | https://doi.org/10.3389/fhort.2024.1413224
This article is part of the Research Topic Light Application in Controlled Environment Horticulture View all articles
Indoor farming technologies, such as aquaponics and hydroponics, are crucial for advancing sustainable agriculture, especially in urban areas with limited land for conventional agriculture. As studies comparing these two systems are limited, this study explored the cultivation of five leafy vegetables—white leaf beets (Beta vulgaris var. cicla), hooker chives (Allium hookeri), basil (Ocimum basilicum), lemon balm (Melissa officinalis), and spearmint (Mentha spicata)—in an indoor greenhouse facility using two different food production platforms: an aquaponic system (AS) with catfish (Silurus asotus) and a conventional hydroponic system (HS). The results showed that the plant growth in AS outperformed that in HS, while the general growth patterns in AS were significantly affected by the different light sources. In addition, the leaf yield of the herbal plants (basil and lemon balm) in AS was 60%–70% more than that in HS. The leaf growth of hooker chives in AS also showed significant improvement (200%) compared with that in HS. It appears that the nutrient sources provided by fish were remarkable, as the average concentration of NO3–N (64 mg/L) was easily achieved in AS. The proposed AS, therefore, would be a promising alternative to the production and supply of supplement organic food, ultimately contributing to promoting sustainable agriculture in a changing climate.
Due to climate changes, social demands, and food safety associated with the application of chemicals, alternative food production systems such as aquaponics and hydroponics are commonly used for eco-friendly food supply chains around the world (Aleksic and Sustersic, 2020; Rodgers et al., 2022). A hydroponic system (HS) can be classified as open or closed based on the management of the nutrient solutions. Although the open HS is commonly used, it is limited to dealing with effluent containing a high concentration of nitrogen in the crop, ultimately causing environmental pollution in waterbodies (Hu et al., 2015; Heo and Baek, 2021). The closed system, on the other hand, recycles the nutrient solution, thereby decreasing the water and nutrient consumption and minimizing its environmental impact. However, careful regulation of the nutrient concentrations, pH, and salinity is required to reduce the deleterious impacts on plant development (AL-HARBI et al., 2008). Unlike the HS, the aquaponic system (AS), which uses fish tanks and crop growth beds equipped with biofloc technology, has become popular for the decomposition of fish wastes into useful microorganisms and converting these into nitrogen and phosphorus, which can be used to grow crops organically without chemical inputs (da Silva et al., 2016; Delaide et al., 2016; Rizal et al., 2018). AS can be coupled or decoupled. In a coupled system, for example, the recirculation of water flows continuously between the fish and plant components, allowing for the direct transfer of nutrients, while a decoupled system separates these processes to provide optimal conditions for each component, increasing the nutrient balance and overall system stability (Monsees et al., 2017; Baganz et al., 2022; Rodgers et al., 2022). Numerous studies have reported that AS is an alternative food production system in that it can provide a variety of economic and social advantages while operating within a controlled environment system (Zou et al., 2016; Knaus and Palm, 2017; Sapkota, 2019; Li et al., 2020; Yang et al., 2021).
Although both AS and HS are commonly used currently, the noticeable issues associated with water temperature control and power consumption still exist. Moreover, as various horticultural crops (e.g., lettuce, strawberry, and tomatoes) still require organic and eco-friendly production technologies to manage nitrogen metabolism, practical applications are under development in commercial sectors (Heo et al., 2015; Love et al., 2016; Lukmantoro et al., 2020; Tawaha et al., 2022; Ryu et al., 2023). Several studies have shown that various methods, including water supply/drainage methods (da Silva et al., 2016; Fan et al., 2019; Li et al., 2020), and the biofilter/biofloc method are commonly used for crop cultivation in AS (da Silva et al., 2016; Knaus and Palm, 2017; Rizal et al., 2018; Fan et al., 2019; Kasozi et al., 2021; Obirikorang et al., 2021).
While minimizing environmental impacts, organic agriculture also plays a crucial role in preserving food safety and in reducing the environmental footprint by abstaining from the use of synthetic pesticides and fertilizers (Reganold and Wachter, 2016). In the context of organic certification, the United States has allowed the certification of products cultivated in HS and AS (Kledal et al., 2019; Park and Williams, 2024). This recognition is based on their compliance with the resource conservation and biodiversity regulations, a stance upheld by a United States District Court in 2021, therefore reinforcing the National Organic Program of the United States Department of Agriculture (USDA) (Dinzeo, 2022). Since July 1, 2014, South Korea and the USA have officially acknowledged and accepted each other’s organic certifications, which has increased the trading of processed organic commodities between the two countries (USDA, n.d.). As the demand for smart aquaponics technology continues to increase in the Republic of Korea (South Korea, hereafter), aquaponics facilities (Kyaw and Ng, 2017; Hafid and Ciptadi, 2020; Taha et al., 2022) and the associated research have started to enhance value-added agriculture using information communication technology (ICT)-based aquaponics in recent years. However, research on the crop growth characteristics, chemical changes in the circulating water, and environmental monitoring and control interfaces is still underway for broader applications. Therefore, in this study, we evaluated the crop growth in two different systems: conventional hydroponics (HS) and aquaponics (AS) with different light inputs as supplement sources. We anticipate that the research findings will provide useful insights into controlled environment agriculture (CEA) communities, contributing to promoting sustainable food production in a changing climate.
To grow crops and to raise catfish (Silurus asotus) in AS, a 5-ton water tank, a 0.5-ton sediment tank, three 0.75-ton filtration tanks, and an air blower were first installed, as shown in Figure 1. Before hydroponic cultivation is put in place, several useful microorganisms, e.g., nitrifying bacteria, were introduced into a filtration tank equipped with a biofilter, consequently decomposing fish excrement using biofloc technology. The fish excrement-derived cultivation water was then supplied to the growth chamber through the AS components, including the water, sedimentation, and filtration tanks (Bakhsh and Shariff, 2007; Fan et al., 2019). In addition, plant growth chambers consisting of six 5-m × 2-m culture beds were used to grow vegetables hydroponically using a 10-cm-high DFT system, which reduces the human effort needed to manage water supply and timing controls.
Figure 1 Overview of the proposed indoor plant production system (upper panel) and the Internet of Things (IoT)-based monitoring system (lower panel).
An integrated environmental monitoring system was also operated using Internet of Things (IoT)-based real-time cloud data services for collection of the environmental variables, including the fish and crop growth and the water quality components [e.g., dissolved oxygen (DO), electrical conductivity (EC), pH, and water temperature (WT)] in AS (Zou et al., 2016). The integrated environmental monitoring system included the MariaDB Node.js and the Real-Time Streaming Protocol (RTSP) web socket image processing modules. A total of eight sensors were installed in the measurement terminal to collect environmental data from the fish tank and the culture beds (see Figure 1). The collected data from the various sensors were then transferred to the cloud via the Arduino and Atlas Whitebox T1 network system for cloud-based web visualization services. Video footage is easily broadcast through power-over-ethernet (PoE) cameras. Note that DO, EC, oxidation–reduction potential (ORP), pH, and WT were measured in the fish tank, the influent, and the effluent from the growth chamber, while air temperature and humidity were monitored in the glasshouse facility.
To equalize the incident light intensity for AS, each of the four culture beds with 60-cm intervals in the holes horizontally was installed at the lower cultivation bed with two different light treatments in addition to natural light. For example, combinations of natural light with blue/red light (N-BR) and natural light with white lights (N-W) were used by employing light-emitting diodes (LEDs) while exposed to natural sunlight through the greenhouse facility, as shown in Figure 1. To maintain consistent light intensity in the range of 60–100 μmol m−2 s−1 in the growth bed, an on/off switch control along with an illuminance sensor was used. The average light intensity recorded in AS was 178 μmol m−2 s−1. The temperature and humidity change for the experimental period, i.e., from June 21 to December 31, 2021, is illustrated in Figure 2.
Figure 2 Time series of the air temperature (in degree Celsius) and relative humidity (in percent) in the aquaponic system (AS) during the experiment period, from June 21 to December 31, 2021.
A set of four vegetable combinations—white leaf beet (Beta vulgaris var. cicla), red leaf beet (B. vulgaris var. cicla), endive (Cichorium intybus), and hooker chives (Allium hookeri)—and a set of three herbs—lemon balm (Melissa officinalis), basil (Ocimum basilicum), and spearmint (Mentha spicata)—were used for this research. Hooker chives have become popular as medicinal food due their root extract containing anti-obesity properties. Consumption of the leaves of hooker chives has also become remarkable in South Korea in recent years. However, no hydroponically grown leaves are available in the market. Therefore, we attempted to compare the performance of leafy vegetables grown in AS and HS. Note that the cultivation periods for leaf beets and basil were recorded at 3 and 4 weeks, respectively, while the other crops require 5 weeks depending on the leaf area and plant height in the culture bed. Hooker chives were harvested three times every 5 weeks to examine the average leaf growth when roots developed. The concentrations of the inorganic components in AS, such as NH3–N, NO3–N, and NO2–N, were also measured hourly with a simple absorbance meter (C-MAC Co. Ltd., Daejeon, South Korea). The customary Yamazaki culture solution (N = 6.5, P = 1.5, K = 4.0, Ca = 2.0, and Mg = 1.0 ppm) was used as the nutrient solution for the growth chamber in HS, and the EC and pH were monitored during the experimental period (Ban et al., 2019; Feng et al., 2023), as shown in Figure 3. For the aquaculture part, catfish with a length of less than 10 cm was introduced, which later gained 15 kg in weight in AS after 6 months. The WT and DO in the water tank were maintained at 23°C and 8 ppm (with aerators), respectively.
Figure 3 Time series of the electrical conductivity (EC; in decisiemens per meter) and pH in the aquaponic system during the experimental period, from June 21 to December 31, 2021.
A total of 18 individual plants were randomly selected from the AS and HS culture beds, and the growth parameters were selected and analyzed for comparisons. Three replicates of each plant were collected, and statistical analysis was conducted using the SAS program (version 6.21; SAS Institute Inc., Cary. NC, USA). Based on the test results, Duncan’s multiple test (p = 0.05) was conducted for analysis of variance (ANOVA).
In the AS, the crops were grown in hydroponic beds using nutrients derived from fish excrement without chemical fertilizers. Therefore, the activity of beneficial microorganisms in the circulating water for the mineralization of necessary nutrients is important for crop growth (Delaide et al., 2016). Thus, for this study, several crops were successfully cultivated without the addition of nutrients other than the catfish excrement-infused cultivated water. As shown in Table 1, the results indicated that the quantitative growth of the crops was influenced by the different light sources (N-BR and N-W) in AS. A test was started on the third week of plant growth, and the results showed that the fresh weight of white leaf beet in AS outperformed that in HS, especially when the different light sources (N-W and N-BR) were supplied (see Table 1). Thus, the fresh weight of white leaf beets in AS increased by about 90% compared with that in HS.
Table 1 Fresh weight, number of unfolded leaves, and soil plant development (SPAD) values of the plant species in a hydroponic system (HS) and an aquaponic system (AS) with the supplement light sources (N-BR and N-W).
Without a supplement light source, the fresh weight of white leaf beets in AS increased by 60% more than that in HS. A similar observation was found in red leaf beets. Unlike that for the white leaf beets, the results for the red leaf beets in AS showed growth performance in the following order: N-BR > N-W > natural light > conventional HS.
During the third week of cultivation, an increase in the number of unfolded leaves in both white and red leaf beets was also observed. The results indicated that the number of unfolded leaves in the white leaf beets in AS with the N-BR light source was 9.6, which was 14% more than that in HS. The number of unfolded leaves in red leaf beets also had a similar result, showing nine unfolded leaves on average in AS with the N-BR option, which was 15% more than that in HS. On the other hand, unlike the increases in fresh weight, dry weight, and leaf development, the soil plant development (SPAD) values in the leaves of white leaf beets increased significantly in HS compared with that in AS. Interestingly, the SPAD value in the leaves of both white and red leaf beets was the largest in HS, which increased by more than 20% and 15%, respectively, compared with that in AS. However, no evidence was found on whether the mineral quality contributes to the SPAD value. Furthermore, the SPAD value of the red leaf beets in AS was higher in the natural light condition than that in the different light sources (N-W and N-BR). It is possible that the pigment synthesis performance in the leaf is likely affected by the difference in the light intensity rather than the supplement light source. However, unlike previous studies, the shoot weight of all plant species grown in AS was significantly increased in pigment synthesis compared with that in HS regardless of the supplement light sources used (Love et al., 2016; Mulay and Reddy, 2021). This result could be due to the differences in environmental factors for crop cultivation and the fish farming skills in addition to independent cultivation practices.
Within AS, although the light intensity of the supplement light sources was lower than that of natural light, a supplement light source with a specific wavelength might be beneficial for plant growth. For instance, N-BR was found to have a significant effect on the increase in the number of unfolded leaves and the promotion of leaf elongation for all crops (see Figure 4). The effect of promoting crop growth with the blue plus red mixed light (BR) has been discussed in various crop species in the plant factory system (Goddek et al., 2015; Heo et al., 2019; Holmes et al., 2019). Numerous studies determining the relationship between light quality and plant growth have reported the positive effects of mixture irradiation of these single LED lights compared with monochromic red or blue light (Knaus et al., 2020). Figure 5 shows that the dry weight of basil on the fourth week of cultivation in AS increased significantly by 67%–71% compared with that in HS, but no significant effect was observed within AS with the use of different light sources (natural, N-W, and N-BR). Similarly, the number of branches also increased by more than 80% compared with that in HS (see Figure 5).
Figure 4 Growth of several plants. From the top: 1) white leaf beets; 2) hooker chives; 3) basil; 4) lemon balm; and 5) spearmint in the hydroponics system and the aquaponic system with different light sources: natural light with white lights (N-W) and natural light and blue/red light (N-BR) with natural light.
Figure 5 Growth of basil, lemon balm, and spearmint after treatments with or without the supplement light source in the hydroponic system (HS) and the aquaponic system (AS). Vertical bar denotes the average ± standard error. Different letters indicate significant difference at the 5% level using Duncan’s multiple range test. ns, not significant.
According to a previous study (Rodgers et al., 2022), when basil is hydroponically cultivated using carp culture water, the SPAD values in the leaves were similar to those in HS, but the fresh or dry weight decreased in the AS (Rupasinghe and Kennedy, 2010). The different growth reactions of crops in AS under open fields and facilities have been reported in other studies (Knaus et al., 2020; Ke et al., 2021) by testing different crops (e.g., basil vs. other crop species) (Kim et al., 2019; Li et al., 2020).
In this study, the growth effect of AS was much more visible than that of HS in the selected crops (basil, lemon balm, and spearmint) from the statistical analysis. In AS, the fresh weight of lemon balm in the N-W condition, for example, increased significantly: 100 times more than that in HS. A similar result was also found for the SPAD value in the leaves of lemon balm in AS. Thus, the leaf yield of lemon balm increased significantly in AS with the supplement light source, which increased by 73% compared with the natural light condition. However, in lemon balm, no significance was found between the different light treatments.
On the other hand, the fresh weight of spearmint was significantly increased in AS with the supplement light source, as opposed to HS with natural light, but no significance was found between the different light treatments in AS (see Figure 5). The effects of hydroponics on herbs such as basil and mint with different fish species [e.g., tilapia (Oreochromis niloticus) and carp (Cyprinus carpio)] have been widely studied in AS settings (Endut et al., 2009; Kim et al., 2019; Aleksic and Sustersic, 2020; Li et al., 2020). Similar results were also observed in this study in the sense that the growth of basil and lemon balm in AS was better than that in HS. Interestingly, although no significant difference in the fresh weight of hooker chive leaves was identified in AS with different light inputs, significant differences in fresh weight were found between HS and AS (see Table 2). Thus, in AS, the average leaf length of hooker chives increased by more than 30% compared with that in HS, and the number of branches also increased by 49%. The SPAD value of the leaves in AS increased in the order AS: N-W > AS: N-BR.
Table 2 Average leaf growth of hooker chives harvested three times every 5 weeks in the hydroponic system (HS) and the aquaponic system (AS) with supplement light sources.
Hooker chives are a medicinal crop, the roots being mainly used, that are in high demand in Korea (Sikawa and Yakupitiyage, 2010; Holmes et al., 2019). Therefore, in order to improve their added value and expand their marketability as fresh leaf vegetables, a comparative analysis study on their nutritional ingredients and the economic feasibility of each cultivation method, such as chemical fertilizer-based hydroponics or organic HS, could be valuable. As previous studies (Suhl et al., 2016; Rizal et al., 2018; Mulay and Reddy, 2021) have suggested, additional investigations to compare the material synthesis performance and crop yields associated with the differences in various crop species (leaf vs. fruit vegetables) would also be beneficial.
In agreement with a previous study (Zou et al., 2016), the pH and the EC in the circulating water affect the nitric acidity of fish wastes, but the NO3–N and PO43− concentrations in the circulating water increased significantly over time (see Figure 6). Essentially, the concentration of PO43− within the customary Yamazaki culture solution (EC = 1.5 dS/m) was approximately 17 ppm, but its concentration increased dramatically (150%), as shown in Figure 6. In addition, the concentration of NH3–N tended to increase over a certain period, but then decreased to 46 mg/L by the end of the study. It appears that physical (e.g., biofilter and fish farming density) and chemical factors (e.g., pH) affect the concentration of NH3–N or NH4–N in AS. It could be that higher concentrations of NH3–N or NH4–N in aquaculture may be stressful for fish growth; however, no negative impact on fish was found when these values were maintained below a certain level, at 100–300 mg L−1 (da Silva et al., 2016; Delaide et al., 2016; Knaus and Palm, 2017; Pinho et al., 2017; Madar et al., 2019).
Figure 6 Time series of the nutrient contents in the aquaponic system (AS) during the catfish culture period. Vertical bars represent standard error.
In AS, the quantitative changes in the nitrogen components also affect the quantitative and qualitative growth or yield of the cultivated crops along with fish growth (Tyson et al., 2011; Tawaha et al., 2022). In the case of catfish farming using a bio-ring in this study, NO2–N was barely detected, while NH3–N increased compared with that in the early stages of fish culture; however, it was considered that the concentration was not at a level that would cause inhibition of fish growth. In addition to the physical and chemical factors mentioned above, other aquaculture environmental factors, such as the water tank management methods and the microbial growth ability, could have possibly contributed to the changes in the concentrations of NH3–N and NO2–N. In general, the utilization efficiency of inorganic components such as nitrogen and phosphoric acid in the circulating water in AS varies depending on the plant species (Karen and Lin, 2014). In the case of hydroponic cultivation of various crop species at the same time, it is not easy to accurately estimate the absorption status of the inorganic components for each plant species during the culture period. Based on engineering assessments, however, it was considered that the conversion of fish waste into NO3–N was effectively achieved with the application of biofloc technology, resulting in the NH3–N decrease after 10 weeks of cultivation and a decrease by more than 38% compared with that of NO3–N. The concentration of phosphoric acid in the circulating water showed an increase in the pattern similar to the change in the concentration of NO3–N, while the concentration of SiO2 among the microelement components in the circulating water was measured relatively high at 8.3 mg/L. The results showed that Fe increased slightly over time, but a small amount (approximately 0.2 mg/L) was posted at the end of the experiment. Al and Mn were measured at while Al and Mn were measured at 0.08 to 0.11 mg/L, respectively. On the other hand, NO2–N, which was approximately 0.2 mg/L at the beginning of the fish culture, decreased as the culture period elapsed and was found to be close to 0 at the end of the culture period.
In AS, NO3–N, an indicator of the nitric oxidation capacity of useful microorganisms in the filtration tank, where the fish culture water is accumulated before the circulating water goes into the culture beds, is an important nutrient for plant growth. NO3–N showed differences in concentration depending on the fish species in AS during the culture period; however, the examined plant growth was significant even under conditions of 59% lower than that (100 mg/L) in HS. This shows that, even with reduced amounts of nitrate nitrogen (NO3–N), aquaponic systems are capable of supporting plant growth, indicating sustainable operation with variable nutrient profiles. Investigation of the occurrence of harmful microorganisms in the circulating water as one of the fish growth indicators showed that no fish pathogenic microorganisms, such as Enterococcus fascism and Vibrio anguillarum, or visible crop diseases were observed. However, according to a previous study (Kasozi et al., 2021), the constant management and monitoring of harmful microorganisms in the circulating water under AS is still necessary for stable and safe food production using biofloc technology (Ke et al., 2021).
The proposed circular AS works well in the production of organically grown leafy vegetables, including white leaf beets, hooker chives, basil, lemon balm, and spearmint, in indoor greenhouse facilities. Three different light sources, namely, natural light and the combinations of natural light with blue/red light (N-BR) and natural light with white lights (N-W), were assessed to compare the system performance of AS and HS. The plant growth in AS with the supplement light source outperformed that in HS based on the plant physiology tests (e.g., dry/fresh weight, leaf yield, and SPAD). The results showed that the plant growth in AS performed better than that in HS, while the general growth patterns were significantly affected by the supplement light sources in AS. For example, the leaf yield of the herbal plants (basil and lemon balm) in AS was 60%–70% more than that in HS, and the leaf growth in hooker chives in AS also showed significant performance (200%) against that in HS. In addition, it appears that the nutrient solutions provided by fish were remarkable in the sense that the average concentration of NO3− (64 ppm) was easily achieved in AS.
Although the proposed AS is promising for the production and supply of supplement organic food, additional studies are still required to better characterize the water consumption associated with evapotranspiration processes during the growing period. It is possible, as indicated in a previous study (Mulay and Reddy, 2021), that countermeasures of evapotranspiration to reduce the water loss in the system could be useful via life cycle analysis and/or water budget analysis in the system dynamics framework.
The raw data supporting the conclusions of this article will be made available by the authors, without undue reservation.
JH: Writing – original draft. JB: Writing – review & editing, Data curation, Software. ZS: Formal analysis, Methodology, Writing – review & editing. JR: Writing – review & editing.
The author(s) declare financial support was received for the research, authorship, and/or publication of this article. This research was financially supported by the Rural Development Administration (Project No. PJ01579601). This publication activity is also supported partially by the National Institute of Food and Agri-culture, U.S. Department of Agriculture (USDA), under JR’s Hatch project 1023305. Any opinions, findings, or recommendations expressed in this publication are those of the authors and do not necessarily reflect the view of USDA.
This is a short text to acknowledge the contributions of specific colleagues, institutions, or agencies that aided the efforts of the authors.
The authors declare that the research was conducted in the absence of any commercial or financial relationships that could be construed as a potential conflict of interest.
All claims expressed in this article are solely those of the authors and do not necessarily represent those of their affiliated organizations, or those of the publisher, the editors and the reviewers. Any product that may be evaluated in this article, or claim that may be made by its manufacturer, is not guaranteed or endorsed by the publisher.
Aleksic N., Sustersic V. (2020). Analysis of application aquappnics system as a model of the circular economy: A review. Recycling Sustain. Devel. 13, 73–86. doi: 10.5937/ror2001073A
Al-Harbi A.-A. R., Abd-Rabbo M., Farag A. A., Medany M. A., Abd-Elmoniem E. M. (2008). Comparison between open and closed hydroponic systems on lettuce yield. Egyptian J. Agric. Res. 86, 695–702. doi: 10.21608/ejar.2008.205772
Baganz GöstaF. M., Junge R., Portella M. C., Goddek S., Keesman K. J., Baganz D., et al. (2022). The aquaponic principle—It is all about coupling. Rev. Aquaculture. 14, 252–264. doi: 10.1111/raq.12596
Bakhsh K., Shariff R. (2007). Nutrient optimization and computerized decision support program in recirculating integrated aquaculture system. Int. J. @ Environmental Cultural Economic Soc. Sustainability. 3, 25–345. doi: 10.18848/1832-2077/CGP/v03i05/54410
Ban B., Ryu D., Lee M. (2019). “Machine learning approach to remove ion interference effect in agricultural nutrient solutions,” in Paper presented at the 2019 International Conference on Information and Communication Technology Convergence (ICTC). Institute of Electrical and Electronics Engineers. Jeju, Korea (South): IEEE. doi: 10.1109/ICTC46691.2019
da Silva C., Lima R., Vale D., Carmo-e-Sa M. (2016). High levels of total ammonia nitrogen as nh4+ Are stressful and harmful to the growth of nile tilapia juveniles. Scientia Hortic. 211, 277–825. doi: 10.4025/actascibiolsci.v35i4.17291
Delaide B., Godde k. S., Gott J., Soyeurt H., Jijakli H. (2016). Lettuce (Lactuca sativa L. Var. Sucrine) growth performance in complemented aquaponic solution outperforms hydroponics. Water. 8, 467–477. doi: 10.3390/w8100467
Dinzeo M. (2022). “Ninth Circuit Finds Hydroponic Crops Can Be Labeled Organic.” September 22, 2022Courthouse News Service, May 23, 2024. Available online at: https://www.courthousenews.com/ninth-circuit-finds-hydroponic-crops-can-be-labeled-organic/.
Endut A., Jusoh A., Ali N., Wan Nik W., Hassan A. (2009). Effect of flow rate on water quality parameters and plant growth of water spinach (Ipomoea aquatica) in and aquaponic recirculation system. Desalination Water Treat. 5, 19–285. doi: 10.5004/dwt.2009.559
Fan X., Xue F., Song B., Chen L., Xu G., Xu H. (2019). Effects of blue and red light on growth and nitrate metabolism in Pakchoi. Open Chem. 17, 456–645. doi: 10.1515/chem-2019-0038
Feng K., Kan X.-Y., Liu Q., Yan Y.-J., Sun N., Yang Z.-Y., et al. (2023). Metabolomics analysis reveals metabolites and metabolic pathways involved in the growth and quality of water dropwort [Oenanthe javanica (Blume) dc.] under nutrient solution culture. Plants. 12, 1459. doi: 10.3390/plants12071459
Goddek S., Delaide B., Mankasingh U., Ragnarsdottir K., Jijakli H., Thorarinsdottir R. (2015). Challenges of sustainable and commercial aquaponics. Sustainability. 7, 4199–2245. doi: 10.3390/su7044199
Hafid H. R., Ciptadi P. W. (2020). “Smart aquaponics design using internet of things technology,” in IOP Conf. Series: Materials Science and Engineering, Vol. 835. Semarang, Indonesia: The 7th International Conference on DV-Xα Method 2-4 September 2019. doi: 10.1088/1757-899X/835/1/012026
Heo J., Baek J. (2021). Effects of light-quality control on the plant growth in a plant facrory system of artificial light type. Korean J. Environ. Agric. 40, 270–785. doi: 10.5338/KJEA
Heo J., Kim H., Lee K., Yoon J., Lee J., Huh Y., et al. (2015). Effect of supplementary radiation on growth of greenhouse-grown kales. Korean J. Environ. Agric. 34, 38–45. doi: 10.5338/KJEA
Heo J., Park K., Hong S., Lee J., Baek J. (2019). Hydroponic nutrient solution and light quality influence on lettuce (Lactuca sativa L.) growth from the artificial light type of plant factory system. Korean J. Environ. Agric. 38, 225–365. doi: 10.5338/KJEA
Holmes S., Wells D., Pickens J., Kemble J. (2019). Selection of heat tolerant lettuce (Lactuca sativa) cultivars grown in deep water culture and their marketability. Horticulture. 5, 50–615. doi: 10.3390/horticulturae5030050
Hu Z., Lee J., Chandran K., Kim S., Brotto A., Khanal S. (2015). Effect of plant species on nitrogen recovery in aquaponics. Biores Tech. 188, 92–98. doi: 10.1016/j.biortech.2015.01.013
Karen M., Lin L. (2014). Scaling aquaponic systems: balancing plant uptake with fish output. Aquacultural Eng. 63, 39–415. doi: 10.1016/j.aquaeng.2014.09.002
Kasozi N., Abraham B., Kaiser H., Wilhelmi B. (2021). The complex microbiome in aquaponics: significance of the bacterial ecosystem. Ann. Microbiol. 71, 1–13. doi: 10.1186/s13213-020-01613-5
Ke X., Yi M., Li Q., Liu Z., Wang M., Cao J. (2021). Effect of the herbal houttuynia cordata floating bed on the nile tilapia pond culturing system. Aquaculture Rep. 20, 100680. doi: 10.1016/j.aqrep.2021.100680
Kim S., Jang J., Kim B., Lim H., Kim S. (2019). Urban aquaculture of catfish, silirus, using biofloc and aquaponics systems. Environ. Biol. Res. 37, 545–553. doi: 10.11626/KJEB.
Kledal P. R., König B., Matulić D. (2019). Aquaponics: the ugly duckling in organic regulation. Aquaponics Food Production Syst. 487, 487–500. doi: 10.1007/978-3-030-15943-6_19
Knaus U., Palm H. (2017). Effects of the fish species choice on vegetables in aquaponics under spring-summer conditions in northern Germany (Mecklenburg western pomerania). Aquaculture. 473, 62–735. doi: 10.1016/j.aquaculture.2017.01.020
Knaus U., Pribbernow M., Xu L., Appelbaum S., Palm H. (2020). Basil (Ocimum basilicum) cultivation in decoupled aquaponics with three hydro-components (Grow pipes, raft, gravel) and African catfish (Clarias gariepinus) production in Northern Germany. Sustainability. 12, 8745–8615. doi: 10.3390/su12208745
Kyaw T. Y., Ng A. K. (2017). Smart aquaponics system for urban farming. Energy Proc. 143, 342–347. doi: 10.1016/j.egypro.2017.12.694
Li N., Zhang L., Li J., Yan K., Wang Y., Wang B., et al. (2020). Effects of aquaponic system on growth and nutrients content ans sustainable production of sprouts in urban area. Austalian J. Crop Sci. 14, 1794–1995. doi: 10.21475/ajcs
Love D., Uhl M., Genello L. (2016). Energy and water use of a small-scale raft aquaponics system in Baltimore, Maryland, United States. Aquacultural Eng. 68, 19–275. doi: 10.1016/j.aquaeng.2015.07.003
Lukmantoro T., Prayogo T., Rahardja B. (2020). Effect of different filter media use on aquaponics system on ammonium (Nh4+), nitrite (No2) and nitrate (No3) concentra-tions of catfish (Clarias sp.) aquaculture. Earth Environ. Sci. 441, 1–55. doi: 10.1088/1755-1315/441/1/012121
Madar A., Ruboczki T., Hajos M. (2019). Lettuce production in aquaponic and hydroponic systems. Acta Universitatis Sapientiae Agric. Environ. 11, 51–59. doi: 10.2478/ausae-2019-0005
Monsees H., Kloas W., Wuertz S. (2017). Decoupled systems on trial: eliminating bottlenecks to improve aquaponic processes. PloS One. 12, e0183056. doi: 10.1371/journal.pone.0183056
Mulay B., Reddy K. (2021). Study of biofilter planted with basil for removal of ammonia in aquaponic water. Civil Environ. Eng. 17, 242–251. doi: 10.2478/cee-2021-0025
Obirikorang K., Sekey W., Gyampoh B., Ashiagbor G. (2021). Aquaponics for improved food security in Africa: A review. Front. Sustain. Food Syst. 5, 1–105. doi: 10.3389/fsufs.2021.705549
Park Y., Williams K. A. (2024). Organic hydroponics: A review. Scientia Hortic. 324, 112604. doi: 10.1016/j.scienta.2023.112604
Pinho S., Molinari D., Mello G., Fitzsimmons K. (2017). Effluent from a biofloc technology (Bft) tilapia culture on the aquaponics production of different lettuce varieties. Ecological Engineering. 103, 146–153. doi: 10.1016/j.ecoleng.2017.03.009
Reganold J. P., Wachter J. M. (2016). Organic agriculture in the twenty-first century. Nat. Plants. 2, 1–8. doi: 10.1038/nplants.2015.221
Rizal A., Dhahiyat Y., Zahidah Y., Andriani A., Handaka A., Sahidin A. (2018). The economic and social benefits of an aquaponic system for the integrated productions of fish and water plants. Asean-Fen Int. Fisheries Sym Earth Environ. 137, 1–7. doi: 10.1088/1755-1315/137/1/012098
Rodgers D., Won E., Timmons M., Mattson N. (2022). Complementary nutrients in decoupled aquaponics enhance basil performance. Horticulture. 8, 111–255. doi: 10.3390/horticulturae8020111
Rupasinghe J., Kennedy J. (2010). Economic benefits of integrating a hydroponic-lettuce system into a barramundi fish production system. Aquaculture Economics Manage. 14, 81–96. doi: 10.1080/13657301003776631
Ryu J. H., Subah Z., Baek J. (2023). An application of system dynamics to characterize crop production for autonomous indoor farming platforms (Aifp). Horticulturae. 9, 1318. doi: 10.3390/horticulturae9121318
Sapkota S. (2019). Effects of nutrient composition and lettuce cultivar on crop production in hydroponic culture. Horticulture. 5, 1–85. doi: 10.3390/horticulturae5040072
Sikawa D., Yakupitiyage A. (2010). The hydroponic production of lettuce (Lactuca sativa L) by using hybrid catfish (Clarias macrocephalu×C. Gariepinus) pond water: potencials and constraints. Agric. Water Manage. 97, 1317–1325. doi: 10.1016/j.agwat.2010.03.013
Suhl J., Dannehl D., Kloas W., Baganz D., Jobs S., Scheibe G., et al. (2016). Advanced aquaponics: evaluation of intensive tomato production in aquaponics vs. Conventional hydroponics. Agric. Water Manage. 178, 335–344. doi: 10.1016/j.agwat.2016.10.013
Taha M. F., ElMasry G., Gouda M., Zhou L., Liang N., Abdalla A., et al. (2022). Recent advances of smart systems and internet of things (Iot) for aquaponics automation: A comprehensive overview. Chemosensors. 10, 1–26. doi: 10.3390/chemosensors10080303
Tawaha A., Wahab P., Jaafar H., Zuan A., Hassan M., Al-Tawaha A. (2022). Yield and nutrients leaf concent of butterhead lettuce (Latuca sativa) in response to fish nutrient solution in a samll scale of aquaponic systems. Ecol. Eng. Environ. Technol. 22, 85–94. doi: 10.12912/27197050/141524
Tyson R., Treadwell D., Simonne E. (2011). Opportunities and challenges to sustainability in aquaponic systems. HortTech. 21, 6–135. doi: 10.21273/HORTTECH.21.1.6
Yang O., Lee H., Heo J., Lee J., Kim T., Kim H., et al. (2021). Design and implementation of aquaponics monitoring system on cloud-computing. J. Kor Institute Commun. Inf. Sci. 46, 2100–2107. doi: 10.7840/kics.2021.46.11.2100
Keywords: aquaponics, hydroponics, environmental monitoring, sustainable agriculture, light sources effect, greenhouse farming
Citation: Heo J, Baek J, Subah Z and Ryu JH (2024) Evaluating crop growth between hydroponics and aquaponics with different light inputs. Front. Hortic. 3:1413224. doi: 10.3389/fhort.2024.1413224
Received: 06 April 2024; Accepted: 20 June 2024;
Published: 18 July 2024.
Edited by:
Oksana Lastochkina, Institute of Biochemistry and Genetics of Ufa Scientific Centre (RAS), RussiaReviewed by:
Margit Olle, NPO Veggies Cultivation, EstoniaCopyright © 2024 Heo, Baek, Subah and Ryu. This is an open-access article distributed under the terms of the Creative Commons Attribution License (CC BY). The use, distribution or reproduction in other forums is permitted, provided the original author(s) and the copyright owner(s) are credited and that the original publication in this journal is cited, in accordance with accepted academic practice. No use, distribution or reproduction is permitted which does not comply with these terms.
*Correspondence: Jae Hyeon Ryu, anJ5dUB1aWRhaG8uZWR1
Disclaimer: All claims expressed in this article are solely those of the authors and do not necessarily represent those of their affiliated organizations, or those of the publisher, the editors and the reviewers. Any product that may be evaluated in this article or claim that may be made by its manufacturer is not guaranteed or endorsed by the publisher.
Research integrity at Frontiers
Learn more about the work of our research integrity team to safeguard the quality of each article we publish.