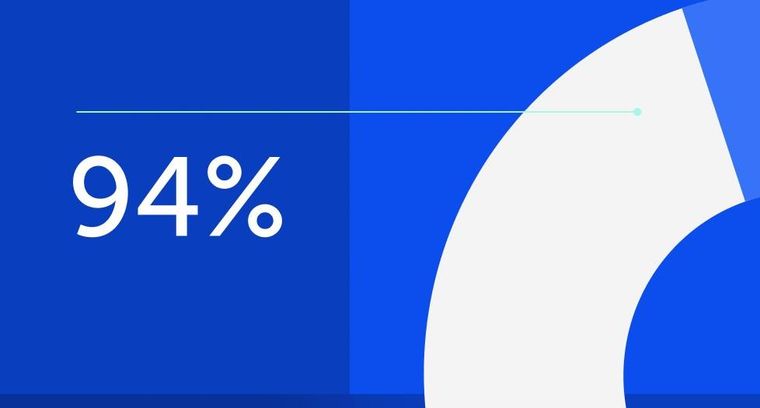
94% of researchers rate our articles as excellent or good
Learn more about the work of our research integrity team to safeguard the quality of each article we publish.
Find out more
REVIEW article
Front. Hematol., 03 March 2025
Sec. Blood Cancer
Volume 4 - 2025 | https://doi.org/10.3389/frhem.2025.1435193
In multiple myeloma (MM), hemostatic disorders such as thrombocytopenia, coagulopathies, and thrombophilia are well-documented. These abnormalities can be partially attributed to therapy, including thrombocytopenia following treatment with proteasome inhibitors such as bortezomib and carfilzomib or thrombosis associated with immunomodulatory drugs such as thalidomide and lenalidomide. However, acquired hemostatic disorders have also been observed in untreated or newly diagnosed MM patients. This review explores these abnormalities in both treated and untreated contexts, presenting recent studies that provide new insights into the mechanisms underlying these complications. It highlights the role of the bone marrow microenvironment, particularly mesenchymal stromal cells (MSCs) and extracellular vesicles (EVs). Additionally, the review discusses future research directions on hemostatic disorders, including bleeding and thrombosis, in MM patients. Overall, this review aims to be a valuable resource for scientists and clinicians in the field.
Multiple myeloma (MM) is a hematologic neoplasm primarily confined to the bone marrow, characterized by a dominant clone of malignant plasma cells and presenting with hypercalcemia, renal failure, anemia, and bone lesions (1). Abnormalities in both primary and secondary hemostatic systems are significant challenges in the clinical management of MM patients. For instance, thrombocytopenia is a common and often unavoidable complication associated with drug treatments (2, 3). Additionally, MM can negatively impact normal thrombopoiesis, platelet function, and the coagulation process (4, 5).
Understanding the molecular mechanisms underlying these abnormalities is crucial for advancing knowledge of MM pathophysiology. This understanding can aid in identifying new therapeutic targets and developing improved clinical management strategies to reduce the risk of serious complications. Early studies and recent research have provided insights into the impact of paraproteins on coagulation factors, the effects of MM tumor cells on platelet production and function, and the role of the bone marrow microenvironment in impaired thrombopoiesis in MM (5–7).
Despite these advances, gaps remain in our understanding of how the MM oncogenic process disrupts normal hemostasis. Further research is needed to elucidate these mechanisms and improve patient outcomes.
Chemotherapy-related thrombocytopenia is common in hematologic malignancies, including MM. Mellors et al. conducted a retrospective study on MM patients treated with chemotherapy over 11 years and found that 18% developed thrombocytopenia, which was correlated with lower overall survival (OS) and progression-free survival (PFS) (2). Shaw et al. reported that thrombocytopenia incidence was higher in hematologic malignancies than in solid tumors, with the highest rate in MM patients (37.7%) (3). In relapsed or refractory MM patients, the combination of daratumumab with bortezomib or dexamethasone resulted in grade 3 or 4 thrombocytopenia in 45.3% of cases, though it also prolonged PFS (8). Thrombocytopenia was also observed in patients treated with teclistamab, a bispecific antibody that targets both T cells and MM cells (9). The exact mechanisms—whether due to drug toxicity on thrombopoiesis, altered platelet release dynamics, or direct platelet toxicity—remain unclear.
Bortezomib-induced thrombocytopenia is unique in its cyclic pattern and kinetics. Bortezomib-related thrombocytopenia typically appears within the first 10 days of each treatment cycle, with a short recovery time and no cumulative or persistent effects. This is reported to be due to a functional alteration in platelet budding (proplatelet formation) rather than marrow megakaryocyte (MK) toxicity (10). Apparently, inhibition of NFκB activity by proteasome inhibitors (PIs) (11–13) underlies this effect, as NFκB activity is crucial for platelet budding and function (14–17). The cyclic nature of bortezomib-related thrombocytopenia was later demonstrated in Murai’s study in a mouse model, which showed that platelet count declined on days 2-4 post-administration and recovered to the normal range on day 6, however, proplatelet formation was significantly decreased without affecting bone marrow MKs or their ploidy distribution (18). Further studies confirmed that proteasome inhibition disrupted proplatelet formation in human and mouse MKs (19), and mice deficient in the proteasome subunit PSMC1 (26S protease regulatory subunit 4) exhibited severe thrombocytopenia and early mortality (19). Digital modeling also suggested that pan-proteasome inhibitors (bortezomib, carfilzomib, and ixazomib) promoted thrombocytopenia via myelosuppression or inhibition of (pro-)platelet formation (20). Additionally, Baaten et al. found that MM patients treated solely with alkylating agents who developed severe thrombocytopenia (<50×10^3/μL) had impaired mitochondrial function, highlighting a different mechanism of drug-induced thrombocytopenia (21).
Thalidomide, an immunomodulatory drug (IMiD), is associated with thrombocytopenia in MM, potentially through immune-mediated mechanisms that trigger platelet destruction. Keshava-Prasad et al. reported a case of thalidomide-induced thrombocytopenia with increased mean platelet volume (MPV) and elevated MKs in the bone marrow, suggesting an immune cause for the low platelet count (<20×10^3/µL) as no other factors were identified (22). Ryuge et al. documented a case of immune thrombocytopenic purpura (ITP) in an MM patient on a thalidomide regimen and indicated that the drug might have modulated normal lymphocytes to produce autoantibodies against platelets (23). In addition, a more recent case report described a 59-year-old MM patient who developed ITP following lenalidomide treatment but responded well to standard ITP therapy with intravenous immunoglobulin (IVIG) (24). These findings support an immune mechanism underlying IMiD-related thrombocytopenia in MM.
While thalidomide and lenalidomide are more commonly known to predispose MM patients to thrombotic events, thrombocytopenia remains an unavoidable, mostly transient, complication of IMiD therapies in MM. A collective evaluation of previous reports to date suggests that an autoimmune response results in the destruction of platelets and the development of ITP in some MM patients on IMiD therapy. Recognizing this potential side effect, it is crucial for clinicians to monitor and manage patients appropriately, adjust treatment regimens, and provide supportive care as needed.
Thrombosis risk factors include hereditary gene mutations, acquired factors such as surgery, a sedentary lifestyle, and advanced age. Both the oncogenesis process in cancer and cancer chemotherapy predispose patients to thrombosis (25). New treatments, including PIs, IMiDs, and monoclonal antibodies, have significantly improved the clinical outcomes for MM patients (26) but have also increased the frequency of thrombotic events, becoming a major cause of morbidity and mortality (mostly IMiDs and some PIs) (27–37). Thalidomide and lenalidomide are particularly associated with a heightened risk of thrombosis, especially when combined with high-dose dexamethasone and other chemotherapy (38, 39). Thrombotic incidence can reach up to 26% in some studies (40–42), with hemostatic changes often developing during the first month of thalidomide and dexamethasone therapy (43). Furthermore, venous thromboembolism (VTE) and arterial thrombosis in MM patients correlate with higher mortality rates compared to those without thrombosis (28). Thalidomide was FDA-approved in 2006 for use with high-dose dexamethasone in newly diagnosed MM, although the first reports of thalidomide-related deep vein thrombosis (DVT) in MM patients treated with this combination appeared in 2001 (44). Table 1 provides further information related to drug-associated thrombotic events in MM.
Table 1. Drug-related thrombotic events reported by clinical studies that used IMiD and/or PI treatment modalities.
Thrombomodulin, a cofactor for thrombin in activating protein C and inhibiting coagulation, undergoes transient reduction during initial therapy, potentially increasing thrombotic risk (45, 46). Certain drugs such as thalidomide derivatives enhance myelopoiesis but downregulate PU.1 transcription factor, promoting promyelocyte accumulation and increased cathepsin G, a potential thrombotic risk (47).
Platelet activation significantly contributes to thrombophilia in various conditions including myeloproliferative neoplasms (48) and COVID-19 (49). IMiDs, particularly thalidomide, have been linked to platelet activation and thrombotic events in MM patients. Thalidomide increases CD62P expression and PLA (platelet-leukocyte aggregate) formation in MM patients (50), markers associated with thrombosis and cardiovascular disease in humans (51, 52). Further studies demonstrate that IMiDs induce procoagulant activity (PCA) in MM by enhancing tissue factor exposure and phosphatidylserine (PS) on monocytes and endothelial cells (53). Using flow cytometry and confocal microscopy, Guo et al. found a significantly higher percentage of PS+ blood cells in MM patients vs healthy donors. In their in vitro studies, incubation of endothelial cells or blood cells with MM patient’s serum or with IMiDs and dexamethasone increased PS exposure on these cells (54). Finally, IMiDs also elevate plasma levels of hemostatic markers in MM patients, suggesting a hypercoagulable state (55).
Proteasome inhibitor-related thrombosis has also been reported in MM. While bortezomib has been associated with a low risk of thrombosis, it has been able to confer some level of protection against thrombosis if combined with IMiDs the mechanism of which is unclear (56). On the contrary, carfilzomib, especially in combination with dexamethasone, has been associated with increased incidence of thrombotic events in some clinical trials including ENDURANCE (57). The study by Alanazi et al. suggested enhanced platelet activation and increased platelet adhesion to type I collagen as the mechanisms underlying this carfilzomib effect (58). Additionally, a very recent study identified a germline mutation in the factor H gene as the cause of complement system dysregulation, hence an explanation for thrombotic microangiopathy (TMA) in MM patients following carfilzomib treatment (59).
Incidence of DVT associated with old doxorubicin-based regimens in MM was also reported (60, 61), which could be (at least partly) due to increased exposure of PS on platelets or increased generation of PS-exposing platelet microparticles by doxorubicin (62).
Findings from all the above studies indicate that IMiDs and chemotherapy might promote or pave the way for the development of thrombosis in MM mainly through interaction with components of the hemostatic system including endothelial cells, platelets and coagulation pathways.
The bone marrow microenvironment in MM is a complex network of hematopoietic and non-hematopoietic cells, including stromal cells, macrophages, adipocytes, and endothelial cells, along with extracellular matrix proteins. This milieu serves as a protective niche for MM cells, promoting their proliferation, protecting against treatment, and contributing to bone lesions (63).
Thrombocytopenia is relatively uncommon in MM at diagnosis despite significant marrow infiltration. This phenomenon may be partly attributed to the thrombopoietic activity of interleukin-6 (IL-6) and elevated levels of thrombopoietin (TPO), two cytokines secreted by bone marrow mesenchymal stromal cells (MSCs) (64, 65). Studies indicate that while IL-6 supports normal platelet counts in newly diagnosed MM, elevated TPO levels are more indicative of disease progression rather than active thrombopoiesis, potentially reflecting a disrupted regulatory role in the bone marrow microenvironment (66–68).
TPO exerts its effects through binding to its receptor c-MPL on MK progenitors, regulating platelet production (69). In MM, there is no reported genetic mutation affecting c-MPL function, suggesting normal receptor activity despite thrombocytopenia. Clinical trials with Eltrombopag, a non-peptide TPO agonist, have shown promise in increasing platelet counts in MM patients (70, 71), further indicating multifactorial mechanisms in MM-associated thrombocytopenia.
Bone marrow MSCs, pivotal in supporting hematopoiesis, including thrombopoiesis (72–74), demonstrate altered functions in MM (75–78). These MSCs may contribute to impaired thrombopoiesis through dysregulated cytokine production, such as transforming growth factor-beta (TGFβ) (79), which inhibits hematopoiesis and promotes MM progression (80–82). In MM, TGFβ also enhances osteoclast activity, contributing to bone lesions (83). Furthermore, MSCs in MM display distinct transcriptomes and cytokine profiles compared to their normal counterparts (77, 78). This altered phenotype may disrupt interactions with hematopoietic stem cells (HSCs), progenitors, and MKs, potentially influencing MK function and platelet production in MM.
Interleukin-8 (IL-8), elevated in MM patients, inhibits normal megakaryopoiesis and is associated with bone lesions and altered responses to immune treatments (84–86). The inhibitory effects of IL-8 on normal megakaryopoiesis were shown in early studies in which IL-8 inhibited MK colony formation or maturation (87, 88). Thus, MSCs in MM may contribute to thrombopoiesis dysregulation through IL-8 and other cytokines, influencing disease pathogenesis and therapeutic responses. A postulated mechanism for MSC-mediated impairment of thrombopoiesis in MM is presented in Figure 1.
Figure 1. Proposed cellular interaction model for MSC-mediated impaired thrombopoiesis in MM. Within the BM microenvironment, MSCs enhance proliferation and survival of MM cells through direct adhesion (adhesion molecule signaling) or indirectly through secretion of cytokines such as IL-6 or TGFβ. Such interaction also indues secretion of cytokines such as IL-6 and extracellular vehicles (EVs) by MM cells which could, respectively, induce thrombopoiesis or suppress thrombopoiesis (through inhibition of MK colony formation and maturation) and platelet function. EVs are known to affect target cells by releasing their miRNA cargo. MM cells may interact with HSCs or HPCs (hematopoietic progenitor cells) and inhibit their proliferation or differentiation through cytokines such as TGFβ. MSCs can also secrete VEGF (vascular endothelial growth factor) which induces proliferation of ECs (endothelial cells) and angiogenesis. Another cytokine which may mediate MSC-mediated suppression of MK colony formation and maturation is IL-8. Note: It is important to mention that a plethora of cytokines is involved in the interaction between MSCs and MM cells, only cytokines related to the context are shown here.
Exosomes, small (30-150nm) extracellular vesicles (EVs) of endosomal origin, play crucial roles in normal and malignant hematopoiesis by transferring mRNAs, miRNAs, and proteins between cells. They are secreted by various cell types, including immune cells, MSCs, and tumor cells, influencing diverse cellular processes such as signaling pathways, proliferation, and migration (89).
Numerous studies have demonstrated the role of exosomes in drug resistance, angiogenesis, immune suppression, and bone lesions of MM (89). Roccaro et al. showed that exosomes released from bone marrow MSCs and transferred to MM cells play a role in disease progression. Specifically, they found a lower concentration of the tumor suppressor miR-15a and a higher concentration of CCL2, IL-6, γ-catenin, and fibronectin in exosomes derived from BM-MSCs of MM patients compared to exosomes derived from normal BM-MSCs (90).
Exosomes not only affect tumor cells directly but also modulate interactions between malignant cells and healthy hematopoietic stem and progenitor cells (HSPCs) within the bone marrow niche. They may impair normal hematopoiesis by reducing the viability and colony formation of HSPCs, particularly affecting late progenitors such as common myeloid progenitors (CMPs), megakaryocyte-erythroid progenitors (MEPs), and B and natural killer (NK) progenitors (91). Notably, elevated levels of miRNAs such as miR-34a, miR-150, miR-155, and miR-21 in HSPCs treated with MM-derived exosomes suggest a regulatory role in MK development. For instance, overexpression of miR-155 in K562 cells blocked differentiation of these cells to MK (92), and significantly reduced the number of MKs in vivo (93). miR-155 levels decreased during megakaryopoiesis in cultured human cord blood and overexpression of miR-155 impaired MK proliferation and development (94). However, increased levels of miR-34a and miR-150 favored MK proliferation and differentiation in other studies (95, 96), and miR-21 deletion significantly reduced the frequencies of CMPs, MEPs, and common lymphoid progenitors in the BM in vivo (97). These findings underscore the complex regulatory mechanisms mediated by exosomal miRNAs in MM-associated thrombopoiesis.
Of note, exosomes have heterogeneous effects on hematopoiesis in other malignancies as well. In acute myeloid leukemia (AML), EVs impair HSPC clonogenicity and induce quiescence (98), while in myelodysplastic syndromes (MDS), they exhibit contrasting effects, enhancing HSPC viability and clonogenicity (99) or inducing apoptosis and DNA damage (100). These discrepancies highlight the context-dependent nature of EV-mediated effects on hematopoiesis, influenced by different malignancies, EV isolation techniques, and the heterogeneity of EV populations.
In MM, thrombocytopenia is not solely attributed to marrow infiltration by malignant plasma cells but could also emerge from various other factors affecting thrombopoiesis. These include issues at the progenitor level, reduced megakaryopoiesis, or increased platelet consumption or destruction in circulation.
Studies indicate that as MM progresses, there is a notable decrease in MK and platelet numbers even in the absence of treatment. Elevated levels of soluble P-selectin, IL-6, and TPO in MM patients correlate with disease severity, suggesting impaired thrombopoiesis at advanced stages (101).
Research by Kuang et al. demonstrated that newly diagnosed MM patients exhibited impaired megakaryopoiesis and thrombopoiesis, which worsened with disease progression. They identified metabolic factors, particularly increased serine secretion by MM cells into the bone marrow microenvironment, as a significant contributor to MK impairment via epigenetic modifications (5).
Thrombocytopenia-related bleeding in MM patients, though uncommon at presentation, can occur more commonly with IgA paraproteins, in the presence of high concentrations of serum immunoglobulins and high serum viscosity, conditions which all affect platelet function (6, 7, 102, 103). Studies have shown a reverse correlation between platelet counts and IgG2b levels in MM patients, indicating a potential role of paraproteins in thrombopoiesis impairment (5).
Qualitative changes in platelet function, such as reduced adhesion and aggregation responses, are also observed in MM. These changes have been attributed to direct interactions between paraproteins and platelet receptors such as GPIb (von Willebrand Factor receptor) or GPVI (collagen receptor) (104), an increased concentration of malondialdehyde (a marker of oxidative stress) (105), or the effect of a highly negatively charged λ dimer (106). These results indicate that impaired platelet function mostly due to the impact of paraproteins may underlie bleeding episodes reported in some MM patients.
Moreover, studies suggest a shortened lifespan of MM platelets compared to healthy controls, potentially due to abnormal platelet function or heightened intravascular activation (107, 108), which could contribute to impaired primary hemostasis in MM.
Overall, the above findings underscore the multifactorial nature of thrombopoiesis impairment in MM, involving both external factors from MM cells and bone marrow stroma (cytokines and EVs) affecting MKs and progenitors, and intrinsic abnormalities that may contribute to isolated thrombocytopenia.
While drug therapy-related thrombosis has been discussed earlier, hypercoagulability remains a significant complication of MM itself. Studies have indicated a heightened risk of VTE in MM and monoclonal gammopathy of undetermined significance (MGUS) patients, with a hazard ratio of 3.7 and 3.4 within the first year of diagnosis, respectively (109).
Hyperactivation of platelets emerges as a critical factor contributing to this risk. Increased levels of soluble P-selectin (101) or exposure of cell surface PS (54), two indicators of platelet activation, have been reported in newly diagnosed MM. O’Sullivan et al. demonstrated that platelet hyperactivation was prevalent not only in MM but also in the precursor stages of smoldering myeloma (SM) and MGUS, suggesting a continuum of platelet dysregulation from early stages of plasma cell neoplasia (110). In another study, they also reported that platelet hyperactivation identified at diagnosis would persist during treatments (111, 112). These studies imply that platelet hyperactivation as a prothrombotic factor exists in the precursor stages of MM, however, what drives this hyperactivation with the initiation phase of plasma cell neoplasia is unclear.
Abnormalities in secondary hemostasis in untreated MM are also documented. The ROADMAP-MM-CAT study identified procoagulant phospholipid clotting time (Procoag-PPL) and endogenous thrombin potential (ETP) as independent risk factors for VTE in MM. MM patients exhibited shortened Procoag-PPL clotting times but attenuated thrombin generation in platelet-poor plasma, accompanied by elevated levels of activated tissue factor, activated factor VII, and tissue factor pathway inhibitor, all posing the risk of thrombosis (113). In another study, MM patients presented a disbalanced thrombin generation profile characterized by an increased ETP but, at the same time, prolonged lag time and time-to-peak (TTP) in whole blood thrombin generation. RBCs and platelets in whole blood were suggested as the source of this disbalanced hemostasis posing the risks of both thrombophilia and bleeding in MM patients (114).
Elevated levels of coagulation proteins including FVIII and von Willebrand factor (vWF) are reported in MM, associated with an increased risk of thrombotic complications, particularly in advanced disease stages (115). Indeed, a strong association of high FVIII and vWF levels with thrombosis risk in general has been documented (116). The mechanism behind this elevation is likely multifactorial, potentially involving endothelial cell activation induced by MM cells or bone marrow MSCs, which secrete angiogenic cytokines such as vascular endothelial growth factor (VEGF) (117).
Rare cases of bleeding diatheses due to coagulopathy, such as acquired von Willebrand syndrome (AvWS) (118, 119), acquired factor IX deficiency (120), and acquired factor X deficiency (121, 122), have also been documented in MM. In all these cases, it has been suggested that paraproteins can interact with the coagulation protein impairing its function and resulting in bleeding symptoms. Of note, amyloid proteins may also underlie acquired factor X deficiency as some patients with amyloid light-chain (AL) amyloidosis develop concomitant factor X deficiency (123).
In summary, MM-related hypercoagulability and bleeding diatheses are complex phenomena involving dysregulation of platelets, abnormalities in coagulation factors, and interactions between paraproteins and hemostatic proteins. These factors collectively contribute to the thrombotic and bleeding risks observed in MM patients.
Thrombocytopenia may develop in MM following treatment with novel agents including VRD (lenalidomide-bortezomib-dexamethasone), carfilzomib, or a combination of the four drugs (124). Autologous stem cell transplantation (ASCT) preceded by a myeloablative high-dose therapy (HDT) such as melphalan and followed by a maintenance protocol including IMiDs or PIs remains the standard of care for newly diagnosed MM patients per evidence-based recommendations (level of evidence I, mSMART 2019) (125), but thrombocytopenia continues to be one of the major adverse events in this approach. According to NCI Common Terminology Criteria for Adverse Events (CTCAE) version 5.0, thrombocytopenia of 25-50 × 10^3/µL is defined as grade 3, and <25 × 10^3/µL as grade 4. Meta-analysis of the effectiveness of PIs (126) and IMiDs (127) in the analyzed randomized controlled trials (RTCs) identified grades 3-4 thrombocytopenia in MM patients. Kroger’s study using BU (busulfan) and CY (cyclophosphamide) as the conditioning regimen for allogeneic stem cell transplantation (allo-SCT) and lenalidomide for maintenance in refractory/relapsed MM, reported grade 3-4 thrombocytopenia in 16% of patients. Furthermore, a phase IIb, multicenter, open-label study of high-dose melphalan (in a propylene glycol-free formulation) for myeloablative conditioning in MM patients undergoing ASCT reported only grade 4 thrombocytopenia in 98% of the patients (60/61) (128).
Thrombocytopenia of grades 3 or 4 may culminate in life-threatening hemorrhage if not managed properly and in a timely manner. If platelet transfusion is decided, it should follow established guidelines, such as those provided by the American Society of Clinical Oncologists (level of evidence II) (129). Although it is recommended that invasive procedures be minimized in MM patients with thrombocytopenia, a platelet count of 40 × 10^3/µL to 50 × 10^3/µL is considered safe for proceeding with invasive procedures provided that the patient does not present any other coagulation abnormalities (129). The consensus statement of the International Myeloma Foundation (IMF) Nurse Leadership Board also recommends that MM patients with thrombocytopenia avoid taking drugs that impair PLT function such as aspirin, ibuprofen, or naproxen unless otherwise instructed (e.g., for prophylaxis of thromboembolic events); avoid activities that can result in bleeding or bruising such as contact sports or tattooing; and use soft sponges and non-abrasive toothpaste for their oral care (130).
Thrombotic events related to drugs such as IMiDs also require robust clinical management. A recent publication of the Intergroupe Francophone du Mye’lome (IFM) group recommends thromboprophylaxis using low molecular weight heparin (LMWH) or direct oral anticoagulants (DOACs) in MM patients who receive IMiDs combined with dexamethasone (131). However, the dose of LMWH or DOACs needs to be adjusted depending on renal function using the creatine clearance (CrCl) test. If CrCl is >30mL/min, no adjustment is required but if it is below 15mL/min, none of these anti-coagulants should be used and the patient must receive unfractionated heparin (UFH) instead (131). Following the International Myeloma Working Group (IMWG) guidelines, the choice of prophylactic treatment should be made on the basis of risk factors including those related to therapy, individual (patient), and active myeloma per se among others (132). They recommend prophylactic dose LMWH or full-dose warfarin (target INR 2-3) for MM patients who have at least one therapy-related or at least two patient- or myeloma-specific risk factors, and aspirin for other patients who may be at lower risk. DOACs such as apixaban and rivaroxaban, the new generation of oral anticoagulants, were not included in the IMWG guidelines. Although most phase III RCTs employing DOACs did not include MM patients, small studies have shown their promising effectiveness as substitutes to LMWH and warfarin which are inconvenient due to parenteral route and need for INR monitoring, respectively (133, 134).
Other than thrombotic events related to IMiDs, targeted therapy-associated cardiovascular adverse events have also been reported in MM following treatments with monoclonal antibodies and PIs. Two separate disproportionality analyses on reports from the FDA Adverse Event Reporting System (FAERS) database identified daratumumab, elotuzumab, isatuximab, and panobinostat to be mostly associated with cardiotoxicity (135, 136). Another study using the same database reported a significant disproportional association between carfilzomib and cardiovascular events in MM patients (137). Although further prospective studies are required, the above reports highlight the importance of closely monitoring MM patients under these treatments, particularly if they present with a cardiac history.
Finally, impaired function of PLTs or coagulation factors unrelated to therapy could also contribute to bleeding risk in MM patients. Thus, comprehensive hemostatic assessments for newly diagnosed or untreated MM patients are crucial to identify underlying primary or secondary hemostasis abnormalities. These assessments should include coagulation factor assays, intrinsic coagulation inhibitor assays, or platelet function studies, particularly aggregometry. Early identification and management of these disorders can improve clinical outcomes and reduce the risk of further complications during treatment.
In the realm of research, several gaps remain in our understanding of how MM impacts coagulation factors during its oncogenic process, how it impairs platelet function, and crucially, the molecular mechanisms driving MSC-mediated impaired thrombopoiesis. Investigating thrombopoiesis in in vitro settings using co-culture models of MM cells with HSCs, especially in the presence of MSCs from MM patients’ bone marrow, could help elucidate the cellular and molecular mechanisms underlying acquired hemostatic abnormalities in MM. Addressing these gaps will not only advance our understanding of MM-associated hemostatic disorders but also pave the way for developing more effective therapies tailored to these specific pathophysiological mechanisms. Future research should focus on targeted therapies that modulate the bone marrow microenvironment and improve platelet production and function in MM patients. This could include exploring the therapeutic potential of MSCs and exosomes and the development of novel drugs that target specific pathways involved in thrombopoiesis and coagulation.
JA: Conceptualization, Supervision, Writing – original draft, Writing – review & editing. PN: Writing – review & editing.
The author(s) declare financial support was received for the research, authorship, and/or publication of this article. This study was financially possible by an internal grant support from College of Health, Human Services, and Nursing at California State University Dominguez Hills.
The authors declare that the research was conducted in the absence of any commercial or financial relationships that could be construed as a potential conflict of interest.
All claims expressed in this article are solely those of the authors and do not necessarily represent those of their affiliated organizations, or those of the publisher, the editors and the reviewers. Any product that may be evaluated in this article, or claim that may be made by its manufacturer, is not guaranteed or endorsed by the publisher.
1. Heider M, Nickel K, Hogner M, Bassermann F. Multiple myeloma: molecular pathogenesis and disease evolution. Oncol Res Treat. (2021) 44:672–81. doi: 10.1159/000520312
2. Mellors P, Binder M, Buadi FK, Lacy MQ, Gertz MA, Dispenzieri A, et al. Development of thrombocytopenia and survival outcomes in newly diagnosed multiple myeloma. Blood. (2018) 132:1902–2. doi: 10.1182/blood-2018-99-112138
3. Shaw JL, Nielson CM, Park JK, Marongiu A, Soff GA. The incidence of thrombocytopenia in adult patients receiving chemotherapy for solid tumors or hematologic Malignancies. Eur J Haematol. (2021) 106:662–72. doi: 10.1111/ejh.v106.5
4. Bruns I, Cadeddu RP, Brueckmann I, Frobel J, Geyh S, Bust S, et al. Multiple myeloma-related deregulation of bone marrow-derived CD34(+) hematopoietic stem and progenitor cells. Blood. (2012) 120:2620–30. doi: 10.1182/blood-2011-04-347484
5. Kuang C, Xia M, An G, Liu C, Hu C, Zhang J, et al. Excessive serine from the bone marrow microenvironment impairs megakaryopoiesis and thrombopoiesis in Multiple Myeloma. Nat Commun. (2023) 14:2093. doi: 10.1038/s41467-023-37699-z
6. Saif MW, Allegra CJ, Greenberg B. Bleeding diathesis in multiple myeloma. J Hematother Stem Cell Res. (2001) 10:657–60. doi: 10.1089/152581601753193869
7. Mortimer J, Gray N, Desborough M, Baker P, Kothari J, Eyre T. Acquired platelet dysfunction in newly diagnosed myeloma. EJHaem. (2021) 2:17–9. doi: 10.1002/jha2.v2.1
8. Plesner T, Arkenau HT, Gimsing P, Krejcik J, Lemech C, Minnema MC, et al. Phase 1/2 study of daratumumab, lenalidomide, and dexamethasone for relapsed multiple myeloma. Blood. (2016) 128:1821–8. doi: 10.1182/blood-2016-07-726729
9. Hindie E. Teclistamab in relapsed or refractory multiple myeloma. N Engl J Med. (2022) 387:1721. doi: 10.1056/NEJMc2211969
10. Lonial S, Waller EK, Richardson PG, Jagannath S, Orlowski RZ, Giver CR, et al. Risk factors and kinetics of thrombocytopenia associated with bortezomib for relapsed, refractory multiple myeloma. Blood. (2005) 106:3777–84. doi: 10.1182/blood-2005-03-1173
11. Liu F, Morris S, Epps J, Carroll R. Demonstration of an activation regulated NF-kappaB/I-kappaBalpha complex in human platelets. Thromb Res. (2002) 106:199–203. doi: 10.1016/S0049-3848(02)00130-5
12. Wang SY, Shih YH, Shieh TM, Tseng YH. Proteasome inhibitors interrupt the activation of non-canonical NF-kappaB signaling pathway and induce cell apoptosis in cytarabine-resistant HL60 cells. Int J Mol Sci. (2021) 23(1):361. doi: 10.3390/ijms23010361
13. Xie H, Gu Y, Wang W, Wang X, Ye X, Xin C, et al. Silencing of SENP2 in multiple myeloma induces bortezomib resistance by activating NF-kappaB through the modulation of ikappaBalpha sumoylation. Sci Rep. (2020) 10:766. doi: 10.1038/s41598-020-57698-0
14. Kojok K, El-Kadiry AE, Merhi Y. Role of NF-kappaB in platelet function. Int J Mol Sci. (2019) 20(17):4185. doi: 10.3390/ijms20174185
15. Spinelli SL, Casey AE, Pollock SJ, Gertz JM, McMillan DH, Narasipura SD, et al. Platelets and megakaryocytes contain functional nuclear factor-kappaB. Arterioscler Thromb Vasc Biol. (2010) 30:591–8. doi: 10.1161/ATVBAHA.109.197343
16. Damien P, Cognasse F, Payrastre B, Spinelli SL, Blumberg N, Arthaud CA, et al. NF-kappaB links TLR2 and PAR1 to soluble immunomodulator factor secretion in human platelets. Front Immunol. (2017) 8:85. doi: 10.3389/fimmu.2017.00085
17. Colberg L, Cammann C, Wesche J, Topfstedt E, Seifert U, Greinacher A. The platelet proteasome and immunoproteasome are stable in buffy-coat derived platelet concentrates for up to 7 days. Transfusion. (2021) 61:2746–55. doi: 10.1111/trf.16605
18. Murai K, Kowata S, Shimoyama T, Yashima-Abo A, Fujishima Y, Ito S, et al. Bortezomib induces thrombocytopenia by the inhibition of proplatelet formation of megakaryocytes. Eur J Haematol. (2014) 93:290–6. doi: 10.1111/ejh.2014.93.issue-4
19. Shi DS, Smith MC, Campbell RA, Zimmerman PW, Franks ZB, Kraemer BF, et al. Proteasome function is required for platelet production. J Clin Invest. (2014) 124:3757–66. doi: 10.1172/JCI75247
20. Lignet F, Becker AD, Gimmi C, Rohdich F, El Bawab S. Using thrombocytopenia modeling to investigate the mechanisms underlying platelet depletion induced by pan-proteasome inhibitors. CPT Pharmacometrics Syst Pharmacol. (2022) 11:594–603. doi: 10.1002/psp4.12743
21. Baaten C, Moenen F, Henskens YMC, Swieringa F, Wetzels RJH, van Oerle R, et al. Impaired mitochondrial activity explains platelet dysfunction in thrombocytopenic cancer patients undergoing chemotherapy. Haematologica. (2018) 103:1557–67. doi: 10.3324/haematol.2017.185165
22. Prasad HK, Kaushal V, Mehta P. Isolated thrombocytopenia induced by thalidomide in a patient with multiple myeloma: case report and review of literature. Am J Hematol. (2007) 82:855–7. doi: 10.1002/ajh.20949
23. Akane Ryuge YK, Mizutani T, Park JiY, Shimizu K. A possible link between ITP developed in a patient with relapsed multiple myeloma and maintenance thalidomide. Ann Oncol. (2015) 26(Supplement 7):vii110. doi: 10.1093/annonc/mdv472.22
24. Forehand Iii W, Ajebo G, Toscano M, Jillella A, Dainer P. Lenalidomide-associated immune thrombocytopenia: A case report and review of the literature. Case Rep Hematol. (2020) 2020:8825618. doi: 10.1155/2020/8825618
25. Mulder FI, Horvath-Puho E, van Es N, van Laarhoven HWM, Pedersen L, Moik F, et al. Venous thromboembolism in cancer patients: a population-based cohort study. Blood. (2021) 137:1959–69. doi: 10.1182/blood.2020007338
26. Facon T, Kumar S, Plesner T, Orlowski RZ, Moreau P, Bahlis N, et al. Daratumumab plus lenalidomide and dexamethasone for untreated myeloma. N Engl J Med. (2019) 380:2104–15. doi: 10.1056/NEJMoa1817249
27. De Stefano V, Larocca A, Carpenedo M, Cavo M, Di Raimondo F, Falanga A, et al. Thrombosis in multiple myeloma: risk stratification, antithrombotic prophylaxis, and management of acute events. A consensus-based position paper from an. ad hoc Expert panel Haematologica. (2022) 107:2536–47. doi: 10.3324/haematol.2022.280893
28. Schoen MW, Carson KR, Luo S, Gage BF, Li A, Afzal A, et al. Venous thromboembolism in multiple myeloma is associated with increased mortality. Res Pract Thromb Haemost. (2020) 4:1203–10. doi: 10.1002/rth2.12411
29. Bradbury CA, Craig Z, Cook G, Pawlyn C, Cairns DA, Hockaday A, et al. Thrombosis in patients with myeloma treated in the Myeloma IX and Myeloma XI phase 3 randomized controlled trials. Blood. (2020) 136:1091–104. doi: 10.1182/blood.2020005125
30. Fouquet G, Tardy S, Demarquette H, Bonnet S, Gay J, Debarri H, et al. Efficacy and safety profile of long-term exposure to lenalidomide in patients with recurrent multiple myeloma. Cancer. (2013) 119:3680–6. doi: 10.1002/cncr.v119.20
31. Richardson PG, Sonneveld P, Schuster MW, Irwin D, Stadtmauer EA, Facon T, et al. Bortezomib or high-dose dexamethasone for relapsed multiple myeloma. N Engl J Med. (2005) 352:2487–98. doi: 10.1056/NEJMoa043445
32. San Miguel JF, Schlag R, Khuageva NK, Dimopoulos MA, Shpilberg O, Kropff M, et al. Bortezomib plus melphalan and prednisone for initial treatment of multiple myeloma. N Engl J Med. (2008) 359:906–17. doi: 10.1056/NEJMoa0801479
33. Stewart AK, Rajkumar SV, Dimopoulos MA, Masszi T, Spicka I, Oriol A, et al. Carfilzomib, lenalidomide, and dexamethasone for relapsed multiple myeloma. N Engl J Med. (2015) 372:142–52. doi: 10.1056/NEJMoa1411321
34. Rajkumar SV, Jacobus S, Callander NS, Fonseca R, Vesole DH, Williams ME, et al. Lenalidomide plus high-dose dexamethasone versus lenalidomide plus low-dose dexamethasone as initial therapy for newly diagnosed multiple myeloma: an open-label randomised controlled trial. Lancet Oncol. (2010) 11:29–37. doi: 10.1016/S1470-2045(09)70284-0
35. Piedra K, Peterson T, Tan C, Orozco J, Hultcrantz M, Hassoun H, et al. Comparison of venous thromboembolism incidence in newly diagnosed multiple myeloma patients receiving bortezomib, lenalidomide, dexamethasone (RVD) or carfilzomib, lenalidomide, dexamethasone (KRD) with aspirin or rivaroxaban thromboprophylaxis. Br J Haematol. (2022) 196:105–9. doi: 10.1111/bjh.v196.1
36. Li A, Wu Q, Warnick G, Li S, Libby EN, Garcia DA, et al. The incidence of thromboembolism for lenalidomide versus thalidomide in older patients with newly diagnosed multiple myeloma. Ann Hematol. (2020) 99:121–6. doi: 10.1007/s00277-019-03860-2
37. Richardson P, Jagannath S, Hussein M, Berenson J, Singhal S, Irwin D, et al. Safety and efficacy of single-agent lenalidomide in patients with relapsed and refractory multiple myeloma. Blood. (2009) 114:772–8. doi: 10.1182/blood-2008-12-196238
38. Rushworth GF, Leslie SJ, Forsyth P, Vincent C. Evidence-based case report: multiple thrombotic episodes associated with lenalidomide and dexamethasone therapy for multiple myeloma. Ther Adv Drug Saf. (2012) 3:115–22. doi: 10.1177/2042098611433773
39. Stawiarski KM, Patil G, Witt D, Pollack A. Pulmonary vein thrombosis in a patient with multiple myeloma on treatment with lenalidomide. World J Oncol. (2021) 12:73–6. doi: 10.14740/wjon1384
40. Carrier M, Le Gal G, Tay J, Wu C, Lee AY. Rates of venous thromboembolism in multiple myeloma patients undergoing immunomodulatory therapy with thalidomide or lenalidomide: a systematic review and meta-analysis. J Thromb Haemost. (2011) 9:653–63. doi: 10.1111/j.1538-7836.2011.04215.x
41. De Stefano V, Za T, Rossi E. Venous thromboembolism in multiple myeloma. Semin Thromb Hemost. (2014) 40:338–47. doi: 10.1055/s-0034-1370793
42. Li P, Xu B, Xu J, Xu Y, Wang Y, Chen C, et al. Lenalidomide promotes thrombosis formation, but does not affect platelet activation in multiple myeloma. Int J Mol Sci. (2023) 24(18):14097. doi: 10.3390/ijms241814097
43. Robak M, Trelinski J, Chojnowski K. Hemostatic changes after 1 month of thalidomide and dexamethasone therapy in patients with multiple myeloma. Med Oncol. (2012) 29:3574–80. doi: 10.1007/s12032-012-0290-0
44. Osman K, Comenzo R, Rajkumar SV. Deep venous thrombosis and thalidomide therapy for multiple myeloma. N Engl J Med. (2001) 344:1951–2. doi: 10.1056/NEJM200106213442516
45. Corso A, Lorenzi A, Terulla V, Airo F, Varettoni M, Mangiacavalli S, et al. Modification of thrombomodulin plasma levels in refractory myeloma patients during treatment with thalidomide and dexamethasone. Ann Hematol. (2004) 83:588–91. doi: 10.1007/s00277-004-0891-6
46. Ito T, Thachil J, Asakura H, Levy JH, Iba T. Thrombomodulin in disseminated intravascular coagulation and other critical conditions-a multi-faceted anticoagulant protein with therapeutic potential. Crit Care. (2019) 23:280. doi: 10.1186/s13054-019-2552-0
47. Pal R, Monaghan SA, Hassett AC, Mapara MY, Schafer P, Roodman GD, et al. Immunomodulatory derivatives induce PU.1 down-regulation, myeloid maturation arrest, and neutropenia. Blood. (2010) 115:605–14. doi: 10.1182/blood-2009-05-221077
48. Bekendam RH, Ravid K. Mechanisms of platelet activation in cancer-associated thrombosis: a focus on myeloproliferative neoplasms. Front Cell Dev Biol. (2023) 11:1207395. doi: 10.3389/fcell.2023.1207395
49. Zhang S, Liu Y, Wang X, Yang L, Li H, Wang Y, et al. SARS-CoV-2 binds platelet ACE2 to enhance thrombosis in COVID-19. J Hematol Oncol. (2020) 13:120. doi: 10.1186/s13045-020-00954-7
50. Dunkley S, Gaudry L. Thalidomide causes platelet activation, which can be abrogated by aspirin. J Thromb Haemost. (2007) 5:1323–5. doi: 10.1111/j.1538-7836.2007.02542.x
51. Shen L, Yang T, Xia K, Yan Z, Tan J, Li L, et al. P-selectin (CD62P) and soluble TREM-like transcript-1 (sTLT-1) are associated with coronary artery disease: a case control study. BMC Cardiovasc Disord. (2020) 20:387. doi: 10.1186/s12872-020-01663-2
52. Pluta K, Porebska K, Urbanowicz T, Gasecka A, Olasinska-Wisniewska A, Targonski R, et al. Platelet-leucocyte aggregates as novel biomarkers in cardiovascular diseases. Biol (Basel). (2022) 11(2):224. doi: 10.3390/biology11020224
53. Isozumi Y, Arai R, Fujimoto K, Koyama T. Activation of coagulation by lenalidomide-based regimens for the treatment of multiple myeloma. PloS One. (2013) 8:e64369. doi: 10.1371/journal.pone.0064369
54. Guo L, Tong D, Yu M, Zhang Y, Li T, Wang C, et al. Phosphatidylserine-exposing cells contribute to the hypercoagulable state in patients with multiple myeloma. Int J Oncol. (2018) 52:1981–90. doi: 10.3892/ijo.2018.4354
55. Nomura S, Ito T, Yoshimura H, Hotta M, Nakanishi T, Fujita S, et al. Evaluation of thrombosis-related biomarkers before and after therapy in patients with multiple myeloma. J Blood Med. (2018) 9:1–7. doi: 10.2147/JBM.S147743
56. Zangari M, Fink L, Zhan F, Tricot G. Low venous thromboembolic risk with bortezomib in multiple myeloma and potential protective effect with thalidomide/lenalidomide-based therapy: review of data from phase 3 trials and studies of novel combination regimens. Clin Lymphoma Myeloma Leuk. (2011) 11:228–36. doi: 10.1016/j.clml.2011.03.006
57. Kumar SK, Jacobus SJ, Cohen AD, Weiss M, Callander N, Singh AK, et al. Carfilzomib or bortezomib in combination with lenalidomide and dexamethasone for patients with newly diagnosed multiple myeloma without intention for immediate autologous stem-cell transplantation (ENDURANCE): a multicentre, open-label, phase 3, randomised, controlled trial. Lancet Oncol. (2020) 21:1317–30. doi: 10.1016/S1470-2045(20)30452-6
58. Fehaid Alanazi IG, Burbury KL, Kwa FAA, Dobie G, Kuriri FA, Abdalhabib EK, et al. Carfilzomib potentiates platelet activation and thrombus growth. J Blood Disord Transfus. (2022) 13(8):521. doi: 10.4172/2155-9864.22.13.521
59. Moscvin M, Liacos CI, Chen T, Theodorakakou F, Fotiou D, Hossain S, et al. Mutations in the alternative complement pathway in multiple myeloma patients with carfilzomib-induced thrombotic microangiopathy. Blood Cancer J. (2023) 13:31. doi: 10.1038/s41408-023-00802-0
60. Libourel EJ, Sonneveld P, van der Holt B, de Maat MP, Leebeek FW. High incidence of arterial thrombosis in young patients treated for multiple myeloma: results of a prospective cohort study. Blood. (2010) 116:22–6. doi: 10.1182/blood-2009-12-257519
61. Zangari M, Siegel E, Barlogie B, Anaissie E, Saghafifar F, Fassas A, et al. Thrombogenic activity of doxorubicin in myeloma patients receiving thalidomide: implications for therapy. Blood. (2002) 100:1168–71. doi: 10.1182/blood-2002-01-0335
62. Kim SH, Lim KM, Noh JY, Kim K, Kang S, Chang YK, et al. Doxorubicin-induced platelet procoagulant activities: an important clue for chemotherapy-associated thrombosis. Toxicol Sci. (2011) 124:215–24. doi: 10.1093/toxsci/kfr222
63. Garcia-Ortiz A, Rodriguez-Garcia Y, Encinas J, Maroto-Martin E, Castellano E, Teixido J, et al. The role of tumor microenvironment in multiple myeloma development and progression. Cancers (Basel). (2021) 13(2):217. doi: 10.3390/cancers13020217
64. Harmer D, Falank C, Reagan MR. Interleukin-6 interweaves the bone marrow microenvironment, bone loss, and multiple myeloma. Front Endocrinol (Lausanne). (2018) 9:788. doi: 10.3389/fendo.2018.00788
65. Ye Jyu, Liang Ey, Li Suyi, Chan GC, Yang Mo. Serotonin regulates TPO production from bone marrow mesenchymal stromal cells. Blood. (2013) 122:3690–0. doi: 10.1182/blood.V122.21.3690.3690
66. Ozkurt ZN, Yagci M, Sucak GT, Kirazli S, Haznedar R. Thrombopoietic cytokines and platelet count in multiple myeloma. Platelets. (2010) 21:33–6. doi: 10.3109/09537100903360007
67. Kaminska J, Koper OM, Mantur M, Matowicka-Karna J, Sawicka-Powierza J, Sokolowski J, et al. Does thrombopoiesis in multiple myeloma patients depend on the stage of the disease? Adv Med Sci. (2014) 59:166–71.
68. Lee JJ, Kang SY, Lee WI. The analysis of thrombopoietin and clinical parameters as a marker for disease progression in patients with multiple myeloma. Korean J Lab Med. (2009) 29:82–8. doi: 10.3343/kjlm.2009.29.1.82
69. Bhat FA, Advani J, Khan AA, Mohan S, Pal A, Gowda H, et al. A network map of thrombopoietin signaling. J Cell Commun Signal. (2018) 12:737–43. doi: 10.1007/s12079-018-0480-4
70. Hagihara M, Inoue M, Kodama K, Uchida T, Hua J. Simultaneous manifestation of chronic myelomonocytic leukemia and multiple myeloma during treatment by prednisolone and eltrombopag for immune-mediated thrombocytopenic purpura. Case Rep Hematol. (2016) 2016:4342820. doi: 10.1155/2016/4342820
71. Jeong JY, Levine MS, Abayasekara N, Berliner N, Laubach J, Vanasse GJ. The non-peptide thrombopoietin receptor agonist eltrombopag stimulates megakaryopoiesis in bone marrow cells from patients with relapsed multiple myeloma. J Hematol Oncol. (2015) 8:37. doi: 10.1186/s13045-015-0136-2
72. Feng Y, Gao L, Chen X. Hematopoietic stromal cells and megakaryocyte development. Hematology. (2011) 16:67–72. doi: 10.1179/102453311X12940641877920
73. Goette NP, Borzone FR, Discianni Lupi AD, Chasseing NA, Rubio MF, Costas MA, et al. Megakaryocyte-stromal cell interactions: Effect on megakaryocyte proliferation, proplatelet production, and survival. Exp Hematol. (2022) 107:24–37. doi: 10.1016/j.exphem.2022.01.002
74. Mendelson A, Strat AN, Bao W, Rosston P, Fallon G, Ohrn S, et al. Mesenchymal stromal cells lower platelet activation and assist in platelet formation. vitro JCI Insight. (2019) 4(16):e126982. doi: 10.1172/jci.insight.126982
75. Corre J, Mahtouk K, Attal M, Gadelorge M, Huynh A, Fleury-Cappellesso S, et al. Bone marrow mesenchymal stem cells are abnormal in multiple myeloma. Leukemia. (2007) 21:1079–88. doi: 10.1038/sj.leu.2404621
76. Zdzisinska B, Bojarska-Junak A, Dmoszynska A, Kandefer-Szerszen M. Abnormal cytokine production by bone marrow stromal cells of multiple myeloma patients in response to RPMI8226 myeloma cells. Arch Immunol Ther Exp (Warsz). (2008) 56:207–21. doi: 10.1007/s00005-008-0022-5
77. Alameda D, Saez B, Lara-Astiaso D, Sarvide S, Lasa M, Alignani D, et al. Characterization of freshly isolated bone marrow mesenchymal stromal cells from healthy donors and patients with multiple myeloma: transcriptional modulation of the microenvironment. Haematologica. (2020) 105:e470–473. doi: 10.3324/haematol.2019.235135
78. Arnulf B, Lecourt S, Soulier J, Ternaux B, Lacassagne MN, Crinquette A, et al. Phenotypic and functional characterization of bone marrow mesenchymal stem cells derived from patients with multiple myeloma. Leukemia. (2007) 21:158–63. doi: 10.1038/sj.leu.2404466
79. Rana PS, Soler DC, Kort J, Driscoll JJ. Targeting TGF-beta signaling in the multiple myeloma microenvironment: Steering CARs and T cells in the right direction. Front Cell Dev Biol. (2022) 10:1059715. doi: 10.3389/fcell.2022.1059715
80. Scandura JM, Boccuni P, Massague J, Nimer SD. Transforming growth factor beta-induced cell cycle arrest of human hematopoietic cells requires p57KIP2 up-regulation. Proc Natl Acad Sci U S A. (2004) 101:15231–6. doi: 10.1073/pnas.0406771101
81. Chabanon A, Desterke C, Rodenburger E, Clay D, Guerton B, Boutin L, et al. A cross-talk between stromal cell-derived factor-1 and transforming growth factor-beta controls the quiescence/cycling switch of CD34(+) progenitors through FoxO3 and mammalian target of rapamycin. Stem Cells. (2008) 26:3150–61. doi: 10.1634/stemcells.2008-0219
82. Budhu S, Schaer DA, Li Y, Toledo-Crow R, Panageas K, Yang X, et al. Blockade of surface-bound TGF-beta on regulatory T cells abrogates suppression of effector T cell function in the tumor microenvironment. Sci Signal. (2017) 10(494):eaak9702. doi: 10.1126/scisignal.aak9702
83. Lu A, Pallero MA, Lei W, Hong H, Yang Y, Suto MJ, et al. Inhibition of transforming growth factor-beta activation diminishes tumor progression and osteolytic bone disease in mouse models of multiple myeloma. Am J Pathol. (2016) 186:678–90. doi: 10.1016/j.ajpath.2015.11.003
84. Robak P, Weglowska E, Drozdz I, Mikulski D, Jarych D, Ferlinska M, et al. Cytokine and chemokine profile in patients with multiple myeloma treated with bortezomib. Mediators Inflamm. (2020) 2020:1835836. doi: 10.1155/2020/1835836
85. Herrero AB, Garcia-Gomez A, Garayoa M, Corchete LA, Hernandez JM, San Miguel J, et al. Effects of IL-8 up-regulation on cell survival and osteoclastogenesis in multiple myeloma. Am J Pathol. (2016) 186:2171–82. doi: 10.1016/j.ajpath.2016.04.003
86. Mekhloufi A, Kosta A, Stabile H, Molfetta R, Zingoni A, Soriani A, et al. Bone marrow stromal cell-derived IL-8 upregulates PVR expression on multiple myeloma cells via NF-kB transcription factor. Cancers (Basel). (2020) 12(2):440. doi: 10.3390/cancers12020440
87. Cortin V, Garnier A, Pineault N, Lemieux R, Boyer L, Proulx C. Efficient in vitro megakaryocyte maturation using cytokine cocktails optimized by statistical experimental design. Exp Hematol. (2005) 33:1182–91. doi: 10.1016/j.exphem.2005.06.020
88. Gewirtz AM, Zhang J, Ratajczak J, Ratajczak M, Park KS, Li C, et al. Chemokine regulation of human megakaryocytopoiesis. Blood. (1995) 86:2559–67. doi: 10.1182/blood.V86.7.2559.2559
89. Menu E, Vanderkerken K. Exosomes in multiple myeloma: from bench to bedside. Blood. (2022) 140:2429–42. doi: 10.1182/blood.2021014749
90. Roccaro AM, Sacco A, Maiso P, Azab AK, Tai YT, Reagan M, et al. BM mesenchymal stromal cell-derived exosomes facilitate multiple myeloma progression. J Clin Invest. (2013) 123:1542–55. doi: 10.1172/JCI66517
91. Laurenzana I, Trino S, Lamorte D, De Stradis A, Santodirocco M, Sgambato A, et al. Multiple myeloma-derived extracellular vesicles impair normal hematopoiesis by acting on hematopoietic stem and progenitor cells. Front Med (Lausanne). (2021) 8:793040. doi: 10.3389/fmed.2021.793040
92. Georgantas RW 3rd, Hildreth R, Morisot S, Alder J, Cg L, Heimfeld S, et al. CD34+ hematopoietic stem-progenitor cell microRNA expression and function: a circuit diagram of differentiation control. Proc Natl Acad Sci U S A. (2007) 104:2750–5. doi: 10.1073/pnas.0610983104
93. O'Connell RM, Rao DS, Chaudhuri AA, Boldin MP, Taganov KD, Nicoll J, et al. Sustained expression of microRNA-155 in hematopoietic stem cells causes a myeloproliferative disorder. J Exp Med. (2008) 205:585–94. doi: 10.1084/jem.20072108
94. Romania P, Lulli V, Pelosi E, Biffoni M, Peschle C, Marziali G. MicroRNA 155 modulates megakaryopoiesis at progenitor and precursor level by targeting Ets-1 and Meis1 transcription factors. Br J Haematol. (2008) 143:570–80. doi: 10.1111/j.1365-2141.2008.07382.x
95. Lu J, Guo S, Ebert BL, Zhang H, Peng X, Bosco J, et al. MicroRNA-mediated control of cell fate in megakaryocyte-erythrocyte progenitors. Dev Cell. (2008) 14:843–53. doi: 10.1016/j.devcel.2008.03.012
96. Navarro F, Gutman D, Meire E, Caceres M, Rigoutsos I, Bentwich Z, et al. miR-34a contributes to megakaryocytic differentiation of K562 cells independently of p53. Blood. (2009) 114:2181–92. doi: 10.1182/blood-2009-02-205062
97. Hu M, Lu Y, Zeng H, Zhang Z, Chen S, Qi Y, et al. MicroRNA-21 maintains hematopoietic stem cell homeostasis through sustaining the NF-kappaB signaling pathway in mice. Haematologica. (2021) 106:412–23. doi: 10.3324/haematol.2019.236927
98. Abdelhamed S, Butler JT, Doron B, Halse A, Nemecek E, Wilmarth PA, et al. Extracellular vesicles impose quiescence on residual hematopoietic stem cells in the leukemic niche. EMBO Rep. (2019) 20:e47546. doi: 10.15252/embr.201847546
99. Muntion S, Ramos TL, Diez-Campelo M, Roson B, Sanchez-Abarca LI, Misiewicz-Krzeminska I, et al. Microvesicles from mesenchymal stromal cells are involved in HPC-microenvironment crosstalk in myelodysplastic patients. PloS One. (2016) 11:e0146722. doi: 10.1371/journal.pone.0146722
100. Meunier M, Guttin A, Ancelet S, Laurin D, Zannoni J, Lefebvre C, et al. Extracellular vesicles from myelodysplastic mesenchymal stromal cells induce DNA damage and mutagenesis of hematopoietic stem cells through miRNA transfer. Leukemia. (2020) 34:2249–53. doi: 10.1038/s41375-020-0738-8
101. Lemancewicz D, Bolkun L, Mantur M, Semeniuk J, Kloczko J, Dzieciol J. Bone marrow megakaryocytes, soluble P-selectin and thrombopoietic cytokines in multiple myeloma patients. Platelets. (2014) 25:181–7. doi: 10.3109/09537104.2013.805405
102. Kwaan HC. Hyperviscosity in plasma cell dyscrasias. Clin Hemorheol Microcirc. (2013) 55:75–83. doi: 10.3233/CH-131691
103. Djunic I, Elezovic I, Ilic V, Milosevic-Jovcic N, Bila J, Suvajdzic-Vukovic N, et al. The effect of paraprotein on platelet aggregation. J Clin Lab Anal. (2014) 28:141–6. doi: 10.1002/jcla.2014.28.issue-2
104. Djunic I, Elezovic I, Vucic M, Srdic-Rajic T, Konic-Ristic A, Ilic V, et al. Specific binding of paraprotein to platelet receptors as a cause of platelet dysfunction in monoclonal gammopathies. Acta Haematol. (2013) 130:101–7. doi: 10.1159/000345418
105. Cieslar P, Masova L, Scheiner T, Rysava J, Krizova P, Danzigova Z, et al. Oxidative stress and platelet function in multiple myeloma and renal insufficiency: clinical relations of different tests. Thromb Res. (2002) 105:277–83. doi: 10.1016/S0049-3848(02)00003-8
106. Shinagawa A, Kojima H, Berndt MC, Kaneko S, Suzukawa K, Hasegawa Y, et al. Characterization of a myeloma patient with a life-threatening hemorrhagic diathesis: presence of a lambda dimer protein inhibiting shear-induced platelet aggregation by binding to the A1 domain of von Willebrand factor. Thromb Haemost. (2005) 93:889–96. doi: 10.1160/TH04-03-0193
107. Fritz E, Ludwig H, Scheithauer W, Sinzinger H. Shortened platelet half-life in multiple myeloma. Blood. (1986) 68:514–20. doi: 10.1182/blood.V68.2.514.514
108. Gorski J, Luka K, Czestochowska E. Platelet function and survival in multiple myeloma. Haematologia (Budap). (1993) 25:131–5.
109. Kristinsson SY, Pfeiffer RM, Bjorkholm M, Goldin LR, Schulman S, Blimark C, et al. Arterial and venous thrombosis in monoclonal gammopathy of undetermined significance and multiple myeloma: a population-based study. Blood. (2010) 115:4991–8. doi: 10.1182/blood-2009-11-252072
110. Sloos PH, Vulliamy P, van 't Veer C, Gupta AS, Neal MD, Brohi K, et al. Platelet dysfunction after trauma: From mechanisms to targeted treatment. Transfusion. (2022) 62 Suppl 1:S281–300. doi: 10.1111/trf.v62.S1
111. O'Sullivan LR, Meade-Murphy G, Gilligan OM, Mykytiv V, Cahill MR, Young PW. Platelet hyperactivation and hyporesponsiveness at diagnosis in multiple myeloma persists during treatment initiation. Thromb Res. (2021) 203:186–9. doi: 10.1016/j.thromres.2021.05.004
112. O'Sullivan LR, Meade-Murphy G, Gilligan OM, Mykytiv V, Young PW, Cahill MR. Platelet hyperactivation in multiple myeloma is also evident in patients with premalignant monoclonal gammopathy of undetermined significance. Br J Haematol. (2021) 192:322–32. doi: 10.1111/bjh.v192.2
113. Fotiou D, Sergentanis TN, Papageorgiou L, Stamatelopoulos K, Gavriatopoulou M, Kastritis E, et al. Longer procoagulant phospholipid-dependent clotting time, lower endogenous thrombin potential and higher tissue factor pathway inhibitor concentrations are associated with increased VTE occurrence in patients with newly diagnosed multiple myeloma: results of the prospective ROADMAP-MM-CAT study. Blood Cancer J. (2018) 8:102. doi: 10.1038/s41408-018-0135-y
114. Li L, Roest M, Sang Y, Remijn JA, Fijnheer R, Smit K, et al. Patients with multiple myeloma have a disbalanced whole blood thrombin generation profile. Front Cardiovasc Med. (2022) 9:919495. doi: 10.3389/fcvm.2022.919495
115. Auwerda JJ, Sonneveld P, de Maat MP, Leebeek FW. Prothrombotic coagulation abnormalities in patients with newly diagnosed multiple myeloma. Haematologica. (2007) 92:279–80. doi: 10.3324/haematol.10454
116. Rietveld IM, Lijfering WM, le Cessie S, Bos MHA, Rosendaal FR, Reitsma PH, et al. High levels of coagulation factors and venous thrombosis risk: strongest association for factor VIII and von Willebrand factor. J Thromb Haemost. (2019) 17:99–109. doi: 10.1111/jth.14343
117. Zhao W, Zhang X, Zang L, Zhao P, Chen Y, Wang X. ILK promotes angiogenic activity of mesenchymal stem cells in multiple myeloma. Oncol Lett. (2018) 16:1101–6. doi: 10.3892/ol.2018.8711
118. Djunic I, Elezovic I, Ilic V, Tomin D, Suvajdzic-Vukovic N, Bila J, et al. Acquired von Willebrand syndrome in multiple myeloma. Hematology. (2011) 16:209–12. doi: 10.1179/102453311X12953015767617
119. RicoRios N, Bowles L, Ayling RM. Multiple myeloma and acquired von Willebrand disease: a combined cause of preanalytical interference causing gel formation? Ann Clin Biochem. (2018) 55:181–4. doi: 10.1177/0004563217710488
120. Lissandre S, Ternisien C, Rousselet M-C, Tanguy-Schmidt A, Ifrah N, Hunault-Berger M. Acquired factor IX (fIX) deficiency associated with a multiple myeloma. Blood. (2006) 108:4061. doi: 10.1182/blood.V108.11.4061.4061
121. Jia J, Wang H, Wu M, Zhang F, Liu X, Chen W, et al. Factor X deficiency caused by nonsecretory myeloma successfully corrected with bortezomib: A case report and review of the literature. Acta Haematol. (2018) 140:46–50. doi: 10.1159/000491545
122. Bangolo A, Waykole T, Niazi B, Sajja C, Akhter M, Gupta B, et al. A rare cause of acquired factor X deficiency in an 87-year-old female. Case Rep Hematol. (2021) 2021:1138329. doi: 10.1155/2021/1138329
123. Patel G, Hari P, Szabo A, Rein L, Kreuziger LB, Chhabra S, et al. Acquired factor X deficiency in light-chain (AL) amyloidosis is rare and associated with advanced disease. Hematol Oncol Stem Cell Ther. (2019) 12:10–4. doi: 10.1016/j.hemonc.2018.05.002
124. Charalampous C, Goel U, Kapoor P, Binder M, Buadi F, Dingli D, et al. Association of thrombocytopenia with disease burden, high-risk cytogenetics, and survival in newly diagnosed multiple myeloma patients treated with novel therapies. Clin Lymphoma Myeloma Leuk. (2024) 24(10):e329–35. doi: 10.1016/j.clml.2024.05.020
125. Gonsalves WI, Buadi FK, Ailawadhi S, Bergsagel PL, Chanan Khan AA, Dingli D, et al. Utilization of hematopoietic stem cell transplantation for the treatment of multiple myeloma: a Mayo Stratification of Myeloma and Risk-Adapted Therapy (mSMART) consensus statement. Bone Marrow Transplant. (2019) 54:353–67. doi: 10.1038/s41409-018-0264-8
126. Yang XX, Yao G, Yang Y, Han Y, Yang L, Zhang Y. Meta-analysis of effectiveness and safety of proteasome inhibitor-dependent maintenance treatment for multiple myeloma a systematic review. Heliyon. (2024) 10:e36311. doi: 10.1016/j.heliyon.2024.e36311
127. Wang Y, Yang F, Shen Y, Zhang W, Wang J, Chang VT, et al. Maintenance therapy with immunomodulatory drugs in multiple myeloma: A meta-analysis and systematic review. J Natl Cancer Inst. (2016) 108(3):djv342. doi: 10.1093/jnci/djv342
128. Hari P, Aljitawi OS, Arce-Lara C, Nath R, Callander N, Bhat G, et al. A phase IIb, multicenter, open-label, safety, and efficacy study of high-dose, propylene glycol-free melphalan hydrochloride for injection (EVOMELA) for myeloablative conditioning in multiple myeloma patients undergoing autologous transplantation. Biol Blood Marrow Transplant. (2015) 21:2100–5. doi: 10.1016/j.bbmt.2015.08.026
129. Schiffer CA, Bohlke K, Delaney M, Hume H, Magdalinski AJ, McCullough JJ, et al. Platelet transfusion for patients with cancer: american society of clinical oncology clinical practice guideline update. J Clin Oncol. (2018) 36:283–99. doi: 10.1200/JCO.2017.76.1734
130. Miceli T, Colson K, Gavino M, Lilleby K, Board IMFNL. Myelosuppression associated with novel therapies in patients with multiple myeloma: consensus statement of the IMF Nurse Leadership Board. Clin J Oncol Nurs. (2008) 12:13–20. doi: 10.1188/08.CJON.S1.13-19
131. Frenzel L, Decaux O, Macro M, Belhadj-Merzoug K, Manier S, Touzeau C, et al. Venous thromboembolism prophylaxis and multiple myeloma patients in real-life: Results of a large survey and clinical guidance recommendations from the IFM group. Thromb Res. (2024) 233:153–64. doi: 10.1016/j.thromres.2023.11.021
132. Palumbo A, Rajkumar SV, Dimopoulos MA, Richardson PG, San Miguel J, Barlogie B, et al. Prevention of thalidomide- and lenalidomide-associated thrombosis in myeloma. Leukemia. (2008) 22:414–23. doi: 10.1038/sj.leu.2405062
133. Cornell RF, Goldhaber SZ, Engelhardt BG, Moslehi J, Jagasia M, Harrell S, et al. Primary prevention of venous thromboembolism with apixaban for multiple myeloma patients receiving immunomodulatory agents. Br J Haematol. (2020) 190:555–61. doi: 10.1111/bjh.v190.4
134. Piedra KM, Hassoun H, Buie LW, Devlin SM, Flynn J, Hultcrantz M, et al. VTE rates and safety analysis of newly diagnosed multiple myeloma patients receiving carfilzomib-lenalidomide-dexamethasone (KRD) with or without rivaroxaban prophylaxis. Blood. (2019) 134:1835–5. doi: 10.1182/blood-2019-124403
135. Zhang Y, Shan C, Zhang X, Liu Y, Xia Y, Wang Y. Cardiovascular adverse events associated with targeted therapies for multiple myeloma: a pharmacovigilance study. Front Immunol. (2024) 15:1400101. doi: 10.3389/fimmu.2024.1400101
136. Al-Yafeai Z, Ghoweba M, Ananthaneni A, Abduljabar H, Aziz D. Cardiovascular complications of modern multiple myeloma therapy: A pharmacovigilance study. Br J Clin Pharmacol. (2023) 89:641–8. doi: 10.1111/bcp.15499
Keywords: multiple myeloma, coagulopathy, bone marrow mesenchymal stromal cells, bleeding diathesis, thrombosis
Citation: Abdi J and Nasr P (2025) Abnormalities of primary and secondary hemostasis in multiple myeloma: insights from studies on thrombopoiesis, the coagulation system, and the bone marrow microenvironment. Front. Hematol. 4:1435193. doi: 10.3389/frhem.2025.1435193
Received: 19 May 2024; Accepted: 10 February 2025;
Published: 03 March 2025.
Edited by:
Zsuzsa Bagoly, University of Debrecen, HungaryReviewed by:
Valentina Giudice, University of Salerno, ItalyCopyright © 2025 Abdi and Nasr. This is an open-access article distributed under the terms of the Creative Commons Attribution License (CC BY). The use, distribution or reproduction in other forums is permitted, provided the original author(s) and the copyright owner(s) are credited and that the original publication in this journal is cited, in accordance with accepted academic practice. No use, distribution or reproduction is permitted which does not comply with these terms.
*Correspondence: Jahan Abdi, amFiZGlAY3N1ZGguZWR1
Disclaimer: All claims expressed in this article are solely those of the authors and do not necessarily represent those of their affiliated organizations, or those of the publisher, the editors and the reviewers. Any product that may be evaluated in this article or claim that may be made by its manufacturer is not guaranteed or endorsed by the publisher.
Research integrity at Frontiers
Learn more about the work of our research integrity team to safeguard the quality of each article we publish.