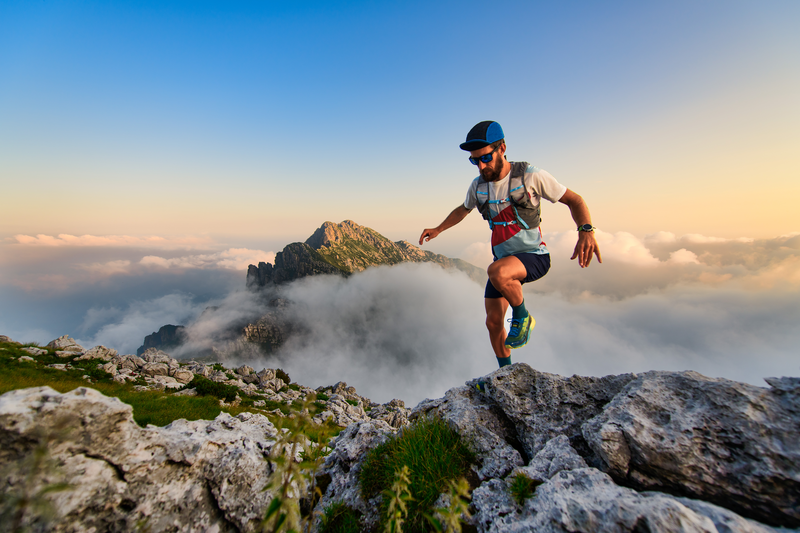
94% of researchers rate our articles as excellent or good
Learn more about the work of our research integrity team to safeguard the quality of each article we publish.
Find out more
OPINION article
Front. Hematol. , 18 March 2024
Sec. Blood Cancer
Volume 3 - 2024 | https://doi.org/10.3389/frhem.2024.1377806
This article is part of the Research Topic Editors' Showcase: Blood Cancer View all 5 articles
NETosis is a regulated cell death pathway primarily used by neutrophils to eliminate abnormal cells and pathogens, including bacteria, protozoa, fungi, and viruses, via neutrophil extracellular traps (NETs) production (1). Extracellular trap (ET) structure consists of DNA, chromatin, histones, granules, cytosolic and antimicrobial proteins produced against pathogens that can be formed by neutrophils (NETs), macrophages (METs), eosinophils (EETs), mast cells (MCETs), and basophils (BETs) involved in the antimicrobial response, thrombosis, diabetes, sepsis, immune regulation, cardiac function, cancer progression, metastasis, and chemoresistance (2). NETs are the first discovered ones released by activated neutrophils, comprising 50-70% of all white blood cells, and considered the first line of defense against pathogens, inflammation, cancer cells, and environmental exposures. Neutrophils regulate innate and adaptive immune systems, where activated neutrophils eliminate abnormal cells and pathogens via three major pathways: NETosis via NET formation, phagocytosis, and the release of cytotoxic enzymes called degranulation (3, 4).
NETosis was first described by Takoma et al. in 1996 (2) and characterized in detail by Brinkman et al. in 2004 (3). NETosis is triggered via various signals and pathways including elevated intracellular calcium levels via β2 integrin, increased reactive oxygen species (ROS) levels, membrane surface receptors such as CD18, toll-like receptor 1 (TLR1), phorbol myristate acetate (PMA), nucleotide oligomerization domain (NOD)-like receptor 2, intracellular signaling cascades including SYK-PI3K-mTorc2 AND Raf-MEK-ERK-MAP kinase, cytokines and chemokines (IL-1, IL-18, TNF), changes in the pH, presence of bacterial toxins and proteins such as lipopolysaccharides (LPS) (5). NETosis is categorized into suicidal and vital NETosis depending on whether neutrophil integrity and function are preserved. Vital NETosis is independent of NADPH oxidase (NOX), in which neutrophils remain vital and take approximately 30 minutes. It is triggered by complement proteins, pathogens, and activated platelets via activation of TLR-2, TLR-4, and complement receptor 3 (CR3) that leads to activation of peptidyl arginine deiminase-4 (PAD4) enzyme involved in the chromatin decondensation (6). After activation, PAD4 enters the nucleus, triggering citrullinated H3 (CitH3) to activate chromatin decondensation. CitH3 is a biomarker associated with NETosis, NET production, venous thromboembolism, and mortality risk. Since NETs are released via exocytosis externally, cells remain intact without membrane rupture in the vital NETosis (7).
On the other hand, suicidal NETosis is a regulated/programmed cell death pathway having distinctive properties from different cell death pathways such as apoptosis, ferroptosis, pyroptosis, or necroptosis (8). It is a NOX-regulated pathway mainly depending on ROS production and is triggered by IL6/8, pathogens, cholesterol crystals, antibodies, and TNF-α via cytokine, TLR, and Fc receptors. After activation, the endoplasmic reticulum (ER) releases stored Ca+2 into the cytoplasm, activating NOX via the protein kinase C (PKC)/Raf/MEK/ERK pathway. Moreover, calcium ions are involved in the PAD4 structure as a cofactor, and activated PAD4 enters the nucleus to trigger chromatin decondensation (5, 7, 9). On the other hand, NOX-induced ROS production starts neutrophil elastase (NE) and myeloperoxidase (MPO) via degrading cytoplasmic granules, and they migrate to the nucleus, leading to the chromatin decondensation via triggering of CitH3. Furthermore, mitochondrial ROS can also activate NOX-dependent NETosis via increased intracellular Ca2+ levels. Triggering of intracellular Ca2+ release, PAD4, and ChitH3 activation are common features of both vital and suicidal NETosis (7, 10).
The NET formation can be evaluated by the detection of NETosis markers, including PAD4, CitH3, MPO, NE, ROS, modified histones (H2A/H2B), and NOX via enzyme-linked immunosorbent assay (ELISA), fluorescence microscopy, electron microscopy, western blot, live imaging, flow cytometry, and multispectral image flow cytometer (MIFC) (11). Neutrophils and neutrophil-associated factors such as NETs and tumor tumor-associated neutrophils (TANs) are involved in the initiation, progression, metastasis, poor prognosis, and resistance to treatment in various cancers, including hematological malignancies. However, the impact and role of NETosis on hematological cancers as a therapeutic target have not been studied widely until now. In this opinion paper, we aim to highlight the possible effects of NETosis on hematological malignancies, including leukemia, lymphoma, and myeloma, as a promising therapeutic target, predictive and progressive marker (6–8, 10).
Neutrophils are the primary cell type responding to the innate immune response considered major hallmarks of acute inflammation. Neutrophils are short-lived immune cells in the blood with approximately 7 hours of half-life; however, they can survive five or more days in the tumor microenvironment (TME) (12). Neutrophils play a significant role in cancer initiation, progression, metastasis, and resistance to treatment in different types of cancers, including lung, ovarian, blood, pancreas, breast, and colon. The TME consists of various cell types, including adaptive cells (T and B cells), innate immune cells (macrophages, neutrophils, dendritic cells (DCs), natural killer (NK) cells, myeloid-derived suppressor cells (MDSCs)), stromal cells (fibroblasts, pericytes, and mesenchymal stromal cells) and extracellular matrix (ECM) cells (12, 13). After synthesis and maturation in the bone marrow, neutrophils are released into the blood and recruited to the infection or tumor sites. Neutrophils are recruited and infiltrated into the tumors, called TAN, divided into two groups, N1 and N2. NI represents an anti-tumor phenotype associated with high expression of TNF-α, intercellular adhesion molecule 1 (ICAM-1), and chemokine ligand 2 (CCL2) (12–14). On the other hand, N2 represents a tumor-promoting effect associated with elevated levels of CCL2, CCL3, CCL4, CCL8, CCL12, CCL17, chemokine ligand 1 (CXCL1), CXCL2, CXCL16 and interleukin 8 (IL-8) and induced NETosis (12).
N1 and N2 can trigger NETosis and enhanced NETosis, and factors released from tumors induce N1 to N2 phenotype transformation. The N1 phenotype is mainly found at the early stages of carcinogenesis; however, the N2 phenotype is observed at late and metastatic stages of cancer, addressing the importance of communication between the neutrophils and tumor microenvironment (15). NETosis promotes cell growth, proliferation, thrombosis, EMT, angiogenesis, metastasis, migration, invasion, tumor aggressiveness, and chemoresistance in cancer cells, including lymphoma, myeloma, and leukemia (7, 9). Neutrophil-induced ROS attacks lipids, proteins, and DNA, resulting in mutations contributing to cancer initiation, progression, and metastasis (16, 17). Also, ROS triggers the production of vascular endothelial growth factor (VEGF) and macrophage migration inhibition factor (MIF), which are involved in cancer progression and chemoresistance (18). Neutrophil-induced ROS causes inflammation and endothelial damage involved in cancer progression and metastasis. ROS molecules such as hydrogen peroxide (H2O2) act as messengers that regulate cell signaling pathways, including MAPK/Erk1/2, PI3K/Akt, and IKK/NF-KB, which are involved in cancer progression (13, 15, 18).
Neutrophils in the TME produce various types of chemokines and cytokines such as oncostatin M, hepatocyte growth factor (HGF), transforming growth factor β (TGF-β), CCL4, CXCL8, I17, BV8, CXCL8, VEGF-A, TNF-α, CCL2, CCL3, VEGF, IL-18, granulocyte-colony stimulating factor (G-CSF), IL-6, IL-10, IL-1β, CCL17, CCL9 and prokineticin 2 contributing to the cancer initiation, progression, immunosuppression angiogenesis, invasion, and metastasis due to their pro-tumor properties (19). On the other hand, neutrophil granules consist of different proteases, including NE, matrix metalloprotease 9 (MMP-9), and cathepsin G (CG) regulating EMT, ECM remodeling, chemoresistance, and NETosis that contribute to the cancer progression and metastasis. Activated neutrophils and TANs in the tumor microenvironment produce NETs consisting of histone proteins, myeloperoxidase, NE, MMP-9, CG, and chromatin (18, 19). NETs induce cancer cell proliferation directly via proteases found in NET structure or indirectly by activating cell signaling pathways such as NF-KB. Also, NETs can enable dormant cancer cells, such as breast and hematological cancers, to be awakened and activated. On the other hand, cancer cells can be captured by neutrophils, and NETs allow the tumor to metastasize to different tissues. Since elevated serum levels of NETs have been found in almost all cancers, NETs are considered potential therapeutic targets and prognostic markers. In conclusion, NETosis plays a vital role in cancer initiation, progression, metastasis, chemoresistance, and prognosis, making it a possible therapeutic approach to treat various cancers, including hematological malignancies (14, 19, 20).
Hematological malignancies, including lymphoma, myeloma, and leukemia, are a critical cause of death worldwide, and their incidence is increasing; therefore, new therapy approaches have been investigated worldwide (4). Neutrophils and neutrophils-associated conditions such as NETs have been reported in a few studies; however, the impact of NETosis has not been widely studied in hematological malignancies regarding molecular and therapeutical aspects, unlike solid tumors (17). NETs contribute to tumor growth, metastasis, thrombosis, poor prognosis, invasion, migration, evasion, and chemoresistance in hematological malignancies, and NET formation significantly increased in lymphoma, leukemia, and myeloma cancers (21). Also, NETs trigger dormant hematological malignancies to be awakened leading to cancer initiation. On the other hand, thrombosis is the second most common cause of death in tumor-affected individuals, and increased NET induces thrombosis in patients having hematological malignancies. Moreover, neutrophils of individuals with hematological malignancies have an increased capacity to produce NETs compared to healthy subjects (22).
Multiple myeloma is a plasma cell cancer mainly located in the bone marrow, and it is still untreatable with a 50% 5-year survival rate. Interaction between bone marrow TME and multiple myeloma cells determines resistance and progression of the disease. Myeloma cells trigger NETosis, whereas inhibition of PAD4 exhibited anti-tumor effects on the multiple myeloma mouse model. On the other hand, the inhibition of NET prolonged the survival time of the mice with multiple myeloma (5, 22). Zhao et al. reported a NET-related risk score to predict prognosis and to characterize the TME in multiple myeloma. Neutrophils, lymphocytes, and macrophages exert NET activity in multiple myeloma patients and several genes, including E3 ubiquitin-protein ligase 125 (RNF125), nucleophosmin 1 (NPM1), cysteine-rich intestinal protein 1 (CRIP1), histone H1.2 (HIST1H1C), S100 calcium-binding protein A6 (S100A6), glyceraldehyde 3-phosphate dehydrogenase (GAPDH), and meiotic double-stranded break formation protein 1 (MEI1), MGAT4A have been associated with high risk for progression, metastasis and chemoresistance in MM patients (23). NETosis causes chemoresistance against doxorubicin and melphalan in multiple myeloma; however, no detailed study addresses the exact mechanism and components of NETosis regarding multiple myeloma pathogenesis in the literature (8). Lymphoma is the most common hematological malignancy affecting the lymphatic system. It is divided into two main groups: Hodgkin Lymphoma represents 10% of all cases, whereas non-Hodgkin Lymphoma accounts for 90% of all lymphoma cases. According to a study, the interaction of NETs with CD5+ B cells triggers the transition of autoimmunity to lymphoma (24). Diffuse large B-cell lymphoma (DLBCL) accounts for 30% of non-Hodgkin Lymphomas, and increased NET levels are associated with progression and poor survival of DLBCL patients. On the other hand, DLBCL-induced IL-8-induced tumor progression via activation of TLR9 and inhibition of IL-8 or TLR9 suppress tumor progression (25). Moreover, TANs promote DLBCL growth and survival via sustained BAFF/APRIL pathway activation and NF-κB-dependent manner, respectively (26).
Leukemia generally begins in the bone marrow, producing abnormal white blood cells that are not fully developed. Four main types of leukemia have been reported: acute lymphoblastic leukemia (ALL), acute myeloid leukemia (AML), chronic lymphocytic leukemia (CLL), and chronic myeloid leukemia (CML). Acute leukemias are characterized by the aberrant proliferation of the malignant progenitor cells and represent altered neutrophil function and reduced NET formation associated with thrombosis and secondary immunodeficiencies (27). On the other hand, CLL results from the accumulation of clonal CD5+ B cells in lymphoid tissues, blood, and bone marrow. Neutrophils derived from CLL patients have increased capacity for NETosis and significantly increased levels of IL-8, TNFα, IL-1β, and HMGB1 (28). CML results from the BCR-ABL1 fusion gene and neutrophils of CML patients showed increased capacity NET production along with PAD4, ROS, and CitH3 associated with disease severity, thrombosis, and chemoresistance (29). On the other hand, increased DNA methylation triggers NETosis in human promyelocytic leukemia (APL) cells in vitro (30).
The role of NETosis and the effects of its inhibitors have not been widely studied in hematological malignancies, unlike solid tumors. A few studies as mentioned above have reported that NET has been implicated with cancer initiation, progression, and chemoresistance in hematological cancers, including myeloma, leukemia, and lymphoma; however, the exact mechanism of NETosis and its inhibition as a possible target have not been fully elucidated (8). Several molecules inhibiting NETosis, including hydroxychloroquine, methotrexate, prednisolone, rituximab, belimumab, tocilizumab, CI-amidine, rapamycin, lapatinib, and bosutinib have been reported in the literature. Indicated inhibitors have not been tested on NETosis widely by using in vitro, in vivo, and humans associated with molecular aspects of inhibitory pathways (9). Furthermore, novel molecules and nanoparticles can be developed to treat hematological malignancies via targeting NETosis. On the other hand, reduced NETosis has been associated with an increased risk of immunosuppression, reduced immune response, and mortality in patients with hematological malignancies. Thus, drug formulations, enzymes, or nanotechnological tools targeting NETosis should be developed to minimize side effects such as immunosuppression or infections by evaluating heterogeneous patterns of hematological malignancies.
DA: Conceptualization, Data curation, Formal analysis, Funding acquisition, Investigation, Methodology, Project administration, Resources, Software, Supervision, Validation, Visualization, Writing – original draft, Writing – review & editing.
The author(s) declare that no financial support was received for the research, authorship, and/or publication of this article.
The author declares that the research was conducted without any commercial or financial relationships that could be construed as a potential conflict of interest.
All claims expressed in this article are solely those of the authors and do not necessarily represent those of their affiliated organizations, or those of the publisher, the editors and the reviewers. Any product that may be evaluated in this article, or claim that may be made by its manufacturer, is not guaranteed or endorsed by the publisher.
1. Xiong S, Dong L, Cheng L. Neutrophils in cancer carcinogenesis and metastasis. J Hematol Oncol. (2021) 14:173. doi: 10.1186/s13045-021-01187-y
2. Takei H, Araki A, Watanabe H, Ichinose A, Sendo F. Rapid killing of human neutrophils by the potent activator phorbol 12-myristate 13-acetate (PMA) accompanied by changes different from typical apoptosis or necrosis. J Leukoc Biol. (1996) 59:229–40. doi: 10.1002/jlb.59.2.229
3. Brinkmann V, Reichard U, Goosmann C, Fauler B, Uhlemann Y, Weiss DS, et al. Neutrophil extracellular traps kill bacteria. Sci (1979). (2004) 303:1532–5. doi: 10.1126/science.1092385
4. Zhang N, Wu J, Wang Q, Liang Y, Li X, Chen G, et al. Global burden of hematologic Malignancies and evolution patterns over the past 30 years. Blood Cancer J. (2023) 13:82. doi: 10.1038/s41408-023-00853-3
5. Masucci MT, Minopoli M, Carriero MV. Tumor associated neutrophils. Their role in tumorigenesis, metastasis, prognosis and therapy. Front Oncol. (2019) 9:1146. doi: 10.3389/fonc.2019.01146
6. Huang J, Hong W, Wan M, Zheng L. Molecular mechanisms and therapeutic target of NETosis in diseases. MedComm (Beijing). (2022) 3(3):e162. doi: 10.1002/mco2.162
7. Vorobjeva NV, Chernyak BV. NETosis: molecular mechanisms, role in physiology and pathology. Biochem (Moscow). (2020) 85:1178–90. doi: 10.1134/S0006297920100065
8. Jaboury S, Wang K, O’Sullivan KM, Ooi JD, Ho GY. NETosis as an oncologic therapeutic target: a mini review. Front Immunol. (2023) 14:1170603. doi: 10.3389/fimmu.2023.1170603
9. Chamardani TM, Amiritavassoli S. Inhibition of NETosis for treatment purposes: friend or foe? Mol Cell Biochem. (2022) 477:673–88. doi: 10.1007/s11010-021-04315-x
10. Ronchetti L, Boubaker NS, Barba M, Vici P, Gurtner A, Piaggio G. Neutrophil extracellular traps in cancer: not only catching microbes. J Exp Clin Cancer Res. (2021) 40:231. doi: 10.1186/s13046-021-02036-z
11. Stoimenou M, Tzoros G, Skendros P, Chrysanthopoulou A. Methods for the assessment of NET formation: from neutrophil biology to translational research. Int J Mol Sci. (2022) 23:15823. doi: 10.3390/ijms232415823
12. Que H, Fu Q, Lan T, Tian X, Wei X. Tumor-associated neutrophils and neutrophil-targeted cancer therapies. Biochim Biophys Acta (BBA) Rev Cancer. (2022) 1877:188762. doi: 10.1016/j.bbcan.2022.188762
13. Yan M, Gu Y, Sun H, Ge Q. Neutrophil extracellular traps in tumor progression and immunotherapy. Front Immunol. (2023) 14:1135086. doi: 10.3389/fimmu.2023.1135086
14. Olsson A-K, Cedervall J. NETosis in cancer - platelet-neutrophil crosstalk promotes tumor-associated pathology. Front Immunol. (2016) 7:373. doi: 10.3389/fimmu.2016.00373
15. Kwak S-B, Kim SJ, Kim J, Kang Y-L, Ko CW, Kim I, et al. Tumor regionalization after surgery: Roles of the tumor microenvironment and neutrophil extracellular traps. Exp Mol Med. (2022) 54:720–9. doi: 10.1038/s12276-022-00784-2
16. Aydemir D, Sarayloo E, Ulusu NN. Rosiglitazone-induced changes in the oxidative stress metabolism and fatty acid composition in relation with trace element status in the primary adipocytes. J Med Biochem. (2019) 39(3):267–75. doi: 10.2478/jomb-2019-0041
17. Demkow U. Neutrophil extracellular traps (NETs) in cancer invasion, evasion and metastasis. Cancers (Basel). (2021) 13:4495. doi: 10.3390/cancers13174495
18. De Meo ML, Spicer JD. The role of neutrophil extracellular traps in cancer progression and metastasis. Semin Immunol. (2021) 57:101595. doi: 10.1016/j.smim.2022.101595
19. Zuo H, Yang M, Ji Q, Fu S, Pu X, Zhang X, et al. Targeting neutrophil extracellular traps: A novel antitumor strategy. J Immunol Res. (2023) 2023:1–11. doi: 10.1155/2023/5599660
20. Hu Y, Wang H, Liu Y. NETosis: Sculpting tumor metastasis and immunotherapy. Immunol Rev. (2024) 321:263–79. doi: 10.1111/imr.13277
21. Kim TY, Gu J-Y, Jung HS, Koh Y, Kim I, Kim HK. Elevated extracellular trap formation and contact system activation in acute leukemia. J Thromb Thromb. (2018) 46:379–85. doi: 10.1007/s11239-018-1713-3
22. Allegra A, Tonacci A, Musolino C, Pioggia G, Gangemi S. Secondary immunodeficiency in hematological Malignancies: focus on multiple myeloma and chronic lymphocytic leukemia. Front Immunol. (2021) 12:738915. doi: 10.3389/fimmu.2021.738915
23. Zhao Z, Huo Y, Du Y, Huang Y, Liu H, Zhang C, et al. A neutrophil extracellular trap-related risk score predicts prognosis and characterizes the tumor microenvironment in multiple myeloma. Sci Rep. (2024) 14:2264. doi: 10.1038/s41598-024-52922-7
24. Sangaletti S, Tripodo C, Vitali C, Portararo P, Guarnotta C, Casalini P, et al. Defective stromal remodeling and neutrophil extracellular traps in lymphoid tissues favor the transition from autoimmunity to lymphoma. Cancer Discov. (2014) 4:110–29. doi: 10.1158/2159-8290.CD-13-0276
25. Nie M, Yang L, Bi X, Wang Y, Sun P, Yang H, et al. Neutrophil extracellular traps induced by IL8 promote diffuse large B-cell lymphoma progression via the TLR9 signaling. Clin Cancer Res. (2019) 25:1867–79. doi: 10.1158/1078-0432.CCR-18-1226
26. Grégoire M, Guilloton F, Pangault C, Mourcin F, Sok P, Latour M, et al. Neutrophils trigger a NF-κB dependent polarization of tumor-supportive stromal cells in germinal center B-cell lymphomas. Oncotarget. (2015) 6:16471–87. doi: 10.18632/oncotarget.4106
27. Ostafin M, Ciepiela O, Pruchniak M, Wachowska M, Ulińska E, Mrówka P, et al. Dynamic changes in the ability to release neutrophil ExtraCellular traps in the course of childhood acute leukemias. Int J Mol Sci. (2021) 22:821. doi: 10.3390/ijms22020821
28. Podaza E, Sabbione F, Risnik D, Borge M, Almejún MB, Colado A, et al. Neutrophils from chronic lymphocytic leukemia patients exhibit an increased capacity to release extracellular traps (NETs). Cancer Immunol Immunother. (2017) 66:77–89. doi: 10.1007/s00262-016-1921-7
29. Telerman A, Granot G, Leibovitch C, Yarchovsky-Dolberg O, Shacham-Abulafia A, Partouche S, et al. Neutrophil extracellular traps are increased in chronic myeloid leukemia and are differentially affected by tyrosine kinase inhibitors. Cancers (Basel). (2021) 14:119. doi: 10.3390/cancers14010119
Keywords: NEtosis, NET, multiple myeloma, lymphoma, leukemia, blood cancer
Citation: Aydemir D (2024) The impact of NETosis on hematological malignancies as a promising therapeutic target. Front. Hematol. 3:1377806. doi: 10.3389/frhem.2024.1377806
Received: 28 January 2024; Accepted: 04 March 2024;
Published: 18 March 2024.
Edited by:
Prashant Ramesh Tembhare, Research and Education in Cancer (ACTREC), IndiaReviewed by:
Francesco La Rocca, Madonna delle Grazie Hospital, ItalyCopyright © 2024 Aydemir. This is an open-access article distributed under the terms of the Creative Commons Attribution License (CC BY). The use, distribution or reproduction in other forums is permitted, provided the original author(s) and the copyright owner(s) are credited and that the original publication in this journal is cited, in accordance with accepted academic practice. No use, distribution or reproduction is permitted which does not comply with these terms.
*Correspondence: Duygu Aydemir, ZGF5ZGVtaXIxNkBrdS5lZHUudHI=
Disclaimer: All claims expressed in this article are solely those of the authors and do not necessarily represent those of their affiliated organizations, or those of the publisher, the editors and the reviewers. Any product that may be evaluated in this article or claim that may be made by its manufacturer is not guaranteed or endorsed by the publisher.
Research integrity at Frontiers
Learn more about the work of our research integrity team to safeguard the quality of each article we publish.