- 1Department of Medicine, New York, Montefiore Medical Center/Albert Einstein College of Medicine, New York, NY, United States
- 2Department of Oncology, Montefiore Medical Center/Albert Einstein College of Medicine, New York, NY, United States
Apoptosis is a tightly regulated process of cell death occurring through extrinsic and intrinsic pathways. The Bcl-2 family of proteins is implicated in the intrinsic pathway and encompasses both pro-apoptotic and anti-apoptotic proteins. Anti-apoptotic Bcl-2 proteins are frequently overexpressed in hematologic malignancies and so Bcl-2 inhibitors have been developed to combat these malignancies. The first and so-far only FDA-approved Bcl-2 inhibitor has been venetoclax, initially for treatment of chronic lymphocytic leukemia (CLL) with 17-p deletion as a second-line agent, followed by later expansion to all CLL and selected acute myeloid leukemia (AML) indications. Venetoclax and inhibitors of other Bcl-2 family members have demonstrated significant potential. However, their use requires careful consideration of disease indication, along with biomarkers associated with disease and optimal drug combinations. Side-effect profiles and specific patterns of resistance must be considered as well. In this review, we examine in detail the characteristics of the Bcl-2 family of proteins and their role in apoptosis. We discuss the drug development process that led to the first-in-class approval of venetoclax, along with relevant use considerations. Finally, we examine future directions in this domain of pharmaceutical development.
1 Introduction: apoptosis and Bcl-2
Apoptosis is a regulated process of cell death that plays important roles in homeostasis, human development, and other vital processes, such as immune responses (1, 2). Apoptosis is characterized by two regulatory pathways, extrinsic and intrinsic (Figure 1). These pathways are interconnected with a common element of caspase activation, which leads to the breakdown of cellular components, followed by cell death.
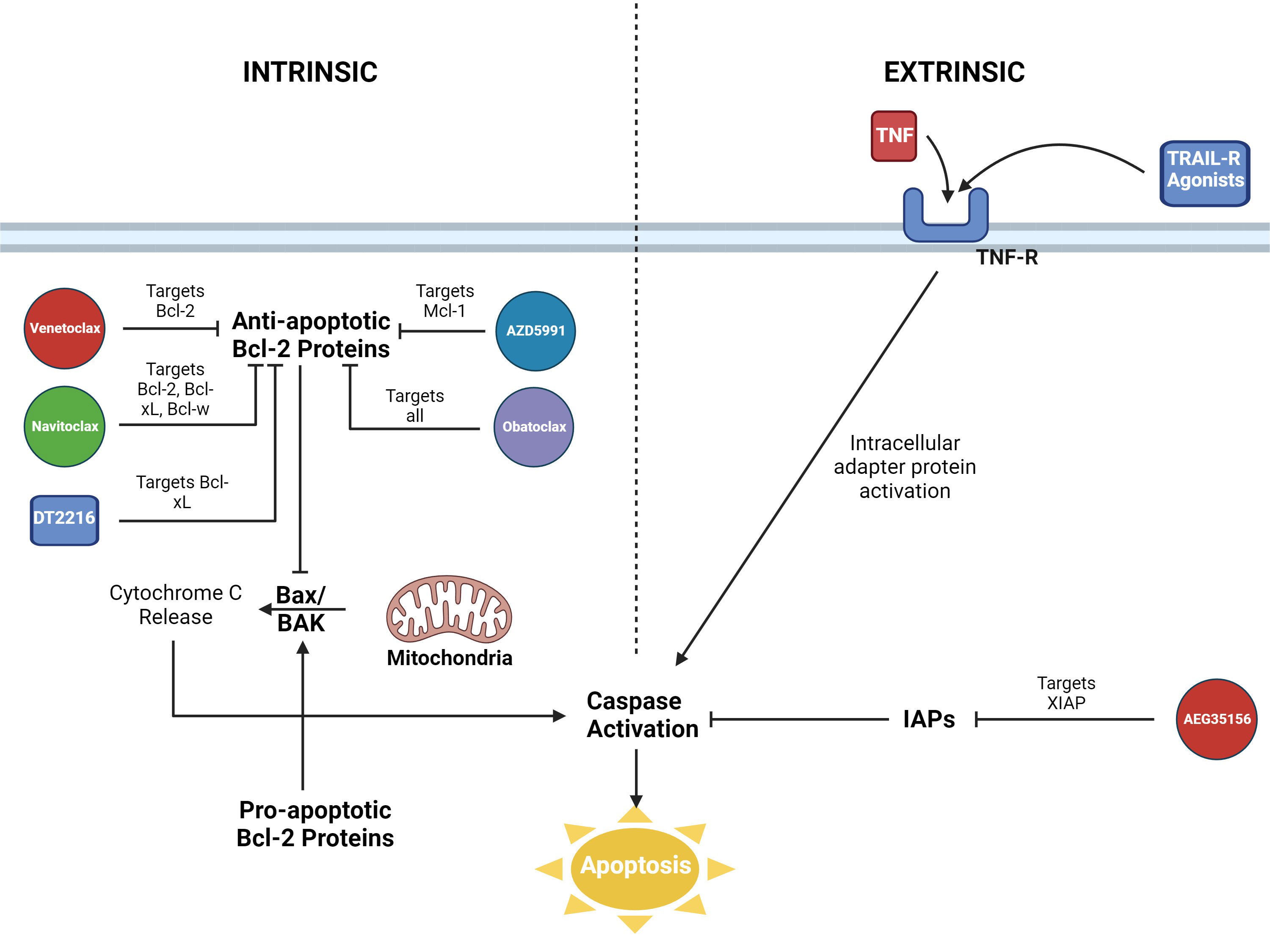
Figure 1 Visualization of the intrinsic and extrinsic pathways of apoptosis. Roles of Bcl-2 inhibitors and other pharmaceutical agents displayed. AZD5991 = Mcl-1 inhibitor. DT2216 = PROTAC Bcl-xL inhibitor. AEG35156 = XIAP inhibitor. Figure created in BioRender.
The extrinsic pathway is initiated when receptors on the cell surface, death receptors, are bound by ligands. Some examples of death receptors include TNFR, FAS (CD95), and DR3/WSL (3). Binding of ligands leads to activation of adapter proteins intracellularly and the formation of signaling complexes that trigger caspases.
The intrinsic or mitochondrial pathway is activated by cellular stress. This is the pathway that the Bcl-2 family of proteins regulates through interactions with mitochondrial outer membrane permeabilization (MOMP) (4). BAX and BAK, two executioner proteins of the Bcl-2 family, interact directly with the outer membrane of mitochondria and induce MOMP, which results in release of proteins such as cytochrome c from the intermembrane space of mitochondria into the cytosol (5, 6). The release of cytochrome c then leads to the formation of an apoptosome protein complex which triggers caspase activation. Defects in the intrinsic regulatory elements of apoptosis are thought to be involved in the development and progression of malignancies. The first pro-survival protein of the Bcl-2 family, Bcl-2, was identified in the early 1990s and it was noted that overexpression of Bcl-2 contributed to lymphomagenesis in follicular lymphoma, especially in cases with chromosome 14 and 18 translocations. Bcl-2 inhibited BAX and BAK through direct protein-protein interactions (7).
Members of the Bcl-2 protein family are grouped together due to sharing one or more of BH domains (BH1-4), despite some members being pro-apoptotic and others anti-apoptotic. Pro-apoptotic members, proteins sharing BH3 domains, include BIM, BID, NOXA, PUMA, BAD, HRK, BMF, and BIK (8). Anti-apoptotic members include Bcl-2, Bcl-xL, Bcl-w, Mcl-1, and A1/BFL-1 (9). Pro-apoptotic and anti-apoptotic members of the Bcl-2 protein family interact through hydrophobic interactions of BH domains, with antagonism of the anti-apoptotic proteins by the pro-apoptotic proteins. The net effect is promotion or demotion of apoptosis. There are several models for the interactions among pro-apoptotic and anti-apoptotic Bcl-2 proteins, which are beyond the scope of this review (6).
2 The role and development of Bcl-2 inhibitors
Evasion of apoptosis promotes tumor initiation followed by progression (10). There has been increasing evidence that all malignancies possess some degree of apoptotic resistance, but mechanisms of this resistance may differ (11). Overexpression of anti-apoptotic proteins, including Bcl-2, has been noted in cancers, such as follicular lymphoma and has been tied to increased radioresistance and chemotherapy resistance (12). Downregulation or inhibition of pro-apoptotic members of the Bcl-2 family has been noted in many tumor types as well. For instance, in Burkitt lymphomas, pro-apoptotic BIM and PUMA are silenced through promoter hypermethylation (13). The premise of Bcl-2 inhibitors evolved out of a desire to inhibit anti-apoptotic members of the Bcl-2 family.
One of the first identified Bcl-2 inhibitors was gossypol, a natural phenol derived from cotton plants. In addition to inhibiting pro-survival Bcl-2 proteins, it can activate pro-apoptotic members of the Bcl-2 family, such as NOXA and PUMA (14). Gossypol has been implicated in other signaling pathways as well, including NF-kB regulation and MDM2-VEGF interactions (15). Though very broad-acting, gossypol has a significant adverse effects profile, including hypokalemia and infertility, and so utilization has been limited (16).
More specific agents for Bcl-2 inhibition, BH3 mimetics, have been developed with better understanding of Bcl-2 protein family structure and BH domains. The first of these agents, ABT-737, was developed by Abbott Laboratories based on analysis of the BAD protein BH3 region via NMR (17). ABT-737 targeted several members of the pro-survival Bcl-2 family (Bcl-2, Bcl-xl, and Bcl-w proteins) with high affinity. It demonstrated promise in animal models causing tumor regression, but is not orally bioavailable, leading to the development of navitoclax (18).
Navitoclax has been evaluated in phase I and II clinical trials and has clinical activity in small cell lung cancer and acute lymphoblastic leukemia but causes significant dose-dependent thrombocytopenia due to inhibition of Bcl-xL (19). Obatoclax has also been developed which targets all members of the pro-survival Bcl-2 family, but experiences issues with solubility and toxicity (20, 21).
ABT-199 or venetoclax was subsequently developed as a more selective Bcl-2-specific agent. Despite venetoclax lacking the pleiotropic inhibition capabilities of other members of the anti-apoptotic Bcl-2 group, venetoclax has demonstrated promise as the first-in-class Bcl-2 inhibitor approved by the FDA, first as a breakthrough therapy for relapsed CLL/SLL in 2015 (22). Since then, its approved uses have expanded to all CLL/SLL patients and to AML patients ≥ 75 years old (or with significant comorbidities) as part of a combination regimen with azacitidine, decitabine, or low-dose cytarabine (23). Venetoclax has minimal toxicity to platelets compared to navitoclax or obatoclax.
3 Venetoclax in CLL
Chemoimmunotherapy with regimens including fludarabine, cyclophosphamide, rituximab, chlorambucil and/or obinutuzumab have traditionally been mainstays in the treatment of CLL (24). In recent years, PI3K inhibitors and BTK inhibitors have been introduced as targeted agents centered on inhibition of oncogenic signaling pathways. The development of venetoclax has continued this trend. CLL is notable for high levels of Bcl-2 expression. Notably in the most common 13q deletion CLL, this has been attributed to a loss of tumor suppressor microRNA (miR-15 and miR-16) expression. miR-15 and miR-16 directly target and inhibit Bcl-2 expression (25).
The first clinical trial for venetoclax was a phase I dose-escalation trial with 116 patients diagnosed with refractory CLL or small lymphocytic leukemia SLL (13). In this cohort, the overall response rate (ORR) was 79% with 20% achieving complete remission (CR), and 5% with no minimal residual disease (MRD). Response rates were 71-79% even among subgroups with poor prognostic features, such as resistance to fludarabine, 17p deletions, or unmutated IGHV. A manageable safety profile was noted as well. Though three of 56 patients experienced tumor lysis syndrome (TLS) in the dose-escalation cohort, none were noted in the expansion cohort after adjustments were made to the dosing schedule with a ramp-up from 20 mg to 200 mg. A subsequent phase II, single arm, multicenter study (M13-982) in 107 patients with 17p deletion demonstrated an ORR 79.4% and CR 8% (26). The most common adverse events were grade 3-4 neutropenia (40%), infection (20%), anemia (18%), and thrombocytopenia (15%).
Though venetoclax monotherapy induced significant response in patients with relapsed CLL, it was notable in these trials that CR occurred only for 8-20% of the cohort with median progression free survival (PFS) at 25 months, which suggested limited efficacy of venetoclax monotherapy. This observation has subsequently led to the development and study of various combination regimens. Current NCCN guidelines recommend venetoclax-obinutuzumab as a preferred first line regimen for CLL with and without del(17p)/TP53 mutation. Additionally, ibrutinib-venetoclax is also recommended first-line, followed by venetoclax-rituximab as preferred second/third line regimens. Several phase III trials summarized below have supported these recommendations (Table 1).
CLL14, a multicenter, open-label, randomized phase III trial examined venetoclax-obinutuzumab vs chlorambucil-obinutuzumab in patients with untreated CLL (27). The venetoclax-obinutuzumab arm had significantly longer PFS than the chlorambucil-obinutuzumab arm (HR 0.31, 0.22-0.44, p <0.0001) and did not reach median PFS as opposed to 35.6 months PFS in the chlorambucil-obinutuzumab arm. Grade 3-4 neutropenia was common in both arms.
Another multicenter open-label, randomized phase III trial, GLOW, examined ibrutinib-venetoclax vs chlorambucil-obinutuzumab in patients ≥65 or those younger with cumulative illness rating scale (CIRS) > 6 (28). PFS was longer for the ibrutinib-venetoclax arm (median PFS not reached) than the chlorambucil-obinutuzumab arm (median PFS 21.0 months, 95% CI 16.6-24.7, HR 0.216, 0.131-0.357, p < 0.001). Overall improvement was consistent between patients ≥65 or those younger with CIRS > 6. Additionally, the proportion of patients with undetectable minimal residual disease (uMRD) from 3 to 12 months after treatment was greater in the ibrutinib-venetoclax arm. Side-effect profiles were consistent with expectations.
Finally, the MURANO trial examined venetoclax-rituximab vs bendamustine-rituximab in patients with relapsed/refractory CLL (29). Two-year rates of PFS were significantly higher in the venetoclax-rituximab arm (84.9% vs 36.3%, HR 0.17, 0.11-0.125, p < 0.001). This was seen across clinical and biologic subgroups. Though rates of grade 3-4 neutropenia were higher in venetoclax-rituximab (57.7% vs 38.8%), grades 3-4 febrile neutropenia (3.6% vs 9.6%), infection, and infestation (17.5% vs 21.8%) were lower.
4 Venetoclax in AML
The current first-line treatment for newly diagnosed AML is induction with cytarabine and anthracycline in what is known as a “7 + 3” regimen, followed by consolidation with 2-4 cycles of intermediate dose cytarabine or hematopoietic stem cell transplant (32). With this therapy, 60-80% of patients ≤60 years old achieve CR and only 40-60% of patients >60 years old achieve CR (33). The presence of small populations of leukemic stems cells (LSC) resistant to therapy is thought to contribute to relapse (34). This is a particularly significant issue in elderly patients and patients with poor functional status that cannot tolerate standard induction. LSCs are thought to be cell cycle quiescent compared to hematopoietic stem cells and overexpress Bcl-2, a rationale for evaluation of Bcl-2 inhibitors, such as venetoclax, as potential AML therapeutics.
The earliest studies to evaluate venetoclax monotherapy in AML was a phase II single arm trial in relapsed/refractory AML in 2016 which achieved ORR 19% (35). Venetoclax demonstrated pharmacologic effect by International Working Group Criteria in these patients with an acceptable safety profile. This study has been a foundation for later trials examining venetoclax combination regimens of venetoclax with hypomethylating agents (HMAs) or low-dose cytarabine (LDAC) for newly diagnosed AML in adults ≥75 years, or adults with comorbidities that prevent standard induction (36).
In initial phase Ib study of venetoclax-decitabine or azacitidine in patients ≥ 65 years old with untreated AML the regimen demonstrated an overall CR/CRi rate of 61% in three arms: venetoclax-decitabine, venetoclax-azacitidine, and a substudy group of venetoclax-decitabine with posaconazole (37). A follow-up phase II study narrowed down the dosing of venetoclax to 400 mg daily (38). Notably in this study, a subgroup analysis of patients with intermediate and poor risk AMLs demonstrated response with CR/CRi of 74% and 60% respectively and patients with TP53, IDH1/2, and FLT3 mutations had response with CR/CRi 47%, 71%, and 72%. A subsequent phase III study demonstrated that venetoclax-azacitidine was superior to azacitidine alone in patients ineligible for standard induction (median OS 14.7 months vs 9.6 months, HR 0.66, 95% CI 0.52–0.85, p = 0.001) (30). CR/CRi was also higher comparing venetoclax-azacitidine at 66.4% vs 28.3% (p = 0.001) in azacitidine alone. It was notable that the incidence of febrile neutropenia was higher in the venetoclax-azacitidine group (42% vs 19%).
In parallel, low dose cytarabine (LDAC) has been evaluated in combination with venetoclax in a phase Ib/II trial with adults 60 years or older or ineligible for intensive therapy with previously untreated AML (39). Forty-nine percent of these patients had secondary AML, 29% with prior HMA treatment, and 32% with poor cytogenic features. Fifty-four percent achieved CR/CRi with median OS 10.1 months and median duration of response (DOR) of 8.1 months. In the group of patients without prior HMA exposure, CR/CRi was documented in 62% and median OS was 13.5 months and DOR 14.8 months. This study showed venetoclax and LDAC combination produced a durable remission. A follow-up randomized placebo-controlled phase III trial in newly diagnosed patients ineligible for intensive induction did not meet primary endpoint, but analysis after an additional six-month follow-up showed improved survival for the venetoclax-LDAC arm (HR 0.70, 0.50-0.99, p = 0.04) (31). Key adverse events in the venetoclax-LDAC arm included febrile neutropenia (32%), neutropenia (46%), and thrombocytopenia (45%). These randomized trials ultimately led to FDA approval of venetoclax in combinations with hypomethylating agents or LDAC in older AML patients unfit for induction chemotherapy in 2018.
It is important to note that despite this progress for older AML patients, TP53 mutation status continues to be a challenge in treatment. Studies in the in vivo mouse models and structural biology findings have supported the conclusion that Bcl-2-induced apoptosis is regulated by p53 (40, 41). It has been shown that loss of or mutant TP53 increases the threshold of BAX/BAK activation resulting in resistance to venetoclax (42, 43). These findings have been consistent with clinical trial results. In a subset analysis of the patients with poor-risk cytogenetics and TP53 mutations in the phase III trial by DiNardo et al., it was noted that remission rates were improved for patients with poor-risk cytogenetics and TP53 mutations, but duration of remission and OS were not similar between venetoclax-azacitidine vs azacitidine cohorts (30, 44). The limitations of venetoclax in patients with the TP53 mutation has been demonstrated in studies with different HMAs as well; for instance, outcomes were noted to be significantly worse in patients with TP53 mutant status compared to TP53 wild-type (ORR 66% vs 89%, p = 0.002) on decitabine-venetoclax in a phase II study of 118 patients with new AML (45). These results collectively show that TP53 mutation status warrants consideration of alternative agents.
Given the overall impact of venetoclax in older AML patients and pre-clinical studies indicating synergy with chemotherapy, combinations of venetoclax as part of standard induction are currently being explored too. A phase Ib/II trial examining venetoclax combined with FLAG-Ida induction in 68 patients with newly diagnosed or refractory AML demonstrated deep remissions and high rates of transition to autologous stem cell transplant (ASCT) (56%) (46). A follow-up report of 45 patients with extended follow-up demonstrated high response rates with MRD-negative CR rates >90%, along with estimated 24-month OS 76% (95% CI, 62%–94%). Other studies have demonstrated support for venetoclax with cladribine, idarubicin, and cytarabine (CLIA) and venetoclax with cytarabine and idarubicin (47, 48). Venetoclax combined with 7 + 3 induction chemotherapy is also being explored in a large, randomized study of the HOVON-AALG intergroup (NCT04628026).
5 Venetoclax in other hematologic malignancies
In myeloma, the t(11;14) translocation found in 15-20% of myeloma patients has been associated with Bcl-2 overexpression and higher Bcl-2 to Mcl-1 ratio (49). Sensitivity to venetoclax has been shown in pre-clinical data with certain myeloma cell lines as well and further attributed to B cell patterns of gene expression (50). Additionally, it has been shown that other myeloma therapies modulate Bcl-2 expression. Dexamethasone promotes a Bcl-2 dependent state, and bortezomib and carfilzomib are thought to reduce Mcl-1-mediated venetoclax resistance (44, 51).
A phase III study, BELLINI, examined bortezomib and dexamethasone with venetoclax vs with placebo in 291 patients with relapsed or refractory MM (52). Seventy-nine percent of these patients had high Bcl-2 expression. Median PFS was higher at 22.4 months vs 11.5 months (HR 0.63, 0.44-0.90, p=0.01). However, increased mortality was seen in the venetoclax group with eight cases (4%) treatment-emergent fatal infections noted and none in the placebo group.
A phase I/II, multi-center, dose escalation trial (NCT03314181) examining venetoclax-daratumumab-dexamethasone in patients with t(11,14) relapsed/refractory MM has recently finished enrollment for part three as of May 31, 2022 with preliminary data demonstrating durable responses: 24-month PFS was 94% (95% CI, 63.2-99.1%) and 83% (95% CI, 27.3-97.5%) in the Ven400Dd and Ven800Dd arms (53).
Venetoclax has been assessed in various subtypes of NHL, but activity was only modest. A phase I study of venetoclax in patients with relapsed/refractory NHL (mantle cell lymphoma (MCL), follicular lymphoma (FL), diffuse large B-cell lymphoma (DLBCL), Waldenström macroglobulinemia (WM), marginal zone lymphoma) showed that venetoclax was well-tolerated. Anemia, neutropenia, and thrombocytopenia were the most recorded adverse events. The response rates for MCL, FL, and DLBCL respectively were 75%, 38%, and 18%. The median PFS was estimated at 14, 11, and 1 month respectively. Of the subtypes of NHL studied, the response in MCL was notable, but durable response on monotherapy was poor with CR 21%. Subsequently, a phase II study of ibrutinib and venetoclax in 24 patients with primarily relapsed/refractory MCL (23/24) demonstrated an impressive 71% CR overall (54).
6 Future directions and discussion
The focus of this review has been on Bcl-2 inhibitors and the first-in-class approved agent venetoclax, but the family of Bcl-2 proteins is broad with other members demonstrating potential as therapeutic targets, such as Bcl-xL and Mcl-1.
It has been shown that cutaneous t-cell lymphomas (CTCL) express high levels of Bcl-xL (55). Furthermore, several AML subsets such as erythroleukemia and megakaryoblastic leukemia, overexpress and are functionally dependent on Bcl-xL for survival (56). The proteolysis-targeting chimera (PROTAC) DT2216 was developed to target Bcl-xL with the design taking advantage of engagement with VHL E3 ligase that is not expressed in platelets. This directs the PROTAC to malignant cells, addressing thrombocytopenia concerns noted with previous inhibitors of Bcl-xL, such as navitoclax (57). DT2216 has been shown in preclinical mouse models to selectively kill various Bcl-xL-dependent lymphoma and leukemia cells, supporting further clinical testing.
Mcl-1 has been implicated as an apoptotic gatekeeper in TCL, myeloma, and AML. Mcl-1 is expressed in every differentiation step of B-cells and in most MM cells (58). Increased Mcl-1 is also one of the key mechanisms of Bcl-2 inhibitor resistance. It has been shown that AML blasts in recurrent disease have higher levels of Mcl-1 and that Mcl-1 inhibition reverses Bcl-2 inhibitor resistance, potentiating venetoclax activity (58, 59).
Clinical trials of Mcl-1 inhibitors have been limited so far despite potential in preclinical studies, such as AZD5991 (55). Preliminary results from 26 patients with relapsed MM in a phase I trial for an intravenous Mcl-1 inhibitor (AMG-176) demonstrated anti-tumor effect (CR, partial CR) in only 3 patients (60). Concerns have additionally been raised about cardiac toxicity of other Mcl-l-inhibitor candidates, such as AMG-397 (61).
With Bcl-2 and Mcl-1 inhibitors targeting the intrinsic pathway of apoptosis, another approach being considered is targeting the extrinsic pathway of apoptosis (62). The IAP family of proteins, including XIAP and cIAP, inhibit apoptosis through the extrinsic pathway and overexpression has been noted to cause poor outcomes in AML. AEG35156, an antisense oligonucleotide inhibitor of XIAP-induced apoptosis of malignant cells in vitro, but in subsequent clinical trials has failed to meet goals (63, 64). Other forms of IAP inhibition, such as cIAP inhibitors and survivin inhibitors have suffered similar fates, where trials in humans have not yielded adequate results (65, 66).
Further upstream in the extrinsic pathway, TRAIL and other ligands with associated death receptors, such as Fas, TNF-R, TRAIL-R, can trigger death in malignant cells. Various recombinant TRAIL and TRAIL-R agonists have been developed in this context for cancer treatments. Though with good safety profiles, initial candidates have had limited effects. This was attributed to acquired TRAIL-resistance and evidence that oligomerization and anchoring of antibodies may be necessary (67). A first-in-human trial of Abbv-621, a TRAIL receptor agonist fusion protein, in 17 patients with refractory/relapsed AML and DLBCL demonstrated antitumor activity in one patient who was able to achieve CR. Hepatoxicity, increased ALT and AST, was the most common treatment related adverse event, noted in 34.8% of patients (68, 69).
Author contributions
JW: Conceptualization, Writing – original draft, Writing – review & editing. MK: Conceptualization, Writing – original draft, Writing – review & editing.
Funding
The author(s) declare that no financial support was received for the research, authorship, and/or publication of this article.
Conflict of interest
MK received the following: consulting/honorarium from AbbVie, AstraZeneca, Genentech, Gilead, Janssen, MEI Pharma, Sanofi, Stemline Therapeutics, Vincerx, Baxk Therapeutics; research funding/clinical trials support: AbbVie, Ablynx, Allogene, AstraZeneca, Cellectis, Daiichi, Forty Seven, Genentech, Gilead, Immunogen, MEI Pharma, Precision Biosciences, Sanofi, Stemline Therapeutics.
The remaining author declare that the research was conducted in the absence of any commercial or financial relationships that could be construed as a potential conflict of interest.
Publisher’s note
All claims expressed in this article are solely those of the authors and do not necessarily represent those of their affiliated organizations, or those of the publisher, the editors and the reviewers. Any product that may be evaluated in this article, or claim that may be made by its manufacturer, is not guaranteed or endorsed by the publisher.
References
1. Montero J, Letai A. Why do BCL-2 inhibitors work and where should we use them in the clinic? Cell Death Differ (2018) 25(1):56–64. doi: 10.1038/cdd.2017.183
2. Gregory CD. Hijacking homeostasis: Regulation of the tumor microenvironment by apoptosis. Immunol Rev (2023) 319:100–27. doi: 10.1111/imr.13259
3. Zimmermann KC, Green DR. How cells die: Apoptosis pathways. J Allergy Clin Immunol (2001) 108(4, Supplement):S99–103. doi: 10.1067/mai.2001.117819
4. Wu CC, Bratton SB. Regulation of the intrinsic apoptosis pathway by reactive oxygen species. Antioxid Redox Signaling (2013) 19(6):546–58. doi: 10.1089/ars.2012.4905
5. Salah HT, DiNardo CD, Konopleva M, Khoury JD. Potential biomarkers for treatment response to the BCL-2 inhibitor venetoclax: state of the art and future directions. Cancers (2021) 13(12):2974. doi: 10.3390/cancers13122974
6. Shamas-Din A, Kale J, Leber B, Andrews DW. Mechanisms of action of bcl-2 family proteins. Cold Spring Harb Perspect Biol (2013) 5(4):a008714. doi: 10.1101/cshperspect.a008714
7. Westphal D, Kluck RM, Dewson G. Building blocks of the apoptotic pore: how Bax and Bak are activated and oligomerize during apoptosis. Cell Death Differ (2014) 21(2):196–205. doi: 10.1038/cdd.2013.139
8. Roberts AW. Therapeutic development and current uses of BCL-2 inhibition. Hematology (2020) 2020(1):1–9. doi: 10.1182/hematology.2020000154
9. Kaloni D, Diepstraten ST, Strasser A, Kelly GL. BCL-2 protein family: attractive targets for cancer therapy. Apoptosis (2023) 28(1):20–38. doi: 10.1007/s10495-022-01780-7
10. Lowe SW, Lin AW. Apoptosis in cancer. Carcinogenesis (2000) 21(3):485–95. doi: 10.1093/carcin/21.3.485
11. Mohammad RM, Muqbil I, Lowe L, Yedjou C, Hsu HY, Lin LT, et al. Broad targeting of resistance to apoptosis in cancer. Semin. Cancer Biol (2015) 35(0):S78–103. doi: 10.1016/j.semcancer.2015.03.001
12. Frenzel A, Grespi F, Chmelewskij W, Villunger A. Bcl2 family proteins in carcinogenesis and the treatment of cancer. Apoptosis (2009) 14(4):584–96. doi: 10.1007/s10495-008-0300-z
13. Roberts AW, Davids MS, Pagel JM, Kahl BS, Puvvada SD, Gerecitano JF, et al. Targeting BCL2 with venetoclax in relapsed chronic lymphocytic leukemia. N Engl J Med. (2016) 374(4):311–22. doi: 10.1056/NEJMoa1513257
14. Meng Y, Tang W, Dai Y, Wu X, Liu M, Ji Q, et al. Natural BH3-mimetic (-)-gossypol chemosensitizes human prostate cancer via Bcl-xL inhibition accompanied by increase of Puma and Noxa. Mol. Cancer Ther (2008) 7(7):2192–202. doi: 10.1158/1535-7163.MCT-08-0333
15. Xiong J, Li J, Yang Q, Wang J, Su T, Zhou S. Gossypol has anti-cancer effects by dual-targeting MDM2 and VEGF in human breast cancer. Breast Cancer Res (2017) 19:27. doi: 10.1186/s13058-017-0818-5
16. Waites GM, Wang C, Griffin PD. Gossypol: reasons for its failure to be accepted as a safe, reversible male antifertility drug. Int J Androl (1998) 21(1):8–12. doi: 10.1046/j.1365-2605.1998.00092.x
17. Kline MP, Rajkumar SV, Timm MM, Kimlinger TK, Haug JL, Lust JA, et al. ABT-737, an inhibitor of Bcl-2 family proteins, is a potent inducer of apoptosis in multiple myeloma cells. Leukemia (2007) 21(7):1549–60. doi: 10.1038/sj.leu.2404719
18. Mérino D, Khaw SL, Glaser SP, Anderson DJ, Belmont LD, Wong C, et al. Bcl-2, Bcl-xL, and Bcl-w are not equivalent targets of ABT-737 and navitoclax (ABT-263) in lymphoid and leukemic cells. Blood (2012) 119(24):5807–16. doi: 10.1182/blood-2011-12-400929
19. Mohamad Anuar NN, Nor Hisam NS, Liew SL, Ugusman A. Clinical review: navitoclax as a pro-apoptotic and anti-fibrotic agent. Front Pharmacol (2020) 11:564108. doi: 10.3389/fphar.2020.564108
20. Nguyen M, Cencic R, Ertel F, Bernier C, Pelletier J, Roulston A, et al. Obatoclax is a direct and potent antagonist of membrane-restricted Mcl-1 and is synthetic lethal with treatment that induces Bim. BMC Cancer (2015) 15:568. doi: 10.1186/s12885-015-1582-5
21. Goard CA, Schimmer AD. An evidence-based review of obatoclax mesylate in the treatment of hematological Malignancies. Core Evid (2013) 8:15–26. doi: 10.2147/CE.S42568
22. Cang S, Iragavarapu C, Savooji J, Song Y, Liu D. ABT-199 (venetoclax) and BCL-2 inhibitors in clinical development. J Hematol Oncol (2015) 8(1):129. doi: 10.1186/s13045-015-0224-3
23. Juárez-Salcedo LM, Desai V, Dalia S. Venetoclax: evidence to date and clinical potential. Drugs Context (2019) 8:212574. doi: 10.7573/dic.212574
24. Jain N. Selecting frontline therapy for CLL in 2018. Hematol Am Soc Hematol Educ Program (2018) 2018(1):242–7. doi: 10.1182/asheducation-2018.1.242
25. Pekarsky Y, Balatti V, Croce CM. BCL2 and miR-15/16: from gene discovery to treatment. Cell Death Differ (2018) 25(1):21–6. doi: 10.1038/cdd.2017.159
26. Stilgenbauer S, Eichhorst B, Schetelig J, Coutre S, Seymour JF, Munir T, et al. Venetoclax in relapsed or refractory chronic lymphocytic leukaemia with 17p deletion: a multicentre, open-label, phase 2 study. Lancet Oncol (2016) 17(6):768–78. doi: 10.1016/S1470-2045(16)30019-5
27. Al-Sawaf O, Zhang C, Tandon M, Sinha A, Fink AM, Robrecht S, et al. Venetoclax plus obinutuzumab versus chlorambucil plus obinutuzumab for previously untreated chronic lymphocytic leukaemia (CLL14): follow-up results from a multicentre, open-label, randomised, phase 3 trial. Lancet Oncol (2020) 21(9):1188–200. doi: 10.1016/S1470-2045(20)30443-5
28. Kater AP, Owen C, Moreno C, Follows G, Munir T, Levin MD, et al. Fixed-duration ibrutinib-venetoclax in patients with chronic lymphocytic leukemia and comorbidities. NEJM Evid (2022) 1(7):EVIDoa2200006. doi: 10.1056/EVIDoa2200006
29. Seymour JF, Kipps TJ, Eichhorst B, Hillmen P, D’Rozario J, Assouline S, et al. Venetoclax–rituximab in relapsed or refractory chronic lymphocytic leukemia. N Engl J Med (2018) 378(12):1107–20. doi: 10.1056/NEJMoa1713976
30. DiNardo CD, Jonas BA, Pullarkat V, Thirman MJ, Garcia JS, Wei AH, et al. Azacitidine and venetoclax in previously untreated acute myeloid leukemia. N Engl J Med (2020) 383(7):617–29. doi: 10.1056/NEJMoa2012971
31. Wei AH, Montesinos P, Ivanov V, DiNardo CD, Novak J, Laribi K, et al. Venetoclax plus LDAC for newly diagnosed AML ineligible for intensive chemotherapy: a phase 3 randomized placebo-controlled trial. Blood (2020) 135(24):2137–45. doi: 10.1182/blood.2020004856
32. Kantarjian H, Kadia T, DiNardo C, Daver N, Borthakur G, Jabbour E, et al. Acute myeloid leukemia: current progress and future directions. Blood Cancer J (2021) 11(2):1–25. doi: 10.1038/s41408-021-00425-3
33. Rowe JM. Optimal induction and post-remission therapy for AML in first remission. Hematology (2009) 2009(1):396–405. doi: 10.1182/asheducation-2009.1.396
34. Stelmach P, Trumpp A. Leukemic stem cells and therapy resistance in acute myeloid leukemia. Haematologica (2023) 108(2):353–66. doi: 10.3324/haematol.2022.280800
35. Konopleva M, Pollyea DA, Potluri J, Chyla B, Hogdal L, Busman T, et al. Efficacy and biological correlates of response in a phase II study of venetoclax monotherapy in patients with acute myelogenous leukemia. Cancer Discov (2016) 6(10):1106–17. doi: 10.1158/2159-8290.CD-16-0313
36. Samra B, Konopleva M, Isidori A, Daver N, DiNardo C. Venetoclax-based combinations in acute myeloid leukemia: current evidence and future directions. Front Oncol (2020) 10:562558. doi: 10.3389/fonc.2020.562558
37. DiNardo CD, Pratz KW, Letai A, Jonas BA, Wei AH, Thirman M, et al. Safety and preliminary efficacy of venetoclax with decitabine or azacitidine in elderly patients with previously untreated acute myeloid leukaemia: a non-randomised, open-label, phase 1b study. Lancet Oncol (2018) 19(2):216–28. doi: 10.1016/S1470-2045(18)30010-X
38. DiNardo CD, Pratz K, Pullarkat V, Jonas BA, Arellano M, Becker PS, et al. Venetoclax combined with decitabine or azacitidine in treatment-naive, elderly patients with acute myeloid leukemia. Blood (2019) 133(1):7–17. doi: 10.1182/blood-2018-08-868752
39. Wei AH, Strickland SA, Hou JZ, Fiedler W, Lin TL, Walter RB, et al. Venetoclax combined with low-dose cytarabine for previously untreated patients with acute myeloid leukemia: results from a phase Ib/II study. J Clin Oncol (2019) 37(15):1277–84. doi: 10.1200/JCO.18.01600
40. Li X, Miao X, Wang H, Xu Z, Li B. The tissue dependent interactions between p53 and Bcl-2 in vivo. Oncotarget (2015) 6(34):35699–709. doi: 10.18632/oncotarget.5372
41. Wei H, Wang H, Wang G, Qu L, Jiang L, Dai S, et al. Structures of p53/BCL-2 complex suggest a mechanism for p53 to antagonize BCL-2 activity. Nat Commun (2023) 14(1):4300. doi: 10.1038/s41467-023-40087-2
42. Thijssen R, Diepstraten ST, Moujalled D, Chew E, Flensburg C, Shi MX, et al. Intact TP-53 function is essential for sustaining durable responses to BH3-mimetic drugs in leukemias. Blood (2021) 137(20):2721–35. doi: 10.1182/blood.2020010167
43. Nechiporuk T, Kurtz SE, Nikolova O, Liu T, Jones CL, D’Alessandro A, et al. The TP53 apoptotic network is a primary mediator of resistance to BCL2 inhibition in AML cells. Cancer Discov (2019) 9(7):910–25. doi: 10.1158/2159-8290.CD-19-0125
44. Pollyea DA, Pratz KW, Wei AH, Pullarkat V, Jonas BA, Recher C, et al. Outcomes in patients with poor-risk cytogenetics with or without TP53 mutations treated with venetoclax and azacitidine. Clin Cancer Res (2022) 28(24):5272–9. doi: 10.1158/1078-0432.CCR-22-1183
45. Kim K, Maiti A, Loghavi S, Pourebrahim R, Kadia TM, Rausch CR, et al. Outcomes of TP53-mutant acute myeloid leukemia with decitabine and venetoclax. Cancer (2021) 127(20):3772–81. doi: 10.1002/cncr.33689
46. DiNardo CD, Lachowiez CA, Takahashi K, Loghavi S, Xiao L, Kadia T, et al. Venetoclax combined with FLAG-IDA induction and consolidation in newly diagnosed and relapsed or refractory acute myeloid leukemia. JCO (2021) 39(25):2768–78. doi: 10.1200/JCO.20.03736
47. Chua CC, Roberts AW, Reynolds J, Fong CY, Ting SB, Salmon JM, et al. Chemotherapy and venetoclax in elderly acute myeloid leukemia trial (CAVEAT): A phase ib dose-escalation study of venetoclax combined with modified intensive chemotherapy. J Clin Oncol (2020) 38(30):3506–17. doi: 10.1200/JCO.20.00572
48. Reville PK, Kantarjian H, Borthakur G, Yilmaz M, Daver N, Short N, et al. Venetoclax combined with cladribine, idarubicin, cytarabine (CLIA) as induction therapy in patients with newly diagnosed acute myeloid leukemia and high-risk myelodysplastic syndrome. Blood (2022) 140(Supplement 1):1702–4. doi: 10.1182/blood-2022-167931
49. Touzeau C, Maciag P, Amiot M, Moreau P. Targeting Bcl-2 for the treatment of multiple myeloma. Leukemia (2018) 32(9):1899–907. doi: 10.1038/s41375-018-0223-9
50. Gupta VA, Barwick BG, Matulis SM, Shirasaki R, Jaye DL, Keats JJ, et al. Venetoclax sensitivity in multiple myeloma is associated with B-cell gene expression. Blood (2021) 137(26):3604–15. doi: 10.1182/blood.2020007899
51. Rahbari KJ, Nosrati JD, Spektor TM, Berenson JR. Venetoclax in combination with bortezomib, dexamethasone, and daratumumab for multiple myeloma. Clin Lymphoma Myeloma Leukemia (2018) 18(9):e339–43. doi: 10.1016/j.clml.2018.06.003
52. Kumar SK, Harrison SJ, Cavo M, de la Rubia J, Popat R, Gasparetto C, et al. Venetoclax or placebo in combination with bortezomib and dexamethasone in patients with relapsed or refractory multiple myeloma (BELLINI): a randomised, double-blind, multicentre, phase 3 trial. Lancet Oncol (2020) 21(12):1630–42. doi: 10.1016/S1470-2045(20)30525-8
53. Kaufman JL, Quach H, Baz RC, Vangsted AJ, Ho SJ, Harrison SJ, et al. An updated safety and efficacy analysis of venetoclax plus daratumumab and dexamethasone in an expansion cohort of a phase 1/2 study of patients with t(11;14) relapsed/refractory multiple myeloma. Blood (2022) 140(Supplement 1):7261–3. doi: 10.1182/blood-2022-163809
54. Tam CS, Anderson MA, Pott C, Agarwal R, Handunnetti S, Hicks RJ, et al. Ibrutinib plus venetoclax for the treatment of mantle-cell lymphoma. N Engl J Med (2018) 378(13):1211–23. doi: 10.1056/NEJMoa1715519
55. Koch R, Christie AL, Crombie JL, Palmer AC, Plana D, Shigemori K, et al. Biomarker-driven strategy for MCL1 inhibition in T-cell lymphomas. Blood (2019) 133(6):566–75. doi: 10.1182/blood-2018-07-865527
56. Kuusanmäki H, Dufva O, Vähä-Koskela M, Leppä AM, Huuhtanen J, Vänttinen I, et al. Erythroid/megakaryocytic differentiation confers BCL-XL dependency and venetoclax resistance in acute myeloid leukemia. Blood (2023) 141(13):1610–25. doi: 10.1182/blood.2021011094
57. He Y, Koch R, Budamagunta V, Zhang P, Zhang X, Khan S, et al. DT2216—a Bcl-xL-specific degrader is highly active against Bcl-xL-dependent T cell lymphomas. J Hematol Oncol (2020) 13(1):95. doi: 10.1186/s13045-020-00928-9
58. Bolomsky A, Vogler M, Köse MC, Heckman CA, Ehx G, Ludwig H, et al. MCL-1 inhibitors, fast-lane development of a new class of anti-cancer agents. J Hematol Oncol (2020) 13(1):173. doi: 10.1186/s13045-020-01007-9
59. Kaufmann SH, Karp JE, Svingen PA, Krajewski S, Burke PJ, Gore SD, et al. Elevated expression of the apoptotic regulator mcl-1 at the time of leukemic relapse. Blood (1998) 91(3):991–1000. doi: 10.1182/blood.V91.3.991
60. Spencer A, Rosenberg AS, Jakubowiak A, Raje N, Chatterjee M, Trudel S, et al. A phase 1, first-in-human study of AMG 176, a selective MCL-1 inhibitor, in patients with relapsed or refractory multiple myeloma. Clin Lymphoma Myeloma Leukemia (2019) 19(10):e53–4. doi: 10.1016/j.clml.2019.09.081
61. Roberts AW, Wei AH, Huang DCS. BCL2 and MCL1 inhibitors for hematologic Malignancies. Blood (2021) 138(13):1120–36. doi: 10.1182/blood.2020006785
62. Choi JH, Bogenberger JM, Tibes R. Targeting apoptosis in acute myeloid leukemia: current status and future directions of BCL-2 inhibition with venetoclax and beyond. Targ Oncol (2020) 15(2):147–62. doi: 10.1007/s11523-020-00711-3
63. Hu Y, Cherton-Horvat G, Dragowska V, Baird S, Korneluk RG, Durkin JP, et al. Antisense oligonucleotides targeting XIAP induce apoptosis and enhance chemotherapeutic activity against human lung cancer cells in vitro and in vivo. Clin Cancer Res (2003) 9(7):2826–36.
64. Schimmer AD, Herr W, Hänel M, Borthakur G, Frankel A, Horst HA, et al. Addition of AEG35156 XIAP antisense oligonucleotide in reinduction chemotherapy does not improve remission rates in patients with primary refractory acute myeloid leukemia in a randomized phase II study. Clin Lymphoma Myeloma Leukemia (2011) 11(5):433–8. doi: 10.1016/j.clml.2011.03.033
65. Donnellan WB, Diez-Campelo M, Heuser M, Ritchie EK, Skolnik J, Font P, et al. A phase 2 study of azacitidine (5-AZA) with or without birinapant in subjects with higher risk myelodysplastic syndrome (MDS) or chronic myelomonocytic leukemia (CMML). JCO (2016) 34(15_suppl):7060–0. doi: 10.1200/JCO.2016.34.15_suppl.7060
66. Erba HP, Sayar H, Juckett M, Lahn M, Andre V, Callies S, et al. Safety and pharmacokinetics of the antisense oligonucleotide (ASO) LY2181308 as a single-agent or in combination with idarubicin and cytarabine in patients with refractory or relapsed acute myeloid leukemia (AML). Invest New Drugs (2013) 31(4):1023–34. doi: 10.1007/s10637-013-9935-x
67. Wajant H. Molecular mode of action of TRAIL receptor agonists—Common principles and their translational exploitation. Cancers (2019) 11(7):954. doi: 10.3390/cancers11070954
68. de Jonge MJA, Carneiro BA, Devriese L, Doi T, Penugonda S, Petrich AM, et al. First-in-human study of abbv-621, a TRAIL receptor agonist fusion protein, in patients (Pts) with relapsed/refractory (RR) acute myeloid leukemia (AML) and diffuse large B-cell lymphoma (DLBCL). Blood (2019) 134:3924. doi: 10.1182/blood-2019-129783
Keywords: venetoclax, Bcl-2, apoptosis, CLL, AML
Citation: Wei JX and Konopleva M (2023) Bcl-2 inhibition in the treatment of hematologic malignancies. Front. Hematol. 2:1307661. doi: 10.3389/frhem.2023.1307661
Received: 05 October 2023; Accepted: 17 November 2023;
Published: 04 December 2023.
Edited by:
Natalia Neparidze, Yale University, United StatesReviewed by:
Sylvain Garciaz, Institut Paoli-Calmettes (IPC), FranceCopyright © 2023 Wei and Konopleva. This is an open-access article distributed under the terms of the Creative Commons Attribution License (CC BY). The use, distribution or reproduction in other forums is permitted, provided the original author(s) and the copyright owner(s) are credited and that the original publication in this journal is cited, in accordance with accepted academic practice. No use, distribution or reproduction is permitted which does not comply with these terms.
*Correspondence: Marina Konopleva, bWFyaW5hLmtvbm9wbGV2YUBlaW5zdGVpbm1lZC5lZHU=