- 1Department of Microbiology & Immunology, Division of Hematology/Oncology, Indiana University School of Medicine, Indianapolis, IN, United States
- 2Department of Hematology and Immune Therapeutics, Moderna Therapeutics, Cambridge, MA, United States
- 3Department of Medicine, Division of Hematology/Oncology, Indiana University School of Medicine, Indianapolis, IN, United States
Prostaglandin E2 (PGE2) signaling through its EP4 receptor regulates hematopoietic stem and progenitor cell (HSPC) functions. Here we generated mouse strains with conditional and inducible deletion of EP4 in stromal cell populations, including osteolineage cells, mesenchymal progenitor cells, perivascular stromal cells, and endothelial cells, to evaluate the role of EP4 in HSPC regulation through signaling in each of these niche cell populations. We found that EP4 deletion in different stromal cells had distinct effects on HSPC proliferation, long-term repopulating capacity, and the peripheral blood stem cell mobilization response. Lack of EP4 signaling in osteolineage cells increased HSPC number but impaired their long-term engraftment and mobilization. EP4 deletion in mesenchymal progenitor cells and endothelial cells reduced HSPC number and function, while EP4 deletion in perivascular stromal cells had sex-specific effects on HSPC engraftment. Our results demonstrate that PGE2/EP4 signaling in bone marrow stromal cells plays a significant and complex role in HSPC regulation, with both positive and negative effects depending on the stromal cell type.
Introduction
The regulation of hematopoiesis, the process of blood cell production and differentiation, depends on the interactions between hematopoietic stem and progenitor cells (HSPCs) and their bone marrow microenvironment, also known as the niche. The bone marrow niche consists of various stromal cell populations that provide physical support, cell-cell contacts, and soluble factors to HSPCs, influencing their maintenance, proliferation, differentiation, and trafficking (1–4). Among the stromal cell types, osteolineage cells, mesenchymal progenitor cells (MPCs), perivascular stromal cells, and endothelial cells (ECs) have been shown to play important roles in regulating HSPC functions through distinct or overlapping mechanisms (4–7).
Prostaglandin E2 (PGE2) is a bioactive lipid mediator that modulates various aspects of hematopoiesis, such as HSPC development (8), self-renewal, homing and engraftment (8–11), proliferation (11–15) and trafficking (12, 13). PGE2 exerts its effects by binding to four G-protein-coupled receptors, EP1-4, which have different signaling pathways and tissue distributions (16, 17). Previous studies have demonstrated that EP4 is the dominant receptor for PGE2 in hematopoietic tissues and that EP4 signaling directly regulates HSPC function (13, 18). Moreover, EP4 signaling in bone marrow stromal cells has been implicated in HSPC regulation, as EP4 activation in MPCs enhances cytokine production that facilitates recovery from myelosuppression (18), and EP4 deletion in osteolineage cells affects HSPC retention and mobilization (13). However, the role of EP4 signaling in other stromal cell populations, such as perivascular stromal cells and ECs, and its impact on HSPC fate and function are not fully understood.
In this study, we generated mouse strains with conditional and inducible deletion of EP4 in specific stromal cell populations, including osteolineage cells, MPCs, perivascular stromal cells, and ECs, to investigate the role of EP4 signaling in HSPC regulation in different bone marrow niches. We found that EP4 deletion in different stromal cells had distinct effects on HSPC number, function, and mobilization, depending on the stromal cell type and the HSPC subset. Our results reveal that EP4 signaling has differential effects in bone marrow stromal cell populations with both positive and negative regulatory actions on hematopoiesis and HSPC trafficking.
Materials and methods
Reagents
AMD3100 (Mozobil®) was a gift from Sanofi-Genzyme, Cambridge, MA. GROβ (SB251353) was obtained from SmithKline Beecham Pharmaceuticals (GlaxoSmithKline, King of Prussia, PA). G-CSF (Neupogen®) was purchased from McKesson (Irving, TX). Tamoxifen was purchased from Sigma-Aldrich (St. Louis, MO) and suspended at 200 mg/ml in sunflower oil.
Mice
B6.Cg-Ndor1Tg(UBC-cre/ERT2)1Ejb/1J (Strain#:007001, UBC-Cre-ERT2) expressing a tamoxifen-inducible Cre recombinase driven by the constitutive Ubiquitin C (UBC) promoter (19), B6.FVB-Tg(BGLAP-cre)1Clem/J (Strain#:019509, OC-cre) transgenic mice with Cre expression directed by the bone gamma carboxyglutamate protein (BGLAP) promoter/enhancer (osteoblast specific) (20), B6.Cg-Tg(Sp7-tTA,tetO-EGFP/cre)1Amc/J (Strain #:006361, Osx1-GFP::Cre) transgenic mice carrying both tTA under the regulation of the osterix (Sp7) promoter and a tetracycline responsive element (TRE; tetO)-controlled GFP/Cre fusion protein, under the direction of doxycycline (21), C57BL/6-Tg(Nes-cre/ERT2)KEisc/J (Strain #:016261, Nestin-cre/ERT2) mice expressing a tamoxifen-inducible Cre recombinase gene under direction of the nestin (Nes) promoter (22), B6.129(Cg)-Leprtm2(cre)Rck/J (Strain #:JAX:008320, ObRb-Cre), transgenic mice with Cre recombinase recognizing the gene for the Leptin receptor (LepR) (23), and B6.FVB-Tg(Tek-icre/ERT2)1Soff/J (Strain #:030597, Tie-2-CreER) mice expressing a tamoxifen-inducible Cre recombinase gene under direction of the TEK receptor tyrosine kinase (Tek) promoter (24), were purchased from Jackson Laboratories, Bar Harbor, ME. The specific stromal cell populations/lineages in which EP4 is deleted are shown in Table 1.
B6.SJL-PtrcAPep3B/BoyJ (BOYJ) (CD45.1) mice were bred at the Indiana University School of Medicine Simon Comprehensive Cancer Center (IUSCC) In Vivo Therapeutic Core facility. Mice containing a conditional allele for the prostaglandin EP4 receptor (EP4ff) mice (25) were a gift from Dr. R Breyer, Vanderbilt University and maintained in house. All mice were housed at 22 ± 2°C and 45-65% humidity with a 12 h light/dark cycle and given free access to food and sterile water. Osx1-Cre transgenic mice and offspring were maintained on water with 1 mg/ml doxycycline and 5 mg/ml glucose. All mice were on the C57Bl/6 (CD45.2) background. All mice were genotyped by PCR on 1% agarose using forward and reverse primers found on the Jackson Laboratories website. The IUSM IACUC approved all studies.
Conditional strain development and gene deletion
Selective Cre reporter strain development was accomplished by mating EP4ff mice to each reporter strain to create EP4 f/+, Cre mice. Heterozygous mice were then crossed to obtain EP4 ff-Cre mice. EP4ff -Nestin-cre, -Tie2-cre and -UBC-cre mice were treated with tamoxifen ip at 100 mg/kg body weight for 5 consecutive days and mice analyzed 10 d after the last tamoxifen treatment. EP4 deletion in transgenic strains was validated by qRT-PCR and/or FACS on sorted cells, or by PCR on tail tissue (Supplementary Figure 1). In all cases, Cre-mediated EP4 deletion was >90%.
Cell preparation
Bone marrow cells were harvested by crushing femurs, tibias and pelvis with a sterile mortar and pestle, in cold DPBS with 2% FBS (STEMCELL, Vancouver, Canada) and cell suspensions harvested. The remaining crushed bone fragments were rinsed with media and combined, centrifuged, and washed once. For isolation of BM stromal cells, flushed femurs and tibias were crushed and digested in collagenase (0.02%) at 37°C for 15 min. Cells were collected and washed twice. Spleen cells were isolated by pressing spleens between the frosted ends of two sterile microscope slides. Spleen cells were washed and suspended in DPBS with 2% FBS. PB was obtained by cardiac puncture using EDTA-coated syringes and dispensed into EDTA microtainers (BD, Franklin Lakes, NJ). PB complete blood counts (CBC) were measured by using an Element HT5 Veterinary Hematology Analyzer (HESKA, Loveland, CO).
Antibodies and flow cytometry analysis
All antibodies were purchased from BioLegend (San Diego, CA) unless otherwise noted. For detection of SLAM SKL, lineage negative BM cells were stained with PE-cy7 conjugated Sca-1 (clone D7), APC- conjugated c-Kit (clone 2B8), Percp-cy5.5–conjugated anti-CD150 (TC15-12F12.2) and PE–conjugated anti-CD48 (clone HM48-1). Leptin+ stromal cells were labeled with anti-LepR (biotinylated, R&D Systems), anti-CD45 (clone 30F-11), anti-CD31 (clone MEC13.3) and anti-Ter119 (clone ter-119) antibodies. MSC were labeled with anti-CD45 (clone 30F-11), anti-Ter119, anti-CD51 (clone RMV-7), and anti-PDGFRα (clone APB5). MSC were also labeled with anti-Nestin (clone 307501, R&D Systems, Minneapolis, MN). EC were labeled with antibodies to CD45, Ter119, CD51, CD31, and VE-cadherin (clone eBioBV13, BD Biosciences, San Diego, CA). Osteoblasts (OBs) and MPC were labeled with antibodies to CD45 (30F-11), CD31 (390), Ter119, Sca-1 and ALCAM. For analysis of PB chimerism, anti-CD45.2 (clone 104) and anti-CD45.1 (clone A20) monoclonal antibodies were utilized. Anti-Mac1/CD11b (clone M1/70), anti-CD45R/B220 (clone RA3-6B2), anti CD3 (clone500A2) and anti-CD19 (clone 6D5) were utilized for analysis of PB tri-lineage reconstitution. Anti-EP4 antibody (ab188761) was purchased from Abcam (Waltham, MA). All flow cytometry analyses were performed on an LSRII flow cytometer (BD). Cell sorting was performed on a BD Aria or Reflection II or Reflection III sorters.
Bone marrow transplantation
Competitive marrow transplantation was performed on a side-by-side basis for each strain and littermate controls (EP4ff, Cre-negative) as we described (26). Equal numbers of total BM cells (0.5x106) from all strains (CD45.2) were admixed with Boy/J (CD45.1) BM cells and transplanted into lethally irradiated Boy/J mice (total body 137 Cs irradiation, 1100 cGy, split dose 6 h apart, 24 h prior to transplant) using a Mark 1 Irradiator (JL Shepard, San Fernando, CA). Peripheral blood chimerism and tri-lineage reconstitution was monitored monthly for up to 24 wk. In each transplant, marrow cells from 4-8 control or EP4 gene-deleted mice of each sex were evaluated. If no sex difference was noted, male and female data were combined. If sex related differences were seen, data are presented separately.
Colony-forming cell assay
Bone marrow, spleen and peripheral blood CFC were quantitated using ColonyGEL™ 1202 mouse complete medium (ReachBio, Seattle, WA) as we described (13). Whole marrow and spleen cells were plated at 2x104 and 1x106 cells per dish. For peripheral blood CFC, 200ul od whole blood was subjected to RBC lysis and 50 ul plated per dish. CFU-GEMM, CFU-GM, BFU-E in triplicate dishes were scored microscopically after 7 days at 37°C, 5% CO2 and 5% O2. Total bone marrow CFCs per 2 femurs, 2 tibias and pelvis was calculated and adjusted according to the fraction of total marrow mass represented by the bones utilized (27).
Peripheral blood stem cell mobilization
Mobilization experiments were performed as we described (28). AMD3100 5 mg/kg and GROβ 2.5 mg/kg were administered subcutaneously (sc) as single doses or in combination. PB was analyzed at 60 min post AMD3100, and 15 min post GROβ alone or AMD plus GROβ. G-CSF was administered sc at 62.5 ug/kg/day, bid for 4 days and PB analyzed ~16 h after the final dose. Injections were scheduled so that all mobilized mice were evaluated at the same time in every experiment. Mice were sacrificed by CO2 asphyxiation and PB was obtained by cardiac puncture using EDTA-coated syringes. Peripheral blood mononuclear cells (PBMC) were obtained by separation on Lympholyte Mammal (Cedarlane Labs Ltd, Hornby, Ontario, Canada (29). CFC and SLAM-SKL cells were evaluated.
Quantitative RT–PCR
EP4 gene deletion was confirmed by qRT-PCR using the following primers: EP4 forward primer- GTGCGGAGATCCAGATGGTC; reverse primer-TCACCACGTTTGGCTGATATAAC. Total RNA was isolated from lineage negative BM cells, and FACS sorted CD45- stromal cells based upon expression of Nestin, LepR, Tie2, Osteocalcin or Osterix, using a QIAGEN RNeasy Mini Kit, or QIAGEN RNeasy Micro Kit. cDNA was reverse transcribed from total RNA using SuperScript VILO (Life technologies, Invitrogen) according to the manufacturers’ protocols and subjected to real-time PCR using SYBR Green Super mix (Life Technologies). Quantitation was performed on an Applied Biosystems quantitative real time PCR thermal cycler (Life Technologies). All samples were run in triplicate. Amplification of glyceraldehyde 3-phosphate dehydrogenase (GAPDH) and hypoxanthine-guanine phosphoribosyltransferase (HPRT) were used for sample normalization.
Statistical analysis
All data are shown as Mean ± SEM. Statistical analysis was performed using Microsoft Excel and GraphPad Prism 7.0 software. Two-tailed Student’s t-tests were performed for statistical analysis between two groups. One-way analysis of variance (ANOVA) with Tukey’s correction was used to compare the difference in means between more than three groups. A P value <0.05 was considered statistically significant.
Results
Deletion of EP4 is perinatal lethal in mice (30, 31). Using EP4(flox) mice (25), we created conditional and inducible mouse strains where EP4 is selectively deleted in BM stromal cell populations to determine the role of PGE2/EP4 signaling in regulating HSPC function.
PB, spleen and BM cellularity and phenotype-defined BM HPC and HSC populations
Steady state peripheral blood counts were essentially normal in mice with a global inducible deletion of EP4, although a modest decrease in red blood cells (RBCs) was noted (Table 2A). A significantly reduced PLT count was seen in Osteocalcin-cre mice with no effect on any other blood parameter. In Osterix-Cre mice, lack of EP4 signaling was associated with decreased white blood cells due to significantly fewer lymphocytes. Deletion of EP4 in Nestin+ MPCs, perivascular LepR stromal cells and Tie-2 ECs had no effect on peripheral blood cell numbers. Total nucleated spleen cells were significantly decreased in Nestin-cre and Tie2-Cre mice (Table 2B). Total bone marrow cellularity was not significantly different between all EP4ff and EP4 knockout mouse strains (Table 2C).
However, although total BM cellularity was unchanged, significant changes in phenotypically defined hematopoietic cells were observed upon EP4 deletion (Table 2C). In Tamoxifen-cre mice, global deletion of EP4 resulted in significantly fewer phenotype-defined hematopoietic progenitor cells (LSK) and stem cells (SLAM-LSK) as well as granulocyte-macrophage progenitor cells (GMPs). In EP4ff-Osteocalcin-cre mice, deletion of EP4 in mature OBs resulted in significantly more LSK cells but fewer common lymphoid progenitor cells (CLPs). EP4 deletion in immature osteolineage cells in EP4-Osterix-cre mice also resulted in fewer CLPs. Deletion of EP4 in Nestin+ MPCs resulted in significantly fewer LSK cells, while deletion of EP4 in Tie2+ sinusoidal ECs resulted in significantly lower numbers of both LSK and SLAM-LSK HSCs.
Overall, these data indicate differential roles for PGE2/EP4 signaling in maintenance of BM hematopoietic cells, by both endosteal and vascular stromal cells.
Regulation of hematopoietic function
Since phenotype defined HPC and HSC do not always accurately reflect function (13), we evaluated functional HPC by colony formation and long-term repopulating HSCs (LTRCs) by primary and secondary hematopoietic transplantation. EP4 gene deletion resulted in increased peripheral blood CFCs in Tamoxifen-cre and Lepr-cre mice, resulting from significant increases in CFU-GM and BFU-E (Figure 1A). In contrast, blood CFCs were significantly lower in Osterix-cre and Tie2-cre mice, primarily due to decreases in CFU-GM. Although the spleen is not a major organ for hematopoiesis under steady state conditions, significantly fewer myeloid and erythroid CFCs were observed in Nestin-cre and Tie2-cre mice (Figure 1B). The decrease in myeloid and erythroid CFCs was also seen in EP4-Tamoxifen-Cre mice. No effect on spleen CFCs was noted in mice where EP4 was deleted in ECs. In steady state bone marrow, significantly fewer myeloid and erythroid CFCs were observed in Tamoxifen-cre, Nestin-Cre, LepR-cre, and Tie2-cre mice (Figure 1C). Modest but significantly greater numbers of CFU-GM and BFU-E were seen in EP4-Osterix-cre mice.
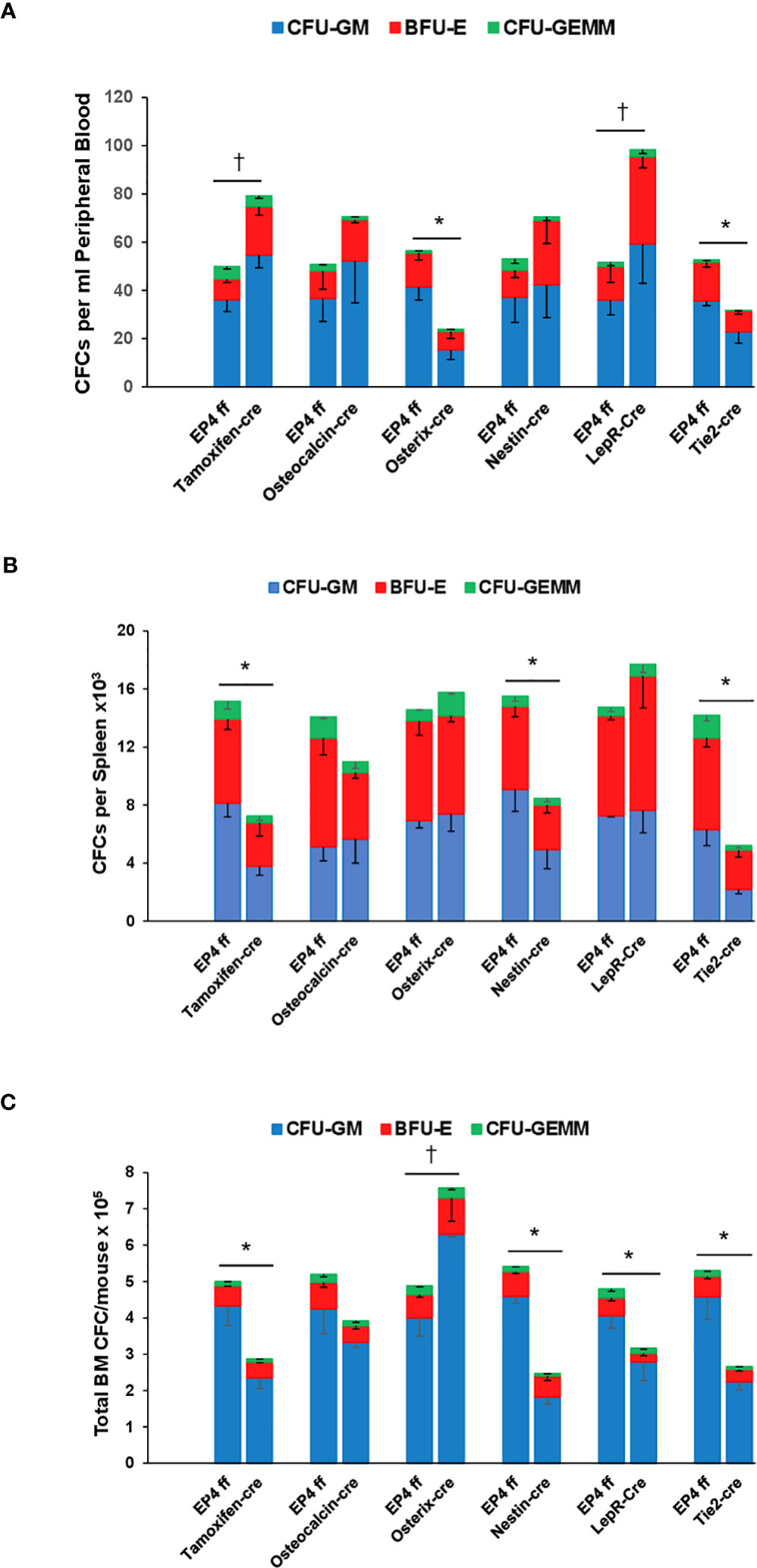
Figure 1 Steady state CFCs (CFU-GM, BFU-E and CFU-GEMM) in (A) peripheral blood, (B) spleen and (C) bone marrow in EP4ff control mice and upon EP4 deletion. Data are expressed as Mean ± SEM of each colony type. Data are presented as CFC per 1 ml peripheral blood, total CFC per whole spleen and total bone marrow CFC per mouse. Data are from 4-6 mice per group each assayed individually. *P<0.05 decrease compared to EP4ff control. †P<0.05 increase compared to EP4ff control.
Next, we evaluated the effect of stromal cell selective EP4 gene deletion on long-term repopulating capacity and lineage reconstitution of HSCs in primary and secondary transplantation assays. Since all genetic strains were on the C57Bl/6 CD45.2 background, competitive transplant of bone marrow cells from littermate EP4ff controls or cre-EP4 gene deletion mice admixed with equal numbers of BoyJ (CD45.1) were transplanted into BoyJ recipient mice. EP4ff control and gene deletion transplants were performed side-by-side on the same day and in the same cohort of irradiated recipient mice.
In primary transplants, no difference was seen in repopulating capacity of HSCs from Osteocalcin-cre or littermate EP4ff mice or in their lineage reconstitution ability (Figure 2A Left). However, upon secondary transplant, marrow cells that had originated from Osteocalcin-cre mice showed significantly reduced ability to contribute to hematopoiesis compared to EP4ff littermate controls Figure 2A-Right. In addition, a significant myeloid bias was observed in these secondary recipients. Long-term repopulation capacity in primary and secondary transplants was equivalent in male and female mice.
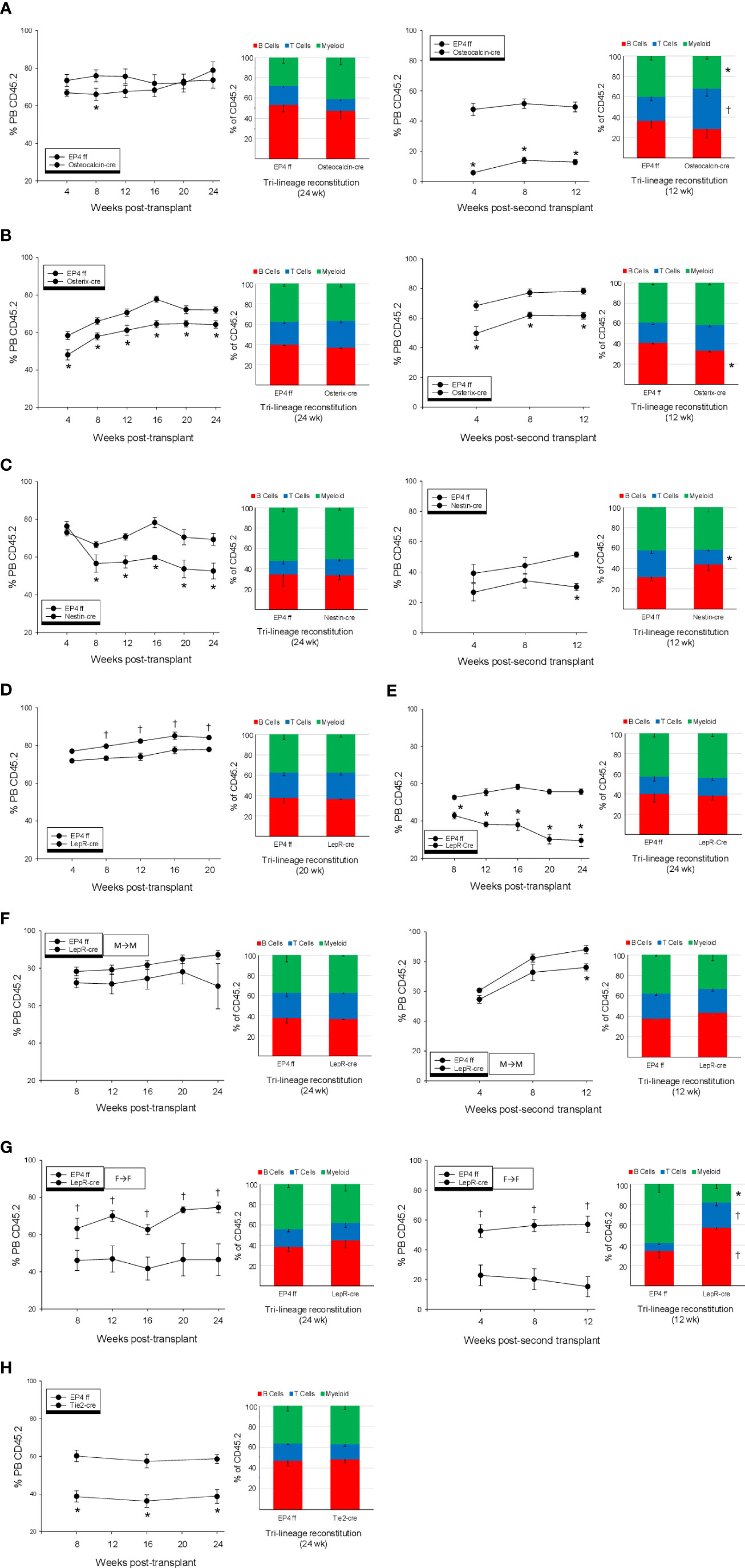
Figure 2 Primary and secondary competitive transplantation of whole bone marrow cells using (CD45.2) from EP4ff controls and mice where EP4 was deleted in (A) Osteoblasts, (B) early osteolineage progenitor cells, (C) Nestin+ Mesenchymal progenitor cells, (D) Leptin receptor expressing perivascular cells and (E) Tie-2 expressing sinusoidal endothelial cells was performed in side-side comparison. In (A–C, H), data from male and female mice are combined; equal numbers per group, N=8-10 per group. Data in (D–G) are from 5 male or female mice per group. In primary transplants, Percent (CD45.2) chimerism in peripheral blood and lineage reconstitution were determined monthly for up to 24 wk in primary transplants and 12 wk in secondary transplants. All data are expressed as Mean ± SEM. *P<0.05 decrease compared to EP4ff control. †P<0.05 increase compared to EP4ff control.
Transplants using marrow from Osterix-cre mice showed significantly reduced repopulation capacity in both primary (Figure 2B-Left) and secondary (Figure 2B-Right) transplants. Blood lineage reconstitution was normal in recipients of marrow from Osterix-cre mice, whereas B cell reconstitution was significantly lower in secondary recipients. No sex differences were noted. LTR capacity of BM cells from Nestin-cre mice were significantly reduced in primary and secondary transplants (Figure 2C, Left and Right panels, respectively). PB lineage reconstitution was normal in primary transplants, whereas T cells were significantly lower in secondary recipients. No sex differences were noted.
A significant difference in repopulation capacity was noted in marrow cells from male and female LepR-cre mice. Male marrow cells showed a modest, but significant enhanced reconstitution compared to male cells from EP4ff littermates (Figure 2D), whereas female cells showed significant reduction (Figure 2E). No differences in lineage reconstitution by male or female cells were observed. Since cells were transplanted into mixed male and female BoyJ recipients, additional transplants were performed where the sex of donor cells and recipients was the same. Male marrow cells from LepR-cre mice when transplanted into male BoyJ recipients showed equal repopulating capacity to EP4ff littermate control in both primary (Figure 2F-Left) and secondary transplants (Figure 2F-Right). Lineage reconstitution in all transplant recipients were equivalent. Surprisingly, female marrow cells from LepR-cre mice when transplanted into female BoyJ recipients showed dramatically enhanced reconstitution compared to female EP4ff littermate controls in both primary (Figure 2G-Left) and secondary (Figure 2G-Right) transplants. Lineage reconstitution was equivalent between EP4ff and LepR-cre recipients in primary transplants, however in secondary transplants recipients of marrow from primary LepR recipients showed a significant increase in lymphoid reconstitution with reduced myeloid cells.
Finally, in evaluation of endothelial cell effects, repopulation from marrow cells from Tie2-cre mice was significantly reduced compared to cells from EP4ff controls in primary transplant (Figure 2H). Lineage reconstitution was equivalent between EP4ff and Tie2-cre mice. No sex differences in LTR capacity or lineage reconstitution were observed.
Hematopoietic mobilization
Inhibition of EP4 signaling in vivo by non-steroidal anti-inflammatory drugs (NSAIDs) or EP4 antagonists enhances HPC/HSC mobilization by G-CSF and mobilizes a graft with enhanced repopulating capacity (13). Mobilization by the combination of AMD3100 and the chemokine GROβ results in rapid mobilization of a high engrafting population of HSCs (28). We therefore tested mobilization by G-CSF, AMD3100 and GROβ alone and in combination in each of the EP4 deletion strains.
Deletion of EP4 in osteoblasts results in enhanced HPC mobilization by G-CSF and by AMD+GROβ (Figure 3A-Left), suggesting that PGE2 signaling through EP4 promotes endosteal retention of HPC. However, mobilization of phenotype-defined (p) HSCs was impaired in response to G-CSF or AMD+GROβ (Figure 3A-Right). Mobilization of HSCs by GROβ alone was also impaired. These results suggest that in contrast to HPC, EP4 signaling is required for HSC mobilization.
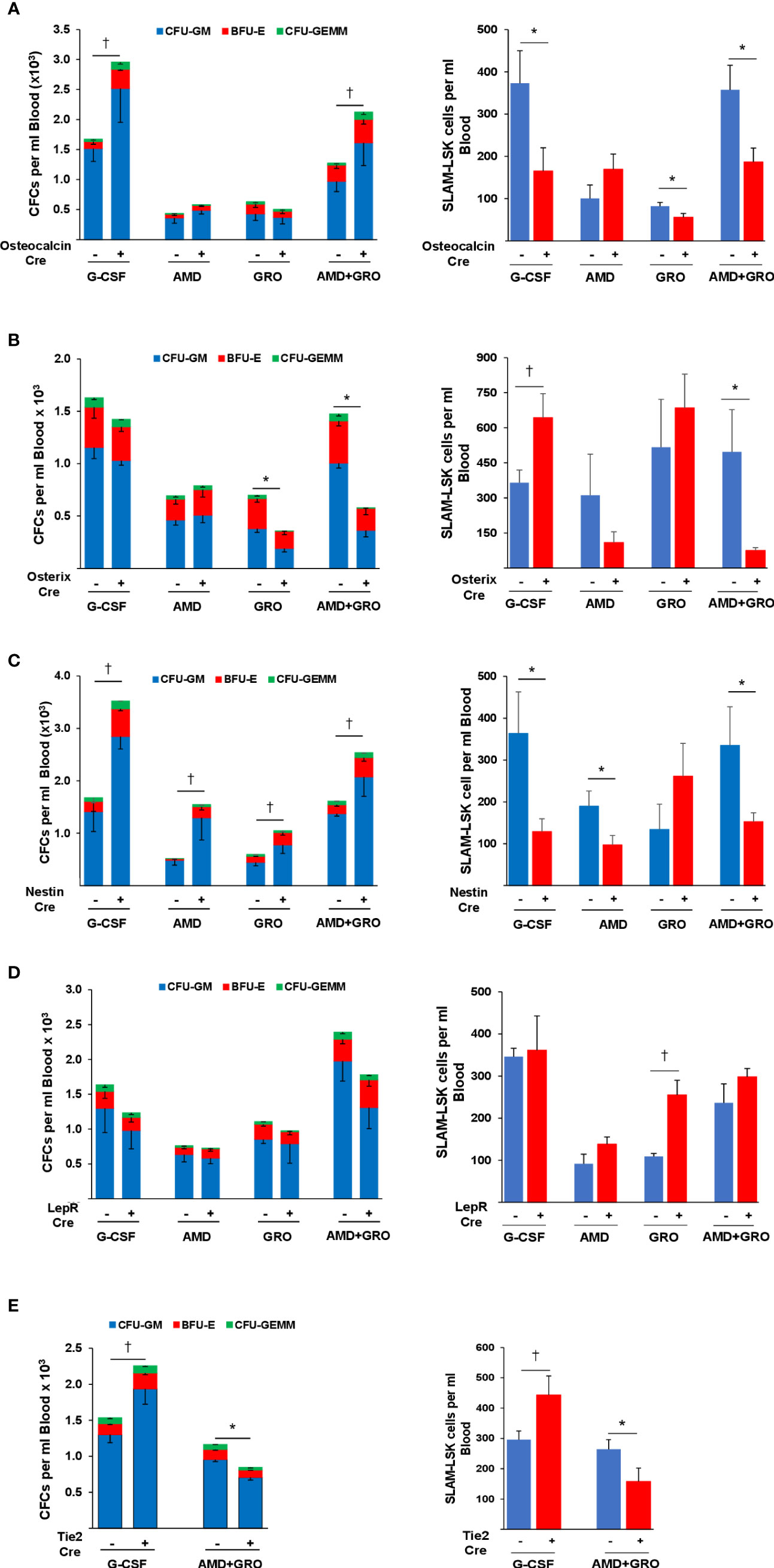
Figure 3 Mobilization of CFCs and pHSCs to peripheral blood by G-CSF, AMD3100, GROβ and the combination of AMD+GROβ in EP4ff control and EP4 gene deleted (A) osteocalcin-cre (B) Osterix-cre (C) Nestin-cre (D) LepR-cre and (E) Tie2-cre mice. Data are Mean ± SEM from 4 mice per group each assayed individually. *P<0.05 decrease compared to EP4ff control. †P<0.05 increase compared to EP4ff control.
In Osterix-cre mice, EP4 deletion in immature osteolineage cells had no effect on mobilization of HPC by G-CSF, but HPC mobilization by GROβ alone and in combination with AMD3100 were significantly impaired (Figure 3B-Left). Mobilization of pHSCs by G-CSF was enhanced by deletion of EP4, but mobilization by AMD+GROβ was significantly impaired (Figure 3B-Right). HPC mobilization by G-CSF, AMD and GROβ alone and AMD+GROβ were all significantly enhanced in EP4ff-Nestin-cre mice (Figure 3C-Left). However, mobilization of pHSCs by G-CSF, AMD and AMD+GROβ were all impaired (Figure 3C-Right).
Deletion of EP4 in LepR-cre mice had no effect on HPC mobilization (Figure 3D-Left). Similarly, HSC mobilization was largely unaffected by EP4 deletion although mobilization by GROβ alone was enhanced (Figure 3D-Right). G-CSF induced mobilization of HPCs (Figure 3E-Left) and pHSCs (Figure 3E-Right) was enhanced after EP4 deletion in sinusoidal EC, while mobilization of HPC and HSC by AMD+GROβ was impaired.
These data reveal distinct and overlapping roles for PGE2/EP4 signaling in stromal cells in the regulation of HPC and HSC mobilization. They also highlight the differences between the mobilization of HPC and HSC by the chronic administration of G-CSF versus the acute administration of AMD3100 and GROβ Furthermore, they demonstrate the heterogeneity and complexity of the stromal cell populations that support and modulate HPC and HSC function in the bone marrow.
Discussion
The bone marrow hematopoietic environment is a complex and heterogeneous system, influenced by various cell types. The notion of spatially segregated HSC niches might be an oversimplification. Instead, bone marrow stromal cell populations may regulate hematopoietic function in unique or overlapping manners. While we and others have demonstrated that PGE2/EP4 signaling can regulate HSPCs via stromal cells (13, 18), a more granular assessment of the differential role of this EP4 signaling was not done. In this study, we sought to increase our understanding of the differential roles of niche cells by developing numerous conditional and inducible genetic stromal cell EP4 knockout strains. Our findings further support that PGE2/EP4 signaling across multiple stromal cell populations is necessary for optimal HPC and HSC regulation, and that this regulatory function is dependent on stromal cell type and context.
Steady state peripheral blood parameters were mostly unaffected by EP4 deletion in stromal cells. However, a slight decrease in RBC was observed in mice with a global inducible deletion of EP4, which likely represents loss of the anti-apoptotic function of PGE2 (9), as RBCs are mainly regulated through apoptosis. A significantly reduced PLT count was observed in Osteocalcin-cre mice with no effect on blood cell parameters. Although PGE2 influences platelet activation and aggregation, effects of PGE2 signaling in OBs on platelet formation have not been reported. In Osterix-Cre mice, the absence of EP4 signaling corresponded with a decrease in white blood cells due to a significant reduction in lymphocyte count. A significant decrease in the number of bone marrow common lymphoid progenitor cells was also noted, possibly pointing to the cause of reduced lymphocyte production. Deletion of SDF-1 in osterix-expressing stromal cells has been associated with loss of B-lymphoid progenitor cells (6). Since PGE2 promotes CXCR4 expression and stabilization (9, 32) and lack of EP signaling is associated with reduced SDF-1 (13, 18), it is possible that the reduced lymphocytes and lymphoid progenitor cells in Osterix-cre mice result from impaired cytokine support of lymphopoiesis, although further exploration is required to definitively determine this finding.
Significant hematopoietic endogenous mobilization/egress occurred in LepR-cre mice, without a change in total peripheral white blood cell count and was associated with a slight decrease in bone marrow CFCs, suggesting that EP4 signaling in LepR stromal cells enforces HSPC retention. In contrast, deletion of EP4 in Osterix-cre mice led to fewer blood progenitors found in steady state peripheral blood with a concomitant increase in marrow progenitor count. This suggests that in Osterix+ immature osteolineage cells, EP4 signaling either opposes HSPC retention or allows for hematopoietic egress. In prior studies, blockade of PGE2 synthesis with NSAIDs or EP4 deletion identified a role in mature osteoblasts in HSC retention (13). Thus, it appears that the functional role of EP4 signaling in HSPC retention is different in mature and immature osteolineage cells. Deletion of EP4 in sinusoidal endothelial cells also identified differential mobilization mechanisms for HSC and HPC.
Lack of EP4 signaling in multiple stromal cell types significantly affected long-term repopulating capacity. EP4 deletion in Tie2+ ECs resulted in a significant reduction in HSC transplant function. Endothelial cells produce growth factors required for HSC maintenance including SCF, SDF-1 and Pleiotrophin, and conditional deletion of any of these factors depletes HSC number (5–7, 33, 34). Deletion of EP4 in these cells also reduced HSC numbers, likely explaining the reduction in transplant repopulation, and suggests that EP4 signaling is necessary to maintain normal endothelial hematopoietic support.
An intriguing and unexpected finding was the sex difference in repopulating capacity in bone marrow acquired from LepR+/EP4 knockout mice. While bone marrow cells from male LepR-cre mice showed a modestly enhanced function compared to controls, bone marrow cells from female LepR-cre mice were significantly impaired. This impairment was only seen when female cells were transplanted into male recipient mice. Functional capacity was restored when the recipients were also female. Why this occurs only with female HSCs is unclear. What is clear is that the finding is a result of decreased EP4 signaling in Lepr+ stromal cells. HSC express receptors for and respond to sex hormones (35, 36), and androgens and estrogens can stimulate hematopoiesis (37–39). A higher proportion of HSC and multipotent progenitors are dividing in female mice and endogenous estrogen increases HSC division in female mice (39). It is possible that when female HSC are transplanted into a male bone marrow environment, they do not receive sufficient sex hormone signal to maintain cell proliferation. LepR+ stromal cells are a major source of growth factors that stimulate HSC division and block apoptosis (5, 7). Lack of EP4 signaling is associated with reduced production of stomal cell factors that support hematopoiesis (13) and PGE2 blocks apoptosis in HSC, through release of stromal growth factors (9, 13). It’s possible that given the higher proliferate rate of female HSC, that in an environment of reduced growth factors and potentially increased apoptosis, a program of impaired proliferation is initiated, but can be rescued and in fact enhanced in a female bone marrow environment.
The EP4 receptor is the most highly expressed EP receptor in hematopoietic tissue (40) and the affinity of PGE2 is highest for the EP4 receptor (41). Prior work, and this current study, support a complex and nuanced regulatory role for PGE2/EP4 within the bone marrow environment for regulation of hematopoiesis. Using genetic animal models, the differential and overlapping roles of distinct niche populations are beginning to be revealed. Future research should focus on further elucidating these signaling pathways, particularly in the context of hematopoietic dysfunction and stem cell transplantation.
Data availability statement
The original contributions presented in the study are included in the article/Supplementary Materials. Further inquiries can be directed to the corresponding author: lpelus@iu.edu.
Ethics statement
The animal study was approved by Indiana University School of Medicine IACUC. The study was conducted in accordance with the local legislation and institutional requirements.
Author contributions
LL: Formal Analysis, Investigation, Methodology, Writing – original draft. HL: Formal Analysis, Investigation, Writing – original draft. PS: Investigation, Methodology, Writing – original draft. JH: Conceptualization, Writing – original draft, Writing – review & editing. LP: Conceptualization, Data curation, Formal Analysis, Funding acquisition, Investigation, Methodology, Supervision, Validation, Writing – original draft, Writing – review & editing.
Funding
The author(s) declare financial support was received for the research, authorship, and/or publication of this article. This work was supported by grant HL096305 (LMP) from the National Heart, Lung and Blood Institute, NIH.Flow cytometry was performed at the Flow Cytometry Resource Facility of the Indiana University (IU) Melvin and Bren Simon Cancer Center (NCI grant P30 CA082709). Flow cytometry was also supported by a Center of Excellence Grant in Molecular Hematology (PO DK090948).
Conflict of interest
Author JH was employed by the company Moderna Therapeutics.
The authors declare that the research was conducted in the absence of any commercial or financial relationships that could be construed as a potential conflict of interest.
The author(s) declared that they were an editorial board member of Frontiers, at the time of submission. This had no impact on the peer review process and the final decision.
Publisher’s note
All claims expressed in this article are solely those of the authors and do not necessarily represent those of their affiliated organizations, or those of the publisher, the editors and the reviewers. Any product that may be evaluated in this article, or claim that may be made by its manufacturer, is not guaranteed or endorsed by the publisher.
Supplementary material
The Supplementary Material for this article can be found online at: https://www.frontiersin.org/articles/10.3389/frhem.2023.1292651/full#supplementary-material
Supplementary Figure 1 | Validation of EP4 deletion. (A) relative expression of EP4 mRNA in total BM cells from Tamoxifen-cre mice. (B) EP4 mRNA expression in CD45-, CD31-, Ter119-, Sca1-, Alcam+ osteoblasts. (C) EP4 gene deletion in Osterix-cre mice. (D) EP4 mRNA expression (left) and EP4 receptor expression (MFI) (right) in CD45-, CD51+, PDGFRα+, Nestin+ MSC. (E) EP4 mRNA expression on CD45-, CD31-, Ter119-, Sca1+, Alcam-, LepR+ MPC (immature osteolineage cells). (F) MFI of EP4 expression on CD45-, Ter119-, CD31+, CD51-, VE-cadherin+ bone marrow sinusoidal endothelial cells.
References
1. Morrison SJ, Scadden DT. The bone marrow niche for haematopoietic stem cells. Nature (2014) 505:327–34. doi: 10.1038/nature12984
2. Frenette PS, Pinho S, Lucas D, Scheiermann C. Mesenchymal stem cell: keystone of the hematopoietic stem cell niche and a stepping-stone for regenerative medicine. Annu Rev Immunol (2013) 31:285–316. doi: 10.1146/annurev-immunol-032712-095919
3. Schofield R. The relationship between the spleen colony-forming cell and the haemopoietic stem cell. Blood Cells (1978) 4:7–25.
4. Hoggatt J, Kfoury Y, Scadden DT. Hematopoietic stem cell niche in health and disease. Annu Rev Pathol (2016) 11:555–81. doi: 10.1146/annurev-pathol-012615-044414
5. Ding L, Morrison SJ. Haematopoietic stem cells and early lymphoid progenitors occupy distinct bone marrow niches. Nature (2013) 495:231–5. doi: 10.1038/nature11885
6. Greenbaum A, Hsu YM, Day RB, Schuettpelz LG, Christopher MJ, Borgerding JN, et al. CXCL12 in early mesenchymal progenitors is required for haematopoietic stem-cell maintenance. Nature (2013) 495:227–30. doi: 10.1038/nature11926
7. Ding L, Saunders TL, Enikolopov G, Morrison SJ. Endothelial and perivascular cells maintain haematopoietic stem cells. Nature (2012) 481:457–62. doi: 10.1038/nature10783
8. North TE, Goessling W, Walkley CR, Lengerke C, Kopani KR, Lord AM, et al. Prostaglandin E2 regulates vertebrate haematopoietic stem cell homeostasis. Nature (2007) 447:1007–11. doi: 10.1038/nature05883
9. Hoggatt J, Singh P, Sampath J, Pelus LM. Prostaglandin E2 enhances hematopoietic stem cell homing, survival, and proliferation. Blood (2009) 113:5444–55. doi: 10.1182/blood-2009-01-201335
10. Hoggatt J, Mohammad KS, Singh P, Pelus LM. Prostaglandin E2 enhances long-term repopulation but does not permanently alter inherent stem cell competitiveness. Blood (2013) 122:2997–3000. doi: 10.1182/blood-2013-07-515288
11. Pelus LM, Hoggatt J. Pleiotropic effects of prostaglandin E2 in hematopoiesis; prostaglandin E2 and other eicosanoids regulate hematopoietic stem and progenitor cell function. Prostaglandins Other Lipid Mediat (2011) 96:3–9. doi: 10.1016/j.prostaglandins.2011.06.004
12. Hoggatt J, Pelus LM. Eicosanoid regulation of hematopoiesis and hematopoietic stem and progenitor trafficking. Leukemia (2010) 24:1993–2002. doi: 10.1038/leu.2010.216
13. Hoggatt J, Mohammad KS, Singh P, Hoggatt AF, Chitteti BR, Speth JM, et al. Differential stem- and progenitor-cell trafficking by prostaglandin E2. Nature (2013) 495:365–9. doi: 10.1038/nature11929
14. Pelus LM, Broxmeyer HE, Kurland JI, Moore MA. Regulation of macrophage and granulocyte proliferation. Specificities of prostaglandin E and lactoferrin. J Exp Med (1979) 150(2):277–92. doi: 10.1084/jem.150.2.277
15. Pelus LM, Broxmeyer HE, Moore MA. Regulation of human myelopoiesis by prostaglandin E and lactoferrin. Cell Tissue Kinet (1981) 14:515–26. doi: 10.1111/j.1365-2184.1981.tb00557.x
16. Sugimoto Y, Narumiya S. Prostaglandin E receptors. J Biol Chem (2007) 282(16):11613–7. doi: 10.1074/jbc.R600038200
17. Breyer RM, Bagdassarian CK, Myers SA, Breyer MD. Prostanoid receptors: subtypes and signaling. Annu Rev Pharmacol Toxicol (2001) 41:661–90. doi: 10.1146/annurev.pharmtox.41.1.661
18. Ikushima YM, Arai F, Hosokawa K, Toyama H, Takubo K, Furuyashiki T, et al. Prostaglandin E(2) regulates murine hematopoietic stem/progenitor cells directly via EP4 receptor and indirectly through mesenchymal progenitor cells. Blood (2013) 121:1995–2007. doi: 10.1182/blood-2012-06-437889
19. Ruzankina Y, Pinzon-Guzman C, Asare A, Ong T, Pontano L, Cotsarelis G, et al. Deletion of the developmentally essential gene ATR in adult mice leads to age-related phenotypes and stem cell loss. Cell Stem Cell (2007) 1:113–26. doi: 10.1016/j.stem.2007.03.002
20. Zhang M, Xuan S, Bouxsein ML, von SD, Akeno N, Faugere MC, et al. Osteoblast-specific knockout of the insulin-like growth factor (IGF) receptor gene reveals an essential role of IGF signaling in bone matrix mineralization. J Biol Chem (2002) 277(46):44005–12. doi: 10.1074/jbc.M208265200
21. Rodda SJ, McMahon AP. Distinct roles for Hedgehog and canonical Wnt signaling in specification, differentiation and maintenance of osteoblast progenitors. Development (2006) 133:3231–44. doi: 10.1242/dev.02480
22. Battiste J, Helms AW, Kim EJ, Savage TK, Lagace DC, Mandyam CD, et al. Ascl1 defines sequentially generated lineage-restricted neuronal and oligodendrocyte precursor cells in the spinal cord. Development (2007) 134:285–93. doi: 10.1242/dev.02727
23. DeFalco J, Tomishima M, Liu H, Zhao C, Cai X, Marth JD, et al. Virus-assisted mapping of neural inputs to a feeding center in the hypothalamus. Science (2001) 291:2608–13. doi: 10.1126/science.1056602
24. Korhonen H, Fisslthaler B, Moers A, Wirth A, Habermehl D, Wieland T, et al. Anaphylactic shock depends on endothelial Gq/G11. J Exp Med (2009) 206(2):411–20. doi: 10.1084%2Fjem.20082150
25. Schneider A, Guan Y, Zhang Y, Magnuson MA, Pettepher C, Loftin CD, et al. Generation of a conditional allele of the mouse prostaglandin EP4 receptor. Genesis (2004) 40:7–14. doi: 10.1002/gene.20048
26. Patterson AM, Liu L, Sampson CH, Plett PA, Li H, Singh P, et al. A single radioprotective dose of prostaglandin E2 blocks apoptotic signaling and early irradiation-induced cycling of hematopoietic stem cells. Stem Cell Rep (2020) 15:358–373. doi: 10.1016/j.stemcr.2020.07.004
27. Boggs DR. The total marrow mass of the mouse: a simplified method of measurement. Am J Hematol (1984) 16:277–86. doi: 10.1002/ajh.2830160309
28. Hoggatt J, Singh P, Tate TA, Chou BK, Datari SR, Fukuda S, et al. Rapid mobilization reveals a highly engraftable hematopoietic stem cell. Cell (2018) 172:191–204. doi: 10.1016/j.cell.2017.11.003
29. Hoggatt J, Tate TA, Pelus LM. Hematopoietic stem and progenitor cell mobilization in mice. Methods Mol Biol (2014) 1185:43–64. doi: 10.1007/978-1-4939-1133-2_4
30. Nguyen M, Camenisch T, Snouwaert JN, Hicks E, Coffman TM, Anderson PA, et al. The prostaglandin receptor EP4 triggers remodelling of the cardiovascular system at birth. Nature (1997) 390:78–81. doi: 10.1038/36342
31. Segi E, Sugimoto Y, Yamasaki A, Aze Y, Oida H, Nishimura T, et al. Patent ductus arteriosus and neonatal death in prostaglandin receptor EP4-deficient mice. Biochem Biophys Res Commun (1998) 246(1):7–12. doi: 10.1006/bbrc.1998.8461
32. Speth JM, Hoggatt J, Singh P, Pelus LM. Pharmacologic increase in HIF1alpha enhances hematopoietic stem and progenitor homing and engraftment. Blood (2014) 123:203–7. doi: 10.1182/blood-2013-07-516336
33. Chen Q, Liu Y, Jeong HW, Stehling M, Dinh VV, Zhou B, et al. Apelin(+) endothelial niche cells control hematopoiesis and mediate vascular regeneration after myeloablative injury. Cell Stem Cell (2019) 25:768–83. doi: 10.1016/j.stem.2019.10.006
34. Himburg HA, Termini CM, Schlussel L, Kan J, Li M, Zhao L, et al. Distinct bone marrow sources of pleiotrophin control hematopoietic stem cell maintenance and regeneration. Cell Stem Cell (2018) 23:370–81. doi: 10.1016/j.stem.2018.07.003
35. Abdelbaset-Ismail A, Suszynska M, Borkowska S, Adamiak M, Ratajczak J, Kucia M, et al. Human haematopoietic stem/progenitor cells express several functional sex hormone receptors. J Cell Mol Med (2016) 20(1):134–46. doi: 10.1111/jcmm.12712
36. Mierzejewska K, Borkowska S, Suszynska E, Suszynska M, Poniewierska-Baran A, Maj M, et al. Hematopoietic stem/progenitor cells express several functional sex hormone receptors-novel evidence for a potential developmental link between hematopoiesis and primordial germ cells. Stem Cells Dev (2015) 24:927–37. doi: 10.1089/scd.2014.0546
37. Maggio M, Snyder PJ, Ceda GP, Milaneschi Y, Luci M, Cattabiani C, et al. Is the haematopoietic effect of testosterone mediated by erythropoietin? The results of a clinical trial in older men. Andrology (2013) 1:24–8. doi: 10.1111/j.2047-2927.2012.00009.x
38. Carreras E, Turner S, Paharkova-Vatchkova V, Mao A, Dascher C, Kovats S. Estradiol acts directly on bone marrow myeloid progenitors to differentially regulate GM-CSF or Flt3 ligand-mediated dendritic cell differentiation. J Immunol (2008) 180:727–38. doi: 10.4049/jimmunol.180.2.727
39. Nakada D, Oguro H, Levi BP, Ryan N, Kitano A, Saitoh Y, et al. Oestrogen increases haematopoietic stem-cell self-renewal in females and during pregnancy. Nature (2014) 505:555–8. doi: 10.1038/nature12932
40. Konya V, Marsche G, Schuligoi R, Heinemann A. E-type prostanoid receptor 4 (EP4) in disease and therapy. Pharmacol Ther (2013) 138(3):485–502. doi: 10.1016/j.pharmthera.2013.03.006
Keywords: prostaglandin E2 EP4 receptor, hematopoietic stem cells (HSCs), colony forming cells (CFCs), bone marrow transplant, peripheral blood hematopoietic cell mobilization
Citation: Liu L, Li H, Singh P, Hoggatt J and Pelus LM (2023) Differential roles of prostaglandin E2 EP4 receptor on stromal cell populations for hematopoietic stem and progenitor cell function. Front. Hematol. 2:1292651. doi: 10.3389/frhem.2023.1292651
Received: 11 September 2023; Accepted: 07 November 2023;
Published: 27 November 2023.
Edited by:
Bin Guo, Shanghai Jiao Tong University, ChinaReviewed by:
Neeraj Kumar Satija, Indian Institute of Toxicology Research (CSIR), IndiaZhongbo Hu, St. Jude Children’s Research Hospital, United States
Copyright © 2023 Liu, Li, Singh, Hoggatt and Pelus. This is an open-access article distributed under the terms of the Creative Commons Attribution License (CC BY). The use, distribution or reproduction in other forums is permitted, provided the original author(s) and the copyright owner(s) are credited and that the original publication in this journal is cited, in accordance with accepted academic practice. No use, distribution or reproduction is permitted which does not comply with these terms.
*Correspondence: Louis M. Pelus, lpelus@iu.edu
†Present address: Pratibha Singh, Department of Pediatrics, Indiana University School of Medicine, Indianapolis, IN, United States
‡These authors have contributed equally to this work