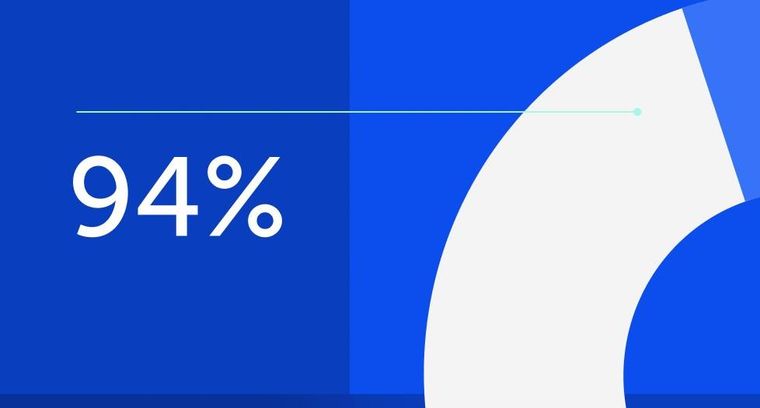
94% of researchers rate our articles as excellent or good
Learn more about the work of our research integrity team to safeguard the quality of each article we publish.
Find out more
ORIGINAL RESEARCH article
Front. Health Serv., 07 September 2022
Sec. Cost and Resource Allocation
Volume 2 - 2022 | https://doi.org/10.3389/frhs.2022.960945
This article is part of the Research TopicAdvancements and Challenges in Cost and Resource Allocation: 2022View all 5 articles
Introduction: Over 50,000 very low birth weight (VLBW) infants are born each year in the United States. Despite advances in care, these premature babies are subjected to long stays in a neonatal intensive care unit (NICU), and experience high rates of morbidity and mortality. In a large randomized controlled trial (RCT), heart rate characteristics (HRC) monitoring in addition to standard monitoring decreased all-cause mortality among VLBW infants by 22%. We sought to understand the cost-effectiveness of HRC monitoring to improve survival among VLBW infants.
Methods: We performed a secondary analysis of cost-effectiveness of heart rate characteristics (HRC) monitoring to improve survival from birth to NICU discharge, up to 120 days using data and outcomes from an RCT of 3,003 VLBW patients. We estimated each patient's cost from a third-party perspective in 2021 USD using the resource utilization data gathered during the RCT (NCT00307333) during their initial stay in the NICU and applied to specific per diem rates. We computed the incremental cost-effectiveness ratio and used non-parametric boot-strapping to evaluate uncertainty.
Results: The incremental cost-effectiveness ratio of HRC-monitoring was $34,720 per life saved. The 95th percentile of cost to save one additional life through HRC-monitoring was $449,291.
Conclusion: HRC-monitoring appears cost-effective for increasing survival among VLBW infants.
The over 50,000 infants born each year in the United States with very low birth weight (VLBW; <1,500 g) are at high risk of mortality and morbidity and spend long periods in a neonatal intensive care unit (NICU) before discharge. Unsurprisingly, expenses to care for these premature patients are high, and the annual cost to care for these VLBW patients may exceed $10B in the US alone (1–7).
In one of the largest randomized controlled trials (RCT) ever conducted among VLBW patients, heart rate characteristics (HRC) monitoring in addition to standard monitoring decreased all-cause mortality by 22% (HR = 0.78; absolute risk from 10.2 to 8.1%) when compared with patients randomized to standard monitoring alone (8). Further, HRC-display was associated with a 40% reduction in mortality after infection (from 19.6 to 11.8%) (9) and improvement in survival without neurodevelopmental impairment among patients who developed a bacterial infection (10).
We sought to contextualize the costs of HRC-monitoring by undertaking a cost-effectiveness analysis of HRC-monitoring to improve survival using resource utilization and outcomes data from the RCT. The results may inform decision-making among clinicians caring for premature infants, hospitals undertaking technology assessments, third-party payers making coverage decisions, and nationalized health systems considering adoption of HRC monitoring.
As HRC-monitoring has been used as an indicator of the inflammatory status of NICU patients and as an early warning of infection (11–14), VLBW patients at eight study centers (nine hospitals) were randomized to either receive standard of care monitoring or standard of care monitoring plus HRC-monitoring (HeRO monitors provided by Medical Predictive Science Corporation, Charlottesville, Virginia, USA) using blocked allocation at each site using computer-generated sequences (NCT00307333). The trial was pragmatic in design; that is, there were no mandated interventions based on HRC. Rather, patients were randomized to receive either HRC-display or non-display while in the NICU during the subsequent 120 days, and then outcomes were tracked, including duration of mechanical ventilation, length of stay, antibiotic usage, and mortality. In the prespecified analyses, patients in the HRC-display group experienced reduced mortality in the 120-days after randomization when compared with controls (10.2 vs. 8.1%, p = 0.04) (8). This RCT had mortality data on 99.5% of the randomized patients in addition to resource utilization data including NICU length of stay and daily ventilatory status over a 120-day time horizon and post-discharge follow-up where appropriate, providing the opportunity to evaluate cost-effectiveness of the intervention.
We performed a retrospective analysis of cost-effectiveness of HRC-monitoring to improve survival from a third-party payer's perspective where we included the direct medical costs and overhead from birth to NICU discharge, up to 120 days since birth, for patients in the RCT. This time horizon is typical of NICHD Neonatal Research Network studies, and includes ~80% of the costs of care and 98–99% of infant mortality in the premature infant population (15–19).
We estimated each patient's cost adjusted to 2021 USD using the resource utilization data from the case report forms gathered daily during the NICU stay and applied to per diem rates that include both hospital and physician services for the level of acuity (20) used in previous economic evaluations of RCTs among premature infants (21, 22). We assigned a daily cost of care for each patient based on the postnatal age and level of acuity (mechanically ventilated vs. non-mechanically ventilated) as follows, Day 1: $4,241.00 or $1,442.44; Days 2–28: $3,562.42 or $1,163.87; Days 29+: $3,577.12 or $1,163.87. These costs were derived from a cost-effectiveness analysis of corticosteroid therapy (20), although we used only two levels of acuity (mechanically ventilated vs. non-mechanically ventilated), the cited study used three levels of acuity (mechanically ventilated, continuous positive air pressure, and no respiratory support). The per-diem hospital costs were derived from patient charge data including personnel charges, non-personnel charges, and allocated hospital overhead values, and were converted to cost using center-specific federal cost-to-charge ratios (21).
For patients randomized to HRC-display, we used information from the manufacturer to calculate costs associated with HRC-monitoring (the system can be leased from the manufacturer for a total cost of 2021 USD 3.25 per patient per day in the NICU with no additional equipment costs; in-person staff training costs are additional, but on-line staff training is provided at no cost. For the purposes of this analysis, only the $3.25/day cost is used). This cost was not attributed to patients in the non-display group.
We computed the incremental cost-effectiveness ratio by calculating the difference in mean cost between the patients randomized to standard monitoring plus HRC-display vs. standard monitoring alone and dividing by the difference in survival between the two study groups. Hence, the incremental cost-effectiveness ratio can be interpreted as the total direct third-party costs in the first 120 days to save one additional life of a very low birth weight infant through HRC-monitoring.
We used non-parametric bootstrapping to evaluate the uncertainty of these estimates. In each of 5,000 simulations, we sampled each patient with replacement. For each simulation, we calculated the difference in mean survival between the two arms of the RCT, the difference in the mean cost, the incremental cost-effectiveness ratio, and the cost per survivor for the HRC-display and non-display patients. We report the results of the bootstrapping analysis as the 95th percentile, corresponding to a traditional confidence interval upper limit.
In addition, we performed deterministic sensitivity analyses by varying (a) the cost of HRC-monitoring, (b) the per-diem cost of time in the NICU, each by 25, 50, 200, and 400%, and then recomputing the incremental cost-effectiveness ratio, (c) varying the ratio of ventilated costs by 25, 50, 200, and 400% while holding non-ventilated costs constant, and (d) varying the ratio of non-ventilated costs by 25, 50, 200, and 400% while holding ventilated costs constant. The latter two address possible changes in ventilatory practice patterns since this dataset was generated. Finally, we corrected for changes in care since the completion of the RCT by decreasing mortality by 20% (23, 24) and recalculating the ICER.
To provide additional context, we evaluated the cost-effectiveness of HRC-monitoring from a hospital perspective, where we converted reimbursable costs to expected charges using the mean cost-to-charge ratio for all US hospitals.
All cost and charge data are presented in 2021 US Dollars. Conversions to US dollars were performed using exchange rates at the time of conversion as published by the US Treasury (for those studies in the Discussion that are used as comparisons) (25), and adjustments to account for inflation were performed using the US Bureau of Labor Statistics Medical CPI (Medical care in U.S. city average, all urban consumers, not seasonally adjusted) (26). All statistical calculations were performed in R (27). We followed the Consolidated Health Economic Evaluation Reporting Standards (CHEERS) (28).
Table 1 shows baseline demographics and outcomes for the patients randomized to standard of care monitoring (non-display) and standard of care monitoring plus HRC-monitoring (HRC-display). The report of the RCT found no statistically significant differences in baseline characteristics between the two arms of the study (8).
Total cost of care for patients randomized to HRC-display was $108,834 (SD: $85,454) vs. $108,066 (SD: $86,877) for non-display. HRC-monitoring alone added a mean cost to HRC-display patients of $208 (SD: $108). There was a longer median length of stay among HRC-display patient [60 days (IQR: 38–90) vs. 59 days (IQR: 37–90)].
Using a time horizon of 120 days, this analysis of cost-effectiveness revealed small increases in both mean cost ($768/patient) and effectiveness (2.2% absolute increase in survival) for patients randomized to HRC-display. From these figures, we calculated the incremental cost-effectiveness ratio as $34,720 per life saved.
Figure 1 shows the results of 5,000 bootstrapped replications of the RCT with difference in cost plotted against difference in effectiveness (survival) for each simulation. HRC-monitoring was effective in improving survival in 98% of the replications, and less expensive in 39% of the replications. In 38% of the replications, HRC-monitoring was both more effective and less expensive (dominant). Whereas, in 1% of the replications, HRC-monitoring was less effective and more expensive (dominated). The highest proportion of replications, 60%, indicated HRC-monitoring to be both more effective and more expensive.
Figure 1. Scatterplot of difference in costs vs. difference in effect (survival) between HRC-display and non-display arms for each of 5,000 simulations. The large majority of points in the right half of the graph indicates a high level of confidence in the conclusion that HRC-monitoring was effective at reducing mortality; the smaller majority of data in the top half indicates a lower level of confidence that HRC-monitoring resulted in increased costs.
Figure 2 plots the cost effectiveness acceptability curve which shows the probability that HRC-monitoring is cost-effective at a range of thresholds of willingness-to-pay to save one life. The 95th percentile, corresponding to a traditional confidence interval limit, was $449,291, plotted with a dashed line.
Figure 2. Cost-effectiveness acceptability curve. The black line represents the fraction of bootstrap replications for which HRC-monitoring was cost effective at the willingness-to-pay threshold represented along the x-axis. The dashed line represents the willingness-to-pay threshold at which 95% of simulations returned a lower incremental cost-effectiveness ratio: $449,291.
The incremental cost-effectiveness ratio was sensitive to changes when we varied both HRC cost (from 0.25x to 4x) and NICU cost (also from 0.25x to 4x). Figure 3 shows that the incremental cost-effectiveness ratio ranged from $27,668 per life saved when HRC costs were adjusted by 0.25x, to $62,927 when HRC costs were adjusted by 4x. The incremental cost-effectiveness ratio ranged from $15,732 when NICU costs were adjusted by 0.25x, to $110,674 when NICU costs were adjusted by 4x. Furthermore, when we broke NICU costs down into ventilated costs and non-ventilated costs, we saw that varying non-ventilated costs caused cost-effectiveness to range from –$1,341 per life saved (where a negative value indicates that the intervention both reduces costs and improves survival) at 0.25x to $178,966 per life saved at 4x, while holding ventilated costs constant. When holding non-ventilated costs constant, cost-effectiveness ranged from $46,102 per life saved when ventilated costs were adjusted by 0.25x to –$33,572 when ventilated costs were varied by 4x. The difference in sign between ventilated and non-ventilated days is explained by non-significant trends toward more ventilated days in the non-display patients, yet more non-ventilated days in the HRC-display patients. We note that the highest figure in the sensitivity analyses, $178,966 per life saved, was obtained when non-ventilatory days were raised by 4x, an adjustment that would make non-ventilated days higher in cost than ventilated days, an implausible scenario. Finally, when we decreased baseline mortality by 20% to adjust for trends in survival since the completion of the RCT, we found the incremental cost-effectiveness ratio increased to $43,400 per life saved.
Figure 3. Sensitivity of incremental cost effectiveness ratio to variation in costs. Cost-effectiveness was more sensitive to changes in NICU Costs than to changes in HRC Costs. When analyzing ventilated vs. non-ventilated as separate components of NICU cost, cost-effectiveness varied directly with ventilated costs, but inversely with non-ventilated costs (that is, cost per life saved went down when ventilated costs went up, and cost per life saved went up when non-ventilated costs went up).
From a hospital perspective, the difference in chargeable costs per patient is (1 – 0.08) × ($108,626–$108,066) (Table 1), or $515 per patient, after assuming that 8% of total per diem costs are represented by physician services, which are not reimbursable to the hospital (22). Using the median cost-to-charge ratio of US hospitals of 0.25 (29), a hospital could expect to be reimbursed for $515/0.25, or $2,061. Hence, the net profit from a hospital perspective to HRC-monitor one VLBW patient is $2,061–$515–$208, or $1,338 per VLBW patient. Figure 4 details the cost decision tree.
Using data from a large RCT conducted among VLBW infants, we estimated the third-party cost per life saved with HRC-monitoring, $34,720. This figure is roughly equivalent to the total cost of caring for infants born at 34-weeks gestational age as reported in a study of birth hospitalization costs in California, and more cost-effective per life saved than caring for a baby of any gestational age category below 34 weeks. Notably, in the same report, the cost per survivor (mean cost per patient divided by rate of survival) for the 23- and 24-week gestational age averaged $700,000–$750,000 in 2021 dollars (7).
The primary driver of the incremental cost to save a life through HRC-monitoring was not the $208 mean cost per patient of HRC-monitoring, which comprised roughly one quarter of the $768 mean cost difference. Instead, the primary cost driver of saving a life through HRC-monitoring was the additional time spent in the NICU, because more patients survived to discharge in the HRC-display arm.
Clinicians evaluating the cost-effectiveness of NICU HRC monitoring may apply these data to their unit from a hospital perspective. HRC-monitoring can be leased from the manufacturer at $3.25 per day per occupied bed. For a 20-bed unit with 80% occupancy the estimated annual cost to HRC-monitor all beds is $3.25 × 20 × 80% × 365, or $18,980 per year. The number needed to treat to save one VLBW life through HRC-monitoring is 48 (8). Assuming that only VLBW patients receive a benefit from HRC-monitoring even though all NICU patients would be monitored, if a 20-bed unit admits 48 VLBW patients per year, then one additional life would be saved at the cost of $18,980 for HRC-monitoring plus chargeable costs of $24,730, that is 48 × (1 – 0.08) × ($108,626 – $108,066), assuming that physician services comprise 8% of per diem costs (22). If the hospital's cost-to-charge ratio is a typical 0.25 (27), the hospital could be expected to bill third-party payers for an additional $2,061 per VLBW patient, that is (1 – 0.08) × ($108,626 – $108,066)/0.25, or $98,918 annually. Hence, the net profit to the hypothetical 20-bed NICU to implement HRC-monitoring is $55,209 per year, that is $98,918–$18,980–$24,730, and would result in one additional life saved per year. Universal HRC-monitoring of NICU patients is likely to improve the top-line and bottom-line of the hospital while survival also improves.
Parental costs either during or after NICU stay were not included, and thus we were unable to evaluate cost-effectiveness from a societal perspective. Other studies indicate that lost time at work is the primary driver of parental costs (22), which would be likely to have been highly correlated with length of stay in the NICU during the 120-day time horizon of this analysis, just as the other drivers of cost that were included in this analysis (HRC-monitoring is priced per day in the NICU, and our cost model of resource utilization is also primarily driven by length of NICU stay). Hence, results when evaluating from a societal perspective are unlikely to be substantively different than those from a third-party payer's perspective.
Some new therapies offer both reduced costs and improved outcomes. Antenatal betamethasone was found to decrease both costs of care and respiratory morbidity (20), and caffeine treatment for apnea of prematurity was found to both lower costs and decrease death or neurodevelopmental impairment (30).
More often, new interventions are expensive when they are introduced, and the incremental cost to improve an outcome is much higher than the average cost of care for the patients without the new technology. Inhaled nitric oxide is labeled for use among term infants with persistent pulmonary hypertension where it was found to improve survival at a cost of $56,132 per survivor (31). While the American Academy of Pediatrics recommended against the routine use of inhaled nitric oxide for premature infants (32), the NO CLD study did find that inhaled nitric oxide was cost-effective at reducing the composite outcome of death or bronchopulmonary dysplasia among premature infants at an estimated cost of $29,696 to reduce one case of death or bronchopulmonary dysplasia (21). Extracorporeal membrane oxygenation among neonates with severe respiratory failure was found to cost $38,885 per year of life saved (converted from pound sterling) (33), universal hearing screening was found to cost $80,691 per case of deafness (34), and donor human milk was found to cost $4,766 per averted case of necrotizing enterocolitis (converted from Canadian dollars) (15). In light of these commonly used neonatal interventions, universal HRC-monitoring among VLBW patients would appear to be a cost-effective means of improving an important outcome.
Strengths of this analysis include a large dataset collected under auspices of an RCT protocol at multiple centers throughout the US including outcomes data along with utilization data. Weaknesses of this analysis include the inability to estimate costs from a societal perspective, and the retrospective analysis of a dataset collected when rates of infection and ventilatory practices were different, and which have likely influenced the incidence, presentation, and response to sepsis in positive and negative ways. Further, the indirect method of estimating patients' costs through utilization records based primarily on ventilatory status rather than directly collecting cost data could have masked differences in resource utilization and cost, more so than the referenced studies (20–22) that used three levels of respiratory support as opposed to two. While the relatively short time-horizon of 120 days adequately captures changes in length of stay in the NICU associated with HRC-monitoring in this population where median length of stay was ~60 days, it may fail to capture life-long costs associated with survival after prematurity and/or increased survival associated with HRC-monitoring (35) (we note that we have found no difference in the rate of neurodevelopmental impairment among ELBW survivors of sepsis that were HRC-monitored compared with those that were not (29 vs. 29%, respectively, p = 0.95) (10), or among all ELBW survivors that were HRC-monitored compared to those that were not (19.8 vs. 17.9%, respectively, p = 0.63) (35), offering some expectation that future costs related to the increased survivorship after HRC-monitoring are not higher than the costs of other NICU survivors). Finally, the RCT was conducted in the United States with distinct patient populations, standards of care, and reimbursement models that could limit generalizability. Since the RCT, HRC-monitoring has been adopted in NICUs worldwide, and a recent study from the Netherlands indicates HRC-monitoring improved morbidity associated with sepsis (decreased nSOFA, p = 0.01) with a trend toward improved survival (p = 0.13) (36). This provides some evidence that the RCT results are generalizable to new patient populations and to current standards of care. Further, the trend that primarily drove the cost difference in our analysis, that is increased survival resulting in 1-day longer median length of stay, seems likely to also result in cost-effectiveness in regions with systems of socialized medicine.
Our estimate of the incremental cost to save a life with HRC-monitoring is lower than the average cost of caring for patients in the NICU without HRC-monitoring. It follows that adopting HRC-monitoring may actually improve the cost-effectiveness of NICUs to save lives. The cost-effectiveness of HRC-monitoring for increasing survival among very low birth weight infants is comparable to, or lower than, other interventions used routinely in the neonatal intensive care unit.
The original contributions presented in the study are included in the article/supplementary material, further inquiries can be directed to the corresponding author.
WK was primarily responsible for the conception and first draft of the work. RS, WC, and TO'S were responsible for the acquisition of the data and interpretation of the work. All authors worked together on revising the work critically for important intellectual content, gave final approval of the version to be published, and agree to be accountable for all aspects of the work.
The original randomized controlled trial was supported by the NIH Grant R01-HD48562 to WC, TO'S, and RS and by MPSC (WK). Open access publication fees were provided by MPSC.
We are indebted to our medical and nursing colleagues and the infants and their parents who agreed to take part in the original randomized controlled HRC monitoring trial. The following investigators, in addition to those listed as authors, participated in the original HeRO RCT: Judy L. Aschner, Eduardo Bancalari, John Kattwinkel, Douglas E. Lake, Joseph Randall Moorman, Christina T. Navarrete, Charles Palmer, Jose A. Perez, Peter J. Porcelli, George J. Stukenborg, and Marshall Whit Walker. We appreciate Dr. Dmitry Dukhovny's detailed review of the manuscript when in preparation.
Author WK was employed by and has equity shares in Medical Predictive Science Corporation, Charlottesville, VA.
The remaining authors declare that the research was conducted in the absence of any commercial or financial relationships that could be construed as a potential conflict of interest.
All claims expressed in this article are solely those of the authors and do not necessarily represent those of their affiliated organizations, or those of the publisher, the editors and the reviewers. Any product that may be evaluated in this article, or claim that may be made by its manufacturer, is not guaranteed or endorsed by the publisher.
1. Martin JA, Hamilton BE, Osterman MJK, Driscoll AK. Births: Final Data for 2019. Natl Vital Stat Rep. (2021) 70:1–51. doi: 10.15620/cdc:100472
2. Fanaroff AA, Stoll BJ, Wright LL, Carlo WA, Ehrenkranz RA, Stark AR, et al. Trends in neonatal morbidity and mortality for very low birthweight infants. Am J Obstet Gynecol. (2007) 196:147.e1-8. doi: 10.1016/j.ajog.2006.09.014
3. Lee HC, Bennett MV, Schulman J, Gould JB, Profit J. Estimating length of stay by patient type in the neonatal intensive care unit. Am J Perinatol. (2016) 33:751–7. doi: 10.1055/s-0036-1572433
4. Tawfik DS, Thomas EJ, Vogus TJ, Liu JB, Sharek PJ, Nisbet CC, et al. Safety climate, safety climate strength, and length of stay in the NICU. BMC Health Serv Res. (2019) 19:738. doi: 10.1186/s12913-019-4592-1
5. Gilbert WM, Nesbitt TS, Danielsen B. The cost of prematurity: quantification by gestational age and birth weight. Obstet Gynecol. (2003) 102:488–92. doi: 10.1097/00006250-200309000-00011
6. Beam AL, Fried I, Palmer N, Agniel D, Brat G, Fox K, et al. Estimates of healthcare spending for preterm and low-birthweight infants in a commercially insured population: 2008-2016. J Perinatol. (2020) 40:1091–9. doi: 10.1038/s41372-020-0635-z
7. Phibbs CS, Schmitt SK, Cooper M, Gould JB, Lee HC, Profit J, Lorch SA. Birth hospitalization costs and days of care for mothers and neonates in California, 2009-2011. J Pediatr. (2019) 204:118–25.e14. doi: 10.1016/j.jpeds.2018.08.041
8. Moorman JR, Carlo WA, Kattwinkel J, Schelonka RL, Porcelli PJ, Navarrete CT, et al. Mortality reduction by heart rate characteristic monitoring in very low birth weight neonates: a randomized trial. J Pediatr. (2011) 159:900–6.e1. doi: 10.1016/j.jpeds.2011.06.044
9. Fairchild KD, Schelonka RL, Kaufman DA, Carlo WA, Kattwinkel J, Porcelli PJ, et al. Septicemia mortality reduction in neonates in a heart rate characteristics monitoring trial. Pediatr Res. (2013) 74:570–5. doi: 10.1038/pr.2013.136
10. King WE, Carlo WA, O'Shea TM, Schelonka RL. HRC neurodevelopmental follow-up investigators. Heart rate characteristics monitoring and reduction in mortality or neurodevelopmental impairment in extremely low birthweight infants with sepsis. Early Hum Dev. (2021) 159:105419. doi: 10.1016/j.earlhumdev.2021.105419
11. Sullivan BA, Fairchild KD. Predictive monitoring for sepsis and necrotizing enterocolitis to prevent shock. Semin Fetal Neonatal Med. (2015) 20:255–61. doi: 10.1016/j.siny.2015.03.006
12. Kurul S, Simons SHP, Ramakers CRB, De Rijke YB, Kornelisse RF, Reiss IKM, et al. Association of inflammatory biomarkers with subsequent clinical course in suspected late onset sepsis in preterm neonates. Crit Care. (2021) 25:12. doi: 10.1186/s13054-020-03423-2
13. Fairchild KD, Saucerman JJ, Raynor LL, Sivak JA, Xiao Y, Lake DE, et al. Endotoxin depresses heart rate variability in mice: cytokine and steroid effects. Am J Physiol Regul Integr Comp Physiol. (2009) 297:R1019–27. doi: 10.1152/ajpregu.00132.2009
14. Fairchild KD, Srinivasan V, Moorman JR, Gaykema RP, Goehler LE. Pathogen-induced heart rate changes associated with cholinergic nervous system activation. Am J Physiol Regul Integr Comp Physiol. (2011) 300:R330–9. doi: 10.1152/ajpregu.00487.2010
15. Trang S, Zupancic JAF, Unger S, Kiss A, Bando N, Wong S, et al. Cost-effectiveness of supplemental donor milk versus formula for very low birth weight infants. Pediatrics. (2018) 141:e20170737. doi: 10.1542/peds.2017-0737
16. De Jesus LC, Pappas A, Shankaran S, Kendrick D, Das A, Higgins RD, et al. Eunice Kennedy Shriver National Institute of Child Health and Human Development Neonatal Research Network. Risk factors for post-neonatal intensive care unit discharge mortality among extremely low birth weight infants. J Pediatr. (2012) 161:70–4.e1-2. doi: 10.1016/j.jpeds.2011.12.038
17. Kono Y. Neonatal Research Network of Japan. Neurodevelopmental outcomes of very low birth weight infants in the Neonatal Research Network of Japan: importance of neonatal intensive care unit graduate follow-up. Clin Exp Pediatr. (2021) 64:313–21. doi: 10.3345/cep.2020.01312
18. Kugelman A, Reichman B, Chistyakov I, Boyko V, Levitski O, Lerner-Geva L, et al. Postdischarge infant mortality among very low birth weight infants: a population-based study. Pediatrics. (2007) 120:e788–94. doi: 10.1542/peds.2006-3765
19. Youn Y, Lee SM, Hwang JH, Cho SJ, Kim EK, Kim EA, et al. National registry data from Korean neonatal network: two-year outcomes of korean very low birth weight infants born in 2013-2014. J Korean Med Sci. (2018) 33:e309. doi: 10.3346/jkms.2018.33.e309
20. Gyamfi-Bannerman C, Zupancic J, Sandoval G, Grobman WA, Blackwell SC, Tita A, et al. Cost-effectiveness of antenatal corticosteroid therapy vs. no therapy in women at risk of late preterm delivery: a secondary analysis of a randomized clinical trial. JAMA Pediatrics. (2019) 173:462–468. doi: 10.1001/jamapediatrics.2019.0032
21. Zupancic JA, Hibbs AM, Palermo L, Truog WE, Cnaan A, Black DM, et al. Economic evaluation of inhaled nitric oxide in preterm infants undergoing mechanical ventilation. Pediatrics. (2009) 124:1325–32. doi: 10.1542/peds.2008-3214
22. Mowitz ME, Zupancic JA, Millar D, Kirpalani H, Gaulton JS, Roberts RS, et al. Prospective economic evaluation alongside the non-invasive ventilation trial. J Perinatol. (2017) 37:61–6. doi: 10.1038/jp.2016.159
23. Lee HC, Liu J, Profit J, Hintz SR, Gould JB. Survival without major morbidity among very low birth weight infants in California. Pediatrics. (2020) 146:e20193865. doi: 10.1542/peds.2019-3865
24. Horbar JD, Edwards EM, Greenberg LT, Morrow KA, Soll RF, Buus-Frank ME, et al. Variation in performance of neonatal intensive care units in the United States. JAMA Pediatr. (2017) 171:e164396. doi: 10.1001/jamapediatrics.2016.4396
25. https://fiscaldata.treasury.gov/datasets/treasury-reporting-rates-exchange/treasury-reporting-rates-of-exchange (accessed December 7, 2021).
26. https://data.bls.gov/cgi-bin/surveymost (accessed October 26, 2021).
27. R Core Team. R: A Language Environment for Statistical Computing. R Foundation for Statistical Computing. Vienna (2020). Available online at: https://www.R-project.org/ (accessed May 16, 2022).
28. Husereau D, Drummond M, Petrou S, Carswell C, Moher D, Greenberg D, et al. Consolidated health economic evaluation reporting standards (CHEERS) statement. Value Health. (2013) 16:e1–5. doi: 10.1016/j.jval.2013.02.010
29. Childers CP, Dworsky JQ, Russell MM, Maggard-Gibbons M. Comparison of cost center-specific vs. hospital-wide cost-to-charge ratios for operating room services at various hospital types. JAMA Surg. (2019) 154:557–8. doi: 10.1001/jamasurg.2019.0146
30. Dukhovny D, Lorch SA, Schmidt B, Doyle LW, Kok JH, Roberts RS, et al. Economic evaluation of caffeine for apnea of prematurity. Pediatrics. (2011) 127:e146–55. doi: 10.1542/peds.2010-1014
31. Lorch SA, Cnaan A, Barnhart K. Cost effectiveness of inhaled nitric oxide for the management of persistent pulmonary hypertension of the newborn. Pediatrics. (2004) 114:417. doi: 10.1542/peds.114.2.417
32. Kumar P Committee Committee on Fetus and Newborn American American Academy of Pediatrics. Use of inhaled nitric oxide in preterm infants. Pediatrics. (2014) 133:164–70. doi: 10.1542/peds.2013-3444
33. Petrou S, Bischof M, Bennett C, Elbourne D, Field D, McNally H. Cost-effectiveness of neonatal extracorporeal membrane oxygenation based on 7-year results from the United Kingdom Collaborative ECMO Trial. Pediatrics. (2006) 117:1640–9. doi: 10.1542/peds.2005-1150
34. Keren R, Helfand M, Homer C, McPhillips H, Lieu TA. Projected cost-effectiveness of statewide universal newborn hearing screening. Pediatrics. (2002) 110:855–64. doi: 10.1542/peds.110.5.855
35. Schelonka RL, Carlo WA, Bauer CR, Peralta-Carcelen M, Phillips V, Helderman J, et al. Mortality and neurodevelopmental outcomes in the heart rate characteristics monitoring randomized controlled trial. J Pediatr. (2020) 219:48–53. doi: 10.1016/j.jpeds.2019.12.066
Keywords: heart rate characteristics (HRC), cost-effectiveness analysis (CEA), very low birth weight (VLBW), neonatal intensive care unit (NICU), incremental cost-effectiveness ratio (ICER), randomized controlled trial (RCT), artificial intelligence (AI), newborn infant
Citation: King WE, Carlo WA, O'Shea TM and Schelonka RL (2022) Cost-effectiveness analysis of heart rate characteristics monitoring to improve survival for very low birth weight infants. Front. Health Serv. 2:960945. doi: 10.3389/frhs.2022.960945
Received: 16 June 2022; Accepted: 16 August 2022;
Published: 07 September 2022.
Edited by:
Asif Raza Khowaja, Brock University, CanadaReviewed by:
Zachary Vesoulis, Washington University in St. Louis, United StatesCopyright © 2022 King, Carlo, O'Shea and Schelonka. This is an open-access article distributed under the terms of the Creative Commons Attribution License (CC BY). The use, distribution or reproduction in other forums is permitted, provided the original author(s) and the copyright owner(s) are credited and that the original publication in this journal is cited, in accordance with accepted academic practice. No use, distribution or reproduction is permitted which does not comply with these terms.
*Correspondence: William E. King, d2tpbmdAaGVyb3Njb3JlLmNvbQ==
Disclaimer: All claims expressed in this article are solely those of the authors and do not necessarily represent those of their affiliated organizations, or those of the publisher, the editors and the reviewers. Any product that may be evaluated in this article or claim that may be made by its manufacturer is not guaranteed or endorsed by the publisher.
Research integrity at Frontiers
Learn more about the work of our research integrity team to safeguard the quality of each article we publish.