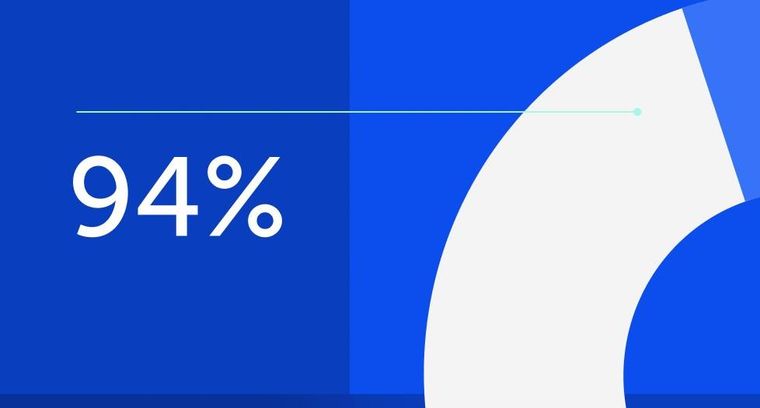
94% of researchers rate our articles as excellent or good
Learn more about the work of our research integrity team to safeguard the quality of each article we publish.
Find out more
ORIGINAL RESEARCH article
Front. Geochem., 18 March 2024
Sec. Mineral Geochemistry
Volume 2 - 2024 | https://doi.org/10.3389/fgeoc.2024.1351631
This article is part of the Research TopicUnderstanding Natural H2 Systems- their Exploration and StimulationView all 5 articles
The energy transition calls for natural hydrogen exploration, with most occurrences discovered either inadvertently or, more recently, at the location of potentially diffusive circles observed from a change of vegetation cover at the surface. However, some notable hydrogen occurrences are not directly associated with the presence of diffusive circles like the Bourakebougou field in Mali. Thus, the objective of this work was to highlight geological areas that have some potential to find natural hydrogen in Quebec, a Canadian province where no diffusive circles have yet been documented but which is rich in potential source rocks and where no exploration for natural hydrogen has been undertaken so far. A review of the different geological regions of Quebec was undertaken to highlight the relevant characteristics and geographical distribution of geological assemblages that may produce or have produced natural hydrogen, in particular, iron-rich rocks but also uranium-rich rocks, supramature shales and zones where significant structural discontinuities are documented or suspected, which may act as conduits for the migration of fluids of mantle origin. In addition to regional and local geological data, an inventory of available geochemical data is also carried out to identify potential tracers or proxies to facilitate subsequent exploration efforts. A rating was then proposed based on the quality of the potential source rocks, which also considers the presence of reservoir rocks and the proximity to end-users. This analysis allowed rating areas of interest for which fieldwork can be considered, thus minimizing the exploratory risks and investments required to develop this resource. The size of the study area (over 1.5 million km2), the diversity of its geological environments (from metamorphic cratons to sedimentary basins) and their wide age range (from Archean to Paleozoic) make Quebec a promising territory for natural hydrogen exploration and to test the systematic rating method proposed here.
When produced from a carbon-neutral source, hydrogen can contribute to the energy transition by replacing the use of fossil fuels, particularly in the industrial, heavy road and marine transport sectors. Worldwide, hydrogen demand amounted to about 94 million tons (Mt) in 2021, but over 99% of the hydrogen manufactured that year came from fossil fuels, resulting in CO2 emissions of over 900 Mt (IEA, 2022). While the share of green hydrogen is set to increase, the technologies involved remain costly and uncompetitive with hydrogen produced by methane steam reforming or from coal. Natural hydrogen, i.e., dihydrogen (H2) produced by natural geological processes in the subsurface, could complement green hydrogen while being produced at competitive costs with grey or black hydrogen, particularly in cases where this resource proves renewable, a possibility that remains to be evaluated (Lapi et al., 2022).
Hydrogen is chemically reactive and ubiquitous in many natural environments. Natural hydrogen can be produced in a wide range of geological settings, by various geological processes, at different temperatures, depths and rates (Zgonnik, 2020; Milkov, 2022). These characteristics provide the incentive to explore for natural hydrogen in locations not previously deemed promising for energy sources. However, the development of a one-size-fits-all exploration strategy remains an elusive goal at present, and most initial discoveries of natural hydrogen remain either fortuitous (Prinzhofer et al., 2018), or guided by the observation at the ground surface of diffusive circles, also known as fairy circles, but that have yet to be linked to subsurface accumulations (McMahon et al., 2023).
In the Canadian province of Quebec, hydroelectricity covers a large part of the energy needs but 53% of these needs are still met by imported fossil fuels (Whitmore and Pineau, 2023), leaving room for natural hydrogen to support the province’s energy transition efforts. Quebec covers an area of over 1.5 million km2 and includes diversified geological assemblages. This diversity is encouraging in terms of the possible presence of natural hydrogen, but likely to slow down and dilute exploration efforts, especially as no diffusive circles have yet been documented in Quebec, and certain circular topographic depressions developed in the permafrost (thermokarsts) may obscure the search for diffusive circles. Nevertheless, some preliminary indications have been documented, notably the presence of dissolved hydrogen gas in the water of some mines near the province of Ontario, where this phenomenon has historically received more attention (Fritz et al., 1987). Based on these statements, we hypothesized that natural hydrogen could play an important role in Quebec’s green economy and we completed a very first assessment of the province’s exploration opportunities.
The objective of this work was to identify geological settings in which natural hydrogen can potentially be found in Quebec. This paper addresses the question “where to start looking?” in two steps. First, an inventory of the most prospective environments is made, based on publicly available data (lithological assemblages and geochemical databases) and the most representative hydrogen production mechanisms. Second, rating of these areas is proposed to prioritize possible future exploration efforts, trying to minimize the exploration risks and investments required to develop this carbon-free resource.
The most representative geological assemblages in Quebec were first reviewed to identify favourable settings for natural hydrogen production. In addition to their relevance for Quebec’s geology, the mechanisms considered here are those that are relatively better understood and documented, and for which anticipated hydrogen production is comparatively more volumetrically significant.
The inventory is mainly based on geological maps, in particular via the Système d’information géominière (SIGEOM, 2023). Cartographic data was accompanied by a literature review undertaken to identify previously documented hydrogen occurrences, if any, or more broadly to identify indicators that support the potential of a given area. This review is not intended to be exhaustive, and focuses mainly on the most representative and best-documented areas. Targets are identified here as “plays” in the simplest sense, i.e., having the possible presence of a source rock and possibly a reservoir rock, while the other items defining a hydrogen system (a trap, a migration conduit, the chronology and rates of production/migration/accumulation) were not considered because of the lack of sufficient information.
Geological maps are obviously useful for locating potential source rocks outcropping at the surface. In the case of sedimentary basins, which cover most of the Canadian Shield in southern Quebec, existing deep drilling and geochemical data are crucial sources of information and were included in this assessment.
In addition to the analyses of gases in the groundwater of certain mines mentioned in the introduction, geochemical data were available for Quebec southern sedimentary basins. Geochemical datasets sometimes contain analyses of hydrogen gas concentration, as in the case of subsurface data collected by the oil and gas industry and available in the Système d’Information Géoscientifique Pétrolier et Gazier du Québec (SIGPEG, 2023), but more often they only contain indirect indicators such as shallow hydrogeochemical parameters that may be used as proxies (salinity, methane (CH4), and/or radon (222Rn) concentrations dissolved in groundwater of shallow aquifers). These are mainly available through the Projets d’acquisition de connaissances sur les eaux souterraines (PACES, 2023) and the Réseau de suivi des eaux souterraines du Québec (RSESQ, 2023), but also in other publications such as Bordeleau et al. (2018; 2019), Moritz et al. (2015), Peel (2014), Pinti et al. (2014) and Raynauld et al. (2014). In addition to these near-surface data, Drolet et al. (2013; 2014) present radon indoor emission potential maps that could also be used as an indirect indicator (data not shown in the present paper).
In particular, oil and gas data contain reports on the analysis of gases sampled during drilling or during drill stem tests (DST), which enable us to assess the nature of the gases present at depth (down to about 4,000 m in the case of Quebec). Data must be examined with caution, as it can be associated with the following biases: 1) an under-representation of hydrogen analyses which, unlike major gases, is not systematically analyzed (Zgonnik, 2020), 2) a geographical distribution that does not meet exploration objectives for natural hydrogen, and 3) the possibility that the hydrogen analyzed is an artifact caused by microbial activity or corrosion of steel casings (Guelard et al., 2017). This dataset may therefore contain “false negatives” and mask the potential for hydrogen on a more regional scale, or conversely may cause a magnifying glass effect on “false positives” that are in fact of anthropogenic origin.
In another perspective, the use of indirect hydrogeochemical indicators available in the near-surface dataset is centered in targeting regions where deep fluids (often saline), associated with dissolved gases (such as CH4, CO2 and potentially hydrogen), naturally ascend towards the surface through permeable fault zones (e.g., Bordeleau et al., 2019). It is postulated that the St. Lawrence Lowlands, in southern Quebec, could have the capacity to trap potential hydrogen produced at depth, the same way that it has preserved hydrocarbons (e.g., Moritz et al., 2015; Bordeleau et al., 2019), since this area exhibits specific faults demonstrating minimal matrix permeability and increased fracture porosity (Ladevèze et al., 2019). Beyond looking for high salinity and CH4, the surveillance of radon concentrations (together with CO2 and helium) stands as a highly effective method for probing the fluid dynamic processes ongoing within the Earth’s crust and detecting fault activity (Baubron et al., 2002; Ignatenko et al., 2016; Lefeuvre et al., 2022). At high concentrations, it also indicates subsurface lithologies enriched in uranium (238U) and might suggest active water radiolysis (Lefeuvre et al., 2021).
Thus, the utilisation of these pathfinders is suitable in a context with extensive, publicly available geochemical data, but where existing analyses of hydrogen are remarkably scarce, as it has not been the focus of exploratory researches up to now. An inventory of the available hydrogeochemical data has been carried out from the sources mentioned here-in, and correlated with mapped geological faults available in the SIGEOM (2023) database. The shallow hydrogeochemical dataset contain information on well depth and geology, along with major ion concentrations (such as calcium, Ca2+; sodium, Na+; potassium, K+; magnesium, Mg2+; chloride, Cl-; bicarbonate, HCO3-; nitrate, NO3-; sulfate, SO42-) and physicochemical parameters (including temperature, dissolved oxygen, pH, electrical conductivity and redox potential), for almost all samples. The water salinity is characterized by dissolved salts, consisting of dissociated cations and anions, and it is usually expressed in milligrams per liter (mg/L) of total dissolved solids (TDS). The calculation is expressed by the following equation Eq. 1), considering the major ions here-in mentioned, excluding NO3-, and including the carbonate (CO3-) and bicarbonate (HCO3-) ions calculated from the measured total alkalinity, pH and temperature; and silicon dioxide (SiO2) calculated from the measured silicon (Si):
Groundwater can be classified by TDS as: freshwater (<1,000 mg/L), brackish (>1,000 mg/L and <10,000 mg/L), and saline water (>10,000 mg/L; Freeze and Cherry, 1979). The high concentrations must be examined with caution, because beyond an upward migration of deep saline fluids, they are associated with possible biases: 1) presence of salt derived from the clays of the Champlain Sea (Cloutier, 2004); 2) intrusion of seawater into aquifers along the coast and 3) use of de-icing salt on roads. Therefore, the presence of wells containing water with high salinity, located alongside a fault zone, can be a good indicator of its permeability. However, those located along the coast or a major road could potentially create a false indicator.
Finally, the indicators are also compared with the different hydrofacies, defined by the dominant cation and anion in water samples. While characterizing hydrofacies, if no cation or anion constitutes at least 40% of the total, it is categorized as “mixed” (e.g., Mixed-HCO3 denotes no dominant cation but bicarbonate, HCO3-, as the dominant anion). If Ca and Mg individually fall below 40% but their sum exceeds 60%, and Na+K is below 40%, the dominant cation is associated with water alkalinity, labeled as “alk-(dominant anion)" due to carbonate dissolution (Ca and Mg being primary cations). When two cations or anions each surpass 40% (e.g., Ca = 44% and Na = 42%), both dominant ions are explicitly named [e.g., Ca-Na-(dominant anion)].
The very high reactivity of hydrogen makes it unlikely that geophysical methods can detect emanations directly from the surface. On the other hand, geophysical and satellite/aerial imagery data can help determine or delineate the presence of hydrogen source rocks. As such, a preliminary inventory of relevant geophysical data and images has been carried out in order to provide a complete picture relying on all datasets available in Quebec. Geophysical data inventory mainly relied on public databases available in SIGEOM (2023) and SIGPEG (2023) for the years 1970–2023, while images were obtained from the open database Québec (2023). Only the geographical distribution and availability of these datasets have been inventoried, and the data themselves have not been examined in detail. For this reason, that inventory is not taken into account in the rating of the different regions, but is presented as an indication of the data density.
The inventory revealed several regions that were shown to have potential because 1) they contain rock types or geological environments conducive to the formation of natural hydrogen, or 2) they contain direct indicators of the presence of hydrogen or 3) they contain indirect indicators that could support the possible presence of natural hydrogen. However, not all these regions are equal in their potential, and their relative ranking is desirable in order to prioritize subsequent exploration efforts. The rating system put forward takes into account three fundamental geological criteria, to which a fourth criterion is added to reflect the feasibility of integrating the resource into the regional economic fabric (Table 1). This analysis is qualitative, as it is not yet possible to objectively quantify the geological criteria considered, in the absence of indicators such as gas fluxes in the ground, volumes of gas in place in a reservoir, or volumes of reactive rocks.
TABLE 1. Classification used to rate the attributes of the various sectors of interest for natural hydrogen in Quebec.
The first criterion, the value of geological indicators and attributes of potential source rocks, reflects the quality of the indicators itself documented in a given area, which suggest that hydrogen can be produced, or has been produced, by the source rock. This criterion receives a mark of 1–3 depending on the amount and quality of local evidence that is available to support the potential source rock.
The criterion on the existence of known analogues elsewhere in the world for the source rock is introduced to nuance the score attributed to local geological evidence. The aim is to avoid undervaluing an area (“false negative” effect) if it has little geological data at the moment. This criterion receives a mark of 1–3 depending on the current knowledge on the potential of known analogues.
The last geological criterion considers the nature of the best possible hydrogen reservoir in the area of interest. If future work confirms the presence of natural hydrogen in one of the areas of interest, the setting in which hydrogen can potentially accumulate could influence the decision to pursue further exploration. With this in mind, this criterion reflects the geological context and the technological challenges associated with finding and exploiting a possible accumulation of natural hydrogen. This criterion receives a mark of 1–3 depending on the current level of understanding on possible reservoir types in the area of interest.
Each of these three fundamental geological criteria receives a weighting factor that modifies its score (Table 1). Geologic evidence documented in the area of interest has a preponderant weight (3) over inferences based on analogues (2). The lowest weight (1) is given to the reservoir criterion, reflecting the fact that this study focusses on potential source rocks, not economic viability. For a given area of interest, the value of each criterion is thus multiplied by its corresponding weighting factor, which gives a possible range between 6 and 18. This range is then converted into percentages (33%–100%) for clarity. The possibility of obtaining a null value is not introduced in this rating system, as the exercise concerns areas of interest that have already been pre-selected for their potential in hydrogen source rocks. Conversely, a maximum score does not mean that there is a 100% chance of hydrogen being produced, as the score is relative to the other sectors. A hydrogen reservoir discovered in a given area would not be evaluated on this scale and would be ranked out of the category.
The rating of the areas of interest is completed by a fourth criterion: the proximity of the area to end-users. This criterion is independent of the area’s geological attributes and is introduced here to provide information on the resource’s development potential in the event of a discovery. A separate grading system (A to C) is applied to this criterion to clearly distinguish it from the geological criteria. This separate grading ensures that an area with favourable geological criteria is not unduly disadvantaged, given that a discovery in a remote sector may in turn attract new end-users and lead to the development of new infrastructures, while the development of a discovery in an urbanized area may be conversely hampered by social acceptability issues and conflict uses.
At the surface, more than 90% of the province of Quebec consists of Precambrian rocks of the Canadian Shield (Figure 1), which is dominated by three main geological provinces: Superior (4.3–2.5 Ga), Churchill (2.9–1.1 Ga) and Grenville (2.7–0.6 Ga). The area south of the St. Lawrence River is occupied mainly by Paleozoic sedimentary basins (570–245 Ma) developed unconformably on the craton.
FIGURE 1. Major geological provinces in Quebec. Map background: SIGEOM (2023).
The Superior Province forms the heart of the Canadian Shield and the North American continent (Card, 1990; Card and Poulsen, 1998). Representing the largest terrestrial Archean craton, it covers an area of over 740,000 km2 in Quebec. World-renowned for its numerous gold, copper, zinc, nickel and silver deposits, it has more recently been the site of major diamond discoveries in kimberlites. Four types of sub-provinces are recognized, with metasedimentary, metamorphic, volcano-plutonic and plutonic facies. The best-known sub-province is the Abitibi, the world’s most extensive Archean volcanosedimentary belt (Chartrand, 1994; Hocq, 1994; Hocq and Verpaelst, 1994).
The Churchill Province covers an area of some 200,000 km2 in Quebec and surrounds the Superior craton (James et al., 1996; Wardle et al., 2002; Lafrance et al., 2018). This province includes fold and thrust belts composed of weakly metamorphosed supracrustal rocks and a hinterland of strongly remobilized Paleoproterozoic Archean crust and magmatic arcs.
The Grenville Province of Quebec covers a vast territory of nearly 495,000 km2. The province is renowned for its iron and ilmenite mines, as well as for its industrial mineral potential. It represents the imprint of the last orogenic event to have shaped the Canadian Shield (Rivers and Corrigan, 2000; Rivers et al., 2012), and is the deep root of an ancient mountain range comparable to today’s Himalayas (Windley, 1986). This province also forms the basement of the sedimentary basins of southern Quebec.
The Paleozoic sedimentary basins of southern Quebec cover an area of over 100,000 km2 on land and extend eastwards into the Gulf of St. Lawrence (St. Julien and Hubert, 1975; Williams, 1979). They are subdivided into three main groups: 1) a slightly deformed autochthonous platform (St. Lawrence Platform) punctuated in its western part by Mesozoic intrusions, 2) an Appalachian domain corresponding to several orogenic pulsations and including an ophiolitic belt accreted during the Taconian orogeny and 3) a post-orogenic successor basin mainly developed offshore in the Gulf of St. Lawrence. Oil and gas exploration in these basins has led to the discovery of conventional and unconventional reservoirs on land (Lavoie et al., 2009; Chen et al., 2014; Chen et al., 2016).
Considering the geological contexts and lithological assemblages most representative of Quebec, four main mechanisms likely to produce natural hydrogen were considered for this study. There is an abundant literature on the mechanisms capable of producing natural hydrogen and the reader is invited to refer to the very detailed inventory presented in Zgonnik (2020) and completed by Milkov (2022). Only the relevant references that are not already listed in these two papers are mentioned here. The mechanisms considered in this study consist of the following:
- Mantle and core outgassing (Melton and Giardini, 1981; Schrauder and Navon, 1994; Matveev et al., 1997; Kagi et al., 2000; Ko et al., 2022; Weiss et al., 2022).
- The radiolysis of water in the presence of weakly radioactive rocks (LaVerne and Tandon, 2003; Lefticariu et al., 2010; Essehli et al., 2011; Kumagai et al., 2013; Costagliola et al., 2017).
- Hydrothermal alteration (Rüpke and Hasenclever, 2017; Geymond et al., 2022).
- Decomposition of organic matter (Mahlstedt et al., 2022; Boreham et al., 2023).
Other mechanisms are likely to produce hydrogen in some of Quebec’s geological environments, but these mechanisms are not well documented or are likely to produce comparatively smaller volumes of hydrogen, such as microbial activity or rock friction in fault zones.
Seventeen areas of interest have been identified in the Quebec’s part of the Canadian Shield, which were grouped into four categories according to their affinities. These areas have been selected and delineated in order to provide a representative overview of the nature and extent of the rocks that may be sources of hydrogen, but the potential for natural hydrogen in the Shield is not limited to these areas.
Several gold mines have been operated or are still active in the Superior Province, in particular a vast mining camp in the Abitibi sub-province along the Cadillac Fault (play A1, Figure 2), as well as the Éléonore (A2) and Casa Berardi (A3) mines and a former mining camp in the Chibougamau region (A4). Gold mineralization occurs in volcano-sedimentary environments rich in mafic and, more rarely, ultramafic rocks, and is also associated with banded iron formations in the case of the Éléonore mine. The Abitibi sub-province is of particular interest here because of the associated direct and possibly indirect indicators of hydrogen.
FIGURE 2. Potential hydrogen source rocks of the Canadian Shield in Quebec. Map background: SIGEOM (2023).
The Abitibi sub-province corresponds to a vast Neoarchean greenstone belt that straddles the Ontario-Quebec border. It consists of successions of folded volcanic and sedimentary rocks and intermediate domes of intrusive rocks. The komatiite successions within some of the volcanic assemblages host magmatic sulfide deposits. In addition to the presence of ultramafic and mafic rocks in these areas, soluble combustible gases (hydrocarbons and hydrogen) have been documented in the groundwater of some gold mines in Ontario and Quebec (Fritz et al., 1987). Vegetation anomalies, generally referred to as “forest rings”, have also been documented locally in the black spruce forests of the Abitibi region, mainly in Ontario but also in western Quebec. In aerial view, these forest rings may evoke the diffusive circles (or “fairy circles”) corresponding to hydrogen emanations in many localities around the world, as already proposed by Malvoisin and Brunet (2023). More detailed analysis, however, reveals that forest rings have different, even opposite, geometric characteristics to diffusive circles, including less dense vegetation depression of the rock surface limited to the outer ring rather than centred on the heart of the structure (Veillette and Giroux, 1999; Giroux et al., 2001; Hamilton et al., 2004; Hamilton, 2005; Hamilton and Hattori, 2008; Brauneder, 2012; Brauneder et al., 2016). Data on hydrogen in Abitibi gold mines and on gases associated with forest rings are presented in greater detail in Section 3.4.1.
Four main kimberlite fields have been documented in Quebec. The Torngat field (play B1, Figure 2) is located on the boundary of the Churchill and Nain provinces, while the other three are in the Superior Province: the Otish Mountains kimberlite dykes (B2), incidentally located about 500 m from a uranium deposit, the Desmaraisville kimberlite field (B3) in the north-central Abitibi sub-province and the Témiscamingue kimberlite field (B4), which is located mainly in Ontario but also extends into Quebec and is centred on the Témiscamingue rift zone.
Kimberlites are volatile-rich ultramafic rocks derived from the mantle. There is abundant evidence in the worldwide literature on the presence of molecular hydrogen and water as fluid inclusions in diamonds associated with kimberlites. In addition, they can also be associated with crustal fractures that can act as conduits for deep fluid migration. These rocks are also likely to be serpentinized. While no data are available to document the presence of natural hydrogen associated with kimberlites in Quebec unlike in the case of the Abitibi gold mines (see above), low-temperature serpentinization has been demonstrated in the Ontario part of the Témiscamingue kimberlite field by Sader et al. (2007).
Five alkaline intrusions are examined here, which are emplaced in the Churchill and Grenville provinces: the Lac Brisson Pluton (play C1, Figure 2) at the Quebec-Labrador border (Bélanger, 1984; Zajac et al., 1984; Currie, 1985; Miller, 1985; Pillet, 1985; Nassif, 1993; Charette et al., 2019), which includes zones of hematite breccia at the intrusion margin, the Misery Syenite (C2) (David et al., 2012; Petrella, 2012; Petrella et al., 2014; Lafrance et al., 2018), the Juillet Syenite (C3) (Hammouche et al., 2012; Charette et al., 2019), which includes gabbroic units, enclaves of banded iron formations from the host rock, and lamprophyres that may represent a mafic rock associated with the syenite (Taner, 1992), the Crevier Alkaline Intrusion (C4) (Bergeron, 1980; Groulier et al., 2014; El Bourki and Moukhsil, 2021; Moukhsil and El Bourki, 2021) and the Kipawa Syenitic Suite (C5) (van Breemen and Currie, 2004; Saucier et al., 2013; Wavrant et al., 2017; Matte et al., 2022).
Some peralkaline granites are known to contain hydrogen in fluid inclusions. This is particularly true of the Lac Brisson Pluton (“Strange Lake” in Labrador), where hydrogen is documented in fluid inclusions at concentrations ranging from 1.1% to 35% (Salvi and Williams-Jones, 1997). Truche et al. (2021) have studied these occurrences at Lac Brisson and identify hydrothermal alteration of arfvedsonite, a ferrous-iron-rich amphibole, as the mechanism responsible for the hydrogen detected. In addition to arfvedsonite alteration, other iron-rich rocks may also be present locally, as in the case of Juillet Syenite. Although in the case of Lac Brisson the hydrogen occurrences documented to date are limited to fluid inclusions, free hydrogen gas is documented in other peralkaline granites, notably in the case of the Ilimaussaq massif in Greenland and in the Khibiny massif in Russia, where hydrogen is also present in natural fracture networks (up to 16.7% H2, Zgonnik, 2020).
Four sedimentary basins within the Canadian Shield are examined here. Three are located in the Superior Province: the Richmond Gulf Graben (play D1, Figure 2), the Hudson Basin (D2) and the contiguous Mistassini-Otish basins (D3). A fourth and larger basin is the Labrador Trough (D4) in the Churchill Province.
The Richmond Gulf Graben (Chandler, 1988; Labbé and Lacoste, 2004) and the Quebec part of the Hudson Basin (Remick et al., 1963; Sanford and Norris, 1975; Hamblin, 2008; Cherns and Wheeley, 2009) are considered here mainly because of the cover they can provide for possible hydrogen accumulations. They rest in unconformity or faulted contact on an Archean basement mainly composed of granitoids possibly rich in radiogenic elements. The sedimentary sequence itself locally includes volcanics and mafic dykes, and the normal or shear faults that affect these basins may act as conduits for deep fluid circulation.
The Mistassini Basin (Chown, 1984; Héroux et al., 2004) includes iron formations and uranium showings. Up to 2.5 km thick, the basin is composed of sandstones and carbonates that may have developed reservoir properties, as well as mudstones that may serve as a seal. A meteorite impact crater (Evangelastos et al., 2009) may also have contributed to the development of fracture networks favouring fluid migration. Hydrogen has been documented in fluid inclusions in the Lac Mistassini region, at concentrations ranging from 6% to 92%, associated with nitrogen and more rarely methane (Héroux et al., 2004). The adjacent Otish Basin (Fryer, 1972; Chown, 1984; Faure, 2010) comprises olivine-bearing mafic dykes and uranium showings. The kimberlite of the Otish Mountains, located on the northern flank of the basin, may be an indicator of the presence of crustal fractures allowing the circulation of deep fluids. Finally, the Labrador Trough (Frarey and Duffell, 1964; Dimroth, 1978; Rohon et al., 1993; Clark, 1994; Wardle et al., 2002; Clark and Wares, 2004) comprises banded iron formations, MORB-type mafic rocks and ultramafic rocks (sills, carbonatite) locally overlain by mudstones and turbidites, which may provide a seal for possible hydrogen accumulations. Sandstones and dolostones, possibly with reservoir quality, are also present.
Ten areas of interest have been identified in the sedimentary basins of southern Quebec, which were grouped into five categories according to their affinities. As for the Canadian Shield, these areas have been selected and delineated in order to provide a representative overview of the nature and extent of the rocks that may be sources of hydrogen, but the potential for natural hydrogen in southern Quebec is not necessarily limited to these areas. Especially, two large regions do not include areas of interest at this time: Anticosti Island and the Gulf of St. Lawrence. The available data do not currently allow the identification of source rocks for hydrogen at reasonable depths in these regions. However, the lack of evidence should not definitively close the door to research in these regions. In the case of Anticosti, for example, forest rings documented in the central part of the island (Dubois, 1993) bear features comparable to those observed in Abitibi (see Section 3.2.1) and deserve further investigation.
The ophiolite complexes present in southern Quebec and Newfoundland belong to the Dunnage Zone, an Ordovician terrane accreted onto the Humber Zone. These ophiolite fragments are correlated with each other, although the accretion is diachronic. The final obduction onto Humber Zone rocks took place at the beginning of the Taconian orogeny between 475 and 457 Ma (De Souza et al., 2011). In Quebec, these ophiolite complexes outcrop discontinuously, mainly in the Eastern Townships (play E1, Figure 3) and in the Monts Chics-Chocs (E2) in Gaspe Peninsula, and these two regions are retained here because of their geographical extent.
FIGURE 3. Potential hydrogen source rocks of the southern Quebec basins. Map background: SIGEOM (2023).
In southwestern Quebec and the Gaspe Peninsula, the ophiolites observed at outcrops are extensively serpentinized in many areas, so that the hydrogen produced by serpentinization had time to migrate out of the ophiolites. However, several areas also feature weakly serpentinized ophiolites, where a hydrogen production process could still be active. This is particularly true of the Mont Olivine region east of Mont Albert in Gaspe (Beaudin, 1980) and of the Lac Canard area south of Thetford Mines (Pagé et al., 2008), and the controls on serpentinization should be further studied as a predictive tool to better constrain the geographical extent of the prospective areas. Furthermore, the composition of Thetford Mines olivines (Fo90 to Fo93; Pagé et al., 2008) is comparable to that of ophiolite complexes where natural hydrogen emanations have been interpreted as the result of low-temperature serpentinization processes that are still active. This is notably the case for the correlative Bay of Islands complex in Newfoundland (Fo89 to Fo92; Batanova et al., 1998), where Szponar et al. (2013) sampled strongly alkaline and highly reducing water sources containing up to 1.18 mg/L of dissolved hydrogen, and the Zambales ophiolite in the Philippines (Fo88 to Fo93; Payot et al., 2013), where natural hydrogen emanations of the order of 42% H2 have been documented (Abrajano et al., 1988). Close to Quebec ophiolite complexes, potential reservoirs and traps are to be sought in nearby sedimentary rocks, such as the Saint-Daniel Melange and the Ruisseau Isabelle Melange, which contain both reservoir and cap rocks.
No hydrogen analyses for Quebec ophiolite complexes were identified in the literature consulted. However, two studies provide indirect clues to this potential. Normand and Williams-Jones (2010) document the presence of gaseous hydrocarbons in rodingite fluid inclusions at the Jeffrey mine in southwestern Quebec. These authors conclude to an abiotic origin for the hydrocarbons, related to the serpentinization process in an environment with high hydrogen fugacity, and they note that small quantities of hydrogen could also be present in the fluid inclusions, but that the minor gas species have not been determined. In another study, Morency (1989) proposes an industrial process for deserpentinization through heating and shows laboratory results in which samples from ophiolites in southeastern Quebec and the Gaspe Peninsula could produce up to 22% hydrogen by this process.
The Monteregian Hills (Clark, 1955; Clark, 1977; Philpotts, 1976; Valiquette and Pouliot, 1977; Globensky, 1985; Gold et al., 1986; Eby, 1987), of Cretaceous age (Eby, 1984), form a set of landforms roughly aligned along a WNW-ESE axis in southwestern Quebec, associated with numerous dykes and sills (Figure 3). Based on gravity data, Feininger and Goodacre (1995) interpret the presence of thick magmatic laccolites emplaced at the interface between the Grenvillian basement and the sedimentary cover, beneath the Monteregian Hills, and they estimate the total volume of magma emplaced at around 200 km3, far greater than the volume corresponding to the landforms alone. The composition of the Monteregian Hills can be simplified into three groups, more or less successive in time, comprised of ultramafic rocks (mainly peridotites and pyroxenites), mafic rocks (mainly gabbros, essexites and gabbroic anorthosites) and finally intermediate rocks (mainly syenites, foidic syenites, nordmarkites, pulaskites and diorites). Some breccias are diamondiferous (Bédard, 1994; Bédard and LeCheminant, 1996). Contemporaneous dykes and sills are mainly alkaline lamprophyres, grouped into three main types: camptonites, monchiquites and alnöites. The alignment of these intrusions has long led to believe that they corresponded to a hot spot, but this theory is now debated and synthesis work (Bédard, 1985; McHone et al., 1987; Matton and Jébrak, 2009) points instead to a mantle plume of shallower origin, whose upward movement was favoured by deep crustal fractures and decompression melting (Courtillot et al., 2003). In fact, the Monteregian intrusions were emplaced through a Grenvillian basement already structured by a set of normal faults corresponding to the opening of the Iapetus Ocean and the Ottawa-Bonnechère Graben (Bédard, 1985; Faure et al., 1996; Allen et al., 2010).
Three processes favourable to the production of natural hydrogen can be associated with the presence of these intrusions. Serpentinization of ultramafic and mafic rocks is considered here to be the dominant process. According to the incomplete data available, olivines in Monteregian intrusions are predominantly magnesian (Fo66 to Fo81; Philpotts, 1976; Valiquette and Pouliot, 1977), but their iron content is higher than that of olivines in Quebec ophiolites (Fo90 to Fo93; see above). This iron content increases even in the periphery of intrusions (Fo44; Philpotts, 1976), presumably by assimilation of crustal material during magma ascent. Evidence of serpentinization is documented at outcrops in one case. In addition to this dominant process, some intrusions and associated dykes and sills are also radioactive (Globensky, 1985) and, when they encounter hydrocarbon source rocks (here, the Utica Shale), contact metamorphism at the time of the emplacement can also induce cracking of hydrocarbon chains and hydrogen production from organic matter.
In addition, two types of seals are present in the region: the mafic sills themselves, by analogy with the Bourakebougou hydrogen reservoir in Mali (Prinzhofer et al., 2018), and the sedimentary cover formed by the Utica Shale and Lorraine Group shales, which can exceed 2,000 m in thickness in the region. Potential reservoirs (sandstones and carbonates) are stratigraphically located between this shale cover and the potential hydrogen source formed by the laccolites emplaced between the platform and the Grenvillian basement.
Several lineaments corresponding to crustal fracture corridors have been identified in southern Quebec on the basis of geophysical data, mainly gravimetric (Thériault et al., 2004; 2005; Pinet et al., 2008; Lyal Harris, personal communication, 2022). The five crustal fracture corridors considered here (plays G1 to G5, Figure 3) were selected because, in addition to geophysical data, their presence is also supported by surface evidence of fluid flow in the form of mineralization, hydrothermal dolomitization and/or lamprophyre dykes. Exploration wells in the G2 corridor have also revealed the presence of partially serpentinized ultramafic rock dykes. Sandstones and carbonates, which may locally have reservoir properties, are present within these structural corridors (Morin and Laliberté, 2002a; 2002b) and are overlain by shale or siltstone seals up to several hundred metres thick. G2 to G4 are crustal fractures with surface expressions (Thériault et al., 2004; Thériault et al., 2005). G1 and G5 are the projection to surface of upper mantle discontinuities identified by Lyal Harris (personal communication, 2022) and G5 can be further extended to the southwest beyond its trace plotted on Figure 3 (Pinet et al., 2008).
Crustal fracture corridors are major faults that are deeply rooted in the basement and may even cut through the crust into the mantle. While these deformation corridors are not hydrogen source rocks, they can act as conduits for the migration of deep-seated hydrogen-bearing fluids of crustal or even mantle origin. Alternatively, these same corridors may have allowed the intrusion of ultramafic rocks that can be serpentinized and thus produce hydrogen in situ.
Several hydrocarbon source rocks have been identified in the basins of southern Quebec. Most of them are either too immature (in areas that have been well studied) or very poorly documented (in areas where they could be sufficiently buried to reach a high level of thermal maturity). However, the Utica Shale in the St. Lawrence Lowlands (Thériault, 2012) shows locally suitable characteristics.
The Utica Shale outcrops at the surface along the St. Lawrence River and extends at depth into the heart of the basin beneath the Lorraine Group flysch. Based on a few deep boreholes drilled in the region and the interpretation of available seismic data (Laliberté and Trépanier, 1982; SOQUIP, 1984; Castonguay et al., 2006; Séjourné et al., 2013), it appears that the Utica Shale extends southeastward beneath the Appalachians (Figure 3) to a maximum depth of at least 6,600 m. Beyond this eastern limit, the data no longer allow us to follow this unit. The thermal maturity map of the Utica Shale (Bertrand and Lavoie, 2006) indicates that the hydrocarbon-bearing source rock has reached the anchizone and epizone stages in this area, which are favourable to the release of hydrogen. In its southern part, the shale is also crosscut locally by intrusives (Monteregian intrusions, see above) which, through contact metamorphism, may have accelerated the thermal maturation of organic matter when magma was emplaced (Héroux and Bertrand, 1991). Potential hydrogen reservoir rocks in the area of interest are the carbonates and sandstones of the parautochthonous domain, imbricated above the autochthonous Utica Shale.
Based on regional correlations and geophysical data, the Grenvillian basement beneath the sedimentary cover south of Montreal (Figure 3) may include rocks rich in ferromagnesian minerals, notably anorthosite (Liu et al., 2022). At this location, the sedimentary basin is only 500 to 1,000 m thick (Globensky, 1993). It comprises sandstones and carbonates which have the attributes of reservoir rocks and may locally form an imperfect seal. The area also contains several structural discontinuities in the basement that can be identified by magnetic and gravity data. Some of these discontinuities correspond to faults at the surface, which are locally associated with breccias and Mississippi Valley-type zinc mineralization (Clark, 1966; Cornish, 1977; Globensky, 1986; Fekete, 1990; Brack, 1995; Brack, 1996).
In the absence of a good quality seal, this area is unlikely to be favourable for the accumulation of large volumes of hydrogen in the sediments. Interest lies in the thickness of the sedimentary cover over potential source rocks and in the presence of structural discontinuities rooted in the basement: the possible presence of hydrogen could be detected more easily at the surface there than in other areas of interest with a thick impermeable shale cover. This area could thus be used to test various investigation methods if the presence of anorthosites in the basement can be confirmed.
In addition to the isolated works documenting the presence of hydrogen or hydrocarbons of abiotic origin in fluid inclusions, which have been mentioned above (see above: Mistassini Basin, Lac Brisson Pluton and Jeffrey Mine Ophiolite), more systematic studies and geochemical databases are available to help complete the inventory of indicators of the possible presence of hydrogen, or of rocks capable of producing hydrogen, in the Canadian Shield and southern Quebec.
Combustible gases (hydrocarbons and hydrogen) have been documented in solution in the groundwater of some gold mines in the Abitibi greenstone belt (play type A1), mainly in Ontario where hydrogen can represent between 0.06% and 57.8% of the dissolved gases but also in Quebec with values between 0.51% and 3.63%, although studies are less detailed (Fritz et al., 1987; Sherwood et al., 1988; Sherwood Lollar et al., 1993a; Sherwood Lollar et al., 1993b; Sherwood Lollar et al., 2006; Sherwood Lollar et al., 2008; Warr et al., 2019; Li et al., 2021). Nitrogen content can be as high as 83.3%, CO2 content is less than 1% with a few exceptions, and helium content can be as high as 19.3% (Sherwood et al., 1988). The major and minor gases measured are characteristic of a source originating from the crystalline basement according to the classification of Milkov (2022). In at least four locations in Ontario, the gases analyzed (CH4, C2H6, C6H8, H2, N2) have a clear abiotic origin (Sherwood Lollar et al., 1993a; Sherwood Lollar et al., 2006; Sherwood Lollar et al., 2008; Montgomery, 1994; Li et al., 2021) and, in at least one case, dihydrogen isotopes suggest that its genesis is linked to a serpentinization process (Sherwood Lollar et al., 1993a).
In Quebec, hydrocarbons sampled in mine groundwater tend to represent a mixture of an abiotic source comparable to that found in Ontario, and a biogenic component accounting for 30%–50% of the total gas volume (Sherwood Lollar et al., 1993b). Hydrogen analyses are available for only two mines in Quebec. Data available in the literature for major and minor gases in these Quebec gold mines are compiled in Tables 2, 3.
TABLE 2. Composition of degassing products from four Abitibi mines, expressed in percents. “-”Not analyzed; “n.d.”: Not detected.
TABLE 3. Isotopic composition of hydrocarbons and dissolved inorganic carbon (DIC) in water from four Abitibi mines, expressed in permil.
The aforementioned authors emphasize the similarities observed between the gases analyzed in the Canadian Shield and those documented in the Fennoscandian Shield in Finland or the Kaapvall Craton in South Africa, and explain the locally low abundance of hydrogen by in situ microbial consumption processes (see also: Karolytė et al., 2022). Sherwood Lollar et al. (2007) propose a model of mixing between hydrogen-rich, micro-organism-poor saline waters and low-salinity meteoric waters with more diverse microbial content. In this model, mixing occurs episodically through the connection of otherwise hydrogeologically isolated natural fractures, as a result of a tectonic event or mining activity that reactivates these fractures: when micro-organism-rich meteoric waters come into contact with hydrogen-rich saline waters, the hydrogen is rapidly consumed by methanogenic bacteria. This model is further supported by the observations of Telling et al. (2017) in the case of the Thompson mine in Manitoba.
Work by the Ontario Geological Survey over the past 20 years has shown that the forest rings (see Section 3.2.2) documented in the Abitibi greenstone belt (play type A1) are the surface expression of methane- and sometimes H2S-rich reducing chimneys, comparable to those developed in soils above some ore bodies (Hamilton et al., 2004; Hamilton and Hattori, 2008; Brauneder, 2012; Brauneder et al., 2016). Soil gas samples were taken from several forest rings in Ontario and analysis revealed mainly methane and CO2 (Hamilton et al., 2004). Hydrogen analyses were carried out for two anomalies but were inconclusive (Majeed, 2020). However, the sampling methodology used in this case (see Hamilton et al., 2019) appears unsuitable for the preservation of hydrogen. Dissolved methane sampled in the centre of forest rings is of biogenic origin (Hamilton et al., 2004), as is the CO2 sampled in ring soils (Majeed, 2020). Some of the forest rings studied are further characterized by specific autotrophic microorganism communities (von Gunten et al., 2018).
The (near-surface) hydrogeochemistry data compiled in this study comprise groundwater analyses from 1,861 shallow private or monitoring wells distributed in southern Quebec. From this dataset, it was possible to calculate the salinity from 1,728 samples, that suggest that southern Quebec’s groundwater is generally categorized as freshwater (<1,000 mg/L TDS), with median levels at 254 mg/L and an average of 525 mg/L (Figure 4A). Brackish or saline waters are relatively rare, comprising respectively 6% and 0.7% of the available data.
FIGURE 4. Box plots showing (A) total dissolved solids (TDS); (B) methane (CH4); and (C) radon (222Rn) concentrations; including the minimum (min), median (median), mean (mean), first and third quartiles (q1, q3), maximum (max), and the threshold beyond which data are considered anomalous (upper fence = q3 +1.5 x (q3-q1)). Not all TDS and CH4 outliers are depicted within this scale. (D) Concentrations of TDS based on different hydrofacies.
The TDS boxplot indicates that outliers are above the upper fence of 866 mg/L, which is not very high considering the total range of measured values extending up to 24,400 mg/L. By visually inspecting the distribution, a first threshold of around 1,500 mg/L can be determined. When comparing with hydrofacies, it appears that the highest salinities are predominantly found in Na-Cl, and in a few cases, Na-SO4 groundwater types (Figure 4D). The Na-Cl hydrofacies might suggest an upward flow of saline fluids along faults, yet it lacks distinctiveness as it could also align with the other primary sources of salinity mentioned earlier. To discern these potential sources based on geochemical data, a more detailed statistical analysis of comprehensive ion profiles becomes imperative. However, by spatially visualizing TDS values, one can explore the likelihood that elevated salinities (>1,500 mg/L) might stem from fault presence as opposed to alternative sources of salinity.
The other two geochemical indicators selected have been analyzed with 399 wells in the dataset that have CH4 measurements and 215 wells that contain 222Rn measurements. The boxplot distribution for CH4 is depicted in Figure 4B, showing a median value of 0.03 mg/L and an average of 2.5 mg/L. The average significantly surpasses the third quartile (0.2 mg/L) and the upper fence (0.6 mg/L), primarily due to the considerable presence of positive outliers that markedly impact the mean value. Moreover, 10% of the higher values fall between 7.3 and 45.9 mg/L. Regarding 222Rn, the median value is established at 8.2 Bq/L, and the average is 23.7 Bq/L (Figure 4C). Like for the other indicators, the prevalence of outliers is noteworthy and illustrates a great heterogeneity of the distribution, that could be due to different conditions and the aquifers diversity. However, this study does not aim to provide a thorough characterization of the aquifers. Therefore, the use of outliers (geochemical anomalies) as a proxy is regarded as a valuable tool to identify possible permeable faults.
Hydrogeochemical anomalies in the study area exhibiting heightened salinity levels are localized along the coastal zone of the Lower Saint Lawrence region, coinciding also with Highway 20 between Kamouraska and Rimouski. These readings are not specifically aligned with mapped faults. Hence, the escalated salinity levels in these cases likely stem from the intrusion of seawater or the use of de-icing salts. Salinity values surpassing 1,500 mg/L also manifest in the St. Lawrence Lowlands, near Montreal and along the corridor linking Montreal and Quebec City (Figure 5). Noteworthy, this area possibly correlates with the existence of clays from the ancient Champlain Sea (Cloutier, 2004). The region also encompasses several major thoroughfares, such as Road 148 between Montreal and Ottawa, Highway 15 to the north and south of Montreal, and Highway 20 connecting Montreal and Quebec City.
FIGURE 5. Total Dissolved Solids concentrations (TDS, mg/L) in groundwater across southern Quebec, with emphasis on boxed sectors: (A) Rivière Jacques-Cartier Fault near Saint-Édouard-de-Lotbinière, (B) Aston Fault and Logan Line west of Drummondville, and (C) Tracy Brook and Delson faults south of Montreal. Concentrations exceeding 1,500 mg/L are highlighted in red. Map background source: SIGEOM (2023).
However, upon closer examination of values near geological faults, it is apparent that pronounced salinity levels are related to the Aston and Rivière Jacques-Cartier faults, along with the Logan Line. Remarkably, these heightened values adjacent to faults become particularly evident when amalgamating all other TDS data under 1,500 mg/L (Figure 5). Three sectors delineate potential permeable fault zones: the region encompassing the Rivière Jacques-Cartier Fault near Saint-Edouard-de-Lotbinière (normal fault, boxed area A), the area involving the Aston Fault and Logan Line west of Drummondville (thrust faults, boxed area B), and the sector of the Tracy Brook and Delson faults, situated to the south of Montreal (normal faults, boxed area C).
A similar pattern of anomalies is reinforced when observing the CH4 concentrations (Figure 6), as there appears to be a correlation between the highest values and the sectors demarcated by salinity. The exception is expressed within the sector to the south of Montreal (boxed area C), which presents a lack of CH4 measurement data points, except for the western section, where the available analyses have shown mostly high concentrations. Finally, few samples contain 222Rn measurements, reflecting a lack of data in some regions, such as the delimited sector C (Figure 7). Elevated anomalous values are evident in groundwater near the Mont-Saint-Hilaire magmatic intrusion (233.4 Bq/L) and within metasedimentary aquifers of the Appalachian Mountains. Overall, the values in the Appalachians exceed those recorded in the St. Lawrence Platform, possibly due to lithological disparities. Indeed, wells in the St. Lawrence Platform draw groundwater from fractured carbonate-shale formations, while those in the Appalachians interact with shales and metasediments (slates, schists, and phyllades), anticipated to contain higher uranium content (Pinti et al., 2014). Furthermore, notably high concentrations of 222Rn are still visible in the sectors targeted by the other indicators, particularly in sectors A and B.
FIGURE 6. Methane concentrations (CH4, mg/L) in groundwater across southern Quebec, grouped according to percentile classes, with emphasis on boxed sectors: (A) Rivière Jacques-Cartier Fault near Saint-Édouard-de-Lotbinière, (B) Aston Fault and Logan Line west of Drummondville, and (C) Tracy Brook and Delson faults south of Montreal. Map background: SIGEOM (2023).
FIGURE 7. Radon concentrations (222Rn, Bq/L) in groundwater of southern Quebec [data from Bordeleau et al. (2019); Pinti et al. (2014)], grouped according to percentile classes. Emphasis on boxed sectors: (A) Rivière Jacques-Cartier Fault near Saint-Édouard-de-Lotbinière, (B) Aston Fault and Logan Line west of Drummondville, and (C) Tracy Brook and Delson faults south of Montreal. Map background: SIGEOM (2023).
Oil and gas exploration data in the sedimentary basins of southern Quebec provide information on the nature of the fluids present in the subsurface, information that is rarely available in the Canadian Shield. The systematic inventory of publicly available information in the SIGPEG (2023) database identified 147 gas analyses from 63 wells, of which around half (33 wells) included at least one hydrogen analysis (100 analyses in total). Information on nitrogen, CO2 and helium concentrations was also examined, as these gases can serve as indicators of the source of fluids and therefore of the possible presence of hydrogen (Milkov, 2022). Figure 8 shows the location of all data compiled.
FIGURE 8. Gas analyses compiled for the hydrocarbon wells based on the SIGPEG (2023) database. Map background: SIGEOM (2023).
Around 78% of measured hydrogen concentrations were below 0.1%, and no correlation was observed between H2 and N2, CO2 and He or N2 and CO2 concentrations. Only twenty-one analyses from nine wells stood out with values above 0.1% H2 (Table 4). This threshold was chosen here because it corresponds to the observations of Milkov (2022), where out of a worldwide population of 6,246 hydrogen analyses, 69% had a concentration of less than 0.1%. For each of the nine wells mentioned above, the analytical results, the history of activities carried out in the well prior to sample collection, and the local geological context were examined in detail to assess the significance of results. The aim was to assess the natural or anthropogenic origin of the hydrogen. It appears that the highest hydrogen values (between 6.7% and 71.75%) can be explained by the corrosion of steel casings or acidification operations. Hydrogen concentration values for which no artificial cause could be established or suspected range from 0.1% to 2.43%, and correspond to wells located in regions where potential geological sources of hydrogen are documented. These may be local sources such as mafic dykes, or more diffuse sources such as metamorphic basement with local evidence of serpentinization (Figure 9), or even tectonic melanges of serpentinized ultramafic rocks. In all cases, however, there is insufficient information to establish a direct link between the hydrogen detected in the wells and the possible hydrogen-source rocks present in the same region and, notably, these occurrences of non-anthropic hydrogen do not coincide geographically with the main plays previously identified.
TABLE 4. Details of gas analyses for hydrocarbon wells where H2 ≥ 0.1%, with possible artificial and natural sources for hydrogen. HC: Hydrocarbons.
FIGURE 9. Hydrocarbon wells studied in southwestern Quebec, for which hydrogen values greater than 0.1% were measured. The nature of the basement encountered in drilling was compiled from drilling data and Liu et al. (2022). Map background: SIGEOM (2023).
Given the dominant natural hydrogen production models identified in Quebec, the most relevant geophysical methods for natural hydrogen exploration are, in descending order of priority: 1) Earth magnetic field measurements, 2) heat flux assessments, 3) gamma-ray spectrometric measurements, 4) magnetotelluric measurements to search for deep faults, and 5) electromagnetic measurements to locate FeS-rich conductors.
The aeromagnetic method is particularly well suited to the detection of ferrous iron, and especially in the partially oxidized state, as in magnetite. Very high-resolution helicopter-borne surveys are perfectly suited to the size of these sources. To identify surface structures, aeromagnetic measurements taken at low altitude with very tight flight lines, on the order of 25 m apart, would be necessary, but such data are not publicly available.
Other geophysical methods may also be useful, notably those that determine the presence of radioactive rocks or heat flows (hence deep fractures). In this respect, certain thorium and/or uranium anomalies could reflect local enrichment due to the upward flow of fluids or deep materials, likely to create thermal anomalies. This argues in favor of high-resolution gamma-ray spectrometry surveys (low height and narrow flight lines) to identify the presence of anomalies at selected sites (Prinzhofer et al., 2022). This method thus offers an advantageous alternative to heat flux measurements, which are difficult to implement on a large scale. Thorium is the element that shows the strongest contrasts between different rock types, and therefore the element most clearly representative of the presence of radioactive isotopes likely to provide a heat source.
The inventory of airborne electromagnetic, magnetometric and radiometric data available in Quebec shows that the magnetometric method is the most represented, followed by electromagnetic methods. This is largely due to the lower cost of magnetometric surveys, and their interest in the context of natural resource exploration in Quebec. Most of the surveys identified are of private origin. They are, however, highly concentrated over limited areas and relatively small surfaces. Public projects, on the other hand, are less numerous but more extensive, and generally cover all identified areas of geological interest. Most data acquisition projects were carried out between 2000 and 2016.
The inventory of magnetotelluric data shows limited coverage in Quebec, restricted to the kimberlite and mining areas. Since the primary objective of this method is to identify deep-seated structures, it may be possible to follow such lineaments into the areas of interest when a given survey is itself located at a distance from these areas. These data, even old ones, can be an asset and can be correlated with available seismic data.
Most seismic-reflection data are located in southern Quebec and were collected for hydrocarbon exploration purposes. Southwestern Quebec is the region with the most extensive seismic coverage, compared with the Gaspe region, even though the latter has a longer history of oil exploration. Most of the seismic lines recorded in the areas of potential hydrogen source rocks remain in the private domain and are not currently available. In the southwestern part of the Canadian Shield, seismic-reflection and seismic-refraction data were acquired as part of the Lithoprobe 8 project between 1990 and 1993 (NRCan, 2023).
Hydrogen seepage can be associated with faults or diffusive circles. The latter are easier to locate on the basis of field or airborne observations, but are also controversial in that the measurement of hydrogen in soils is not a standardized practice (McMahon et al., 2023) and diffusive circles have not yet been linked to underlying subsurface accumulations. Despite these uncertainties, the location of diffusive circles may point to areas where hydrogen is naturally present in the ground and should therefore be considered in the exploration toolbox, although it is not a definitive proof of the existence of a subsurface accumulation of natural hydrogen.
Surface depressions that correspond to diffusive circles can be detected by the main imaging methods if they have the appropriate spatial resolution. Several types of imagery and satellite data are publicly available for the whole province (Québec, 2023). The two main data sources are topographic imagery and satellite imagery, which are available across Quebec at a resolution of 20–30 m. Although this resolution is too low to detect surface depressions potentially generated by hydrogen, this map can be useful when combined with regional and local fault data to detect large deformation zones. LiDAR data contain the digital terrain model at a spatial resolution of 1 m and are available south of latitude 52° north. Satellite image mosaics are available, designed by combining several adjacent Landsat or Sentinel satellite image tiles. Mosaic coverage varies according to the year of acquisition, from southern Quebec to all of Quebec. Recent satellite data (2021 and 2022) come from the Sentinel-2 sensor and offer a spatial resolution of 10 m across Quebec.
There are two main points to note when analyzing airborne or satellite images. First, because the area of interest is so large, it is impossible to manually detect and interpret any circular depressions that may exist. A machine learning approach must be considered to enable analysis over such a large area. Second, as a large portion of the study area (the Canadian Shield) lies in a permafrost region where the action of glaciers has left traces, we need to be able to distinguish diffusive circles from other types of circular depressions (Moretti et al., 2021). Once again, an approach using machine learning would enable us to respond to this requirement.
Each of the twenty-seven plays identified above has been evaluated and rated according to three geological criteria and the proximity with possible end-users. The results are represented geographically in Figure 10 and summarized in Table 5. Detailed scores for each sector are presented in Supplementary Table S1.
FIGURE 10. Rating of the potential hydrogen source rock areas considered in Quebec. The values shown correspond to the percentage rating assigned to each of the areas considered. Map background: SIGEOM (2023).
TABLE 5. Rating score of different areas of interest for natural hydrogen source rocks in the Canadian Shield and southern Quebec.
The rating of the areas of interest reveals three main groups based on the geological criteria. The first group stands out with scores of 89% or higher. The five areas included in this group all achieve the highest scores for the local geological criterion and the analogue criterion, with the only difference occurring at the level of the potential reservoir criterion. The second group comprises eight areas with geological scores ranging from 56% to 72%. The plays in this group almost all achieve an intermediate score for the local geological criterion and more variable scores for the other two criteria. Finally, a third group comprises the areas of interest that have obtained a geological score of 44% or less (for a minimum possible of 33%). Fourteen areas are included in this tail group, all of which are characterized by a minimum score for the local geological criterion and low scores for the other two criteria. It is important to note that the areas of interest that scored highest on the criterion of proximity to end-users (mark A) are all included in the top group defined independently by the geological criteria.
Unsurprisingly, this examination of the literature and databases did not immediately lead to major discoveries of natural hydrogen in Quebec, but it uncovers convincing direct and indirect evidence of the presence of hydrogen in some regions and to the potential of others.
While anecdotal, evidence of hydrogen in fluid inclusions demonstrates the presence of a production mechanism that was once active and supports the possibility that accumulations can be discovered in geological environments, particularly in the Mistassini Basin, which has yet to be studied thoroughly from a reservoir/trap/seal standpoint. Similarly, the presence of dissolved hydrogen in the groundwater of some mines objectively represents small volumes in an environment that implies significant challenges in terms of extraction techniques, but this phenomenon is geographically widespread and supported by similar observations in other ancient cratons around the world. The presence of hydrogen in some hydrocarbon wells and the evidence of fluid circulation through deep-seated faults also open up the field of investigation to areas otherwise devoid of geological information supporting the presence of source rocks for hydrogen at depth. Existing data still convey a very hazy picture of the potential in southern Quebec due to their non homogeneous distribution, but available data must be put into perspective with the incremental advancement of knowledge in other parts of the world. For example, the emphasis in Kansas has been on the study of two wells with up to 50% hydrogen (Goebel et al., 1983; Coveney et al., 1987; Guelard et al., 2017) but should not obscure the long-known existence of at least seven other wells in which hydrogen values of 0.2%–0.5% have been documented, in a region where hydrogen emanations in soils (up to 6,400 ppm) show a strong spatial correlation with the position of lineaments interpreted as the surface expression of basement faults (Johnsgard, 1988; Angino et al., 1990). Finally, this work reveals some geological environments considered highly promising elsewhere in the world, notably some ophiolite complexes and Archean banded iron formations.
So, where to start looking for natural hydrogen in Quebec? The rating proposed here offers a first evaluation of the main exploration areas that should be considered. Any rating is intimately linked to the notion of risk, and while it remains imperfect because it is not exhaustive and is purely qualitative, the proposed rating does at least enable us to adapt an exploration strategy according to objective geological criteria, as well as more subjective criteria that touch on the spheres of profitability and social acceptability. Indeed, unlike other rating systems that consider more elaborate criteria, such as those developed for petroleum systems (Magoon and Dow, 1994; Otis and Schneidermann, 1997) or helium systems (McDowell et al., 2017; Tedesco, 2022), in the case of the very first exploration efforts in a frontier region, it is important to take into account factors that are not limited to geological considerations.
Considering the customer or end-user at an early point in the exploration strategy introduces the notion of remoteness. Do we want to focus on sectors with the highest geological value, or those that are the most accessible? This issue is not trivial in the case of Quebec, where most of the population and infrastructure are concentrated in the south, while exploration in the North is a costly process that presents numerous logistical and climatic challenges. A discovery in a remote location also poses problems of profitability. Hydrogen is more difficult to transport than other sources of energy and it must first be considered as a local resource until economies of scale can be achieved for transportation, which will only be justified by a volumetrically large discovery.
Another pragmatic choice addresses the question of technological maturity. A source of natural hydrogen present beneath a sedimentary basin can lead to accumulations in the overlying sedimentary cover, exploitable with off-the-shelf technologies, whereas a source identified in the rocks of the Canadian Shield can only be exploited in situ, i.e., without prior accumulation, which represents significant technological challenges and, incidentally, has implications for the volumes and flows that could be produced.
Another possible criterion could be the potentially renewable nature of the resource. This criterion is not considered here, as exploration in Quebec is not sufficiently mature to document this aspect. However, it could become an important factor of social acceptability in the event of a discovery. In this respect, the literature already includes numerous examples worldwide where low-temperature hydrogen production processes appear to be currently occurring (Abrajano et al., 1988; Szponar et al., 2013; Prinzhofer et al., 2018; Zgonnik et al., 2019; Geymond et al., 2022; Lévy et al., 2023). However, considering the slow production rates of hydrogen by known natural processes, the renewability of a natural hydrogen discovery may not be compatible everywhere with its economic viability and, as pointed out by Lapi et al. (2022), several aspects of the possible renewability of natural hydrogen sources remain elusive. The possibility of some source rocks being stimulated by water injection to produce hydrogen could also be considered, particularly in conjunction with carbon sequestration (Osselin et al., 2022).
The rating presented here focusses on potential source rocks. Geochemical data were taken into account in the evaluation of play A in the Canadian Shield (see Section 3.4.1), but the relationship with source rocks is less obvious in the case of the geochemical datasets available in southern Quebec and these were not considered in the evaluation of the southern plays. The benefits of the geochemical data in these cases lie in the fact that they complement the inventory of the potential source rocks and open up the possibility of adding new prospective areas to the list in the future. Thus, the occurrence of hydrogen in some hydrocarbon wells (see Section 3.4.2.2) hints to the presence of potential source rocks that are currently not included in the inventory due to their limited geographical extent, but which may be reconsidered in the future should new information becomes available. A typical example is the documented occurrence in well C100, located 10 km south of a melange of serpentinized ultramafic rocks that are laterally correlative with the ophiolite complex identified as play E2. In addition, the hydrogen occurrences documented in four wells east of Lake Saint-Pierre (Figure 9) are located in an area where deep hydrocarbon wells have penetrated the Precambrian basement and have shown that this basement can be locally serpentinized, although evidence of this is still sparse and scattered. A third example is how hydrogeochemical datasets indicate that play I area is a location where deep fluid flow is likely to occur along normal faults (play I extends southwestward from the Delson and Tracy Brook faults, see boxed area C in Figure 5). This area, although having received a low score, has been identified as suitable for testing various methods of investigation: the combination of a prospective area with deep fluid conduits independently identified by hydrogeochemical studies makes play I all the more interesting to consider for a “proof of concept” approach.
In any case, the results of this study support the possibility that natural hydrogen could be present in commercial quantities in Quebec, but dedicated exploration work remains to be carried out to confirm this hypothesis. Examples of exploration work could include the search for hydrogen emanations at the surface in areas of interest identified as priorities here, but also along fault lineaments where hydrogeochemical data indicate deep-seated fluid flows, and geophysical investigations focussed on high-resolution radiometry and heat flows. The rating of some of the plays considered here could then be re-evaluated if new geological evidence is discovered.
This study documents the presence and quality of potential source rocks for natural hydrogen in Quebec, a province of Canada that covers more than 1.5 million km2 and includes highly diverse geological environments. The purpose of this study is not to demonstrate the presence of exploitable accumulations of hydrogen on the territory, but rather to develop a framework that allows prioritizing future exploration efforts, with a view to minimize the exploration risks and investments required to develop this carbon-free resource that can potentially help with Quebec’s energy transition. Dedicated exploration work, starting with the detection of hydrogen emanations at the surface, remains to be carried out. Highlights of this study include the following:
- Four main mechanisms for the production of natural hydrogen have been selected, based on the most representative geological assemblages in the province: 1) degassing of the Earth’s mantle and core, 2) radiolysis of water in the presence of weakly radioactive rocks, 3) hydrothermal alteration and 4) decomposition of organic matter.
- Numerous geological environments favourable for the formation of natural hydrogen are documented, from the Canadian Shield in the north to the sedimentary basins of southern Quebec. A total of twenty-seven distinct areas have been retained.
- The presence of hydrogen is attested in various environments, in fluid inclusions, in dissolved gases present in the groundwater of some mines, as well as in gases sampled in some hydrocarbon wells.
- The analysis of oil and gas industry data also highlights the importance of screening the data to avoid the false-positive effect of artificially-produced hydrogen.
- Local vegetation anomalies show a regional spatial coincidence with hydrogen evidence, but a genetic link remains to be confirmed.
- The hydrogeochemical dataset reveals anomalies near mapped faults in three specific areas in southern Quebec: the Rivière Jacques-Cartier Fault sector, the Tracy Brook and Delson faults region, and the Aston Fault and the Logan Line. Sectors with normal fault systems, as the two first examples, take precedence for potential on-site hydrogen researches due to the increased possibility of deep fluids migrating upwards to the surface.
- The main areas with potential source rocks for natural hydrogen are rated according to three geological criteria to assess their respective merits. The areas that stand out according to this rating include a series of ultramafic and mafic rock intrusions (the Monteregian Hills), two ophiolite complexes (in southwestern Quebec and the Gaspe Peninsula), two contiguous Proterozoic sedimentary basins (the Mistassini and Otish basins) and a cluster of gold mines in an Archean greenstone belt (in the Abitibi sub-province).
- A fourth rating criterion considers the ease with which this resource could be integrated into the economic fabric. This criterion, distinct from the geological ones, is deemed important in a region such as Quebec, where the distribution of population and industry is highly heterogeneous, and transportation distances are long.
- Taken together, the studied criteria enable to quickly filter regions and draw a preliminary portrait of areas where natural hydrogen exploration could be prioritized and contribute to Quebec’s green economy.
The original contributions presented in the study are included in the article/Supplementary Material, further inquiries can be directed to the corresponding author.
SS: Conceptualization, Data curation, Investigation, Methodology, Project Administration, Supervision, Visualization, Writing–original draft. F-AC: Conceptualization, Data curation, Investigation, Methodology, Visualization, Writing–original draft. MLMdS: Conceptualization, Data curation, Investigation, Methodology, Visualization, Writing–original draft. GB: Conceptualization, Data curation, Investigation, Methodology, Visualization, Writing–original draft. MC: Conceptualization, Data curation, Investigation, Methodology, Visualization, Writing–original draft. PM: Conceptualization, Data curation, Investigation, Methodology, Visualization, Writing–original draft. VM: Conceptualization, Data curation, Investigation, Methodology, Visualization, Writing–original draft. MM: Conceptualization, Data curation, Investigation, Methodology, Visualization, Writing–original draft. BG: Conceptualization, Data curation, Investigation, Methodology, Visualization, Writing–original draft. EG: Conceptualization, Data curation, Investigation, Methodology, Visualization, Writing–original draft. JR: Funding acquisition, Writing–review and editing.
The author(s) declare financial support was received for the research, authorship, and/or publication of this article. The evaluation of the near-surface geochemical data is part of MLMDS M. Sc. Project of INRS-ETE, funded by National Science and Engineering Research Council (NSERC) Discovery Grants program # RGPIN-2021-03802. The Ministère de l'Économie, de l'Innovation et de l'Énergie (MEIE) of Quebec contributed financially to the authors’ research on assessing natural hydrogen potential in Quebec. It must be noted that the information and conclusions presented in this article have not been endorsed by the MEIE.
The authors would like to acknowledge Viacheslav Zgonnik (Natural Hydrogen Energy LLC), Isabelle Moretti (Université de Pau et des Pays de l’Adour), Ugo Geymond and Dan Lévy (Institut de Physique du Globe de Paris) for their insights on the different hydrogen production mechanisms, and Lyal Harris (INRS, Centre Eau Terre Environnement), Abdelali Moukhsil (Ministère des Ressources naturelles et des Forêts du Québec), Alain Tremblay (Université du Québec à Montréal) and Jean-Michel Schroetter (Bureau de recherches Géologiques et Minières, Unité CGEO, Orléans) for their insights and constructive discussions on some potentially prospective areas in Quebec. Jacinthe Légaré-Laganière and Mabrouk Ouederni (Ministère de l'Économie, de l'Innovation et de l'Énergie du Québec) are also acknowledged for improving the quality of the manuscript. The authors wish to acknowledge the two reviewers, LT and MG, for their thorough review of the article.
Author SS was employed by Enki GeoSolutions.
The remaining authors declare that the research was conducted in the absence of any commercial or financial relationships that could be construed as a potential conflict of interest.
All claims expressed in this article are solely those of the authors and do not necessarily represent those of their affiliated organizations, or those of the publisher, the editors and the reviewers. Any product that may be evaluated in this article, or claim that may be made by its manufacturer, is not guaranteed or endorsed by the publisher.
The Supplementary Material for this article can be found online at: https://www.frontiersin.org/articles/10.3389/fgeoc.2024.1351631/full#supplementary-material
Abrajano, T. A., Sturchio, N. C., Bohlke, J. K., Lyon, G. L., Poreda, R. J., and Stevens, C. M. (1988). Methane-hydrogen gas seeps, Zambales Ophiolite, Philippines: deep or shallow origin? Chem. Geol. 71, 211–222. doi:10.1016/0009-2541(88)90116-7
Allen, J. S., Thomas, W. A., and Lavoie, D. (2010). “The Laurentian margin of northeastern North America,” in From rodinia to pangea: the lithotectonic record of the appalachian region. Editors R. P. Tollo, M. J. Bartholomew, J. P. Hibbard, and P. M. et Karabinos (Boulder, Colorado, United States: Geological Society of America), 71–90. doi:10.1130/2010.1206(04)
Angino, E. E., Zeller, E. J., Dreschhoff, G. A. M., Goebel, E. D., and Coveney, R. M. J. (1990). “Spatial distribution of hydrogen in soil gas in central Kansas, USA,” in Geochemistry of gaseous elements and compounds. Editor E. M. Durrance, 485–493.
Batanova, V. G., Suhr, G., and Sobolev, A. V. (1998). Origin of geochemical heterogeneity in the mantle peridotites from the Bay of islands ophiolite, Newfoundland, Canada: ion probe study of clinopyroxenes. Geochimica Cosmochimica Acta 62, 853–866. doi:10.1016/S0016-7037(97)00384-0
Baubron, J. C., Rigo, A., and Toutain, J. P. (2002). Soil gas profiles as a tool to characterize active tectonic areas: the Jaut Pass example (Pyrenees, France). Earth Planet. Sci. Lett. 13, 69–81. doi:10.1016/S0012-821X(01)00596-9
Beaudin, J. (1980). Région du Mont Albert et du Lac Cascapédia. Ministère de l’Énergie et des Ressources. Rapp. DPV 705, 84.
Bédard, J. H. (1985). The opening of the Atlantic, the Mesozoic New England igneous province, and mechanisms of continental breakup. Tectonophysics 113, 209–232. doi:10.1016/0040-1951(85)90197-0
Bédard, J. H. (1994). Mesozoic east North American alkaline magmatism: Part 1. Evolution of Monteregian lamprophyres, Québec, Canada. Geochimica Cosmochimica Acta 58, 95–112. doi:10.1016/0016-7037(94)90449-9
Bédard, J. H., and LeCheminant, A. N. (1996). “Alnoïtes et roches apparentées, province ignée des collines montérégiennes, Québec,” in La Recherche de Diamants au Canada (Commission géologique du Canada), 3228, 119–123. doi:10.4095/208203
Bélanger, M. (1984). Région du lac Brisson, Territoire du Nouveau-Québec. Ministère de l’Énergie et des Ressources. https://gq.mines.gouv.qc.ca/documents/EXAMINE/DP8420/.
Bergeron, A. (1980). Pétrographie et géochimie du complexe igné alcalin de Crevier et de son encaissant métasomatisé. Univ. Québec à Chicoutimi; mémoire maîtrise, 142. doi:10.1522/1300469
Bertrand, R., and Lavoie, V. (2006). Hydrocarbon source rocks and organic maturation of lower Paleozoic successions in the St. Lawrence Platform and in the external domain of the Quebec Appalachians. https://gac.ca/product/gac-mac-2006-abstracts-volume-31-montreal-2006/.
Bordeleau, G., Rivard, C., Lavoie, D., Lefebvre, R., Malet, X., and Ahad, J. M. E. (2019). Geochemical and isotopic data used to infer the origins of natural gas in groundwater of the Saint-Édouard area, southern Québec. Geol. Surv. Can. Open File 8505, 91. doi:10.4095/321053
Bordeleau, G., Rivard, C., Lavoie, D., Lefebvre, R., Malet, X., and Ladevèze, P. (2018). Geochemistry of groundwater in the Saint-Édouard area, Quebec, Canada, and its influence on the distribution of methane in shallow aquifers. Appl. Geochem. 89, 92–108. doi:10.1016/j.apgeochem.2017.11.012
Boreham, C. J., Edwards, D. S., Feitz, A. J., Murray, A. P., Mahlstedt, N., and Horsfield, B. (2023). Modelling of hydrogen gas generation from overmature organic matter in the Cooper Basin, Australia. APPEA J. 63, S351–S356. doi:10.1071/AJ22084
Brack, W. (1995). Progress report on the Hemmingford Property, Sawmill Grid, Southern Quebec. Ministère des Ressources naturelles et des Forêts. http://sigeom.mrnf.gouv.qc.ca.
Brack, W. (1996). Progress report on the Hemmingford Property, Walklate Grid, Southern Quebec. Ministère des Ressources naturelles et des Forêts. http://sigeom.mrnf.gouv.qc.ca.
Brauneder, K., Hamilton, S. M., and Hattori, K. (2016). Geochemical processes in the formation of "forest rings": examples of reduced chimney formation in the absence of mineral deposits. Geochem. Explor. Environ. Anal. 16, 85–99. doi:10.1144/geochem2015-360
Brauneder, K. M. (2012). Geochemistry of forest rings in Northern Ontario: identification of ring edge processes in peat and soil. M.Sc. thesis. Univeristy of Ottawa.
Breemen, O. v., and Currie, K. L. (2004). Geology and U–Pb geochronology of the Kipawa Syenite Complex — a thrust related alkaline pluton — and adjacent rocks in the Grenville Province of western Quebec. Can. J. Earth Sci. 41, 431–455. doi:10.1139/e04-010
Card, K. D. (1990). A review of the superior province of the Canadian shield, a product of archean accretion. Precambrian Res. 48, 99–156. doi:10.1016/0301-9268(90)90059-Y
Card, K. D., and Poulsen, K. H. (1998). “Geology and mineral deposits of the superior province of the Canadian shield,” in Geology of the precambrian superior and Grenville provinces and precambrian fossils in north America (lucas, S. And st-onge, M.R., coordinators) (Canada: Geological Survey of Canada). doi:10.1130/DNAG-GNA-C1.13
Castonguay, S., Dietrich, J., Shinduke, R., and Laliberté, J.-Y. (2006). Nouveau regard sur l’architecture de la Plate-forme du Saint-Laurent et des Appalaches du sud du Québec par le retraitement des profils de sismique réflexion M-2001, M-2002 et M-2003. Canada: Commission géologique du Canada. doi:10.4095/222405
Chandler, F. W., 1988. The early proterozoic Richmond Gulf graben, east coast of Hudson Bay, Quebec. Geol. Surv. Can. Bull. 362, 76 doi:10.4095/126313
Charette, B., Lafrance, I., Vanier, M.-A., and Godet, A. (2019). Domaine de Mistinibi-Raude, sud-est de la Province de Churchill, Nunavik, Québec, Canada: synthèse de la géologie. Ministère de l’Énergie et des Ressources naturelles, Québec. BG 2019-07. https://gq.mines.gouv.qc.ca/bulletins-geologiques/churchill/mistinibi-raude/.
Chartrand, F. (1994). “Synthèse des gisements métallifères dans le Nord-Ouest québécois,” in Géologie du Québec (Québec: Ministère des Ressources naturelles), 40–46.
Chen, Z., Lavoie, D., Jiang, C., Duchesne, M. J., and Malo, M., 2016. Geological characteristics and petroleum resource assessment of the macasty formation, Anticosti island, Quebec, Canada. Geological Survey of Canada. doi:10.4095/297865
Chen, Z., Lavoie, D., and Malo, M., 2014. Geological characteristics and petroleum resource assessment of Utica Shale, Quebec, Canada. Geol. Surv. Can., 7606, 43. doi:10.4095/293793
Cherns, L., and Wheeley, J. R. (2009). Early palaeozoic cooling events: peri-gondwana and beyond. London: Geological Society Special Publication. doi:10.1144/SP325.13
Chown, E. H. (1984). “Mineralization controls in the aphebian formations, Chibougamau, Mistassini and otish areas,” in Chibougamau - stratigraphy and mineralization. Editors J. Guha, and E. H. Chown (Canada: Canadian Institute of Mining and Metallurgy).
Clark, T. (1994). “Géologie et gîtes de l’Orogène du Nouveau-Québec et de son arrière-pays,” in Géologie du Québec (Hocq, M., coordonnateur) (Québec: Ministère des Ressources naturelles), 47–65.
Clark, T., and Wares, R. (2004). Synthèse lithotectonique et métallogénique de l’Orogène du Nouveau-Québec (Fosse du Labrador). MM 2004-01. Québec: Ministère des Ressources naturelles de la Faune et des Parcs.
Clark, T. H. (1966). Région de Châteauguay. Ministère des Richesses naturelles. Rapp. géologique 122, 68.
Clark, T. H. (1977). Région de Granby (ouest). Ministère des Richesses naturelles du Québec. Rapp. RG 177, 109.
Cloutier, V. (2004). Origine et évolution géochimique des eaux souterraines du système aquifère des Basses-Laurentides dans les roches sédimentaires paléozoïques des Basses-Terres-du Saint-Laurent. Ph.D. thesis. Québec, Canada: Institut national de la recherche scientifique (INRS), Québec, Canada. Available at: https://espace.inrs.ca/id/eprint/1445/
Cornish, R. A. (1977). Report on drill programme: hemmingford, Quebec, project 7710. Rapport No. GM 39112. Quebec: Ministère des Ressources naturelles et des Forêts. Available at: https://sigeom.mines.gouv.qc.ca
Costagliola, A., Vandenborre, J., Blain, G., Baty, V., Haddad, F., and Fattahi, M. (2017). Radiolytic dissolution of calcite under gamma and helium ion irradiation. J. Phys. Chem. C 121, 24548–24556. doi:10.1021/acs.jpcc.7b07299
Courtillot, V., Davaille, A., Besse, J., and Stock, J. (2003). Three distinct types of hotspots in the Earth’s mantle. Earth Planet. Sci. Lett. 205, 295–308. doi:10.1016/S0012-821X(02)01048-8
Coveney, R. M., Goebel, E. D., Zeller, E. J., Dreschhoff, G. A. M., and Angino, E. E. (1987). Serpentinization and the origin of hydrogen gas in Kansas. AAPG Bull. 71, 39–48. doi:10.1306/94886D3F-1704-11D7-8645000102C1865D
Currie, K. L. (1985). An unusual peralkaline granite near Lac Brisson, Quebec-Labrador. Canada: Geological survey of Canada, 73–80. doi:10.4095/120018
David, J., Simard, M., Bandyayera, D., Goutier, J., Hammouche, H., Pilote, P., et al. (2012). Datations U-Pb effectuées dans les Provinces du Supérieur et de Churchill en 2010-2011. RP 2012-01. Québec: Ministère de l’Énergie, des Ressources naturelles et de la Faune. Available at: https://sigeom.mines.gouv.qc.ca
De Souza, S., Tremblay, A., Ruffet, G., and Pinet, N. (2012). Ophiolite obduction in the Quebec Appalachians, Canada — 40Ar/39Ar age constraints and evidence for syn-tectonic erosion and sedimentation1Geological Survey of Canada Contribution 20100430.2GEOTOP (Centre de recherche en géochimie et géodynamique) Contribution 2011-0002.3This article is one of a series of papers published in this CJES Special Issue: In honour of Ward Neale on the theme of Appalachian and Grenvillian geology. Can. J. Earth Sci. 49, 91–110. doi:10.1139/e11-037
Dimroth, E. (1978). Région de la Fosse du Labrador (54°30′-56°30′). RG-193. Québec: Ministère des Richesses naturelles, 396. Available at: https://sigeom.mines.gouv.qc.ca
Drolet, J.-P., Martel, R., Poulin, P., and Dessau, J.-C. (2014). Methodology developed to make the Quebec indoor radon potential map. Sci. Total Environ. 473-474, 372–380. doi:10.1016/j.scitotenv.2013.12.039
Drolet, J.-P., Martel, R., Poulin, P., Dessau, J.-C., Lavoie, D., Parent, M., et al. (2013). An approach to define potential radon emission level maps using indoor radon concentration measurements and radiogeochemical data positive proportion relationships. J. Environ. Radioact. 124, 57–67. doi:10.1016/j.jenvrad.2013.04.006
Dubois, J. M. (1993). Origine mycologique de ronds dans la forêt coniférienne de l’île d’Anticosti, golfe du Saint-Laurent (Canada). Photo-Interprétation 31, 207–212.
Eby, G. N. (1984). Geochronology of the monteregian hills alkaline igneous province, Quebec. Geology 12, 468–470. doi:10.1130/0091-7613(1984)12<468:GOTMHA>2.0.CO;2
Eby, G. N. (1987). “The monteregian hills and white mountains alkaline igneous provinces, eastern north America,” in Alkaline igneous rocks. Editors J. G. Fitton, and B. G. J. Upton (London: Geological Society Special Publications). doi:10.1144/GSL.SP.1987.030.01.21
El Bourki, M., and Moukhsil, A. (2021). Géologie de la région de Dolbeau-Blondelas, Province de Grenville, région du Saguenay-Lac-Saint-Jean. BG 2022-02. Québec, Canada: MERN. Available at: https://sigeom.mines.gouv.qc.ca
Essehli, R., Crumière, F., Blain, G., Vandenborre, J., Pottier, F., Grambow, B., et al. (2011). H2 production by γ and He ions water radiolysis, effect of presence TiO2 nanoparticles. Int. J. Hydrogen Energy 36, 14342–14348. doi:10.1016/j.ijhydene.2011.05.136
Evangelatos, J., Butler, K. E., and Spray, J. G. (2009). A marine magnetic study of a carbonate-hosted impact structure: ile Rouleau, Canada. Geophys. J. Int. 179, 171–181. doi:10.1111/j.1365-246X.2009.04304.x
Faure, S. (2010). Perméabilité crustale dans le Moyen-Nord québécois: guides d’exploration géophysique pour l’or, l’uranium et le diamant. CONSOREM, Projet 2009-10. Québec: Ministère des Ressources naturelles et de l’Énergie. Available at: https://sigeom.mines.gouv.qc.ca
Faure, S., Tremblay, A., and Angelier, J. (1996). State of intraplate stress and tectonism of northeastern America since Cretaceous times, with particular emphasis on the New England–Quebec igneous province. Tectonophysics 255, 111–134. doi:10.1016/0040-1951(95)00113-1
Feininger, T., and Goodacre, A. K. (1995). The eight classical Monteregian hills at depth and the mechanism of their intrusion. Can. J. Earth Sci. 32, 1350–1364. doi:10.1139/e95-109
Fekete, M. (1990). Summary report on geological, geochemical, and geophysical surveys and reverse-circulation drill programme completed on the Southwestern Quebec Zinc Project for Freewest Resources. Rapport No. GM 49643. Ministère des Ressources naturelles et des Forêts. Available at: https://sigeom.mines.gouv.qc.ca
Frarey, M. J., and Duffell, S., 1964. Revised stratigraphic nomenclature for the central part of the Labrador Trough. Geol. Surv. Can. Stud. 64-25, 13 doi:10.4095/123909
Fritz, P., Frape, S. K., and Miles, M. (1987). “Methane in the crystalline rocks of the Canadian Shield,” in Saline water and gases in crystalline rocks. Editors P. Fritz, and S. K. Frape (London: Geological Association of Canada Special Paper).
Fryer, B. J. (1972). Age determinations in the circum – ungava geosyncline and the evolution of precambrian banded iron-formations. Can. J. Earth Sci. 9, 652–663. doi:10.1139/e72-055
Geymond, U., Ramanaidou, E., Lévy, D., Ouaya, A., and Moretti, I. (2022). Can weathering of banded iron formations generate natural hydrogen? Evidence from Australia, Brazil and South Africa. Minerals 12, 163. doi:10.3390/min12020163
Giroux, J.-F., Bergeron, Y., and Veillette, J. J. (2001). Dynamics and morphology of giant circular patterns of low tree density in black spruce stands in northern Quebec. Can. J. Bot. 79, 420–428. doi:10.1139/b01-022
Globensky, Y. (1985). Géologie des régions de Saint-Jean (partie nord) et de Beloeil. Rapport MM 84-03. Ministère de l’Énergie et des Ressources.
Globensky, Y. (1986). Géologie de la région de Saint-Chrysostome et de Lachine (sud). Rapport MM 84-02. Ministère de l’Énergie et des Ressources.
Globensky, Y. (1993). Lexique stratigaphique canadien - Région des Appalaches, des Basses-Terres du Saint-Laurent et des Îles de la Madeleine. Direction générale de l’exploration géologique et minérale, Gouvernement du Québec. Rapp. DV 91-23, 327.
Goebel, E. D., Coveney, R. M., Angino, E. E., and Zeller, E. (1983). Naturally occurring hydrogen gas from a borehole on the western flank of Nemaha Anticline in Kansas. AAPG Bull. 67, 1324. doi:10.1306/03B5B76D-16D1-11D7-8645000102C1865D
Gold, D. P., Eby, G. N., Bell, K., and Vallée, M. (1986). Carbonatites, diatremes, and ultra-alkaline rocks in the Oka area, Quebec. London: Geological Association of Canada.
Groulier, P.-A., Ohnenstetter, D., Andre-Mayer, A. S., Zeh, A., Solgadi, F., Moukhsil, A., et al. (2014). Étude des minéralisations en Nb-Ta de l’intrusion alcaline de Crevier. UMR 7359 Géoressources, AQAT – urstm. MB 2014-33. Goethe Universitat, MERN. Available at: https://sigeom.mines.gouv.qc.ca
Guelard, J., Beaumont, V., Rouchon, V., Guyot, F., Pillot, D., Jézéquel, D., et al. (2017). Natural H2 in Kansas: deep or shallow origin? Geochem. Geophys. Geosystems 18, 1841–1865. doi:10.1002/2016GC006544
Hamblin, A. P. (2008). Hydrocarbon potential of the Paleozoic succession of Hudson Bay/James Bay: preliminary conceptual synthesis of background data. London: Geological survey of Canada. doi:10.4095/225183
Hamilton, S. M. (2005). "Forest rings" and their implications for geochemical exploration of oil, gas and mineral deposits. https://www.appliedgeochemists.org/sites/default/files/Hamilton_2_Forest_Rings.pdf.
Hamilton, S. M., Burt, A. K., Hattori, K. H., and Shirota, J. (2004). The distribution and source of forest ring-related methane in northeastern Ontario. Ontario, Canada: Ontario Geological Survey. Available at: http://www.geologyontario.mndm.gov.on.ca/mndmfiles/pub/data/imaging/OFR6145//OFR6145.pdf
Hamilton, S. M., and Hattori, K. (2008). Spontaneous potential and redox responses over a forest ring. Geophysics 73, B67–B75. doi:10.1190/1.2890287
Hamilton, S. M., von Gunten, K., Sherwood Lollar, B., DiLoreto, Z. A., Alam, M. S., Snihur, K., et al. (2019). “Soil gas compositional changes and electrical field (SP) responses at the edges of the Thorn North forest ring,” in Summary of field work and other activities (Ontario, Canada: Ontario Geological Survey). Available at: http://www.geologyontario.mndm.gov.on.ca/mndmfiles/pub/data/imaging/OFR6360//OFR6360.pdf
Hammouche, H., Legouix, C., Goutier, J., and Dion, C. (2012). Géologie de la région du lac Zéni. RG 2012-02. Québec: Ministère des Ressources naturelles. Available at: https://sigeom.mines.gouv.qc.ca
Héroux, Y., and Bertrand, R. (1991). Maturation thermique de la matière organique dans un bassin du Paléozoïque inférieur, basses-terres du Saint-Laurent, Québec, Canada. Can. J. Earth Sci. 28, 1019–1030. doi:10.1139/e91-093
Héroux, Y., Chagnon, A., Diagana, B., Richer-Laflèche, M., and Moar, R. (2004). Potentiel minéral du bassin de Mistassini: une réévaluation basée sur des outils empruntés de l’exploration pétrolière. MB 2004-07. Québec: Ministère des Ressources naturelles et de l’Énergie. Available at: https://sigeom.mines.gouv.qc.ca
Hocq, M. (1994). “La province du Supérieur,”. MM 94-01 in Géologie du Québec (Québec: Ministère des Ressources naturelles).
Hocq, M., and Verpaelst, P. (1994). “Les sous-provinces de l'Abitibi et du Pontiac,”. MM 94-01 in Géologie du Québec (Québec: Ministère des Ressources naturelles).
Ignatenko, G. K., Gremchenko, P. I., and Glushkov, Y. M. (2016). Hydrogen concentration monitoring in subsoil air on tectonic faults on the territory adjacent to NPP site. Nucl. Energy Technol. 2, 151–155. doi:10.1016/j.nucet.2016.07.014
James, D. T., Connelly, J. N., Wasteneys, H. A., and Kilfoil, G. J. (1996). Paleoproterozoic lithotectonic divisions of the southeastern Churchill Province, western Labrador. Can. J. Earth Sci. 33, 216–230. doi:10.1139/e96-019
Johnsgard, S. K. (1988). The fracture pattern of north-central Kansas and its relation to hydrogen soil gas anomalies over the Midcontinent Rift System. https://www.kgs.ku.edu/Publications/OFR/1988/OFR88_25/.
Kagi, H., Lu, R., Davidson, P., Goncharov, A., Mao, H., and Hemley, R. (2000). Evidence for ice VI as an inclusion in cuboid diamonds from high P-T near infrared spectroscopy. Mineral. Mag. 64, 1089–1097. doi:10.1180/002646100549904
Karolytė, R., Warr, O., van Heerden, E., Flude, S., de Lange, F., Webb, S., et al. (2022). The role of porosity in H2/He production ratios in fracture fluids from the Witwatersrand Basin, South Africa. Chem. Geol. 595, 120788. doi:10.1016/j.chemgeo.2022.120788
Ko, B., Chariton, S., Prakapenka, V., Chen, B., Garnero, E. J., Li, M., et al. (2022). Water-induced diamond formation at Earth's core-mantle boundary. Geophys. Res. Lett. 49, e2022GL098271. doi:10.1029/2022GL098271
Kumagai, Y., Kimura, A., Taguchi, M., Nagaishi, R., Yamagishi, I., and Kimura, T. (2013). Hydrogen production in gamma radiolysis of the mixture of mordenite and seawater: fukushima NPP accident related. J. Nucl. Sci. Technol. 50, 130–138. doi:10.1080/00223131.2013.757453
Labbé, J.-Y., and Lacoste, P. (2004). Minéralisations en Cu-Ag dans les basaltes protérozoïques de la région du lac Guillaume-Delisle, Nouveau-Québec. ET 2004-02. Québec: Ministère des Ressources naturelles et de l’Énergie. Available at: https://sigeom.mines.gouv.qc.ca
Ladevèze, P., Rivard, C., Lavoie, D., Séjourné, S., Lefebvre, R., and Bordeleau, G. (2019). Fault and natural fracture control on upward fluid migration: insights from a shale gas play in the St. Lawrence Platform, Canada. Hydrogeology J. 27, 121–143. doi:10.1007/s10040-018-1856-5
Lafrance, I., Charette, B., and Vanier, M.-A. (2018). Sud-est de la Province de Churchill, Nunavik, Québec, Canada: synthèse de la géologie. Québec: Ministère de l’Énergie et des Ressources naturelles. Available at: https://gq.mines.gouv.qc.ca/bulletins-geologiques/churchill/
Laliberté, J.-Y., and Trépanier, A. (1982). Carte structurale du marqueur bleu (Trenton autochtone), Basses-Terres du Saint-Laurent. Rapport 1982TA000-01. Québec: Ministère des Richesses naturelles. Available at: http://sigpeg.mrnf.gouv.qc.ca
Lapi, T., Chatzimpiros, P., Raineau, L., and Prinzhofer, A. (2022). System approach to natural versus manufactured hydrogen: an interdisciplinary perspective on a new primary energy source. Int. J. Hydrogen Energy 47, 21701–21712. doi:10.1016/j.ijhydene.2022.05.039
LaVerne, J. A., and Tandon, L. (2003). H2 production in the radiolysis of water on UO2 and other oxides. J. Phys. Chem. B 107, 13623–13628. doi:10.1021/jp035381s
Lavoie, D., Pinet, N., Dietrich, J., Hannigan, P., Castonguay, S., Hamblin, A. P., et al. 2009. Petroleum resource assessment, paleozoic successions of the St. Lawrence platform and Appalachians of eastern Canada. Geol. Surv. Can., 6174, 275 doi:10.4095/248071
Lefeuvre, N., Truche, L., Donzé, F.-V., Ducoux, M., Barré, G., Fakoury, R.-A., et al. (2021). Native H2 exploration in the western Pyrenean foothills. Geochem. Geophys. Geosystems 22, e2021GC009917. doi:10.1029/2021GC009917
Lefeuvre, N., Truche, L., Donzé, F.-V., Gal, F., Tremosa, J., Fakoury, R.-A., et al. (2022). Natural hydrogen migration along thrust faults in foothill basins: the North Pyrenean Frontal Thrust case study. Appl. Geochem. 145, 105396. doi:10.1016/j.apgeochem.2022.105396
Lefticariu, L., Pratt, L. A., LaVerne, J. A., and Schimmelmann, A. (2010). Anoxic pyrite oxidation by water radiolysis products - a potential source of biosustaining energy. Earth Planet. Sci. Lett. 292, 57–67. doi:10.1016/j.epsl.2010.01.020
Lévy, D., Boka-Mene, M., Meshi, A., Fejza, I., Guermont, T., Hauville, B., et al. (2023). Looking for natural hydrogen in Albania and Kosova. Front. Earth Sci. 11, 1167634. doi:10.3389/feart.2023.1167634
Li, L., Li, K., Giunta, T., Warr, O., Labidi, J., and Sherwood Lollar, B. (2021). N2 in deep subsurface fracture fluids of the Canadian Shield: source and possible recycling processes. Chem. Geol. 585, 120571. doi:10.1016/j.chemgeo.2021.120571
Liu, H., Ban, S., Bédard, K., and Giroux, B. (2022). Characteristics of Precambrian basement intruded by Cretaceous geological intrusions in Monteregian Igneous Province and their impacts on regional thermal structure. Adv. Geo-Energy Res. 6, 206–220. doi:10.46690/ager.2022.03.04
Lollar, B. S., Lacrampe-Couloume, G., Voglesonger, K., Onstott, T. C., Pratt, L. M., and Slater, G. F. (2008). Isotopic signatures of CH4 and higher hydrocarbon gases from Precambrian Shield sites: a model for abiogenic polymerization of hydrocarbons. Geochimica Cosmochimica Acta 72, 4778–4795. doi:10.1016/j.gca.2008.07.004
Magoon, L. B., and Dow, W. G. (1994). “The petroleum system,” in The petroleum system - from source to trap. Editors L. B. Magoon, and W. G. Dow (Tulsa, OK, USA: AAPG Memoir). doi:10.1306/M60585
Mahlstedt, N., Horsfield, B., Weniger, P., Misch, D., Shi, X., Noah, M., et al. (2022). Molecular hydrogen from organic sources in geological systems. J. Nat. Gas Sci. Eng. 105, 104704. doi:10.1016/j.jngse.2022.104704
Majeed, H. (2020). Investigation of soil gas Geochemistry at thorn north forest ring. M.Sc. thesis. University of Toronto. Available at: https://tspace.library.utoronto.ca/bitstream/1807/103477/3/Majeed_Hamnah_202011_MSc_thesis.pdf
Malvoisin, B., and Brunet, F. (2023). Barren ground depressions, natural H2 and orogenic gold deposits: spatial link and geochemical model. Sci. Total Environ. 856, 158969. doi:10.1016/j.scitotenv.2022.158969
Matte, S., Constantin, M., and Stevenson, R., 2022. Mineralogical and geochemical characterisation of the Kipawa syenite complex, Quebec: implications for rare-earth element deposits. Geol. Surv. Can. Sci. Present. 129: 36 doi:10.4095/329212
Matton, G., and Jébrak, M. (2009). The Cretaceous Peri-Atlantic Alkaline Pulse (PAAP): deep mantle plume origin or shallow lithospheric break-up? Tectonophysics 469, 1–12. doi:10.1016/j.tecto.2009.01.001
Matveev, S., Ballhaus, C., Fricke, K., Truckenbrodt, J., and Ziegenben, D. (1997). Volatiles in the Earth's mantle: I. Synthesis of CHO fluids at 1273 K and 2.4 GPa. Geochimica Cosmochimica Acta 61, 3081–3088. doi:10.1016/S0016-7037(97)00142-7
McDowell, B. P., Milkov, A. V., and Anderson, D. S. (2017). The He system - a modification of the petroleum system for inert gases. Tulsa, OK, USA: AAPG. Search and Discovery. Available at: https://www.searchanddiscovery.com/documents/2017/42098mcdowell/ndx_mcdowell.pdf
McHone, J. G., Ross, M. E., and Greenough, J. D. (1987). “Mesozoic dyke swarms of eastern North America,” in Mafic dyke swarms. Editors H. C. Halls, and W. F. Fahrig (London: Geological Association of Canada). Available at: http://earth2geologists.net/Publications/McHoneGreenoughRoss_GSCSpPaper87.pdf
McMahon, C. J., Roberts, J. J., Johnson, G., Edlmann, K., Flude, S., and Shipton, Z. K. (2023). Natural hydrogen seeps as analogues to inform monitoring of engineered geological hydrogen storage. London: Geological Society. doi:10.1144/SP528-2022-59
Melton, C. E., and Giardini, A. A. (1981). The nature and significance of occluded fluids in three Indian diamonds. Am. Mineralogist 66, 746–750.
Milkov, A. V. (2022). Molecular hydrogen in surface and subsurface natural gases: abundance, origins and ideas for deliberate exploration. Earth-Science Rev. 230, 104063. doi:10.1016/j.earscirev.2022.104063
Miller, R. R. (1985). “Geology of the Strange Lake alkalic complex and the associated Zr-Y-Nb-Be-REE mineralization,” in Granite-related mineral deposit; geology, petrogenesis and tectonic setting. Editors R. P. Taylor, and D. F. Strong (Canada: Canadian Institute of Mining).
Montgomery, J. (1994). An isotopic study of CH4 and associated N2 and H2 gases in Canadian shiel mining environments. M.Sc., University of Toronto. Available at: https://librarysearch.library.utoronto.ca/permalink/01UTORONTO_INST/fedca1/cdi_proquest_journals_304170435
Morency, M. (1989). Production of elements and compounds by deserpentinization of ultramafic rock – summary of patent 1262031. Canadian Patent Database. Canadian Intellectual Property Office. Available at: https://www.ic.gc.ca/opic-cipo/cpd/fra/brevet/1262031/sommaire.html?query=1262031&type=basic_search
Moretti, I., Brouilly, E., Loiseau, K., Prinzhofer, A., and Deville, E., 2021. Hydrogen emanations in intracratonic areas: new guide lines for early exploration basin screening. Geosciences 11, 145 doi:10.3390/geosciences11030145
Morin, C., and Laliberté, J.-Y. (2002a). The unexpected Silurian-Devonian structural style in Western Gaspe - new insight for promising hydrocarbon plays. Québec: Ministère des Ressources Naturelles.
Morin, C., and Laliberté, J.-Y. (2002b). Une nouvelle image structurale: Résultats des mégatransects de la sismique réflexion à travers la ceinture appalachienne de la péninsule gaspésienne. Québec: Ministère des Ressources Naturelles.
Moritz, A., Hélie, J.-F., Pinti, D. L., Larocque, M., Barnetche, D., Retailleau, S., et al. (2015). Methane baseline concentrations and sources in shallow aquifers from the shale gas-prone region of the St. Lawrence lowlands (Quebec, Canada). Environ. Sci. Technol. 49, 4765–4771. doi:10.1021/acs.est.5b00443
Moukhsil, A., and El Bourki, M. (2021). Géologie de la région de Girardville, Province de Grenville, région du Saguenay – lac-Saint-Jean. BG 2021-02. Québec, Canada: MERN. Available at: https://gq.mines.gouv.qc.ca/bulletins-geologiques/girardville
Nassif, G. J. (1993). The Strange Lake peralkaline Complex, Québec-Labrador: the hypersolvus-subsolvus granite transition and feldspar mineralogy. M.Sc. thesis. McGill University, 104. Available at: https://escholarship.mcgill.ca/concern/theses/7h149q66x
Normand, C., and Williams-Jones, A. E. (2010). Origin of low molecular weight hydrocarbon gases during serpentinization: insights from fluid inclusions in rodingites. GeoCanada 2010
NRCan, (2023). Transect Lithoprobe 8 - Abitibi-Grenville. Ressources naturelles Canada, Archive de données sismiques régionales 2D. https://open.canada.ca/data/fr/dataset/6a480542-1f8d-495f-99c9-db4f623c780b.
Osselin, F., Soulaine, C., Fauguerolles, C., Gaucher, E. C., Scaillet, B., and Pichavant, M. (2022). Orange hydrogen is the new green. Nat. Geosci. 15, 765–769. doi:10.1038/s41561-022-01043-9
Otis, R. M., and Schneidermann, N. (1997). A process for evaluating exploration prospects. AAPG Bull. 81 (1997), 1087–1109. doi:10.1306/522B49F1-1727-11D7-8645000102C1865D
PACES (2023). Projets d’acquisition de connaissances sur les eaux souterraines. Ministère de l’Environnement, de la Lutte contre les changements climatiques, de la Faune et des Parcs. online database: https://www.environnement.gouv.qc.ca/eau/souterraines/programmes/acquisition-connaissance.htm.
Pagé, P., Bédard, J. H., Schroetter, J.-M., and Tremblay, A. (2008). Mantle petrology and mineralogy of the Thetford mines ophiolite complex. Lithos 100, 255–292. doi:10.1016/j.lithos.2007.06.017
Payot, B. D., Arai, S., Tamayo, R. A., and Yumul, G. P. (2013). Textural evidence for the chromite-oversaturated character of the melt involved in podiform chromitite formation. Resour. Geol. 63, 313–319. doi:10.1111/rge.12011
Peel, M. (2014). Investigation géologique et géochimique des relations entre un réservoir pétrolier et un aquifère superficiel dans le secteur Haldimand, Gaspé. M.Sc. thesis. Québec, Canada: Institut national de la recherche scientifique INRS. Available at: https://espace.inrs.ca/id/eprint/2612/
Petrella, L. (2012). The nature and origin of REE mineralization in the Misery syenitic Intrusion. M.Sc. thesis. northern Quebec, Canada: McGill University. Available at: https://escholarship.mcgill.ca/concern/theses/cf95jf701
Petrella, L., Williams-Jones, A. E., Goutier, J., and Walsh, J. (2014). The nature and origin of the rare earth element mineralization in the Misery syenitic intrusion, northern Quebec, Canada. Econ. Geol. 109, 1643–1666. doi:10.2113/econgeo.109.6.1643
Philpotts, A. R. (1976). Petrography of mounts st-bruno and rougemont. Rapport ES 016. Québec: Ministère des Richesses naturelles. Available at: https://sigeom.mines.gouv.qc.ca
Pillet, D. (1985). Le granite peralcalin du lac Brisson, Territoire du Nouveau-Québec: résultats préliminaires. MB 85-37. Québec: Ministère de l’Énergie et des Ressources. Available at: https://sigeom.mines.gouv.qc.ca
Pinet, N., Lavoie, D., Keating, P., and Brouillette, P. (2008). Gaspé belt subsurface geometry in the northern Québec Appalachians as revealed by an integrated geophysical and geological study: 1 — potential field mapping. Tectonophysics 460, 34–54. doi:10.1016/j.tecto.2008.07.006
Pinti, D. L., Retailleau, S., Barnetche, D., Moreira, F., Moritz, A. M., Larocque, M., et al. (2014). 222Rn activity in groundwater of the St. Lawrence Lowlands, Quebec, eastern Canada: relation with local geology and health hazard. J. Environ. Radioact. 136, 206–217. doi:10.1016/j.jenvrad.2014.05.021
Prinzhofer, A., Rigollet, C., Berthelot, J., and Françolin, J. (2022). Gamma spectrometry as the best proxy for hydrogen exudations. https://www.hnatsummit.com/.
Prinzhofer, A., Tahara Cissé, C. S., and Diallo, A. B. (2018). Discovery of a large accumulation of natural hydrogen in Bourakebougou (Mali). Int. J. Hydrogen Energy 43, 19315–19326. doi:10.1016/j.ijhydene.2018.08.193
Québec, D. (2023). Données ouvertes géoréférencées pour la Province de Québec. Gouvernement du Québec, online database. https://www.donneesquebec.ca/.
Raynauld, M., Peel, M., Lefebvre, R., Crow, H., Molson, J., Ahad, J., et al. (2014). Caractérisation hydrogéologique du secteur Haldimand. Rapport de recherche R-1497. Québec, Canada: Institut national de la recherche scientifique. Available at: https://www.environnement.gouv.qc.ca/eau/rapport-haldimand/haldimand_rapport.pdf
Remick, J. H., Gillain, P. R., and Durden, C. J. (1963). Géologie de la baie de Rupert - rivière Missisicabi, territoires d’Abitibi et de Mistassini. Rapport Préliminaire RP 498. Québec: Ministère des Ressources naturelles. Available at: https://sigeom.mines.gouv.qc.ca
Rivers, T., and Corrigan, D. (2000). Convergent margin on southeastern laurentia during the mesoproterozoic: tectonic implications. Can. J. Earth Sci. 37, 359–383. doi:10.1139/e99-067
Rivers, T., Culshaw, N., Hynes, A., Indares, A., Jamieson, R., and Martignole, J. (2012). “The Grenville orogen – a post-LITHOPROBE perspective,” in Tectonic styles in Canada: the LITHOPROBE perspective. Editors J. A. Percival, F. A. Cook, and R. M. Clowes (London: Geological Association of Canada). Chapter 3. Available at: https://gac.ca/product/tectonic-styles-in-canada/
Rohon, M.-L., Vialette, Y., Clark, T., Roger, G., Ohnenstetter, D., and Vidal, P. (1993). Aphebian mafic-ultramafic magmatism in the Labrador Trough (New Quebec): its age and the nature of its mantle source. Can. J. Earth Sci. 30, 1582–1593. doi:10.1139/e93-136
RSESQ (2023). Réseau de suivi des eaux souterraines du Québec. Ministère de l’Environnement, de la Lutte contre les changements climatiques, de la Faune et des Parcs. online database: https://www.environnement.gouv.qc.ca/eau/piezo/index.htm.
Rüpke, L. H., and Hasenclever, J. (2017). Global rates of mantle serpentinization and H2 production at oceanic transform faults in 3-D geodynamic models. Geophys. Res. Lett. 44, 6726–6734. doi:10.1002/2017GL072893
Sader, J. A., Leybourne, M. I., McClenaghan, M. B., and Hamilton, S. M. (2007). Low-temperature serpentinization processes and kimberlite groundwater signatures in the Kirkland Lake and Lake Timiskiming kimberlite fields, Ontario, Canada: implications for diamond exploration. Geochem. Explor. Environ. Anal. 7, 3–21. doi:10.1144/1467-7873/06-900
Salvi, S., and Williams-Jones, A. E. (1997). Fischer-Tropsch synthesis of hydrocarbons during sub-solidus alteration of the Strange Lake peralkaline granite, Quebec/Labrador, Canada. Geochimica Cosmochimica Acta 61, 83–99. doi:10.1016/S0016-7037(96)00313-4
Sanford, B. V., and Norris, A. W. (1975). Devonian stratigraphy of the Hudson platform. London: Geological survey of Canada. doi:10.4095/127031
Saucier, G., Noreau, C., Casgrain, P., Côté, P., Larochelle, E., Bilodeau, M., et al. (2013). Feasibility study for the Kipawa project, Temiscamingue area. NI 43-101 technical report. Québec, Canada.
Schrauder, M., and Navon, O. (1994). Hydrous and carbonatitic mantle fluids in fibrous diamonds from Jwaneng, Botswana. Geochimica Cosmochimica Acta 58, 761–771. doi:10.1016/0016-7037(94)90504-5
Séjourné, S., Lefebvre, R., Malet, X., and Lavoie, D., 2013. Synthèse géologique et hydrogéologique du Shale d'Utica et des unités sus-jacentes (Lorraine, Queenston et dépôts meubles), Basses-Terres du Saint-Laurent, Québec, Doss. public 7338, 165 doi:10.4095/292430
Sherwood, B., Fritz, P., Frape, S. K., Macko, S. A., Weise, S. M., and Welhan, J. A. (1988). Methane occurrences in the Canadian shield. Chem. Geol. 71, 223–236. doi:10.1016/0009-2541(88)90117-9
Sherwood Lollar, B., Frape, S. K., Fritz, P., Macko, S. A., Welhan, J. A., Blomqvist, R., et al. (1993b). Evidence for bacterially generated hydrocarbon gas in Canadian shield and fennoscandian shield rocks. Geochimica Cosmochimica Acta 57, 5073–5085. doi:10.1016/0016-7037(93)90609-Z
Sherwood Lollar, B., Frape, S. K., Weise, S. M., Fritz, P., Macko, S. A., and Welhan, J. A. (1993a). Abiogenic methanogenesis in crystalline rocks. Geochimica Cosmochimica Acta 57, 5087–5097. doi:10.1016/0016-7037(93)90610-9
Sherwood Lollar, B., Lacrampe-Couloume, G., Slater, G. F., Ward, J., Moser, D. P., Gihring, T. M., et al. (2006). Unravelling abiogenic and biogenic sources of methane in the Earth’s deep subsurface. Chem. Geol. 226, 328–339. doi:10.1016/j.chemgeo.2005.09.027
Sherwood Lollar, B., Voglesonger, K., Lin, L.-H., Lacrampe-Couloume, G., Telling, J., Abrajano, T. A., et al. (2007). Hydrogeologic controls on episodic H2 release from Precambrian fractured rocks - energy for deep subsurface life on Earth and Mars. Astrobiology 7, 971–986. doi:10.1089/ast.2006.0096
SIGEOM (2023). Système d’information géominière. Ministère des Ressources naturelles et des Forêts du Québec. online database https://sigeom.mines.gouv.qc.ca.
SIGPEG (2023). Système d’Information Géoscientifique Pétrolier et Gazier. Ministère des Ressources naturelles et des Forêts du Québec. online database http://sigpeg.mrnf.gouv.qc.ca.
SOQUIP (1984). Carte structurale du Trenton autochtone. Société québécoise d’initiative pétrolière (SOQUlP). Rapport DP 84-31. Québec: Ministère des Richesses naturelles. Available at: https://sigpeg.mrn.gouv.qc.ca
St. Julien, P., and Hubert, C. (1975). Evolution of the taconian orogen in the Quebec Appalachians. Am. J. Sci. 275A, 337–362.
Szponar, N., Brazelton, W. J., Schrenk, M. O., Bower, D. M., Steele, A., and Morrill, P. L. (2013). Geochemistry of a continental site of serpentinization, the tablelands ophiolite, gros morne national park: a mars analogue. Icarus 224, 286–296. doi:10.1016/j.icarus.2012.07.004
Taner, M. F. (1992). Reconnaissance géologique de la région du lac Juillet, Territoire du Nouveau-Québec. MB 91-19. Québec: Ministère de l’Énergie et des Ressources. Available at: https://sigeom.mines.gouv.qc.ca
Tedesco, S. (2022). Geology and production of helium and associated gases. Amsterdam, Netherlands: Elsevier. Available at: https://www.elsevier.com/books/geology-and-production-of-helium-and-associated-gases/tedesco/978-0-323-90988-4
Telling, J., Voglesonger, K., Sutcliffe, C. N., Lacrampe-Couloume, G., Edwards, E., and Sherwood Lollar, B. (2018). Bioenergetic constraints on microbial hydrogen utilization in Precambrian deep crustal fracture fluids. Geomicrobiol. J. 35, 108–119. doi:10.1080/01490451.2017.1333176
Thériault, R. (2012). Caractérisation du Shale d’Utica et du Groupe de Lorraine, Basses-Terres du Saint-Laurent - partie 2: interprétation géologique. Rapport DV 2012-04. Québec: Ministère de l’Énergie et des Ressources.
Thériault, R., Laliberté, J.-Y., and Brisebois, D. (2004). Prolongement du Graben du Saguenay sous les roches appalachiennes: implications pour l’exploration des hydrocarbures dans le Bas-Saint-Laurent. Québec: Ministère des Ressources naturelles.
Thériault, R., Laliberté, J.-Y., and Brisebois, D. (2005). Empreintes des grabens d’Ottawa-Bonnechère et du Saguenay dans la plate-forme du Saint-Laurent et les Appalaches: cibles pour l’exploration des hydrocarbures. Québec: Ministère de l’Énergie et des Ressources.
Truche, L., Bourdelle, F., Salvi, S., Lefeuvre, N., Zug, A., and Lloret, E. (2021). Hydrogen generation during hydrothermal alteration of peralkaline granite. Geochimica Cosmochimica Acta 308, 42–59. doi:10.1016/j.gca.2021.05.048
Valiquette, G., and Pouliot, G. (1977). Géologie des monts Brome et Shefford. Rapport ES 028. Québec: Ministère de l’Énergie et des Ressources. Available at: https://sigeom.mines.gouv.qc.ca
Veillette, J., and Giroux, J.-F. (1999). The enigmatic rings of the James Bay Lowland: a probable geological origin. London: Geological survey of Canada. doi:10.4095/210206
von Gunten, K., Hamilton, S. M., Zhong, C., Nesbø, C., Li, J., Muehlenbachs, K., et al. (2018). Electron donor-driven bacterial and archaeal community patterns along forest ring edges in Ontario, Canada. Environ. Microbiol. Rep. 10, 663–672. doi:10.1111/1758-2229.12678
Wardle, R. J., James, D. T., Scott, D. J., and Hall, J. (2002). The southeastern Churchill Province: synthesis of a Paleoproterozoic transpressional orogen. Can. J. Earth Sci. 39, 639–663. doi:10.1139/e02-004
Warr, O., Giunta, T., Ballentine, C. J., and Sherwood Lollar, B. (2019). Mechanisms and rates of 4He, 40Ar, and H2 production and accumulation in fracture fluids in Precambrian Shield environments. Chem. Geol. 530, 119322. doi:10.1016/j.chemgeo.2019.119322
Wavrant, L.-M., Sappin, A.-A., Beland, C. M. J., Chartier-Montreuil, W., Fleury, F., Zhao, P., et al. (2017). Projet terres rares au Québec. Rapport MB 2017-17. Québec: Ministère de l’Énergie et des Ressources naturelles. Available at: https://sigeom.mines.gouv.qc.ca
Weiss, Y., Czas, J., and Navon, O. (2022). Fluid inclusions in fibrous diamonds. Rev. Mineralogy Geochem. 88, 475–532. doi:10.2138/rmg.2022.88.09
Whitmore, J., and Pineau, P.-O. (2023). État de l’énergie au Québec, Édition 2023. Chaire de gestion du secteur de l’énergie. https://energie.hec.ca/wp-content/uploads/2023/05/EEQ2023_WEB.pdf.
Williams, H. (1979). Appalachian orogen in Canada. Can. J. Earth Sci. 16, 792–807. doi:10.1139/e79-070
Windley, B. F. (1986). “Comparative tectonics of the western Grenville and the western Himalaya,” in The Grenville province. Editors J. M. Moore, A. Davidson, and A. J. Baer (St. John’s, Newfoundland, Canada: GAC Special Paper).
Zajac, I. S., Miller, R. R., Birkett, T. C., and Nantel, S. (1984). Le gîte de Zr, Y, Nb et Be du complexe alcalin de Strange Lake. DV 84-18. Québec: Québec-Labrador. Ministère de l’Énergie et des Ressources. Available at: https://sigeom.mines.gouv.qc.ca
Zgonnik, V. (2020). The occurrence and geoscience of natural hydrogen: a comprehensive review. Earth-Science Rev. 203, 103140. doi:10.1016/j.earscirev.2020.103140
Keywords: energy transition, underground reservoir, source rock, hydrogeochemistry, exploration screening, Superior province, Canadian Shield, Appalachian basin
Citation: Séjourné S, Comeau F-A, Moreira dos Santos ML, Bordeleau G, Claprood M, Mouge P, Mulliez V, Malo M, Giroux B, Gloaguen E and Raymond J (2024) Potential for natural hydrogen in Quebec (Canada): a first review. Front. Geochem. 2:1351631. doi: 10.3389/fgeoc.2024.1351631
Received: 06 December 2023; Accepted: 01 February 2024;
Published: 18 March 2024.
Edited by:
Viacheslav Zgonnik, Natural Hydrogen Energy LLC, United StatesReviewed by:
Laurent Truche, Université Grenoble Alpes, FranceCopyright © 2024 Séjourné, Comeau, Moreira dos Santos, Bordeleau, Claprood, Mouge, Mulliez, Malo, Giroux, Gloaguen and Raymond. This is an open-access article distributed under the terms of the Creative Commons Attribution License (CC BY). The use, distribution or reproduction in other forums is permitted, provided the original author(s) and the copyright owner(s) are credited and that the original publication in this journal is cited, in accordance with accepted academic practice. No use, distribution or reproduction is permitted which does not comply with these terms.
*Correspondence: Stephan Séjourné, c3Nlam91cm5lQGVua2lnZW9zb2x1dGlvbnMuY2E=
†Present address: Maxime Claprood, Centre d’études sur les ressources minérales, Université du Québec à Chicoutimi, Saguenay, QC, Canada
Disclaimer: All claims expressed in this article are solely those of the authors and do not necessarily represent those of their affiliated organizations, or those of the publisher, the editors and the reviewers. Any product that may be evaluated in this article or claim that may be made by its manufacturer is not guaranteed or endorsed by the publisher.
Research integrity at Frontiers
Learn more about the work of our research integrity team to safeguard the quality of each article we publish.