- 1Geosciences Water and Environment Laboratory, Faculty of Sciences, Mohammed 5 University, Rabat, Morocco
- 2Regional Center for Education and Training Professions CRMEF Casablanca-Settat, Casablanca, Morocco
- 3Laboratory of Natural Resources and Environment, Polydisciplinary Faculty of Taza, Sidi Mohamed Ben Abdellah University, Taza, Morocco
Under arid and semi-arid climate conditions, as is the case in many Moroccan regions, the availability and quality of water are the main keys to the sustainable development of agricultural zones. In the Fritissa region covering Middle Moulouya and a part of Lower Moulouya, North-eastern Morocco, irrigation practices are necessary for agricultural activities, in an environment with significant population growth, and limited water resources. The main aim of this study is to assess the quality of groundwater and its suitability for irrigation purposes, and to determine the possible water-rock interaction processes that influence the groundwater chemistry. The approach is based on the analysis of major ions contents and the calculation of quality indices namely pH, Electrical conductivity (EC), Total Dissolved Solids (TDS), Total Hardness (TH), Sodium Percentage (Na%), Residual Sodium Carbonate (RSC), Sodium Adsorption Ratio (SAR) and Permeability Index (PI) for 94 groundwater samples. Piper classification is used to determine the chemical water facies, while commonly used distribution and geochemical diagrams helped to discuss the possible interaction processes between water and aquifer rocks. The studied groundwater showed varying values for pH (5.8–8.8), salinity (367–3301 mg/L) and total hardness (15–186 mg/l as CaCO3). Ca-Mg-HCO3, Ca-Mg-SO4-Cl, and mixed water facies types are found, while one sample showed Na-K-SO4-Cl facies. The plotting of samples in Gibbs diagrams suggests possible water-rock interactions, that are confirmed by geochemical distribution diagrams. The Wilcox diagram showed that most of the water samples are of excellent to good and good to permissible categories, while the US salinity Hazard diagram revealed low sodium hazard and low, medium and high levels of salinity. Groundwater is suitable for irrigation according to current international standards if some precautions are considered.
1 Introduction
The kingdom of Morocco has implemented the regionalization program as a strategic reform to create viable and sustainable regions (Consultative Commission of Regionalization, 2011). In many regions of Morocco, agriculture is the main human activity as a key of development. But under arid to semi-arid climate conditions, sustainable development requires a good knowledge of natural parameters: geology, morphology, pedology and climate, and anthropic parameters: demography, poverty and vulnerability to poverty, but it remains strongly influenced by the availability and quality of water resources (Ettazarini, 2011). Indeed, natural waters are threatened by various sources of pollution and the assessment of their suitability for different purposes is necessary before use. In addition, groundwater chemistry is mainly dependent on the aquifer lithology, the residence time in the reservoir and the reactive substances to which it may be exposed (Yao et al., 2012; Amadou et al., 2014). In agricultural zones the irrigation is an inevitable method that is widely practiced, especially in areas where rainwater is scarce. Agricultural production depends on the quality of irrigation water and it is affected by the concentration of dissolved salts according to the United State Environmental Protection Agency (USEPA, 1976).
Many approaches are commonly used to assess the suitability of water to irrigation, based on the analysis of major ions (Piper, 1944; Eaton, 1950; Richards et al., 1954; Wilcox, 1955; Gibbs, 1970; Salem et al., 2021). Information given from the water chemical composition offers the possibility to calculate water-derived quality indices that are frequently used (Ettazarini, 2004; Ettazarini, 2005; Naseem et al., 2010; Ishaku et al., 2011; Asiwaju-Bello et al., 2013; Nagaraju et al., 2014; Nayak and Sahoo, 2014).
Piper (1944) proposed a tri-linear graphic representation to illustrate the water chemistry, based on cations and anions contents, whose combination allows the determination of the water facies. Eaton (1950) highlighted the role of carbonates in irrigation water and then the importance of considering the Residual Sodium Carbonate (RSC) in the water analysis process. Richards et al. (1954) proposed a classification of groundwater quality based on the RSC values. The United States Salinity Laboratory adopted a hazard diagram, based on the works of Richards et al. (1954) where electrical conductivity (EC) and Sodium Adsorption Ratio (SAR) are combined to assess the water usefulness. The Wilcox’s (1955) diagram uses electrical conductivity and sodium percentage (Na%) to determine the water quality classes. Gibbs (1970) suggested a pair of diagrams where dominant cations and anions are compared to total dissolved solids (TDS), helpful to illustrate the main processes controlling the water chemistry, notably water-rock interaction, evaporation, and dissolution-precipitation. Many distribution graphs are commonly used to discuss the possible water-rock interaction processes (Handa, 1969; Cerling et al., 1989; Fisher and Mulican, 1997; Ettazarini, 2004; Ettazarini, 2005).
The ability of solid substances from the aquifer matrix to exchange ions with other ions in aqueous solution is called ion-exchange capacity. In natural systems anions are exchanged very rarely, if compared to cations (Merkel and Planer-Friedrich, 2002). In addition, multivalent ions such as calcium and magnesium ions are more strongly bound than monovalent ions such as potassium and sodium ions (Stumm and Morgan, 1996).
Ion exchange process mainly refers to a release of adsorbed calcium and magnesium ions from the aquifer matrix, and their replacement by sodium ions existing in the circulating water (Luo et al., 2018). This type of ion exchange is equally known as direct ion exchange if the exchange can be done without intervention of an intermediate element. It is frequent in coastal aquifers where sodium-rich seawater penetrates a carbonate aquifer rocks (Nadler et al., 1980; Magaritz and Luzier, 1985). It occurs when deep waters rich in dissolved NaCl encounter rocks with exchangeable calcium ions (Morgan and Jankowski, 2004), but it happens also when calcimagnesian soils are irrigated with sodium-rich water (Fantong et al., 2009; Gaofeng et al., 2009). When the ion exchange requires an intermediate element, mainly carbonic acid, it is called indirect ion exchange in which water plays the role of mediator (Elliott, 2007).
Reverse ion exchange takes place when dissolved calcium and magnesium ions in water are replaced with sodium ions from solid phase. This is possible when Ca-Mg-rich water encounters aquifer rocks with exchangeable Na-loaded clays (Coetsiers and Walraevens, 2006; Nagaraju et al., 2006; Fantong et al., 2009).
The aim of this study is to assess the groundwater suitability for irrigation purpose in the Fritissa region. The approach is based on the analysis of major ions in 94 well water samples. It includes calculation of quality indices notably Total Hardness (TH), Sodium Percentage (Na%), Residual Sodium Carbonate (RSC), Sodium Adsorption Ratio (SAR) and Permeability Index (PI). These are helpful for understanding the main characteristics of water chemistry and assessing its usefulness. The suitability of groundwater for irrigation use is discussed according to the Richards et al. (1954) and Wilcox (1955) diagrams.
2 Presentation of the study area
The Fritissa region is a plain between 2.35 and 4.4 west longitudes and 32.7–34.5 north latitudes. It is bordered by the Middle Atlas, to the west, and the Hauts Plateaux to the east. The study area has a width of 62.54 km and a length of 188.15 km in the form of a rectangle. It covers the Middle Moulouya and a part of Lower Moulouya (Figure 1). The terrains are mainly drained by the Moulouya River and secondarily by the Za River. Elevation is ranging between 336 m and 3380 m, where 75% of total surface has altitudes from 770 m to 1640 m and slopes less than 12% (Figure 2).
According to the Agency of Hydraulic Basin of Moulouya (AHBM, 2022) annual precipitation varies from the southeast 100–200 mm/year to the northwest 200–300 mm/year. Wet period is short between march and may, while the rest of the year is a dry period.
Following the territorial reorganization program, the study area includes parts of four provinces: these are provinces of Boulmane, Taourirt, Guercif Jerrada and Figuig, and it covers totally or partially 32 communes where most of them is in the rural domain. According to the Consultative Commission of Regionalization (CCR, 2011) reports the local population undergoes moderate to severe poverty conditions and the irrigated agriculture remains the main resource for sustainable development in the region. Thus, the assessment of water suitability for irrigation is necessary.
The Figure 3 illustrates the land use map where five classes are revealed, notably (i) water areas that are limited to 0.02% only of global surface and corresponding to the Mouloya river and its tributaries, in addition to some waterways related to the Za river; (ii) vegetation areas dominated by trees counting for 0.53% of surface; (iii) agricultural zone occupied by variable crops and covering 1.47% of studied area; (iv) built area including urban, suburban and rural agglomerations representing 0.15% of surface; and (v) bare lands that are dominating in the study and accounting for 97.83% of global area.
3 Geological and hydrogeological settings
Geological formations encountered in the region of interest are of variable ages and are resulting from the filling of a subsident basin. The basement corresponds to Paleozoic shists and quartzites outcropping in the Debdou Buttonhole. The Secondary cover is unconformably laying on the basement formations, and it is composed of stratigraphic levels, presented based on their dominant lithologies and according to the Geological Service of Morocco (GSM) as follows (Figure 4).
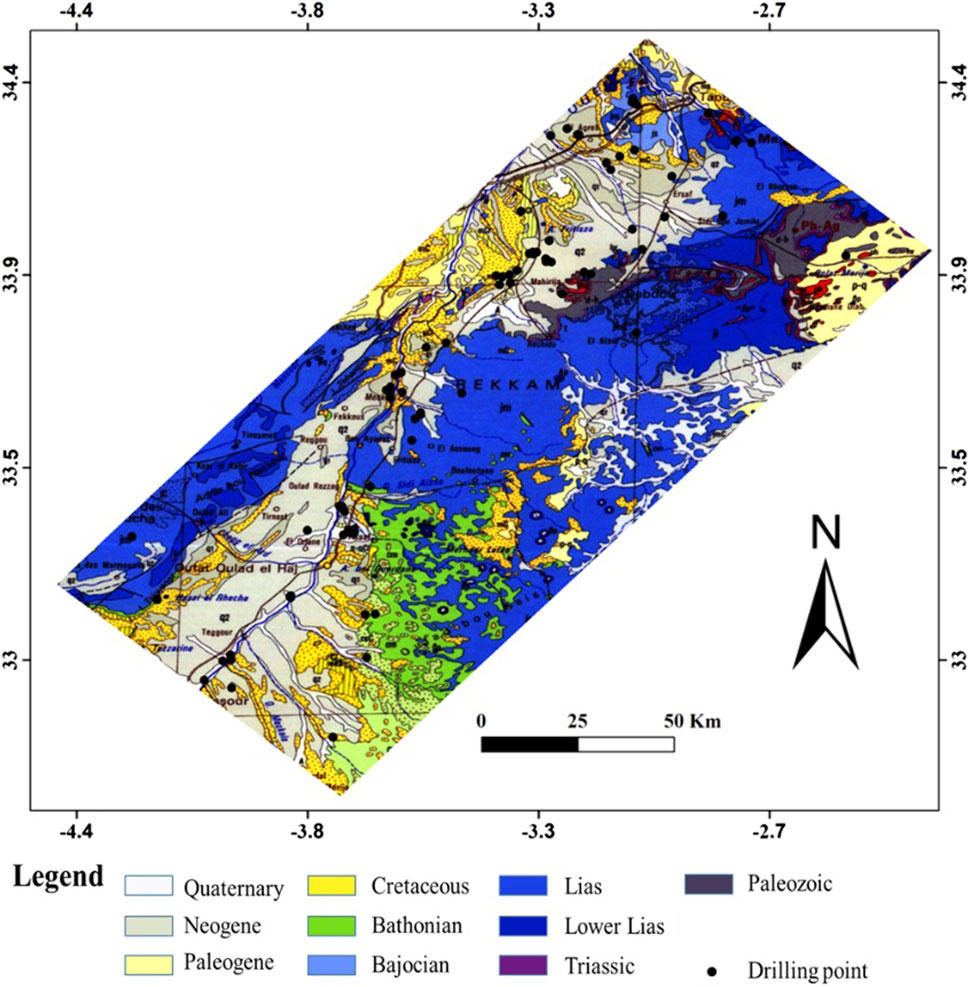
FIGURE 4. Geological map of the study area extracted from the Moroccan Geological Map at 1/1000000 scale.
Triassic formations are red clayey deposits and basalt intrusions (GSM, 1985; 1991; 1995); Lias corresponds to limestone and red to green marl; Aalenian-Bajocian represented by dominant limestone, dolomite and sandy limestone; Upper Bajocian dominated by limestone; Bathonian with red sandstone, marl and limestone; Callovian to probable Oxfordian with sandy deposits passing laterally to limestone and marl (GSM, 1964a; b; 1972a,b); Cretaceous is characterized by clayey marl and limestone deposits; Miocene corresponds to marl, limestone and conglomerate; Pliocene formations show limestone, sand and conglomerate deposits; and Quaternary deposits that form complex formations including sand, clay, silt, limestone, marl and conglomerate (GSM, 1964a,b, 1972a,b, 1985, 1991,1995).
Many studies focused on the tectonic evolution of the eastern Morocco revealed that geological formations of Meso-Cenozoic ages are controlled by basement tectonic structures, reactivated during Hercynian and Late-Hercynian phases, and other tectonic episodes of Mesozoic, Neogene and Plio-Quaternary ages (Ait Brahim and Chotin, 1990; Bernini et al., 2000; Sani et al., 2000; Gharmane et al., 2019; El Kati et al., 2022; Yaagoub et al., 2023). These later works highlighted the existence of a fault system of NE-SW and NW-SE dominating directions that affect the different terrains in the region.
The aquifer system in the Moulouya corresponds to geological levels with rocks whose permeability and fracturing conditions are favorable for aquifer formation. The main aquifer levels are described by Combe and Simonot (1971) and are from the deepest to the most superficial as follows:
Triassic sandstones and basalts form discontinuous aquifers and are encountered in some boreholes, but their significance is limited due to low water yields in boreholes and degraded quality. The Liassic limestones constitute the deepest and most exploitable aquifer. However, its extension is frequently limited by the existence of marls being a lateral change in the lithology of the geological formations. Another aquifer corresponds to limestones, dolomites and sandy limestones of Aalenian-Bajocian formations. The third aquifer is made up of Callovian-Oxfordian sandstones passing laterally to limestones then to marls which limit the extent of the aquifer. Miocene, Pliocene and Quaternary formations form a complex superficial aquifer where water occurs in variable levels of different lithologies. These are mainly sands, sandstones, siltstones, and conglomerates, intercalated with clayey and marly layers.
4 Material and methods
The analysis of chemical characteristics of groundwater in Fritissa region is based on the data provided by the Agency of the Hydraulic Basin of Moulouya (AHBM), concerning 94 water samples from drilling points. The data includes pH, Electrical Conductivity (EC), and major ions concentrations in mg/L. The data are obtained by using the standard methods in the Moulouya Labotratory, according to protocols described by Rodier et al. (1975). Indeed, pH and EC are measured on field using appropriate pH-meter and conductivity-meter. Sulfate, nitrate and nitrite are analyzed by colorimetric method with a spectrophotometer, Potassium and sodium are determined by the atomic absorption method with a flame spectrophotometer. Calcium and magnesium are obtained by complexometric method, while carbonates are assessed by sulfuric acid titration. Chloride is evaluated by the Mohr method. In this study the samples whose chemical analysis are showing ionic balance less than 6 are retained.
The data processing aiming at the groundwater characterization as well as the assessment of its suitability for irrigation is based on the calculation of commonly used irrigation indices as follows:
Total dissolved solids (TDS) are calculated from conductivity according to Eq. 1:
TDS is used with major ions concentration to reveal possible processes influencing water chemistry in Gibbs diagram.
Total Hardness (TH), expressed in mg/L as CaCO3 and calculated as shown in Eq. 2:
When concentrations are calculated in mg/L.
The percentage of sodium Na%, calculated as follows in Eq. 3:
Na% is used in global quality classification of Wilcox (1955), when it is compared to the Electrical conductivity (EC).
Residual Sodium Carbonate (RSC) is calculated using the Eq. 4:
Waters with RSC values up to 1.25 are considered as safe, RSC between 1.25 and 2.5 indicate marginal water, while waters of RSC upper than 2.5 are of poor quality.
The sodium Adsorption Ratio (SAR), which provides information on the degree to which soil water undergoes cationic interaction. It is obtained using the Eq. 5:
The SAR and EC are the two entrees for the classification of groundwater quality according to the US Salinity Hazard approach (Richards et al., 1954).
The permeability index PI is also used and calculated as shown in Eq. 6:
According to Doneen (1964) three categories of water can be distinguished following PI value: water with PI upper than 75 is suitable, it is good if PI ranges from 25 to 75, and it is unsuitable when PI is less than 25. For the determination of Na%, SAR, RSC and PI, concentration values are calculated in meq/L.
Statistical analysis is helpful to understand the distribution of used variables and the possible relationships between them. This task is completed by using the SPSS software and by performing a Principal Component Analysis (PCA). Another way to understand the chemistry of studied waters is to determine their chemical facies, which is possible by the help of the Piper software. To apprehend the conditions and processes controlling the chemistry of waters, it is necessary to perform deep analysis of data by common distribution diagrams to reveal possible water-rock interaction processes. The main objective of this study, corresponding to the assessment of the groundwater suitability for irrigation purposes, is obtained by using quality classifications notably Richards and Wilcox diagrams. The results are then discussed and measures to take before using the groundwater are communicated.
5 Results and discussion
5.1 Socio-economic data
The socio-economic data show significant variations between regions in the study area (Table 1). Population growth rates vary from −39.74% registered in 2014 in rural centers to 85.04% in 2004 observed in urban centers. This shows the large difference between urban and semi-urban areas on the one hand, and rural areas on the other. The disparity between urban and rural domains is also observed in terms of rates of poverty (5.55%–90.8%) and vulnerability to poverty (6.92%–31.78). However, constraints resulting from the lack of water resources to meet the needs of the population remain the common problem affecting all areas of the region. Indeed, the irrigated areas in rural and semi-urban communes reach up to 1600 km2, equivalent to about 86% of communal surface. These remarks demonstrate that agriculture and grazing are the main human activities on which the development of the Moulouya region is based.
5.2 Statistical analysis of chemical data
The descriptive statistics are illustrated in Table 2, where 18 variables are considered. By focusing on the standard-deviation values it is shown that many variables have raised values indicating a large dispersion and significant variability of water chemistry. Maximum values reveal that major ions concentrations are mainly in acceptable ranges, according to the Food and Agriculture Organization (FAO) quality guidelines for irrigation waters (Mauritius Government Gazette, 1999). Exceptions are observed for sulfate (1455 ±1 mg/L) exceeding the permissible limit (250 mg/L), chloride (699 ±1 mg/L) above the limit (350 mg/L) for surface irrigation, and HCO3 (574 ±1 mg/L) slightly beyond the limit (518 mg/L). Waters with TDS upper than 2000 mg/L may present severe salinity problems for many sensible plants (James et al., 1982; Ayer and Westcot, 1985). The SAR variable shows equally values upper than the permissible limit of 6 (up to 10.11), suggesting thus necessary precautions to take before using the concerned waters (Ayer and Westcot, 1985). According to the permeability index PI values, 25 samples are revealed unsuitable (PI < 25), while 87 are good (25 < PI < 75).
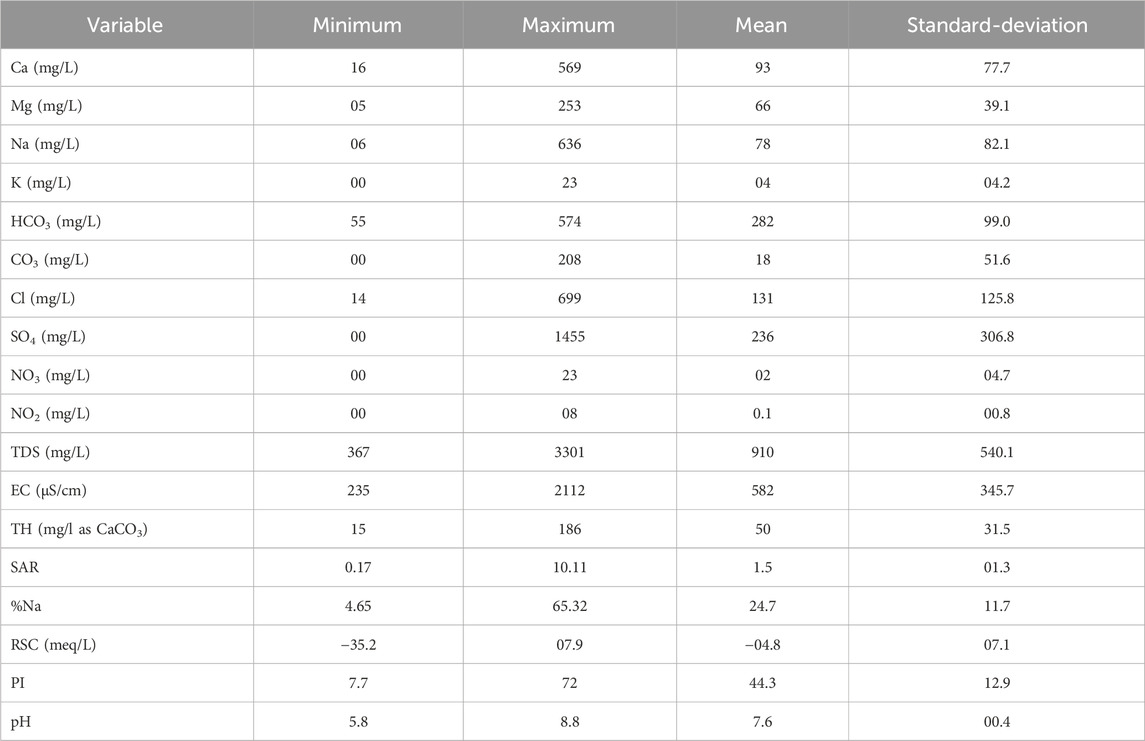
TABLE 2. Descriptive statistics concerning the 18 variables used to characterize the groundwater chemistry in the study area.
For deep analysis of the global dataset, the Principal Component Analysis CPA is performed to present the information on 18 variables (18 dimensions) in a space of reduced dimensions. Indeed, Table 3 presents the evolution of the total expressed variance that refers to the quantity of information explained by the factors in the space of reduced dimensions. It is revealed that the four first factors explain a cumulated variance of 77.08%, while the two first factors conserve 61.74% of global information.
Figure 5 shows the 18 considered variables plotted in a two dimensions graph, constructed with the two first factors. It highlights significant positive correlations between TDS, EC, SO4, TH, Ca and Mg variables and the first factor that explains 42.97% of global variance. RSC shows negative correlation with this factor. Na%, PI and SAR variables present positive correlation with the second factor that explains 18.77% of global information. Potassium seems to be moderately correlated with the first factor. Other variables (HCO3, CO3, NO2, NO3 and pH) are feebly correlated with the two factors and are located at the center of the graph and distributed independently to other variables.
5.3 Chemical groundwater facies
The determination of chemical water facies is performed by using the Piper classification, as shown in Figure 6. Three water categories are dominating in the Fritissa area: Ca-Mg-HCO3 facies is observed in 28 samples representing 29.79% of total observation points, Ca-Mg-SO4 facies is detected in 29 samples (30.85%), while Mixed facies is retrieved in 36 samples (38.30%). One sample only showed Na-K-SO4-Cl facies. These results demonstrate the contribution of calcium, magnesium, carbonates, and sulfate ions to the chemistry of groundwater, if compared to sodium, potassium, and chloride ions. This is due to variable geochemical processes acting on different chemical characters of water.
5.4 Possible processes controlling the groundwater chemistry
The evolution of total salinity of groundwater shows a large variation without significant correlation with all the major ions distribution (Figure 5), suggesting that there are a variety of water-rock interaction processes that control water chemistry. To confirm the possibility of water-rock interaction, the Gibbs diagrams are used as shown in Figure 7.
The distribution of groundwater samples in the Gibbs diagrams suggests that water-rock interaction may be the principal process affecting groundwater quality in the research area. A significant part of samples shows a tendency to evaporation process with elevated values of TDS.
For more precision in revealing water-rock processes, analyzed samples are plotted in distribution diagrams (Figure 8). Reverse ion exchange involves the release of calcium and magnesium into the water, and Ca+Mg/SO4+HCO3 ratios can rise up to 1.2. In the opposite direction, the ion exchange process involves capturing calcium and magnesium by clay minerals containing sodium, and resulting in an excess of SO4+HCO3 over Ca+Mg (Fisher and Mulican, 1997). By plotting the samples in the Ca+Mg vs. SO4+HCO3 diagram (Figure 8A), it is shown that these potential processes are confirmed. The samples above the line d:1/1 are dominant with the remark that most of samples are near the line d:1/1 suggesting other possible processes involved in the control of water chemistry. Figure 8B is a representation of studied waters in the graph HCO3 vs. Ca+Mg that determines waters undergoing direct and indirect ion exchange processes (Handa, 1969). Samples falling on the d:1/1 line suggest that all the Ca and Mg originate from carbonate dissolution, giving a Ca + Mg/HCO3 ratio of approximately 1.0 (Jia et al., 2020). The excess of Ca and Mg giving a ratio (Ca+Mg/HCO3 > 1) can be explained by an additional source of Ca and Mg ions, such as the replacement of Ca and Mg in the rock matrix by sodium and potassium ions in water (Luo et al., 2018). For the samples plotted below the d:1/1 line, reverse ion exchange is a possible process leading to a ratio (Ca+Mg/HCO3 < 1). Excess of HCO3 may result from indirect ion exchange involving the participation of carbonic acid (Elliott, 2007). In addition, the weathering of silicates is a frequent source of HCO3 ions which leads to a decrease in the Ca+Mg/HCO3 ratio (Mukherjee and Fryar, 2008; Saha et al., 2011). Following the analysis data, It is demonstrated that most points fall below the line d:1/1, suggesting that direct ion exchange is dominant over indirect ion exchange, and the situation of points far from the line reveals that limestone and dolomite minerals are not the only source of calcium and magnesium in waters.
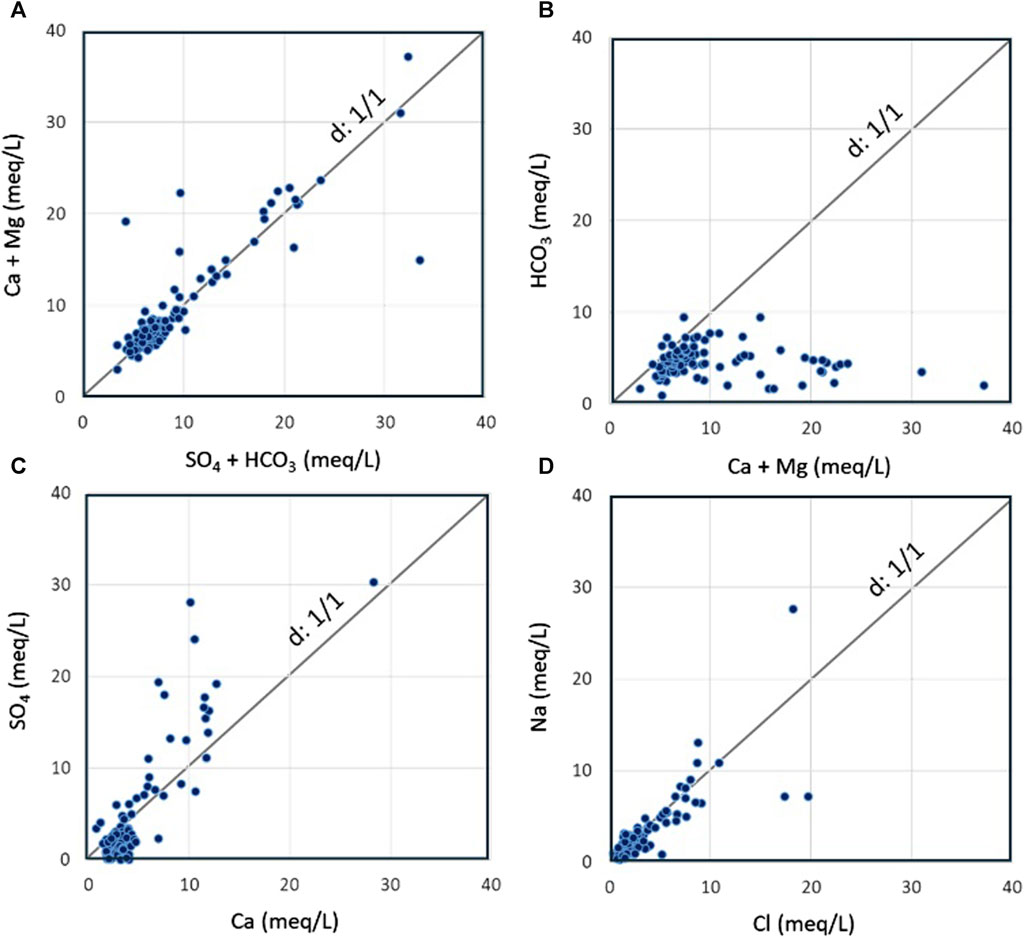
FIGURE 8. Distribution diagrams used to characterize the groundwater in Fritissa area, (A,B) Checking of ion exchange processes, (C) Contribution of gypsum and anhydrite minerals, and (D) Contribution of halite minerals to groundwater chemistry.
Dissolution-precipitation of minerals is a possible process that affects the water chemistry, and it is assessed by plotting the water samples in the SO4 vs. Ca diagram (Figure 8C), which takes into account the fact that the evaporitic minerals occur in many levels of the Fritissa aquifers. The possible dissolution of gypsum and anhydrite is confirmed for 27 points that fit on the line d:1/1. Excess of calcium over sulfate is observed in 57 samples, while excess of sulfate is detected in 10 points. On the other hand, the Na vs. Cl plot (Figure 8D) is used to examine how halite dissolution affects the hydrochemistry of water. The graph shows the distribution of 44 samples close to the d:1/1 line indicating that halite is significantly a potential source of dissolved sodium and chloride ions. Excess of chloride is detected in 42 points, while excess of sodium is revealed in 8 samples only. Consequently, other sources of sodium and chloride are probable for samples falling far from the equilibrium line.
5.5 Groundwater suitability for irrigation purposes
The assessment of groundwater suitability for irrigation is performed by using the Wilcox (1955) diagram that compares water electrical conductivity to sodium percentage and delineates five water quality zones. Results are illustrated in Figure 9 that shows that most of samples are mainly falling in the excellent to good class and secondarily in good to permissible class. One sample shows doubtful to unsuitable conditions. Due to the fact that the Wilcox diagram does not take in consideration the other elements contributing to the total salinity, such as calcium and magnesium, frequent in carbonate rocks in the region, it is interesting to assess the water suitability by using the US Salinity Hazard diagram that uses electrical conductivity and SAR values, and determines water classes according to the levels of sodium hazard and global salinity threat (Richards et al., 1954). Figure 10 illustrates the classification result, where three groundwater categories are defined: (i) the S1C1 class that corresponds to low sodium hazard level and low global salinity threat, and concerns four samples only; (ii) the S1C2 class with low sodium hazard and moderate conductivity (250–750 μS/cm), characterizing most of samples (75.53%); (iii) the S1C3 class that designates conditions of low sodium hazard but high global salinity threat, revealed for eighteen samples (19.15%). One sample shows exceptional conditions and belongs to the S3C3 class with high sodium hazard and high salinity threat.
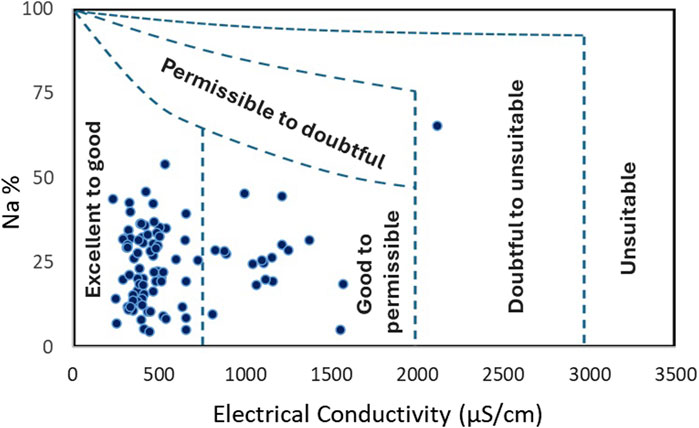
FIGURE 9. Irrigation groundwater quality based on the Wilcox (1955) classification.
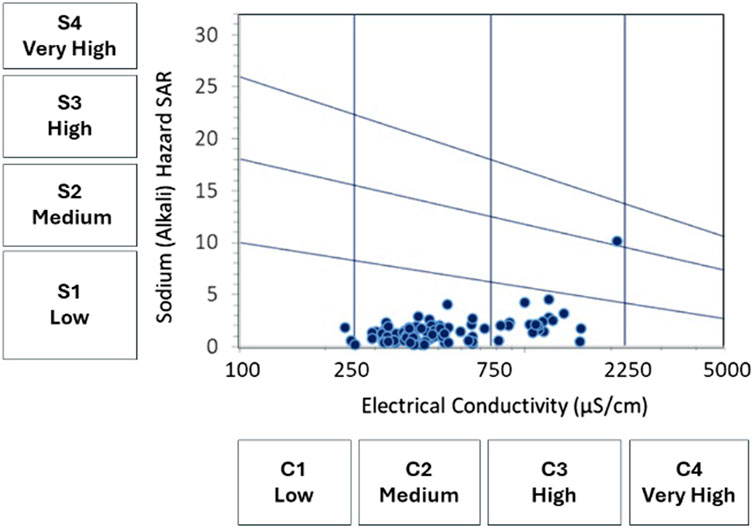
FIGURE 10. Irrigation groundwater quality based on the US Salinity Hazard diagram after Richards et al. (1954).
5.6 Discussion
The results obtained in this study make it possible to highlight several characteristics of the region, and to recommend measures and precautions to be taken for the secure use of groundwater. On a socio-economic level, it is noted that demographic development is increasing in the region, but water resources remain limited under pressing climatic conditions. It is expected that the demand for drinking water and irrigation water will be increasingly high with the expansion of built zones and cultivated lands, progressively replacing the bare lands, thanks to investments in regional agriculture.
Chemical analysis of groundwater has revealed the diversity of its chemical facies, resulting from numerous water-rock interaction processes that control the distribution of dissolved elements in water, including direct and indirect ion exchange, reverse ion exchange, evaporation, and possibly dissolution-precipitation, such is the case in various arid and semi-arid regions of Morocco (Ettazarini, 2004; Ettazarini, 2005; Ettazarini, 2006; Bahaj et al., 2013; Ait Brahim et al., 2015; Kassou et al., 2016; Al Yacoubi et al., 2017; Ettazarini and El Jakani, 2017; Ait Lemkademe et al., 2023; Laghrib et al., 2023).
The possible contribution of gypsum dissolution confirmed for 27 samples and halite dissolution for 44 samples supports the geological control of water chemistry through evaporitic rocks. Anterior works demonstrated that the risk of marine submersion is limited to the coastal zone, up to 10 km far from the coastline (Ramdani et al., 2021). In addition, other studies provided geophysical data, targeting groundwater salinisation, showed that salinisation is limited to the coastal area, and it is mainly caused by evaporitic deposits after a deepening of water level in the phreatic aquifer (Boughriba et al., 2006). Therefore, the hypothesis of seawater as source of halite is excluded.
The quality indices used in this study target several aspects of water behavior when used for irrigation. Considering the values of pH (5.8–8.8 ±0.1) and TDS (367 ±1 mg/L—3301 ±1 mg/L) in the studied waters, it is convenient to consider the pH and global salinity levels and compare them to the tolerance level of plants to these two parameters. Crops productivity largely depends on the salinity in both soils and irrigation water (Grattan, 2002; Ezlit et al., 2010). High salinity can also cause the clogging of voids and a reduction in the porosity of soils, and thus reduce their aeration conditions (Gonçalves et al., 2010; Ahamefule et al., 2019). Indeed, high salinity can affect plants and cause saline soil conditions, while excess sodium affecting the soil leads to sodic soil conditions (Fipps, 2021). The hardness values in mg/L as CaCO3 (15 ±1 mg/L—186 ±1 mg/L) encourage reflection on the use of this water. Indeed, soft water with hardness less than 50 mg/L is not necessarily beneficial for plants, but the desired hardness is between 50 and 150 mg/L. When hardness exceeds 300 mg/L, there is a long-term risk of foliar deposits, damage to irrigation systems and clogging of pipes (Schiavon and Moore, 2021).
RSC values revealed in (−35.2−7.9 ±0.1 meq/L) range depend on carbonates and bicarbonates concentration that influence the suitability of water to irrigation purposes (Amadi et al., 2019). Waters with RSC upper than 1.25 are not observed among the studied samples. Over the 2.5 threshold, water is of fair quality and the risk of bringing sodium carbonates after precipitation of alkaline-earthy carbonates occurs (Servant, 1978). Indeed, the calculation of the permeability index PI helps to predict the behavior of the soil after prolonged use of irrigation water (Easton, 1950; Doneen, 1964; Singh et al., 2015). Based on the results obtained for the PI values (7.7–72), it is remarked that waters from 92.55% of samples are good for long-term irrigation use. These can be used without any threat if moderate amount of leaching occurs (Doneen, 1964; Ragunath, 1987), while the remaining 7.45% are unsatisfactory and should be used with caution. These are non-suitable for feebly drained soils (Doneen, 1964; Ragunath, 1987).
Combining TDS, EC and SAR variables is a common way for evaluating the groundwater suitability for irrigation purposes. The classification of groundwater according to Wilcox diagram revealed two main classes suggesting that waters are of excellent to good type and good to permissible type, except one sample that is ranged in doubtful to unsuitable category and that will be discussed later. The US Salinity classification of Fritissa groundwater showed low sodium hazard (S1) but progressing salinity levels (C1, C2 and C3). According to the US Salinity Laboratory guidelines (Fipps, 2021), low sodium water (S1) is suitable for almost all soils, but caution is demanded for sodium-sensitive crops. Low salinity water (C1) is suitable for most crops on most soils with little leaching required. Caution is recommended for soils of extremely low permeability. Medium salinity water (C2) is usable when moderate amount of leaching occurs, and for salinity-tolerant crops. High salinity water (C3) is risky for soils with restricted drainage. Management for salinity control may be required in this case. Only crops presenting a good salinity tolerance can be considered. The sample making exception with highest sodium hazard and highest salinity is classified in S3C3 category. The same sample is revealed as doubtful to unsuitable by the Wilcox classification. It shows high amounts of sodium, chloride and sulfate, and its water facies is of Na-K-SO4-Cl type. The sample is taken from a superficial water level (12.6 m depth) and its location falls on the bare zone, but near a waterway downstream of built area. These remarks suggest possible contamination of waters caused by wastewater.
Furthermore, the spatial distribution of nitrates and nitrites is compared to the land use map (Figure 11) where it is realized that sample points showing amounts of nitrites and/or nitrates are localized either in agricultural areas, where fertilizers are used to increase soil fertility, or downstream of built zones, where household waste and wastewaters are frequently rejected. These observations support the possible anthropic source of contamination. Thus, anthropic influence should be considered to explain the water chemistry in regions where wastewaters are directly projected in the natural environment.
6 Conclusion
The chemical analysis of groundwater from Fritissa region aimed at the determination of main water characters and the assessment of its suitability for irrigation purposes. The approach is based on the analysis of major ions (Ca, Mg, Na, K, HCO3, CO3, Cl, SO4, NO3, NO2) concentrations, pH values and the calculation of many common quality indices, notably total dissolved solids TDS, total hardness TH, sodium percentage Na%, residual sodium carbonate RSC, sodium adsorption ratio SAR, and permeability index PI. The results revealed that the region, of growing population, undergoes pressing constraints caused by increasing demands on drink and irrigation waters, coupled with a significant scarcity of water resources under arid and semiarid climate conditions. The study demonstrated variable characters of water chemistry in the region of interest. Indeed, waters are of Ca-Mg-HCO3, Ca-Mg-SO4-Cl and mixed facies types. Distribution graphs revealed many possible water-rock interaction processes controlling the water chemistry, such as ion exchange, reverse ion exchange, direct ion exchange, indirect ion exchange, in addition to evaporation and possible dissolution-precipitation processes.
The assessment of water suitability for irrigation purposes is performed by two common classifications: The classification of Wilcox (1955) that revealed excellent to good and good to permissible categories, as dominant in the region; and the classification of Richards et al. (1954) adopted by the United States Salinity Laboratory, and that provided more precisions and helped to distinguish three classes: S1C1, S1C2, and S1C3 for which some caution and measures to consider are determined. The two classifications were able to detect one sample making exception, whose quality is probably deteriorated by household waste and wastewaters. The anthropic impact is then demonstrated by the location of the water points contaminated with nitrates and nitrites in agricultural zones and near residential areas. Thus, the good understanding of water chemical characters and the factors controlling their evolution, in time and space, necessitate the consideration of anthropic impact in addition to natural common processes.
Data availability statement
The original contributions presented in the study are included in the article, further inquiries can be directed to the corresponding author.
Author contributions
MB: Data curation, Funding acquisition, Methodology, Project administration, Resources, Writing–original draft. AI: Methodology, Project administration, Writing–review and editing. SE: Methodology, Supervision, Validation, Visualization, Writing–review and editing. YH: Supervision, Validation, Visualization, Writing–review and editing. LB: Methodology, Project administration, Writing–review and editing. ME: Supervision, Visualization, Writing–review and editing. SC: Formal Analysis, Methodology, Software, Writing–review and editing.
Funding
The author(s) declare that no financial support was received for the research, authorship, and/or publication of this article.
Conflict of interest
The authors declare that the research was conducted in the absence of any commercial or financial relationships that could be construed as a potential conflict of interest.
Publisher’s note
All claims expressed in this article are solely those of the authors and do not necessarily represent those of their affiliated organizations, or those of the publisher, the editors and the reviewers. Any product that may be evaluated in this article, or claim that may be made by its manufacturer, is not guaranteed or endorsed by the publisher.
References
Agency of Hydraulic Basin of Moulouya (2022). Carte des isohyètes de la pluviomètrie de Moulouya. Rapport interne inédit.
Ahamefule, H. E., Olaniyan, J. O., Eifediyi, E. K., Izuogu, B. N., Amana, M. S., Nwokocha, C. C., et al. (2019). Effluents in irrigation water: a case study of Asa River on farmlands in Ilorin, Nigeria. Bulg. J. Soil Sci. Agrochem. Ecol. 53, 2–2019.
Ait Brahim, L., and Chotin, P. (1990). Oriental Moroccan Neogene volcanism and strike-slip faulting. J. Afr. Earth Sci. (and Middle East) 11 (3–4), 273–280. doi:10.1016/0899-5362(90)90005-Y
Ait Brahim, Y., Benkaddour, A., Agoussine, M., Ait Lemkademe, A., Yacoubi, L. A., and Bouchaou, L. (2015). Origin and salinity of groundwater from interpretation of analysis data in the mining area of Oumjrane, Southeastern Morocco. Environmental Earth Sciences 74, 4787–4802. doi:10.1007/s12665-015-4467-7
Ait Lemkademe, A., El Ghorfi, M., Zouhri, L., Heddoun, O., Khalil, A., and Maacha, L. (2023). Origin and salinization processes of groundwater in the semi-arid area of zagora graben, southeast Morocco. Water 15, 2172. doi:10.3390/w15122172
Amadi, A. N., Tukur, A., Dan-Hassan, M. A., Okunlola, I. A., Shauibu, I., Egharevba, J. K., et al. (2019). Groundwater quality assessment for irrigation purposes: a case study of minna, Niger state, north-central Nigeria. J. Chem Soc. Nigeria 44 (4), 633–640.
Amadou, H., Laouali, M. S., and Manzola, A. S. (2014). Analyses physico-chimiques et bactériologiques des eaux de trois aquiferes de la region de tillabery: application des methodes d’analyses statistiques multi variees. Larhyss. Journal 20, 25–41.
Asiwaju-Bello, Y. A., Olabode, F. O., Duvbiama, O. A., Iyamu, J. O., Adeyemo, A. A., and Onigbinde, M. T. (2013). Hydrochemical evaluation of groundwater in Akure area. South-western Nigeria. for irrigation purpose. European International Journal of Science and Technology 2 (8), 235–249. http://www.cekinfo.org.uk/images/frontImages/Vol._2_No._8/21.pdf.
Ayer, R. S., and Westcot, D. W. (1985). Water quality for agriculture. Irrigation and drainage paper 29. Rome: FAO, 97p.
Bahaj, T., Kacimi, I., Hilali, M., Kassou, N., and Mahboub, A. (2013). Preliminary study of the groundwater geochemistry in the sub-desert area in Morocco: case of the ziz-ghris basins. Procedia Earth and Planetary Science 7, 44–48. doi:10.1016/j.proeps.2013.03.162
Bernini, M., Boccaletti, M., Moratti, G., and Papani, G. (2000). Structural development of the taza-guercif basin as a constraint for the Middle Atlas shear zone tectonic evolution. Marine and Petroleum Geology 17 (3), 391–408. doi:10.1016/S0264-8172(99)00042-2
Boughriba, M., Melloul, A., Zarhloule, Y., and Ouardi, A. (2006). Extension spatiale de la salinisation des ressources en eau et modèle conceptuel des sources salées dans la plaine des Triffa (Maroc nord-oriental). Comptes Rendus Geoscience 338, 768–774. doi:10.1016/j.crte.2006.07.007
Cerling, T. E., Pederson, B. L., and Von Damm, K. L. (1989). Sodium-calcium ion exchange in the weathering of shales. Implications for global weathering budgets. Geology 17, 552–554. doi:10.1130/0091-7613(1989)017<0552:SCIEIT>2.3.CO;2
Coetsiers, M., and Walraevens, K. (2006). Chemical characterization of the Neogene aquifer, Belgium. Hydrogeol J 14, 1556–1568. doi:10.1007/s10040-006-0053-0
Combe, M., and Simonot, M. (1971). “La moyenne Moulouya,” in Ressources en eau du Maroc. Tome 1- Domaine du Rif et du Maroc Oriental. Edition service géologique Maroc, Rabathttps://fr.scribd.com/document/496857679/Ressources-en-Eau-du-MAROC-Tome-I.
Consultative Commission of Regionalization CCR (2011). Rapport sur le régionalisation avancée. Livre1: conception générale. Rabat. http://www.regionalisationavancee.ma/PDF/Rapport/Fr/L1_ConceptionGenerale.pdf.
Doneen, L. D. (1964). Notes on water quality in agriculture. Published as a water science and engineering paper 4001. Davis: Department of Water Science and Engineering, University of California, 48.
Easton, F. M. (1950). Significance of carbonate in irrigation water. Soil Science 67 (3), 128–133. doi:10.1097/00010694-195002000-00004
El Kati, I., Benammi, M., Tabyaoui, H., Ouabid, M., and Benammi, M. (2022). Structural style and deformation mechanism of Neogene series of the Guercif basin (NE-Morocco). J Iber Geol 48, 281–296. doi:10.1007/s41513-022-00196-1
Elliott, S. S. (2007). Demonstration of the carbonic acid exchange theory: employing magnets and different colored paperclips. The American Biology Teacher 69 (6), 331. doi:10.2307/4452172
Ettazarini, S. (2004). Incidences of water-rock interaction on natural resources characters. Oum Er-rabia basin, Morocco. Environmental Geology 47 (1), 69–75. doi:10.1007/s00254-004-1129-6
Ettazarini, S. (2005). Processes of water-rock interaction in the Turonian aquifer of Oum Er-rabia basin, Morocco. Environmental Geology 49, 293–299. doi:10.1007/s00254-005-0088-x
Ettazarini, S. (2006). Mapping of groundwater quality in the Turonian aquifer of Oum Er-Rabia Basin, Morocco: a case study. Environ Geol 50 (6), 919–929. doi:10.1007/s00254-006-0263-8
Ettazarini, S. (2011). GIS-based multi-source database, a strategic tool for sustainable development planning: case of Qalaat Mgouna, Morocco. Environ Earth Sci 62 (7), 1437–1445. doi:10.1007/s12665-010-0629-9
Ettazarini, S., and El Jakani, M. (2017). Assessment of water suitability for irrigation purposes in Qalaat Mgouna area, Morocco. Am. Journ. of Innov. Res. and Appl. Sc. 4 (5), 126–135. ISSN:2429-5396.
Ezlit, Y. D., Smith, R. J., and Raine, S. R. (2010). A review of salinity and sodicity in irrigation. Cooperative research Center for irrigation futures irrigation matters series No. 01/10.
Fantong, W. Y., Satake, H., Ayonghe, S. N., Aka, F. T., and Asai, K. (2009). Hydrogeochemical controls and usability of groundwater in the semi-arid Mayo Tsanaga River Basin: far north province, Cameroon. Environ Geol 58, 1281–1293. doi:10.1007/s00254-008-1629-x
Fipps, G. (2021). Irrigation water quality standards and salinity management strategies. Texas, United States: Texas A&M University. EB-1667. 18p.
Fisher, R. S., and Mullican III, W. F. (1997). Hydrochemical evolution of sodium-sulfate and sodium-chloride groundwater beneath the northern chihuahuan desert, trans-pecos, Texas, USA. Hydrogeology Journal 5 (2), 4–16. Available:. doi:10.1007/s100400050102
Gaofeng, Z., Yonghong, S., Chunlin, H., Qi, F., and Zhiguang, L. (2009). Hydrogeochemical processes in the groundwater environment of Heihe River Basin, northwest China. Environ Earth Sci 60, 139–153. doi:10.1007/s12665-009-0175-5
Geological Service of Morocco GSM (1964a). Geological map of Morocco, sheet of hassi El ahmar 1/100 000 scale. Rabat, Morocco: Ministère de l’Energie et des Mines.
Geological Service of Morocco GSM (1964b). Geological map of Morocco, sheet of maatarka 1/100 000 scale. Rabat, Morocco: Ministère de l’Energie et des Mines.
Geological Service of Morocco GSM (1972a). Geological map of Morocco, sheet of Debdou 1/100 000 scale. Rabat, Morocco: Ministère de l’Energie et des Mines.
Geological Service of Morocco GSM (1972b). Geological map of Morocco, sheet of hassian ed diab 1/100 000 scale. Rabat, Morocco: Ministère de l’Energie et des Mines.
Geological Service of Morocco GSM (1985). Geological map of Morocco 1/1 000 000 scale, 260. Rabat, Morocco: Ministère de l’Energie et des Mines.
Geological Service of Morocco GSM (1991). Geological map of Morocco, sheet of ain bni mathar 1/100 000 scale. Rabat, Morocco: Ministère de l’Energie et des Mines.
Geological Service of Morocco GSM (1995). Geological map of Morocco, sheet of Taourirt 1/100 000 scale. Rabat, Morocco: Ministère de l’Energie et des Mines.
Gharmane, Y., Hinaje, S., Amrani, S., El Fartati, M., and Yaagoub, D. (2019). Role of the chronology of the tectonic phases in the distributionof hydrothermal mineralizations in the mining district ofTazekka (Morocco). Journal of Engineering Research and Application 9 (6), 49–62.
Gibbs, R. J. (1970). Mechanisms controlling world water chemistry. Science 170 (3962), 1088–1090. doi:10.1126/science.170.3962.1088
Gonçalves, R. A. B., Gloaguen, T. V., Folegatti, M. V., Libardi, P. L., Lucas, Y., and Montes, C. R. (2010). Pore size distribution in soils irrigated with sodic water and wastewater. Revista Brasileira de Ciência do Solo 34, 701–707. doi:10.1590/s0100-06832010000300011
Grattan, S. R. (2002). Irrigation, water salinity and crop production. California, United States: University of California, Publication n° 8066. 10p. Available at: http://anrcatalog.ucdavis.edu.
Handa, B. K. (1969). Chemical composition of monsoon rains over Calcutta. Part II. Tellus 21 (1), 101–106. doi:10.1111/j.2153-3490.1969.tb00422.x
Ishaku, J. M., Ahmed, A. S., and Abubakar, M. A. (2011). Assessment of groundwater quality using chemical indices and GIS mapping in Jada area. Northeastern Nigeria. Journal of Earth Sciences and Geothechnical Engineering 1 (1), 35–60.
James, D. W., Hanks, R. J., and Jurinak, J. H. (1982). Modern irrigated soils. USA: John Wiley and Sons NY.
Kassou, N., Bahaj, T., Morarech, M., Hejja, Y., Hilali, M., Baidder, L., et al. (2016). Assessment of groundwater chemistry for the Reg basin (Oriental Anti-Atlas) south-eastern Morocco. Environ. Sci. 7 (12), 4511–4524.
Laghrib, F., Bahaj, T., El Kasmi, S., Hilali, M., Kacimi, I., Nouayti, N., et al. (2024). Hydrogeochemical study of groundwater in arid and semi-arid regions of the Infracenomanian aquifers (Cretaceous Errachidia basin, Southeastern Morocco). Using hydrochemical modeling and multivariate statistical analysis. Journal of African Earth Sciences 209 (209), 105132. doi:10.1016/j.jafrearsci.2023.105132
Latifa, A. Y., Lhoussaine, B., Etienne, J., Moussa, M., Yassine, A. B., Ahmed, E. M., et al. (2017). Impact of rock-water interactions and recharge on water resources quality of the Agadir-Essaouira basin, southwestern Morocco. Arab J Geosci 10, 169. doi:10.1007/s12517-017-2968-2
Luo, W., Gao, X., and Zhang, X. (2018). Geochemical processes controlling the groundwater chemistry and fluoride contamination in the Yuncheng Basin, China—an area with complex hydrogeochemical conditions. PloS ONE 13 (7), e0199082. doi:10.1371/journal.pone.0199082
Magaritz, M., and Luzier, E. (1985). Water-rock interactions and seawater freshwater mixing effects in the coastal dunes aquifer, Coos Bay, Oregon. Geochimica et Cosmochimica Acta 49, 2515–2525. doi:10.1016/0016-7037(85)90119-X
Mauritius Government Gazette (1999). Guidelines for irrigation water quality. General Notice n° 617(1999). Available at: https://faolex.fao.org/docs/pdf/mat53079.pdf.
Merkel, B. J., and Planer-Friedrich, B. (2002). “Groundwater geochemistry. A practical guide to modeling of natural and contaminated aquatic systems,” in Translated from the German version "grundwasserchemie". Editor D. K. Nordstrom Ed. (New York: Springer Berlin Heidelberg), 200.
Morgan, K., and Jankowski, J. (2004). Saline groundwater seepage zones and their impact on soil and water resources in the Spicers Creek catchment, central west, New South Wales, Australia. Environmental Geology 46, 273–285. doi:10.1007/s00254-004-0977-4
Mukherjee, A., and Fryar, A. E. (2008). Deeper groundwater chemistry and geochemical modeling of the arsenic affected western Bengal basin, West Bengal, India. Applied Geochemistry 23, 863–894. doi:10.1016/j.apgeochem.2007.07.011
Nadler, A., Magaritz, M., and Mazor, E. (1980). Chemical reactions of sea water with rocks and freshwater: experimental and field observations on brackish waters in Israel. Geochimica et Cosmochimica Acta 44, 879–886. doi:10.1016/0016-7037(80)90268-9
Nagaraju, A., Sunil Kumar, K., and Thejaswi, A. (2014). “Assessment of groundwater quality for irrigation: a case study from Bandalamottu lead mining area,” in Guntur district. Andhra pradesh. South India (Article in Applied Water Science), 385–396. doi:10.1007/s13201-014-0154-1
Nagaraju, A., Surresh, S., Killam, K., and Hudson-Edwards, K. (2006). Hydrogeochemistry of waters of mangampeta barite mining area, cuddapach basin, Andhra Pradesh, India. Turk J Eng Environ Sci 30, 203–219.
Naseem, S., Hamza, S., and Bashir, E. (2010). Groundwater geochemistry of winder agricultural farms, balochistan, Pakistan and assessment for irrigation water quality. Athens, Greece: European Water Resources Association EWRA. Available at: https://ewra.net/ew/pdf/EW_2010_31_03.pdf.
Nayak, K. M., and Sahoo, H. K. (2014). Hydrogeochemical evaluation of mahanga block. Cuttack district. Odisha. India. Journal of Geosciences and Geomatics 2 (5), 16–21. doi:10.12691/jgg-2-5A-4
Piper, A. M. (1944). A graphic procedure in the geochemical interpretation of water-analyses. Trans. AGU 25 (6), 914. doi:10.1029/TR025i006p00914
Richards, A., Allison, L. E., Bernstein, C., Bower, L. A., Brown, J. W., Fireman, M., et al. (1954). United States salinity laboratory staff. Agriculture Handbook: United States Departement of Agriculture, 60.
Rodier, J., Geoffray, Ch., Kovacsik, G., Laporte, J., Verneau, J., and Vial, J. (1975). L’analyse de l’eau. Eaux naturelle, eaux résiduelle, eaux de mer. Tome 1. Paris: DUNOD Edition, 629.
Salem, Z. E. S., Fathy, M. S., Helal, A. F. I., Afifi, S. Y., and Attiah, A. M. (2021). “Groundwater quality for irrigation as an aspect of sustainable development approaches: a case study of semi-arid area around ismailia canal, eastern nile delta, Egypt,” in Groundwater in Egypt’s deserts. Editors A. Negm,, and A. Elkhouly (Cham: Springer Water. Springer). doi:10.1007/978-3-030-77622-0_12
Sani, F., Zizi, M., and Bally, A. W. (2000). The Neogene–Quaternary evolution of the Guercif Basin (Morocco) reconstructed from seismic line interpretation. Marine and Petroleum Geology 17 (3), 343–357. doi:10.1016/S0264-8172(99)00058-6
Schiavon, M., and Moore, K. K. (2021). How to properly read your irrigation water analysis for turf and landscape: ENH1352/ep616, 12/2021. EDIS 2021 (6). doi:10.32473/edis-ep616-2021
Singh, S. K., Srivastava, P. K., Singh, D., Han, D., Gautam, S. K., and Pandey, A. C. (2015). Modeling groundwater quality over a humid subtropical region using numerical indices, earth observation datasets, and X-ray diffraction technique: a case study of allahabad district, India. Environ. Geochem. Health. 37, 157–180. doi:10.1007/s10653-014-9638-z
United State Environmental Protection Agency (USEPA) (1976). Quality criteria for water. EPA 440-9-76-023.
Wilcox, L. V. (1955). United States salinity laboratory. Circular No. 969. Washington, United States: United States Department of Agriculture.
Yaagoub, D., Hinaje, S., and El Fartati, M. (2023). Analyse structurale et reconstitution des paléochamps de contraintes tardi-hercyniens dans la boutonnière d’Aouli (massif de la Haute Moulouya, Maroc). Estudios Geológicos 79 (1), e149. doi:10.3989/egeol.44749.620
Keywords: groundwater quality, water chemistry, water-rock interaction, Fritissa, sustainability, Moulouya
Citation: Ben Driss MA, Ifliliss A, Ettazarini S, Hahou Y, Boudad L, El Amrani M and Courba S (2024) Assessment of groundwater suitability for irrigation purposes in the Fritissa region, Moulouya, Morocco. Front. Geochem. 2:1307252. doi: 10.3389/fgeoc.2024.1307252
Received: 04 October 2023; Accepted: 24 January 2024;
Published: 14 February 2024.
Edited by:
Israel Labastida, Metropolitan Autonomous University, MexicoReviewed by:
Ruth Esther Villanueva Estrada, National Autonomous University of Mexico, MexicoCarleton Bern, Colorado Water Science Center (USGS), United States
Copyright © 2024 Ben Driss, Ifliliss, Ettazarini, Hahou, Boudad, El Amrani and Courba. This is an open-access article distributed under the terms of the Creative Commons Attribution License (CC BY). The use, distribution or reproduction in other forums is permitted, provided the original author(s) and the copyright owner(s) are credited and that the original publication in this journal is cited, in accordance with accepted academic practice. No use, distribution or reproduction is permitted which does not comply with these terms.
*Correspondence: Moulay Ahmed Ben Driss, YWhtZWQuYmVuZHJpc3NAdW01ci5hYy5tYQ==