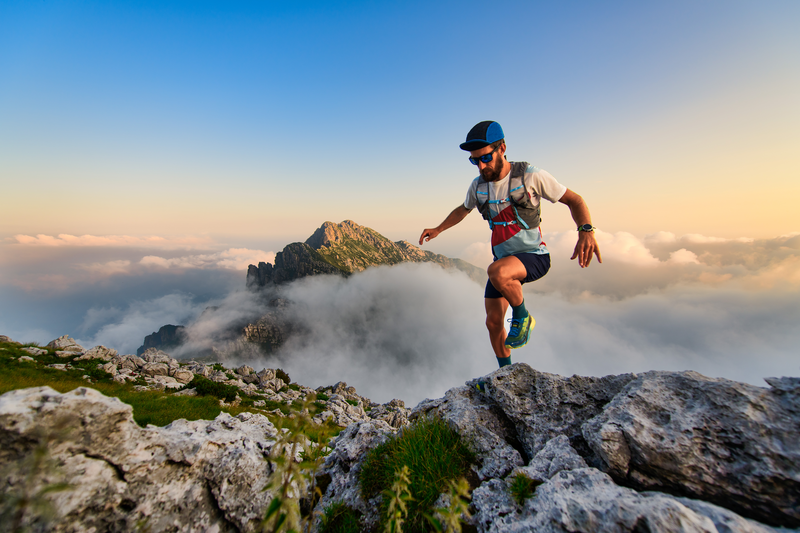
94% of researchers rate our articles as excellent or good
Learn more about the work of our research integrity team to safeguard the quality of each article we publish.
Find out more
REVIEW article
Front. Genet.
Sec. RNA
Volume 16 - 2025 | doi: 10.3389/fgene.2025.1556495
The final, formatted version of the article will be published soon.
You have multiple emails registered with Frontiers:
Please enter your email address:
If you already have an account, please login
You don't have a Frontiers account ? You can register here
Idiopathic Pulmonary Fibrosis (IPF) is a progressive interstitial lung disease characterized by unknown etiology and limited therapeutic options. Recent studies implicate exosomal non-coding RNAs (ncRNAs) as crucial regulators in IPF. These ncRNAs, including long non-coding RNAs (lncRNAs), mi-croRNAs (miRNAs), and circular RNAs (circRNAs), are involved in cellular processes through various mechanisms of selective packaging, intercellular communication, and signaling pathway integration. lncRNAs such as LINC00470 and PVT1 exhibit pro-fibrotic effects, while others like lnc-DC and THRIL show inhibitory roles; some, including UCA1 and MALAT1, demonstrate bidirectional regulation. In miRNAs, pro-fibrotic agents (e.g., miR-486, miR-223) contrast with inhibitory miRNAs (e.g., miR-34a, miR-126), while miR-21 and miR-155 display dual functions. Similarly, circRNAs such as circ_0000479 and circ_0026344 promote fibrosis, whereas circ_0000072 and circ_0000410 act as inhibitors, with certain circRNAs (e.g., circ_002178 and circ_0001246) exhibiting complex regulatory effects. Exosomal ncRNAs modulate key pathways, including TGF-β and Wnt/β-catenin, influencing IPF 设置了格式: 字体: (默认) Arial 带格式的: 居中, 缩进: 首行缩进: 0 字符 设置了格式: 字体: (默认) Times New Roman 带格式的: 居中 带格式的: 左 设置了格式: 字体: (默认) Arial progression. Despite their potential, challenges remain in exosome isolation, functional characterization of ncRNAs, and clinical translation. Addressing these barriers through innovative research strategies is essential to leverage exosomal ncRNAs in the management and treatment of IPF. This review comprehensively examines the roles of exosomal ncRNAs in IPF, elucidates their mechanisms and pathway interactions, and discusses future perspectives to enhance understanding and therapeutic strategies for this disease.
Keywords: Idiopathic Pulmonary Fibrosis, Exosomes, non-coding RNAs, function, review
Received: 08 Jan 2025; Accepted: 10 Mar 2025.
Copyright: © 2025 Wei, Hong, Zhu and Li. This is an open-access article distributed under the terms of the Creative Commons Attribution License (CC BY). The use, distribution or reproduction in other forums is permitted, provided the original author(s) or licensor are credited and that the original publication in this journal is cited, in accordance with accepted academic practice. No use, distribution or reproduction is permitted which does not comply with these terms.
* Correspondence:
Feng Li, Sixth People's Hospital of Nantong, Nantong, China
Disclaimer: All claims expressed in this article are solely those of the authors and do not necessarily represent those of their affiliated organizations, or those of the publisher, the editors and the reviewers. Any product that may be evaluated in this article or claim that may be made by its manufacturer is not guaranteed or endorsed by the publisher.
Research integrity at Frontiers
Learn more about the work of our research integrity team to safeguard the quality of each article we publish.