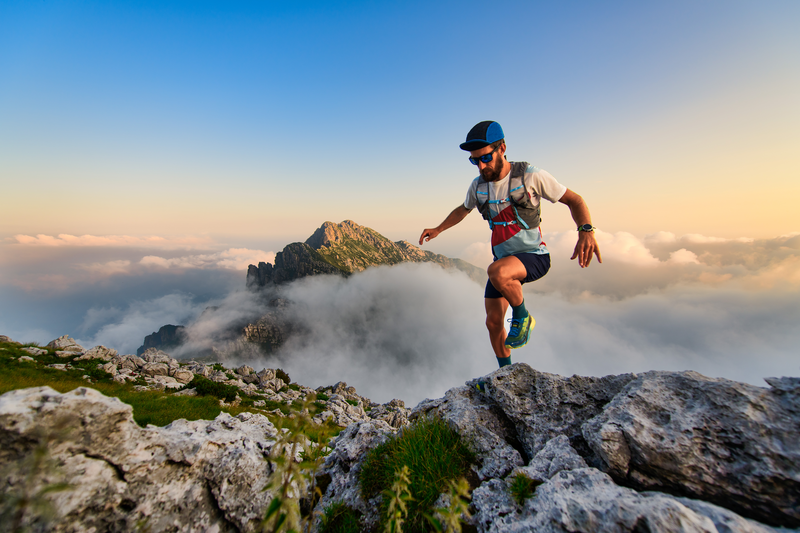
94% of researchers rate our articles as excellent or good
Learn more about the work of our research integrity team to safeguard the quality of each article we publish.
Find out more
CASE REPORT article
Front. Genet.
Sec. Genetics of Common and Rare Diseases
Volume 16 - 2025 | doi: 10.3389/fgene.2025.1554624
The final, formatted version of the article will be published soon.
You have multiple emails registered with Frontiers:
Please enter your email address:
If you already have an account, please login
You don't have a Frontiers account ? You can register here
Hereditary hemorrhagic telangiectasia (HHT) is an autosomal dominant vascular disorder caused by pathogenic variants in genes within the transforming growth factor beta signaling pathway, such as ACVRL1, leading to haploinsufficiency. Homozygous variants in HHT-related genes are exceptionally rare and, to date, have not been reported in ACVRL1-related HHT. We report the first known instance of a novel homozygous missense variant in the ACVRL1 gene (c.576C>G; p.Phe192Leu), identified in two siblings from a family of seven, in which three heterozygotes were also present. Comprehensive clinical evaluations revealed striking phenotypic differences between homozygous and heterozygous family members. Both homozygous individuals exhibited early-onset pulmonary arterial hypertension and diffuse pulmonary arteriovenous malformations. One of them also demonstrated childhood-onset gastrointestinal bleeding—a manifestation unprecedented in HHT, as this typically has a late adulthood onset. In contrast, heterozygotes displayed either mild or equivocal features of HHT, supporting the classification of this variant as a hypomorphic allele. The novel missense variant is located within the intracellular Glycine-Serine (GS) domain of the protein, suggesting a potential impact on receptor regulation and downstream signaling. While these findings expand the phenotypic spectrum of ACVRL1-related HHT, they remain limited to clinical observation. Experimental studies, including functional and molecular assays, will be essential to confirm the pathogenic impact of this variant, validate its classification as a hypomorphic allele, and further elucidate its effects on BMP-TGF-β signaling.
Keywords: Hereditary hemorrhagic telangiectasia (HHT), ACVRL1 gene mutation, Pulmonary arteriovenous malformations (PAVM), hypomorphic allele, genotype-phenotype correlation
Received: 02 Jan 2025; Accepted: 20 Feb 2025.
Copyright: © 2025 Mathavan, Mathavan, Krekora, Rao, Zumberg, Justice, Bayrak-Toydemir, McDonald and Ataya. This is an open-access article distributed under the terms of the Creative Commons Attribution License (CC BY). The use, distribution or reproduction in other forums is permitted, provided the original author(s) or licensor are credited and that the original publication in this journal is cited, in accordance with accepted academic practice. No use, distribution or reproduction is permitted which does not comply with these terms.
* Correspondence:
Ali Ataya, University of Florida, Gainesville, United States
Disclaimer: All claims expressed in this article are solely those of the authors and do not necessarily represent those of their affiliated organizations, or those of the publisher, the editors and the reviewers. Any product that may be evaluated in this article or claim that may be made by its manufacturer is not guaranteed or endorsed by the publisher.
Research integrity at Frontiers
Learn more about the work of our research integrity team to safeguard the quality of each article we publish.