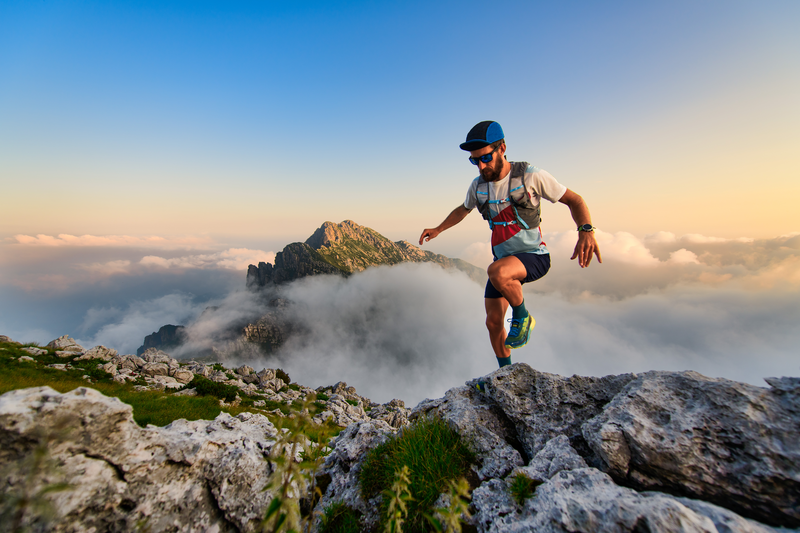
94% of researchers rate our articles as excellent or good
Learn more about the work of our research integrity team to safeguard the quality of each article we publish.
Find out more
MINI REVIEW article
Front. Genet.
Sec. Genetics of Common and Rare Diseases
Volume 16 - 2025 | doi: 10.3389/fgene.2025.1535732
This article is part of the Research Topic Insights in Genetics of Common and Rare Diseases 2024 View all 11 articles
The final, formatted version of the article will be published soon.
You have multiple emails registered with Frontiers:
Please enter your email address:
If you already have an account, please login
You don't have a Frontiers account ? You can register here
Congenital heart disease (CHD) is the most common type of birth defect and results from anomalies in the cardiogenesis process. There are multiple genetic mechanisms contributing to CHD, including copy number variants (CNVs). One such CNV is the 15q11.2 (BP1-BP2) microdeletion, which contains four evolutionarily conserved genes: NIPA1, NIPA2, CYFIP1 and TUBGCP5. The deletion causes a syndrome which includes developmental delays and multiple anatomical malformations including CHD.The link between the 15q11.2 (BP1-BP2) microdeletion and CHD has been previously described in the literature but not explored in terms of mechanistic investigations. The characteristics of the BP1-BP2 deletion also prove challenging in the context of genetic counselling. Here we discuss the 15q11.2 (BP1-BP2) microdeletion syndrome with a focus on CHD.
Keywords: congenital heart disease, Genetics, development, BP1-BP2/15q11.2 deletion syndrome, copy number variant, Phenotypic heterogeneity, Low penetrance
Received: 27 Nov 2024; Accepted: 07 Mar 2025.
Copyright: © 2025 Fifirig, Abraham, Keavney and Hentges. This is an open-access article distributed under the terms of the Creative Commons Attribution License (CC BY). The use, distribution or reproduction in other forums is permitted, provided the original author(s) or licensor are credited and that the original publication in this journal is cited, in accordance with accepted academic practice. No use, distribution or reproduction is permitted which does not comply with these terms.
* Correspondence:
Kathryn E Hentges, The University of Manchester, Manchester, United Kingdom
Disclaimer: All claims expressed in this article are solely those of the authors and do not necessarily represent those of their affiliated organizations, or those of the publisher, the editors and the reviewers. Any product that may be evaluated in this article or claim that may be made by its manufacturer is not guaranteed or endorsed by the publisher.
Research integrity at Frontiers
Learn more about the work of our research integrity team to safeguard the quality of each article we publish.