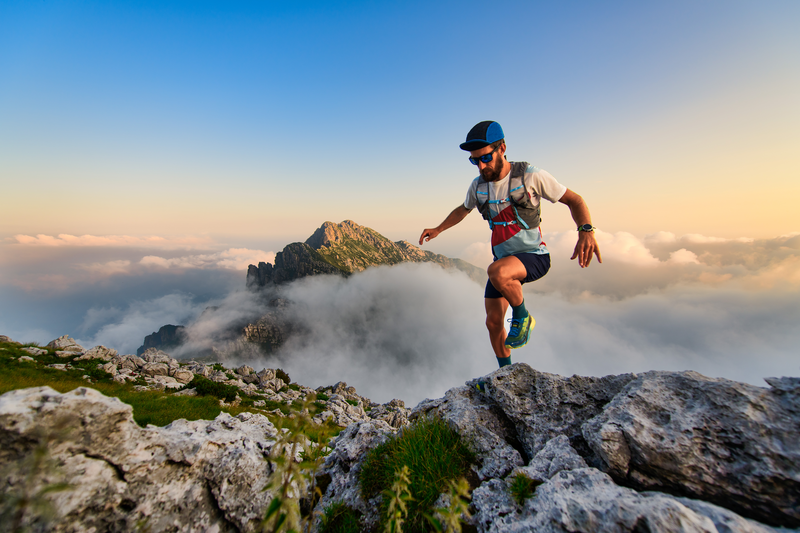
94% of researchers rate our articles as excellent or good
Learn more about the work of our research integrity team to safeguard the quality of each article we publish.
Find out more
ORIGINAL RESEARCH article
Front. Genet.
Sec. Genetics of Aging
Volume 16 - 2025 | doi: 10.3389/fgene.2025.1531832
This article is part of the Research Topic Rising Stars in Genetics of Aging View all 5 articles
The final, formatted version of the article will be published soon.
You have multiple emails registered with Frontiers:
Please enter your email address:
If you already have an account, please login
You don't have a Frontiers account ? You can register here
Background:Cockayne syndrome (CS) is a multisystem degenerative disorder in which dysplasia and microcephaly represent the primary criteria for diagnosis.we present the cases of two patients who exhibited distinctive facial features and a range of other clinical manifestations, including growth failure, developmental delay, microcephaly, dental anomalies, and unstable gait.Methods:Clinical information pertaining to the patient's family was collated and the Pedigree chart was drawn.Two milliliters of peripheral blood were drawn from each of the two patients (III1and III3) and their parents, The causative genes were identified by Medical exome sequencing. Furthermore, the pregnant women underwent amniotic fluid prenatal diagnosis at midpregnancy(III5). Results:Medical exome sequencing revealed that both patients had a homozygous deletion of Exon4 in the ERCC8 gene and that both parents were carriers. Prenatal diagnosis by amniotic fluid confirmed that the fetus (III5) did not carry the variant. Conclusion:This clarified the diagnosis at the genetic level, deepened our understanding of the disease, and facilitated the ability to provide accurate genetic counseling and prenatal diagnosis, with the goal of reducing the number of new affected individuals in the family 1
Keywords: Cockayne Syndrome1, Medical exome sequencing2, ERCC83, prenatal diagnosis4, rare inherited disorders5
Received: 21 Nov 2024; Accepted: 21 Feb 2025.
Copyright: © 2025 Ding, FEI, Zhao and Jin. This is an open-access article distributed under the terms of the Creative Commons Attribution License (CC BY). The use, distribution or reproduction in other forums is permitted, provided the original author(s) or licensor are credited and that the original publication in this journal is cited, in accordance with accepted academic practice. No use, distribution or reproduction is permitted which does not comply with these terms.
* Correspondence:
Hua Jin, Jinan Maternity And Child Care Hospital, Jinan, China
Disclaimer: All claims expressed in this article are solely those of the authors and do not necessarily represent those of their affiliated organizations, or those of the publisher, the editors and the reviewers. Any product that may be evaluated in this article or claim that may be made by its manufacturer is not guaranteed or endorsed by the publisher.
Research integrity at Frontiers
Learn more about the work of our research integrity team to safeguard the quality of each article we publish.