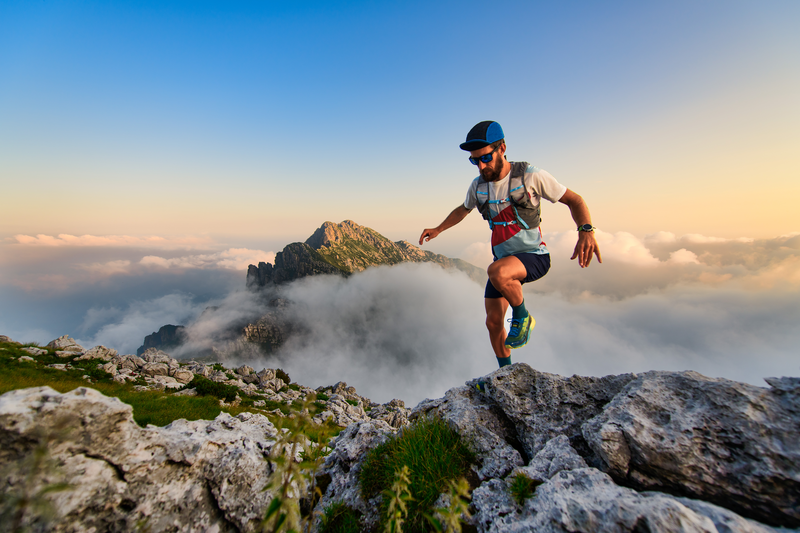
94% of researchers rate our articles as excellent or good
Learn more about the work of our research integrity team to safeguard the quality of each article we publish.
Find out more
REVIEW article
Front. Genet. , 03 February 2025
Sec. Genomics of Plants and the Phytoecosystem
Volume 16 - 2025 | https://doi.org/10.3389/fgene.2025.1523240
This article is part of the Research Topic Postharvest Ripening, Senescence, and Technology, Volume II View all 4 articles
Watermelon (Citrullus lanatus L.) fruit is widely consumed for its sweetness, flavor, nutrition and health-promoting properties. It is commonly commercialized in fresh-cut format, satisfying consumer demand for freshness and convenience, but its shelf-life is limited. Despite the potential for growth in fresh-cut watermelon sales, the industry faces the challenge of maintaining quality attributes during storage. Fresh-cut processing induces a series of physiological and biochemical events that lead to alterations in sensory, nutritional and microbiological quality. A signal transduction cascade involving increases in respiration and ethylene production rates and elevated activities of cell wall and membrane-degrading enzymes compromise cellular and tissue integrity. These responses contribute to the development of quality defects like juice leakage, firmness loss and water-soaked appearance. They also drive the loss of bioactive compounds like lycopene, affecting flesh color and reducing nutritional value, ultimately culminating in consumer rejection, food losses and waste. Although great research progress has been achieved in the past decades, knowledge gaps about the physiological, biochemical and molecular bases of quality loss persist. This review article summarizes the advances in the study of physicochemical, microbiological, nutritional, and sensory changes linked to the deterioration of watermelon after processing and during storage. Different technological approaches for quality improvement and shelf-life extension are summarized: pre- and postharvest, physical, and chemical. We also discuss the advantages, disadvantages and challenges of these interventions and propose alternative directions for future research aiming to reduce qualitative and quantitative fresh-cut watermelon losses.
Watermelon (Citrullus lanatus L.) is a widely cultivated fruit from the Cucurbitaceae family. It is one of the economically leading fruit crops globally, with a world production of 100 million tons, and China as the top producer (Faostat, 2022).
Watermelon consumption has increased in the last decade due to its juicy and fresh pulp, appealing color, high water content, and flavor in addition to its bioactive compounds such as lycopene and citrulline (Perkins-Veazie et al., 2012a; Petrou et al., 2013; Hong et al., 2015; Nelson et al., 2022). In the United States, watermelon is the most preferred melon type, accounting for over 67% of total melon availability, and it is the sixth most consumed fruit, purchased by 53% of households (Weber et al., 2023; Liu et al., 2024). However, its relatively large size limits portability and affects the purchasing decisions of customers (Hu et al., 2022). This has created an avenue for convenience, making fresh-cut watermelon a viable option. Fresh-cut or minimally processed produce are fruits and vegetables subjected to cutting, shredding, or peeling to provide convenient ready-to-eat or ready-to-cook servings (Watada and Qi, 1999; Brecht et al., 2003).
In the US, fresh-cut watermelon accounts for 14.7% of total watermelon sales, increasing by 18.3% in the 2023-2024 cycle (United Fresh Produce Association, 2020; National Watermelon Promotion Board, 2024). Despite the potential of the fresh-cut watermelon market to continue expanding, quality degradation during storage poses a challenge to the industry (Sipahi et al., 2013; Mendoza-Enano et al., 2019). Fresh-cut processing induces a dramatic metabolic remodeling that leads to changes in color, texture, and flavor and increases watermelon susceptibility to spoilage (Mao et al., 2006; Hu et al., 2022). These alterations compromise marketability and shorten shelf-life, contributing to sales stagnation in recent years (Artés-Hernández et al., 2021). Ultimately, quality loss leads to postharvest losses and waste (Wang et al., 2018; Hu et al., 2022; Wu et al., 2022).
The implementation of pre- and postharvest approaches applied directly to fresh-cut watermelon, or to whole fruit before processing has provided insights into the biological basis of postharvest quality loss and offered alternatives for shelf-life extension. Some of these strategies include grafting (Roberts et al., 2005), cutting tools and processing formats (Mcglynn et al., 2003; Petrou et al., 2013; Artés-Hernández et al., 2021; Lee, 2021), exogenous application of chemical agents (Kaveh, 2016; Ajami and Nemati, 2024; Jacuinde-Guzmán et al., 2024), modified atmosphere packaging (Cartaxo and Sargent, 1998; Lee, 2021), and irradiation treatments (Wang et al., 2018; Shi et al., 2020; Artés-Hernández et al., 2021; Jaiswal and Srivastava, 2024).
This literature review aims to consolidate the knowledge about the mechanisms underlying quality degradation in fresh-cut watermelon during storage and strategies for quality maintenance. We also highlight current research gaps, challenges, and avenues for future research.
The quality of fresh-cut watermelon is underscored by a combination of attributes such as appearance, texture, and flavor, as well as nutritional value and food safety considerations that influence consumer perception. Fresh-cut operations promote physical and physiological changes (Figure 1) that lead to quality alterations and culminate in reduced shelf-life and marketability.
Figure 1. Organoleptic, nutritional, and microbiological quality parameters and physiological changes commonly reported in fresh-cut watermelon studies.
Also known as “drip loss” or “purge,” juice leakage is a common phenomenon during the storage of fresh-cut watermelon that affects its quality, safety, and marketability (Figure 2). Juice from the fruit cells migrates to and deposits at the bottom of containers by gravity, creating an undesirable appearance associated with freshness loss (Artés-Hernández et al., 2010; Wang et al., 2018). Juice leakage ranges an average of 4%–10% during storage on a fresh weight basis (Wang et al., 2018) and is influenced by ripening stage and storage temperature (Petrou et al., 2013). Perkins-Veazie and Collins, (2004) reported slightly higher values attributed to cultivar-dependent variations. Herein, during 10 days of storage at 2°C, fresh-cut samples from the seeded cultivar “Summer Flavor 800” presented 13% juice leakage in contrast to 11% observed in seedless “Sugar Shack” after 2 days of storage. No significant changes were observed beyond this point. In addition, leaked watermelon juice experiences changes throughout storage that act as an indicator of shelf-life. The juice contains sugars that create a favorable environment for microbial growth and anaerobic fermentation (Perkins-Veazie et al., 2012b). Increases in juice turbidity, i.e., the degree of clarity or cloudiness of the purge, correlate with higher microbial load, an indicator of food safety, as well as with declines in sensory quality and consumer preference (Gombas et al., 2017; Mohamad Salin et al., 2022). Despite the relevance of this quality defect in fresh-cut fruit, changes in the quality characteristics of leaked juice in watermelon have been scarcely examined in the literature.
Figure 2. Juice leakage in fresh-cut watermelon. Juice accumulates at the bottom of containers during storage, increasing in volume and turbidity.
Textural characteristics such as firmness, crispiness and juiciness are important indicators of the freshness and quality of fresh-cut produce including watermelon (Francis et al., 2012; Mendoza-Enano et al., 2019). Changes in texture are closely associated with alterations in cell wall structure and mechanical properties, particularly in fleshy fruits (Toivonen and Brummell, 2008). During ripening, pectin solubilization and depolymerization largely affect texture and quality (Vicente et al., 2007; Liu et al., 2023).
Extensive research on flesh firmness has been conducted in watermelon. In the last decades, consumer demand for firmer flesh has propelled breeding programs to integrate this trait into newer cultivars, departing from heirloom varieties that have a softer texture (Davis and King, 2007). During fruit development, cell wall remodeling largely determines watermelon quality and texture. Studies on the interplay between the hormones abscisic acid (ABA) and indole-3-acetic acid (IAA), along with cell wall-modifying enzymes have led to the identification of relevant regulators of fruit firmness (Sun et al., 2020; Anees et al., 2021; 2023).
In fresh-cut products, softening results from concerted processes initiated by physical damage and mediated by enzymes (Ragaert et al., 2007; Toivonen and Brummell, 2008) (Figure 3). Pectate lyase (PL), polygalacturonase (PG), and pectin methylesterase (PME) are the most widely studied and characterized pectinases that catalyze this disintegration (Karakurt and Huber, 2003; Toivonen and Brummell, 2008). Interestingly, in fresh-cut watermelon, cell wall dynamics have not been thoroughly described during storage, and only a few reports are available in the literature. Xisto et al. (2012) showed that PME activity did not vary in slices stored at 5°C for 10 days, and no PG activity was detected. Nonetheless, loss of firmness was observed along with an increase in soluble pectin, an indicator of cell wall disassembly. This observation suggests the action of alternative players in cell wall modification.
Figure 3. Texture-associated changes and proposed mechanisms influenced by fresh-cut processing. These alterations result from processes initiated by physical damage and tissue disruption [1] that promote juice leakage [2] and water loss in the form of vapor to the atmosphere [3]. Mechanical stress triggers a signal transduction cascade that stimulates physiological and metabolic responses, including increased respiration and ethylene production [4]. Enzymes like pectate lyase (PL), pectin methylesterase (PME), and polygalacturonase (PG) catalyze the degradation of the pectin fraction of the cell wall [5], generating various products. Other enzymes such as phospholipase C (PLC), D (PLD) and lipoxygenase (LOX) are involved in degrading cell membrane phospholipids [6] that result in the generation of lipid peroxidation byproducts [7] and reactive oxygen species (ROS) that participate in further peroxidation reactions. The dashed lines symbolize that further research is needed to determine if the events occur in parallel or are driven by ethylene. The question mark indicates that additional studies are required to determine if ethylene triggers defense-signaling compounds that detoxify or scavenge the byproducts of oxidative reactions, as seen in other fruits (Li et al., 2022; Wang et al., 2023).
Loss of firmness due to decreased turgor is also reported in fresh-cut produce and linked to cell disruption, water loss and juice leakage (Hu and Jiang, 2007; Francis et al., 2012) (Figure 3). In watermelon, Myriah Mason et al. (2017) observed a slight, non-significant decrease in firmness in fresh-cut samples of four cultivars after a 5-day storage period at 5°C. In a study by Xisto et al. (2012), scanning electron micrographs of slices stored at 5°C revealed the release of liquid from the fruit tissue due to cell disintegration after 10 days. This observation aligned with an increased content of soluble pectin, juice leakage, and a greater rate of firmness loss.
Fresh-cut processing disrupts cell membranes, triggering physiochemical changes that compromise structural integrity (Figure 3). This leads to functional impairments, ultimately manifesting as altered texture (Brecht et al., 2003). In watermelon, these processes manifest as a loss of selective permeability, and greater ion or electrolyte leakage (Mao et al., 2004; Fan and Sokorai, 2005). Organelle decompartmentalization triggers the release of the enzymes phospholipase C (PLC) and D (PLD) and lipoxygenase (LOX), along with lipids that serve as substrates for further biochemical reactions (Brecht et al., 2003; Karakurt and Huber, 2004; Mao et al., 2004).
Malondialdehyde (MDA) is a byproduct of lipid peroxidation and a biomarker of oxidative stress and membrane damage (Brecht et al., 2003; Hu et al., 2021). Subsequent peroxidation of the resultant lipids leads to the formation of reactive oxygen species (ROS) that cause further membrane disruption and loss of cell integrity (Shewfelt and Rosario, 2000; Brecht et al., 2003) (Figure 3). Watermelon slices from five cultivars stored at 4°C for 5 days were assessed for membrane integrity via MDA, ROS content and peroxidase (POD) activity, which catalyzes the degradation of hydrogen peroxide (H2O2) to oxygen (O2) and water. Data showed that “Jingxin #1” and “8424 seeded” recorded the highest MDA contents. High POD activity, concomitant with low H2O2 and high O2 levels were shown by “Qilin” and the opposite in “8424 seeded”, thus highlighting a genotype-dependent response. Interestingly, cultivars that presented high MDA also recorded high juice leakage (Myriah Mason et al., 2017). Further research is needed to explore a potential correlation between these parameters.
Visual appearance is a pivotal criterion of consumer perception in the fresh-cut produce market, affecting purchase decisions and market value (Lund et al., 2000). The diversity of flesh colors of watermelon; red, pink, pale yellow, white and orange is underscored by carotenoid composition (Tadmor et al., 2005). In red-fleshed watermelons, α and β-carotene, phytofluene, phytoene, and lycopene are commonly reported carotenoids, with the latter being the most predominant (up to 97%) (Perkins-Veazie et al., 2001; Zhao et al., 2013; Ilahy et al., 2019).
Fresh-cut processing induces changes in watermelon flesh color that can be detected instrumentally or by sensory evaluations and consumer panels (Figure 1). Watermelon cubes monitored for 9 days of storage at 5°C were linked to low visual appearance scores by panelists and increased Hue values, dark red appearance and development of water-soaked areas compared to whole fruits (Gil et al., 2006). Perkins-Veazie and Collins (2004) recorded increased L* and decreased a* values in cubes stored at 2°C for 10 days, denoting loss of lightness and redness, respectively. Correspondingly, reductions in lycopene content were observed, however, the correlation with objective color descriptors was weak. In another study, in addition to a 6%–16% lycopene loss, fresh-cut cubes stored at 5°C for 11 days displayed low subjective sensory scores for appearance and overall quality due to off-color development and translucent damaged edges (Artés-Hernández et al., 2010). Nevertheless, cultivar-dependent variations in flesh color may present a limitation to objective color measurements. Evaluation of this trait requires complementary indicators to comprehensively assess fluctuations during storage.
Flavor is the combined perception of taste and aroma and determines consumer acceptance (Ramirez et al., 2020). The primary determinants of fruit taste are the levels of sugar and organic acids and their balance, which influence sweetness and acidity (Figure 1). The aroma, on the other hand, is primarily determined by the quantity and diversity of volatile organic compounds (VOCs) (Clark, 1998; More et al., 2020). As respiratory substrates, these components are depleted due to the elevated respiration rates resulting from cutting operations, affecting the overall flavor of fresh-cut watermelon (Xisto et al., 2012; Hu et al., 2022).
Total soluble solids (TSS) is a commonly used estimator of the sweetness and sugar content of watermelon fruit (Huang et al., 2022). The produce industry is highly reliant on this parameter, and hence, its management is crucial (Tarazona-Díaz et al., 2011). In fresh-cut watermelon, TSS responses during storage are variable and influenced by genotype and handling conditions. During 10 days of storage of fresh-cut cubes at 2°C, Perkins-Veazie and Collins (2004) observed a decline in TSS after 2 and 7 days in “Sugar Shack” (seedless) and “Summer Flavor 800” (seeded), respectively. In contrast, Myriah Mason et al. (2017) reported that sliced samples from four out of five cultivars did not exhibit significant TSS changes, and one showed an increase after 5 days at 5°C. Gil et al. (2006) found that cubes and whole fruits stored at 5°C did not differ in TSS after 6 days. However, after 9 days, whole fruits had higher TSS than the cubes.
Studies on the aroma profile of watermelon have been primarily carried out on fruit immediately after cutting (Beaulieu and Lea, 2006; Liu et al., 2018; Fredes et al., 2024) and on juice (Xiaowei et al., 2012; Liu et al., 2018), with fewer reports on fluctuations throughout postharvest storage. Xisto et al. (2012) analyzed the volatile profile of fresh-cut samples during a 10-day storage period at 5°C using Gas Chromatography-Mass Spectrometry (GC-MS) from Solid-Phase Microextraction (SPME). Six-carbon and nine-carbon alcohols and aldehydes associated with the characteristic aroma of watermelon were detected during this period. Four of these compounds, (E,Z)-2,6-nonadienal, (Z,Z)-3,6-nonadienol, Z-6-nonenol and E-2-nonenal declined progressively, proportional to the decrease in aroma perceived by an untrained sensory panel.
Bioactive compounds contained in watermelon, such as lycopene, vitamin C and polyphenols, have antioxidative, anti-inflammatory and antimicrobial activities associated with potential benefits for human health (Manivannan et al., 2020; Meghwar et al., 2024) (Figure 1). Fresh-cut processing has been shown to alter the levels of these compounds; however, there is little published data on fluctuations of other relevant phytonutrients in watermelon, like β-carotene and citrulline, during postharvest storage.
For lycopene, Perkins-Veazie and Collins, (2004) described a 6%–11% decrease in cubes stored at 2°C for 10 days. A similar trend has been reported in fresh-cut samples of “Tri-X 313” after 3 and 6 days (Gil et al., 2006), and “Crimson Sweet” after 14 days at 5°C (Kaveh, 2016).
Phenolic compounds, apart from their beneficial role to humans, also play a vital role as secondary metabolites in response to biotic and abiotic stress. Fresh-cut operations trigger phenolic alterations; loss, biosynthesis and metabolic turnover (Babic et al., 1993; Hodges and Toivonen, 2008). Decreases in total phenol content were observed in fresh-cut watermelon during storage at 5°C for 5 days (Gómez et al., 2017; Artés-Hernández et al., 2021) and 11 days (Artés-Hernández et al., 2010). Nonetheless, flesh discoloration is not commonly associated with enzymatic browning as this fruit has a relatively low content of phenolic compounds (Gil et al., 2006).
Ascorbic acid (AA) is a key biomarker of oxidation in fresh-cut fruits and vegetables (Barth et al., 1993). In fresh-cut watermelon, AA declined up to 6% after 11 days of storage at 5°C (Gil et al., 2006; Artés-Hernández et al., 2010).
Ensuring microbial safety is a major priority of the fresh-cut industry. The rising incidence of fresh-cut associated alimentary toxicosis outbreaks has become a growing public concern (Li et al., 2013; Hu et al., 2022). In the United States, a multistate outbreak of Salmonella on fresh-cut fruit salad resulted in 60 cases across six states in 2018 (Food and Drug Administration, 2018); therefore, ensuring quality and microbial safety is crucial for the industry. Tissue disruptions due to fresh-cut operations facilitate the release of cellular contents that migrate to adjacent intercellular spaces and non-wounded tissues (Saltveit, 2002; Brecht et al., 2003; Iturralde-García et al., 2022). This movement and accumulation provide a nutrient-rich environment for the growth of both spoilage and pathogenic microorganisms, culminating in reduced quality and shelf-life as well as public health risks (Brecht et al., 2003; Yousuf et al., 2020).
In watermelon, fresh-cut-induced changes in microbial populations have been described during refrigerated storage (Figure 1). Increased counts of yeast, mold and enterobacteria were reported in “Fashion” (Artés-Hernández et al., 2021), “Texiaofeng” and “8424 seeded” after 7 days of storage at 5°C (Myriah Mason et al., 2017). In “Fashion” cubes, the growth dynamics of different microbial classes, mesophilic and psychrophilic, showed an increase in population counts after 4–6 days, reaching the maximum by the end of the 12-day storage period at 5°C (Artés-Hernández et al., 2010).
The potential of sanitation, good manufacturing practices, temperature management as well as the application of physical and chemical treatments to address microbial and food safety risks during storage has been explored (Soliva-Fortuny and Martı́n-Belloso, 2003; Lee, 2021). These approaches are summarized in section 3.
Fresh-cut watermelon meets consumer demand for nutrition, sensory quality and convenience; however, its short shelf-life poses a challenge. Different strategies at the pre- and postharvest levels have broadened our understanding of the factors contributing to quality decline during storage. Some of these approaches have been directly evaluated on cut watermelon, while others have been applied to whole fruit prior to cutting, followed by processing and subsequent assessment of postharvest quality. They have been summarized in Table 1.
Table 1. Overview of outcomes of applied techniques to improve the quality and shelf-life of fresh-cut watermelon.
Pre-harvest management practices such as irrigation (Zhou et al., 2023), fertilization (Aitbayeva et al., 2021), grafting (Yang et al., 2024), growing methods (Kyriacou et al., 2018), and others (Kahan, 2013; Zaaroor-Presman et al., 2020) have been widely reported to influence the postharvest physiology and quality of whole watermelon fruit. These strategies have been reviewed in detail by Kyriacou et al. (2018) and Nadeem et al. (2022). Interestingly, there is little information about the effect of pre-harvest practices on fresh-cut watermelon quality and shelf-life, and only a few reports are available in the literature. The effect of grafting, a technique where the root system from one plant, i.e., rootstock, is joined with the top part of another plant, i.e., the scion, was tested in fresh-cut watermelon during storage at 5°C for 10 days. Cubes from grafted cultivars presented greater firmness retention than those from the non-grafted plants. However, grafting had no effect on TSS, bacterial counts or lycopene content, which showed a decrease of up to 10% (Roberts et al., 2005).
Temperature is the most important factor influencing the postharvest quality of fresh produce (Kader, 2002). It exerts effects on metabolic and physiological processes leading to changes in texture, appearance, flavor, and microbial growth (Gidado et al., 2024). In fresh-cut watermelon, a storage temperature of 5°C or below is recommended to ensure microbiological quality (Fleming et al., 2005; Ukuku and Sapers, 2007). Cold chain breaks during distribution, retail and at the consumer level can expose the product to abusive temperature conditions that may jeopardize food safety. Similarly, lack of temperature management at processing sites, storage and sale points promotes the growth of various microorganisms, including human pathogens, increasing the risk of foodborne illness for consumers (Bai et al., 2024; Nketiah et al., 2024; Oluka et al., 2024). Del Rosario and Beuchat, (1995) investigated the microbial growth dynamics of inoculated Escherichia coli on cubes stored at 25 or 5°C for 21 days. Cubes stored at 5°C recorded lower E. coli counts compared to samples at 25°C. Likewise, reduced microbial growth of Listeria monocytogenes was achieved by storing cubes at 4°C compared to 13°C in a 6-day study (Moreira et al., 2023). Microbial inhibition of Salmonella has also been reported for cubed samples stored at 5°C in comparison to 10 or 22°C (Ukuku and Sapers, 2007).
Evidence also suggests that storage temperature drives changes in microbial populations and diversity. In this regard, findings from Feng et al. (2017) showed that in addition to inhibiting the growth of E. coli and Salmonella, natural microbiota was best maintained in cubes stored at 5°C, in contrast to 13°C and 25°C. Hu et al. (2022) monitored cubes on a 3-day study at 4 or 28°C using next-generation sequencing. Although microbial growth increased regardless of storage temperature, cubes at 4°C presented lower total bacterial counts than at 28°C. The microbiome analysis revealed that storage at 4°C had no effect on the initial microbial diversity, with proteobacteria and cyanobacteria as the dominant bacteria phylum. However, a distinct shift marked by elevated proteobacteria growth and emergence of firmicutes was observed in cubes at 28°C.
In terms of changes in organoleptic quality influenced by storage temperature, Sargent (1998) found that cubes refrigerated at 1°C presented 50% more juice leakage than those at 3°C for 10 days. In a different investigation, the shelf-life of cubes at 1, 3, 7, 11, 15, or 30°C was monitored for 10 days. Samples at 1 or 3°C had a longer shelf-life (10 d) than cubes at or above 7°C (<6 d), corroborated by less flesh darkening, off-odor development, juice leakage and microbial load (Fonseca et al., 2004). Mendoza-Enano et al. (2019) reported that panelists’ flavor liking scores of fresh-cut cubes decreased progressively during storage at 3°C and 7°C for 8 days. In parallel, objective measurements of odor-active volatiles revealed increased concentrations of compounds associated with reduced watermelon freshness, such as 2-butanone, 1-penten-3-one, hexanal and dimethyl trisulfide and acetophenone.
In an attempt to simulate commercial practices, the influence of pre- or post-cut hold temperature regimes prior to fresh-cut processing is further demonstrated in the following studies. Cut watermelon samples held at 20°C for 2 h before storage at 4°C were not commercially acceptable (visual quality and aroma) after 14 days compared to those that were not held. Nonetheless, the holding condition before storage had no effect on respiration and other quality traits like firmness and juice leakage (Lee, 2021). In another study, pre-cut storage of whole watermelons at 4, 20 or 30°C for 2 days led to quality alterations in cubes stored at 4°C for 7 days. Cubes from whole fruits incubated at 20°C presented better aroma and visual quality and lower juice leakage than the other treatments, while 4°C storage led to the highest firmness (Lee, 2021). Lee et al. (2018) incubated whole watermelons at 4, 21 or 30°C for 21 h, followed by fresh-cut processing and storage for 5 days at 4°C and 7°C. Findings revealed that across the pre-cut temperatures, 4°C-storage led to higher TSS than 7°C. In a study by Hu et al. (2022), TSS, glucose and fructose decreased more in cubes stored at 28 than at 4°C after 72 h. Furthermore, at 28°C, a concomitant decrease in pH was seen with the organic acids malate and citrate, while the fermentation markers, ethanol and lactate, increased. This trend contrasted with cubes stored at 4°C. In a report by Ukuku and Sapers (2007), holding cubes at 22°C for 3 or 5 h prior to storage at 5°C resulted in a progressive increase in Salmonella growth, whereas storing cubes immediately after processing inhibited its growth. Collectively, these reports highlight the relevance of refrigeration for organoleptic as well as microbiological quality maintenance.
Fresh-cut operations induce physical damage that alters the overall quality and shelf-life of produce (Portela and Cantwell, 2001; Incardona et al., 2024). In fresh-cut watermelon, different cut sizes have been shown to influence organoleptic quality during storage. Cylindrical-shaped fragments of 1, 2, 4, and 8 cm diameter stored at 5°C revealed that 1 and 8-cm samples had the lowest and highest visual appearance scores by panelists, respectively (Artés-Hernández et al., 2021). A similar finding by Lee, (2021) showed a differential effect of cut size (2, 2.5 and 3 cm) on cube quality during storage at 4°C. Here, the highest appearance and aroma scores were displayed by larger cubes (3 cm), and as the cut size increased, respiration was reduced, and firmness was better retained. The higher surface area to volume ratio of smaller fragments, as seen in melon, may explain some of these responses (Spadafora et al., 2019).
The presence of the rind, or the white layer surrounding the flesh, plays a significant role in the quality of fresh-cut watermelon. In this regard, Petrou et al. (2013) assessed the quality of slices with or without rinds stored at 4°C for 9 days. Findings revealed that while the presence of the rind had no effect on electrolyte leakage, slices with rind displayed 47% less juice leakage compared to rind-free slices. Furthermore, the presence of the rind minimized losses in TSS, total sugars, glucose, fructose and sucrose compared to rind-free slices.
Water-jet, a cold and high-pressure cutting technology is commonly used in the food industry for being safe and environmentally friendly (Henning, 1997). Mcglynn et al. (2003) studied the effect of this tool on the shelf-life and microbial quality of fresh-cut watermelon. Compared to knife-cut samples, water-jet led to firmness retention, but no differences were found in terms of flesh color and microbial counts during storage at 4°C for 12 days.
The potential benefit of hydrogen gas (H2) in the maintenance of postharvest quality of fruits and vegetables has been reported via increased antioxidant capacity and reduced ethylene production (Hu et al., 2014; Gong et al., 2018). In fresh-cut watermelon, Lee et al. (2020) investigated the effect of an exogenous H2 application as a shock treatment (4 h after cutting) or under continuous application at 4.2 and 42 μL L−1 during cube storage at ∼5°C for 8 days. Regardless of the concentration, continuous H2 application was optimal for quality maintenance evidenced by better firmness retention, higher TSS and subjective quality scores. Respiration rates and water-soaking incidence were reduced in the continuous treatment compared with the shock treatment and control. Cubes treated with 4.2 μL L−1 H2 presented higher subjective aroma and appearance scores, less water-soaking incidence, and better firmness retention.
The inhibitor of ethylene perception 1-methylcyclopropane (1-MCP) plays an important role in maintaining the quality of fresh produce commercially. In a study by Saftner et al. (2007), whole watermelon was first treated with 1-MCP at 0.5 and 1 μL L−1, followed by ethylene treatment. Slices were obtained from the placenta, heart and rind and stored at 5°C for 12 days. Samples from all three parts treated with ethylene alone presented the lowest firmness and textural scores. Interestingly,1-MCP suppressed ethylene-induced softening in all tissues but did not influence firmness when no ethylene was applied. This was consistent with findings by Zhou et al. (2006), wherein 1-MCP exposure suppressed juice leakage and slowed microorganism growth in slices from ethylene-treated whole fruits. However, 1-MCP-associated benefits were not observed in samples obtained from whole fruits treated with 10 μL L−1 1-MCP (without ethylene) prior to fresh-cut processing during 7 days of storage at 10°C (Mao et al., 2006).
The effect of potassium permanganate-based (KMnO4) ethylene removal sachets on the quality of fresh-cut watermelon was tested by Lee et al. (2020). KMnO4-treated cubes had higher crispness, aroma and appearance scores, and lower juice and electrolyte leakage than the air-stored (control) during storage at 4°C for 9 days.
In other conventional chemical treatments, calcium (Ca2+) and its salts are notable for their general role in postharvest quality maintenance, especially in decreasing produce softening by strengthening the cell wall (Glenn and Poovaiah, 1990; Braccini and Pérez, 2001; Soliva-Fortuny and Martı́n-Belloso, 2003; Pelloux et al., 2007). The application of different Ca-based products like salts and Ca-nanoparticles has been tried for microbial safety and shelf-life extension in fresh-cut watermelon with mixed results. The quality of cut samples dipped in 1, 5, 10 or 20% calcium ascorbate (CaAsc) solutions for 2 min showed a negative concentration-dependent relationship. Increasing CaAsc concentrations elevated weight loss, juice leakage and loss of redness (a* values) during 10 days of storage at 10°C. Nonetheless, 1% CaAsc was optimal to achieve a desirable effect on quality (Lichanporn et al., 2014). Findings by Lee et al. (2020) further corroborated this observation wherein cubes treated with 0.6 M CaAsc presented lower appearance and aroma scores, firmness and higher juice leakage than 0.3 M-treated cubes stored at 4°C for 10 days. In a study by Jacuinde-Guzmán et al. (2024), the quality and shelf-life of cubes immersed in 1% CaCl2 or Ca-based nanoparticles (CaO or Ca(OH)2-NP) were assessed during storage at 5°C for 12 days. Samples treated with 150 and 200 mg L-1 Ca(OH)2-NP exhibited lower water-soaking incidence and increased firmness by reducing PG and PME activities relative to other treatments and the control (water).
Evidence suggests that the benefits of calcium treatments are enhanced by hot-water dips. Dipping of watermelon cubes in hot (45°C) calcium chloride (CaCl2) at 1% displayed higher firmness retention, lower respiration, and reduced microbial growth during storage at 5°C for 8 days compared to cold-dipped cubes at 5°C (Aguayo et al., 2013).
The use of CaCl2 in combination with 1-MCP was examined during storage at 10°C for 9 days. Findings revealed that samples treated with 2% CaCl2 alone presented increased electrolyte leakage and respiration but these responses were suppressed by 1-MCP (Mao et al., 2006).
Salicylic acid (SA) is a phytohormone that has been shown to improve the postharvest quality and shelf-life of numerous horticultural crops when applied exogenously (Jing-Hua et al., 2008; Dokhanieh et al., 2013). Immersion of cubes in 2 mM SA effectively reduced weight loss and microbial growth and improved firmness retention by 82% compared to the untreated control during storage at 4°C for 14 days (Ajami and Nemati, 2024).
Citric acid is a naturally occurring organic acid used as an external treatment for fresh produce and is generally recognized as safe (GRAS). Watermelon cubes were immersed in 0.5 or 1 mM citric acid for 2 min and stored at 4°C for 14 days. A 3.24 log-fold reduction in microbial growth, lower weight loss and greater firmness retention were achieved in fruit treated with 1 mM citric acid (Ajami and Nemati, 2024).
Sanitizing whole watermelon in 1,000 ppm sodium hypochlorite for 1 min prior to cutting resulted in a 1.3-log reduction in colony forming units (CFU) of coliforms in fresh-cut pieces compared to the control (de-ionized water) during storage at 4°C for 7 days (Mcglynn et al., 2003).
Lee et al. (2020) evaluated the effect of chlorine-free sanitizers applied to whole watermelons on the quality of cubes. Samples treated with 200 μL L−1 neutral pH electrolyzed water (NEW) recorded lower juice leakage, and higher firmness and subjective sensory scores for aroma and appearance compared to those treated with water (control) after 10 days of storage at 4°C.
Peracetic acid at 150–200 mg L−1 was applied as a sanitation treatment to watermelon cubes. Samples were sealed with non-perforated film, stored at 3°C for 1 and 8 days, and compared to untreated cubes. Microbial counts remained within safe limits in both groups, but subjective sensory scores and TSS content were reduced in sanitized samples. Respiration rates were also higher in the sprayed group. These responses were attributed to the potential wash-off of flavor-related compounds induced by the post-cut sanitation step (Mendoza-Enano et al., 2019).
The effect of ozone on the microbial quality of cut watermelon was tested by Man and Huy (2008). Fresh-cut samples were ozone-treated at a concentration of 4.2 mg dm-3 (30°C, 1 atm) for 1, 2 or 3 min and stored at 4°C for 6 days. Findings revealed a time-dependent effect in microbial reduction within the treatments. Furthermore, samples treated for 3 min showed the highest reductions in aerobic bacteria (1-log), yeasts and mold counts (1.5-log), compared to the untreated control.
Plant extracts are bioactive chemical-containing mixtures obtained through water and/or organic solvent extraction (Das et al., 2010). In postharvest management, their application has improved produce quality and shelf-life, making them a viable alternative to conventional chemical treatments (Das et al., 2010; El Khetabi et al., 2022).
Findings from Kaveh (2016) showed the antimicrobial and quality preservation potential of saffron petal extracts on fresh-cut watermelon stored at 5°C. Cubes treated with 10% (v/v) extracts for 10 min reduced lycopene degradation, preserved visual quality, and inhibited microbial growth. Similarly, cubes dipped in clove basil leaf extracts for 10 min prior to storage at 4°C had lower microbial growth and polyphenol losses compared to untreated samples (Ebabhi et al., 2019). In another study, eugenol and carvacrol essential oils applied at 750 and 200 ppm inhibited the growth of pathogenic fungi Aspergillus carbonarius and Penicillium roqueforti (Šimović et al., 2014). The application of cinnamon oil (CO) at 0.0204, 0.0408 or 0.0612 g per 100 g of watermelon or as an inclusion complex with β-Cyclodextrin (CO-β-CD) was investigated for quality preservation of cubes stored at 4°C for 4 days. Weight loss increased throughout storage; however, 0.0408 g CO-β-CD resulted in the least weight loss compared to the other treatments. Similarly, this concentration was more effective in preserving flesh color and TSS while limiting microbial growth, keeping total counts within acceptable limits (Li et al., 2019).
A drawback of essential oils application in foods is the potential trade-off in sensory quality. However, no changes in this regard were reported due to the low concentration (<1000 ppm) of eugenol and carvacrol used in fresh-cut watermelon studies (Šimović et al., 2014). In contrast, CO application resulted in low subjective flavor ratings. Interestingly, CO-β-CD improved flavor and consumer acceptance scores, likely due to its ability to absorb CO’s strong odor. (Li et al., 2019).
Edible coatings are thin, edible films of thickness less than 0.3 mm that are applied to produce surfaces to function as protective barriers. They are considered a type of active packaging because they provide a physical barrier to gas between the product and the environment. In addition, they can deliver additives and nutrients with biological activity that improve produce safety and quality (Han, 2014; Trajkovska Petkoska et al., 2021).
In fresh-cut watermelon, Song et al. (2023) evaluated the shelf-life and microbial quality of cubes coated with an agar/carrageenan (AG/CA) polysaccharide-based film or AG/CA loaded with the antimicrobial agent Nisin (AG/CA-N) during storage at 4 or 20°C for 18 and 6 days, respectively. Microbial analysis revealed that AG/CA-N effectively inhibited the growth of spoilage microorganisms Staphylococcus aureus and L. monocytogenes. Likewise, AG/CA-N outperformed AG/CA and the uncoated control in firmness and vitamin C retention. In a different study, the efficacy of a sodium alginate (0.5, 1.0 or 2 g 100 g⁻1)-based multilayered edible coating containing the antimicrobial agent trans-cinnamaldehyde was evaluated during 15 days of storage at 4°C. Findings revealed that the coating inhibited the growth of psychrotrophic microbes, yeasts and molds compared to the uncoated control, with the 2 g 100 g-1 concentration achieving the most significant reductions. A positive correlation between alginate concentration, firmness retention, respiration rate and overall acceptability was observed (Sipahi et al., 2013). Regarding the consumer acceptance of the tested coatings, the outcomes were heterogeneous. The 2 g 100 g-1 coating was the least preferred due to its appearance. Consistent with objective color measurements, the 0.5 and 1.0 g 100 g⁻1-coated samples ranked higher than the uncoated control. Similarly, the control and 1 g 100 g⁻1-coated samples received high flavor scores, whereas the 2 g 100 g-1 treatment had the lowest values explained by flavor changes imparted by trans-cinnamaldehyde. Altogether, these findings highlight the importance of material selection and dosage to achieving quality preservation in combination with meeting consumer sensory appeals.
Irradiation is a non-thermal food processing technology commonly used to achieve sterilization, modification and/or preservation of food qualities (Diehl, 2002). Based on the electromagnetic wavelength and frequency, this technology is classified as non-ionizing or ionizing irradiation (Lewandowski, 2001). Non-ionizing irradiation, such as visible light and ultraviolet (UV), primarily uses thermic effects produced by these rays. On the other hand, ionizing irradiation—electron beams and X-rays—acts via alterations in molecular structures, achieving microbial reduction and extended shelf-life (Zhong et al., 2021; Liu et al., 2023). These different methods have been applied to fresh-cut watermelon to assess their effects on quality and shelf-life.
Wang et al. (2018) exposed fresh-cut watermelon to visible light at 10, 150 (control), and 3000 Lux to simulate supermarket cabinet storage conditions for 5 days at 4°C. The 3,000 Lux treatment showed the lowest cell wall degradation ratio, PL and PG activities, electrolyte leakage, as well as fresh weight and drip loss among treatments. The exposure of cubes to blue, yellow, green and red light was evaluated during storage at 4°C for 4 days. Red light exposure led to significant firmness, color, appearance and aroma retention. Moreover, weight loss and water-soaking symptoms were reduced by 50% and 67%, respectively (Shi et al., 2020).
Pulsed light irradiation involves the application of very short to a high-intensity spectrum of light covering UV (100–400 nm), visible (400–700 nm), and infrared (700–1100 nm) for the preservation of produce shelf-life (Dunn et al., 1995; Elmnasser et al., 2007; Mahendran et al., 2019; Yao et al., 2023). In watermelon, pulsed light (12 J cm-2, 180-1,100 nm) applied to fresh-cut pieces resulted in a 3-fold log reduction of E. coli and Listeria innocua populations, decreased ethylene production and maintained firmness compared to the untreated control during 15 days of storage at 5°C (Ramos-Villarroel et al., 2012). Similarly, the use of pulsed light (12 J cm−2, 180-1,100 nm) in combination with 2% malic acid resulted in greater microbial reduction than their individual treatments and the untreated group by the end of 15 days of storage at 5°C (Ramos-Villarroel et al., 2015).
UV is one of the most common irradiation preservation technologies in the fruit and vegetable industry (Liu et al., 2023). In the US, UV-C is widely used because it is environmentally friendly and toxic-residue-free (Rhim et al., 1999; Food and Drug Administration, 2002).
In watermelon, Fonseca and Rushing (2006) found that UV-C irradiation (4.1 kJ m−2) was more effective in inhibiting microbial growth than chlorine and ozone in cubes at the end of 8 days of storage at 3°C. UV-C also reduced juice leakage and loss of red flesh color. Artés-Hernández et al. (2010) assessed the overall quality, microbial, and bioactive compound alterations induced by UV-C exposure post-cutting during storage at 5°C for 12 days. A general reduction in bacterial counts was achieved by all treatments (1.6–7.2 kJ m−2) in a dose-dependent manner. Low doses (1.6 and 2.8 kJ m−2) presented higher organoleptic quality and bioactive compound retention than higher doses. Additionally, the combination of UV-C and cut size was investigated on cylinders during storage at 4°C for 7 days. Results revealed an interaction between these factors, where the combination of 4.8 kJ m−2 and large cylinders (≥4 cm) led to delayed microbial growth, and greater lycopene and phenolic content retention (Artés-Hernández et al., 2021).
This irradiation technology uses high-energy electrons generated from the electromagnetic field to achieve insecticidal and bacteriostatic activity effects on agricultural products (Nam et al., 2019; Liu et al., 2023).
In watermelon, cubes exposed to 1.0 kGy electron beam radiation maintained firmness and color, reduced microbial growth and had higher consumer acceptability scores than the untreated control during 21 days of storage at 4°C (Smith et al., 2017). In a different study, cubes irradiated with 0.5 and 1.0 kGy gamma presented no difference in firmness, flesh color and TSS during storage at 4°C for 12 days, but 1.0 kGy effectively reduced respiration rates, and microbial growth up to 2-log fold. On the other hand, irradiation at 0.5 kGy extended shelf-life by four additional days compared to 1 kGy by improving appearance, aroma and flavor (Trigo et al., 2006). Similarly, maintenance of shelf-life and microbiological quality has been observed in fresh-cut watermelon exposed to gamma radiation during storage at 5°C for 8 days (Mohacsi-Farkas et al., 2006) and 7°C for 12 days (Landgraf et al., 2006).
Reduction of oxygen (O2) and increase of carbon dioxide (CO2) levels to 1%–5% and 3%–20%, respectively, have been reported as effective conditions for microbial inhibition and shelf-life maintenance of fresh produce (Kader and Watkins, 2000). MAP alters the initial gaseous composition inside of a package with no further control, whereas CA has a higher degree of control of gas levels (Lee et al., 1995; Farber et al., 2003; Wilson et al., 2019).
In fresh-cut watermelon, MAP storage with 3%–5% O2 and 10% CO2 has been recommended for preserving quality (Gorny, 2003). Smith et al. (2017) investigated the microbial counts and sensory and consumer acceptability of fresh-cut cubes placed in 5% O2, 10% CO2, and 85% Nitrogen (N2) MAP and stored at 4°C for 21 days. The proliferation of microorganisms was reduced, and firmness was maintained more effectively than air-stored (control) samples. Similarly, MAP conditions of 7 kPa CO2 + 18 kPa O2 + 85 kPa N2 reduced respiration, improved appearance and aroma and decreased juice and ion leakage in comparison to air-stored cubes at 5°C for 8 days (Lee, 2021). On the other hand, Mendoza-Enano et al. (2019) reported that regardless of the use of lidding films, MAP conditions of 5% O2, 10% CO2, and 85% N2 did not improve consumer acceptability scores of cut samples stored at 3°C after 6 days.
Cartaxo and Sargent, (1998) monitored fresh-cut watermelon samples stored under CA conditions. Cubes were placed into sealed jars connected to a humidified flow-through gas system at 3% O2 + 5, 10, 15 or 20% CO2 for 15 days at 3°C. The combination of 3% O2 and 10%–20% CO2 controlled microbial growth and maintained firmness, but increased juice leakage and induced a dark and dull water-soaked appearance compared to other treatments. These observations suggest the occurrence of physiological injury by either low O2, high CO2 or a combination of both.
The advent of omics technologies, i.e., genomics, transcriptomics, metabolomics and epigenomics has become a powerful tool for identifying targets for improving the quality and shelf-life of fresh produce (Habibi et al., 2024), including watermelon (Guo et al., 2015; Gao et al., 2020). While most of the current reports have focused on improving the sensory quality of whole fruit, research on the maintenance of quality traits during the postharvest storage of fresh-cut watermelon remains largely unexplored.
Texture is a complex trait that has significantly benefited from omics-based approaches. Gao et al. (2020) investigated the molecular mechanisms underlying texture during watermelon fruit development in two varieties, “PI186490” (white pulp, bitter, dense and hard pulp, green rind) and “Sanbai” (white and soft pulp, white rind). “PI186490” presented higher firmness and cellulose, hemicellulose and pectin contents than “Sanbai”. Comparative transcriptomic analysis revealed that among the 10 pectin esterase genes identified, Cla004896, Cla014927, Cla015505 and Cla023049 were differentially expressed during development and upregulated in “PI186490” relative to “Sanbai”. A similar trend was observed for two differentially expressed PG genes (Cla009218 and Cla010077) and one PL gene (Cla002573). Anees et al. (2021) carried out weighted genes co-expression network analysis (WGCNA) of a near isogeneic line with high average flesh firmness “HWF” and an inbred line with low average flesh firmness “203Z”. The study revealed that three main gene networks were correlated with water-soluble pectin, cellulose, hemicellulose, and protopectin, all pertinent to the structural integrity and firmness of watermelon fruit. A total of eight genes were identified as involved in cell wall biosynthesis and ethylene signaling. Linkage mapping and comparative transcriptome analysis identified key genes controlling center flesh firmness located on chromosomes 2 and 8 respectively: Cla012507, a MADS-box transcription factor and potential candidate gene regulating cell wall contents, and ClERF1, predicted to encode an ethylene-responsive transcription factor (Zhou et al., 2024). In another study, Genome-wide association studies (GWAS) and bulked segregant RNA-Seq analysis (BSR-Seq) identified an auxin-responsive gene (Aux/IAA) associated with flesh firmness on chromosome 6 (Anees et al., 2024).
Genome-wide comparative expression analysis of the carotenoid biosynthesis pathway has revealed gene regulatory networks associated with flesh color. Li et al. (2020) reported that Cla018767, Cla018768, Cla018769, Cla018770, and Cla018771 were linked to red-flesh color development in watermelon via the lycopene biosynthesis pathway. The lycopene β-cyclase, ClCYB gene, was identified as a key regulator of red flesh color (Wang et al., 2019). Downregulation of ClCYB via an antisense construct in a pale yellow-fleshed line changed the color to red, whereas ClCYB overexpression in a red-fleshed line, induced an orange color development accompanied by diminished lycopene accumulation (Zhang et al., 2020). Similarly, quantitative trait loci (QTL) analysis has contributed evidence to the identification of genomic regions responsible for watermelon fruit color (Mashilo et al., 2023). In two Korean inbred watermelon lines with unique color and fruit-type characteristics, 15 QTLs associated with fruit quality-related traits were identified, two and four for lycopene content and flesh color, respectively. Fine-mapping identified 33 genes, including Cla97C01G008760, annotated as a phytoene synthase, a candidate gene that is crucial for the regulation of flesh color (Nie et al., 2023). Shahwar et al. (2024) used GWAS and genotyping-by-sequencing (GBS)-based QTL analysis on 130 watermelon recombinant inbred lines. The study identified a major QTL on chromosome 4, qFC-4.1, as well as Cla97C04G070940 (lycopene β-cyclase, LCYB) and Cla97C02G039880 (pentatricopeptide repeat, PPR) as candidate genes for flesh color.
Flavor is one of the main traits determining watermelon consumption and a relevant target of breeding programs (Mashilo et al., 2022; Liu et al., 2024). Comparative transcriptome analysis revealed that the genes Cla97C01G013600, Cla97C05G089700, Cla97C01G001290, Cla97C05G095170, and Cla97C06G118330, involved in alcohol and aldehyde (ADH) biosynthesis, enhanced flavor and aroma formation of ripe watermelon fruit (Gong et al., 2021). In a study by Peng et al. (2025), differentially expressed genes involved in sugar and organic acid metabolic pathways were identified in four commercial watermelon genotypes, including trehalose-phosphate synthase/phosphatase genes Cla97C11G223240 (TPS1) and Cla97C07G136350 (TPPJ), sucrose synthase genes Cla97C10G194010 (SUS2) and Cla97C02G041890.
Fresh-cut processing promotes unique physiological changes in watermelon that significantly alter its storability compared to intact fruit. This presents an opportunity for omics research to investigate the dynamics of quality-related traits during postharvest storage.
This article reviewed relevant studies on quality changes, technological interventions and emerging approaches to extend the shelf-life of fresh-cut watermelon. However, further research is imperative to gain a more comprehensive understanding of the biological mechanisms that determine quality loss. This knowledge is crucial for developing effective strategies to reduce postharvest losses and waste.
Research efforts to understand the mechanisms underlying fresh-cut watermelon quality decline have increased in the past two decades. Early studies primarily focused on characterizing the changes in sensory attributes such as color, flavor, and texture during postharvest storage (Fonseca et al., 1999; Perkins-Veazie and Collins, 2004; Gil et al., 2006). In the last 10 years, there has been a shift towards investigating the use of exogenous treatments to delay quality loss (Sipahi et al., 2013; Šimović et al., 2014; Ebabhi et al., 2019; Song et al., 2023; Jacuinde-Guzmán et al., 2024) and a greater focus on the role of aroma volatiles in consumer acceptance (Xisto et al., 2012; Mendoza-Enano et al., 2019). Nevertheless, only a few reports have examined the biological mechanisms involved in texture-related defects like juice leakage, beyond the influence of the hormone ethylene (Petrou et al., 2013) (Figure 3). Given the importance of texture on the sensory experience of fresh-cut produce (Barrett et al., 2010) and the role of juice leakage in consumer rejection of fresh-cut watermelon (Mao et al., 2006; Mphahlele et al., 2020; Lee, 2021), further studies in this direction are needed.
From a nutritional perspective, there has been a strong focus on investigating the changes in total polyphenols, ascorbic acid and lycopene content during postharvest storage (Perkins-Veazie and Collins, 2004; Gil et al., 2006), but other bioactive compounds have received considerably less attention. Watermelon represents a valuable source of citrulline, a non-essential, non-proteinogenic amino acid, as well as Vitamin A, flavonoids and β-carotene (Sorokina et al., 2021). Rising consumer awareness of the nutritional content of fresh produce represents an opportunity to explore approaches to improve the retention of these phytonutrients during postharvest storage in fresh-cut watermelon.
Numerous strategies to improve fresh-cut watermelon quality have been documented in the literature (Table 1). Pre-harvest and post-harvest practices and physical and chemical treatments applied before or after processing present advantages but also limitations that must be addressed to develop long-term solutions to quality loss. Most of the chemically-based treatments have been tested on the cut ready-to-eat fruit which raises legitimate concerns about regulations on dosage and concentration, potential toxicity and public health risks. Alternatively, exploring the use of elicitation approaches applied to whole watermelon fruit before processing could expand the range of available options to address quality loss. Elicitors are stress factors that can be applied in a controlled manner to the plant during cultivation, i.e., preharvest, or postharvest. They trigger stress responses by activating the synthesis and accumulation of secondary metabolites responsible for protecting cells against damage (Baenas et al., 2014). Exposure of whole fruits to elicitors has been previously tested in melon and resulted in quality maintenance during storage (Atress and Attia, 2011; Liu et al., 2022).
Many exogenous treatments have been tested at the laboratory scale, providing valuable insights into the quality traits affected by fresh-cut processing and physical damage (Table 1). However, translational research is imperative for enhancing industry competitiveness by converting these findings into practical applications. Analysis of the sustainability and environmental impact of the proposed technologies are commonly overlooked, and knowledge gaps persist. The scarcity of available cost reports presents an opportunity to conduct research that could inform on the economic feasibility of implementing these methods. As pointed out by Lee (2021), the processing industry is receptive to incorporating technologies that involve existing equipment and infrastructure. In this context, the storage of watermelon fruit under optimal temperature conditions—pre- and post-cut—still represents the most viable intervention to maintain the quality of the fresh-cut product.
Technological interventions to extend fresh-cut watermelon shelf-life often have trade-offs, as some traits are improved while others are impaired. Additional research involving sensory evaluations could optimize the development of solutions to quality loss that are aligned with market and consumer demands.
Gene editing through CRISPR-Cas9 could further expand our understanding of complex traits like firmness and flavor (Shipman et al., 2021), which are greatly compromised by fresh-cut processing. (Huang et al., 2011; Liu et al., 2016). Research efforts have advanced in this area by exploring methods that increase both transformation and editing efficiencies (Tian et al., 2017; Pan et al., 2022; Wang et al., 2024). Nevertheless, gene editing approaches specifically oriented to improve fresh-cut watermelon quality and postharvest traits are still in the early stages of development. Leveraging this tool in conjunction with omics technologies holds significant potential to develop robust, long-term solutions for mitigating quality loss during storage and extending shelf-life.
A multidisciplinary approach, integrating applied, molecular, and biotechnological resources, can provide a robust understanding of the regulatory mechanisms underlying fresh-cut quality decline, which can then be effectively integrated into breeding programs. Enhanced quality will not only expand market access, stimulate consumption, and drive sales growth but also contribute to food losses and waste reduction.
Consumer interest in fresh-cut watermelon, as an alternative to whole fruit is increasing as it meets demand for convenience. However, fresh-cut operations induce stress and trigger biological processes that ultimately lead to quality deterioration. Color loss, bioactive compounds decline, and textural alterations underscore organoleptic and nutritional quality traits impacted by processing. The literature has reported a strong influence of the genotype on the differences in the rate and magnitude of quality decline for most traits. To address the challenges imposed by quality deterioration in fresh-cut watermelon, different approaches for shelf-life extension have been tested in the last decades. Pre-harvest interventions, like grafting on different rootstocks improved or maintained the firmness of the resulting fresh-cut product. Postharvest techniques applied pre- or post-cutting, such as irradiation or chemical treatments, had heterogeneous effects in maintaining quality and ensuring microbiological safety when used alone or in combination. Low-temperature storage improved organoleptic quality retention during storage, consistently reducing microbial growth. The application of edible coatings and controlled and modified atmosphere packaging were effective in maintaining some quality traits but led to trade-offs in other aspects. Overall, treatment effectiveness has been disparate, thus highlighting the need for additional research efforts on alternative methods for quality retention that are cost-effective. The increased application of gene editing and omics technologies to watermelon represents a promising opportunity to shorten knowledge gaps on the molecular basis of quality loss, and to develop improved cultivars for the fresh-cut market that complement current breeding efforts.
EQ: Conceptualization, Data curation, Formal Analysis, Investigation, Methodology, Visualization, Writing–original draft, Writing–review and editing. KA: Conceptualization, Data curation, Formal Analysis, Investigation, Methodology, Visualization, Writing–original draft, Writing–review and editing, Funding acquisition, Project administration, Resources, Supervision, Validation.
The author(s) declare that financial support was received for the research, authorship, and/or publication of this article. Technical Contribution No. 7373 of the Clemson University Experiment Station. This material is based upon work supported by NIFA/USDA, under project numbers SC-1700674 and SC-1700666.
We are grateful to Dr. Richard Hassell and Mr. Lee Wroten for their valuable input during the preparation of this manuscript.
The authors declare that the research was conducted in the absence of any commercial or financial relationships that could be construed as a potential conflict of interest.
The author(s) declare that no Generative AI was used in the creation of this manuscript.
All claims expressed in this article are solely those of the authors and do not necessarily represent those of their affiliated organizations, or those of the publisher, the editors and the reviewers. Any product that may be evaluated in this article, or claim that may be made by its manufacturer, is not guaranteed or endorsed by the publisher.
Aguayo, E., Gómez, E., Artés, F., Escalona, V. H., and Silveria, A. C. (2013). Hot water calcium dips to improve quality of fresh-cut watermelon. Acta Hortic. (1012), 1013–1019. doi:10.17660/ActaHortic.2013.1012.136
Aitbayeva, A., Zorzhanov, B., Mamyrbekov, Z., Absatarova, D., Rakhymzhanov, B., and Koshmagambetova, M. (2021). Comparison of different types of fertilizers on growth, yield and quality properties of watermelon (Citrullus lanatus) in the Southeast of Kazakhstan. Eurasian J. Soil Sci. 10 (4), 302–307. doi:10.18393/ejss.959078
Ajami, V., and Nemati, S. H. (2024). Investigating the effect of different concentrations of salicylic acid and citric acid on some quality characteristics and microbial load of fresh cut watermelon. J. Hortic. Sci. Prepr. doi:10.22067/jhs.2024.88902.1361
Anees, M., Gao, L., Gong, C., Umer, M. J., Yuan, P., Zhu, H., et al. (2023). Aux/IAA gene Cla004102, is involved in synergistic regulation of various endogenous hormones, regulating flesh firmness in watermelon. Sci. Hortic. 310, 111719. doi:10.1016/j.scienta.2022.111719
Anees, M., Gao, L., Umer, M. J., Yuan, P., Zhu, H., Lu, X., et al. (2021). Identification of key gene networks associated with cell wall components leading to flesh firmness in watermelon. Front. Plant Sci. 12, 630243. doi:10.3389/fpls.2021.630243
Anees, M., Zhu, H., Umer, M. J., Gong, C., Yuan, P., Lu, X., et al. (2024). Identification of an Aux/IAA regulator for flesh firmness using combined GWAS and bulked segregant RNA-Seq analysis in watermelon. Hortic. Plant J. 10 (5), 1198–1213. doi:10.1016/j.hpj.2023.05.018
Artés-Hernández, F., Robles, P. A., Gómez, P. A., Tomás-Callejas, A., and Artés, F. (2010). Low UV-C illumination for keeping overall quality of fresh-cut watermelon. Postharvest Biol. Technol. 55 (2), 114–120. doi:10.1016/j.postharvbio.2009.09.002
Artés-Hernández, F., Robles, P. A., Gómez, P. A., Tomás-Callejas, A., Artés, F., and Martínez-Hernández, G. B. (2021). Quality changes of fresh-cut watermelon during storage as affected by cut intensity and UV-C pre-treatment. Food Bioprocess Technol. 14 (3), 505–517. doi:10.1007/s11947-021-02587-1
Atress, A. S. H., and Attia, M. M. (2011). Effect of 1-Methylcyclopropene and hot water treatments on retarding ripeness of cantaloupe fruits during cold storage. Egypt. J. Agric. Res. 89 (3), 1089–1103. doi:10.21608/ejar.2011.177630
Babic, I., Amiot, M. j., Nguyen-The, C., and Aubert, S. (1993). Changes in phenolic content in fresh ready-to-use shredded carrots during storage. J. Food Sci. 58 (2), 351–356. doi:10.1111/j.1365-2621.1993.tb04273.x
Baenas, N., García-Viguera, C., and Moreno, D. A. (2014). Elicitation: a tool for enriching the bioactive composition of foods. Molecules 19 (9), 13541–13563. doi:10.3390/molecules190913541
Bai, Y., Li, J., Huang, M., Yan, S., Li, F., Xu, J., et al. (2024). Prevalence and characterization of foodborne pathogens isolated from fresh-cut fruits and vegetables in Beijing, China. Int. J. Food Microbiol. 421, 110804. doi:10.1016/j.ijfoodmicro.2024.110804
Barrett, D. M., Beaulieu, J. C., and Shewfelt, R. (2010). Color, flavor, texture, and nutritional quality of fresh-cut fruits and vegetables: desirable levels, instrumental and sensory measurement, and the effects of processing. Crit. Rev. Food Sci. Nutr. 50 (5), 369–389. doi:10.1080/10408391003626322
Barth, M. M., Kerbel, E. L., Perry, A. K., and Schmidt, S. J. (1993). Modified atmosphere packaging affects ascorbic acid, enzyme activity and market quality of broccoli. J. Food Sci. 58 (1), 140–143. doi:10.1111/j.1365-2621.1993.tb03230.x
Beaulieu, J. C., and Lea, J. M. (2006). Characterization and semiquantitative analysis of volatiles in seedless watermelon varieties using solid-phase microextraction. J. Agric. Food Chem. 54 (22), 8654. doi:10.1021/jf0680135
Braccini, I., and Pérez, S. (2001). Molecular basis of C(2+)-induced gelation in alginates and pectins: the egg-box model revisited. Biomacromolecules 2 (4), 1089–1096. doi:10.1021/bm010008g
Brecht, J. K., Saltveit, M. E., Talcott, S. T., Schneider, K. R., Felkey, K., and Bartz, J. A. (2003). “Fresh-cut vegetables and fruits,” in Horticultural reviews (John Wiley and Sons, Ltd), 185–251. doi:10.1002/9780470650837.ch6
Cartaxo, C. B., and Sargent, S. A. (1998). “Controlled atmosphere storage suppresses microbial growth on fresh-cut watermelon,” in Proceedings of the annual meeting of the Florida state horticultural society.
Clark, J. E. (1998). Taste and flavour: their importance in food choice and acceptance. Proc. Nutr. Soc. 57 (4), 639–643. doi:10.1079/PNS19980093
Das, K., Tiwari, R., and Shrivastava, D. (2010). Techniques for evaluation of medicinal plant products as antimicrobial agent: current methods and future trends. J. Med. plants Res. 4 (2), 104–111. doi:10.5897/JMPR09.030
Davis, A. R., and King, S. R. (2007). MSW-28, a full-flavor crisp watermelon line with high lycopene and medium brix. HortScience 42, 1715–1716. doi:10.21273/HORTSCI.42.7.1715
Del Rosario, B. A., and Beuchat, L. R. (1995). Survival and growth of enterohemorrhagic Escherichia coli O157:H7 in cantaloupe and watermelon. J. Food Prot. 58 (1), 105–107. doi:10.4315/0362-028X-58.1.105
Diehl, J. F. (2002). Food irradiation—past, present and future. Radiat. Phys. Chem. 63 (3), 211–215. doi:10.1016/S0969-806X(01)00622-3
Dokhanieh, A. Y., Aghdam, M. S., Fard, J. R., and Hassanpour, H. (2013). Postharvest salicylic acid treatment enhances antioxidant potential of cornelian cherry fruit. Sci. Hortic. 154, 31–36. doi:10.1016/j.scienta.2013.01.025
Dunn, J., Ott, T., and Clark, W. (1995). Pulsed-light treatment of food and packaging. Food Technol. Chic. 49 (9), 95–98.
Ebabhi, A. M., Adeogun, O. O., Adekunle, A. A., and A. O, O. (2019). Bio-preservation potential of leaf extracts of ocimum gratissimum L. On fresh-cut fruits of Citrullus lanatus (thunb). J. Appl. Sci. Environ. Manag. 23 (7), 1383–1389. doi:10.4314/jasem.v23i7.30
El Khetabi, A., Lahlali, R., Ezrari, S., Radouane, N., Lyousfi, N., Banani, H., et al. (2022). Role of plant extracts and essential oils in fighting against postharvest fruit pathogens and extending fruit shelf life: a review. Trends Food Sci. and Technol. 120, 402–417. doi:10.1016/j.tifs.2022.01.009
Elmnasser, N., Guillou, S., Leroi, F., Orange, N., Bakhrouf, A., and Federighi, M. (2007). Pulsed-light system as a novel food decontamination technology: a review. Can. J. Microbiol. 53 (7), 813–821. doi:10.1139/W07-042
Fan, X., and Sokorai, K. J. B. (2005). Assessment of radiation sensitivity of fresh-cut vegetables using electrolyte leakage measurement. Postharvest Biol. Technol. 36 (2), 191–197. doi:10.1016/j.postharvbio.2004.12.004
FAOSTAT (2022). Food and agriculture organization. Available at: https://www.fao.org/faostat/en/#data/QI (Accessed September 4, 2024).
Farber, J., Harris, L., Parish, M., Beuchat, L., Suslow, T., Gorney, J., et al. (2003). Microbiological safety of controlled and modified atmosphere packaging of fresh and fresh-cut produce. Compr. Rev. food Sci. food Saf. 2, 142–160. doi:10.1111/j.1541-4337.2003.tb00032.x
Feng, K., Hu, W., Jiang, A., Saren, G., Xu, Y., Ji, Y., et al. (2017). Growth of Salmonella spp. and Escherichia coli O157:H7 on fresh-cut fruits stored at different temperatures. Foodborne Pathogens Dis. 14 (9), 510–517. doi:10.1089/fpd.2016.2255
Fleming, P., Pool, B., and Gorny, J. R. (2005). Commodity specific food safety guidelines for the melon supply chain. Available at: https://www.fda.gov/media/116691/download (Accessed September 30, 2024).
Fonseca, J. M., and Rushing, J. W. (2006). Effect of ultraviolet-C light on quality and microbial population of fresh-cut watermelon. Postharvest Biol. Technol. 40 (3), 256–261. doi:10.1016/j.postharvbio.2006.02.003
Fonseca, J. M., Rushing, J. W., and Testin, R. F. (1999). “Shock and vibration forces influence the quality of fresh-cut watermelon”, in Proceedings of the Florida State Horticultural Society, 147–152.
Fonseca, J. M., Rushing, J. W., and Testin, R. F. (2004). The anaerobic compensation point for fresh-cut watermelon and implications for postprocess handling. HortScience 39, 562–566. doi:10.21273/HORTSCI.39.3.562
Food and Drug Administration (2002). Ultraviolet radiation for the processing and treatment of food. Maryland, United States: Code of Federal Regulations. Vol. 21.
Food and Drug Administration (2018). FDA Fast Facts: FDA works with partners to investigate Salmonella outbreak linked to pre-cut melon. Available at: https://www.fda.gov/news-events/fda-newsroom/fda-fast-facts-fda-works-partners-investigate-salmonella-outbreak-linked-pre-cut-melon (Accessed October 1, 2024).
Francis, G. A., Gallone, A., Nychas, G. J., Sofos, J. N., Colelli, G., Amodio, M. L., et al. (2012). Factors affecting quality and safety of fresh-cut produce. Crit. Rev. Food Sci. Nutr. 52 (7), 595–610. doi:10.1080/10408398.2010.503685
Fredes, A., Cebolla-Cornejo, J., Beltrán, J., Gisbert, C., Picó, B., Valcárcel, M., et al. (2024). Indirect evaluation of watermelon volatile profile: detection of subtle changes with e-nose systems. LWT 203, 116337. doi:10.1016/j.lwt.2024.116337
Gao, Y., Guo, Y., Su, Z., Yu, Y., Zhu, Z., Gao, P., et al. (2020). Transcriptome analysis of genes related to fruit texture in watermelon. Sci. Hortic. 262, 109075. doi:10.1016/j.scienta.2019.109075
Gidado, M. J., Gunny, A. A. N., Gopinath, S. C. B., Ali, A., Wongs-Aree, C., and Salleh, N. H. M. (2024). Challenges of postharvest water loss in fruits: mechanisms, influencing factors, and effective control strategies – a comprehensive review. J. Agric. Food Res. 17, 101249. doi:10.1016/j.jafr.2024.101249
Gil, M. I., Aguayo, E., and Kader, A. A. (2006). Quality changes and nutrient retention in fresh-cut versus whole fruits during storage. J. Agric. Food Chem. 54 (12), 4284–4296. doi:10.1021/jf060303y
Glenn, G. M., and Poovaiah, B. W. (1990). Calcium-mediated postharvest changes in texture and cell wall structure and composition in `Golden delicious apples. J. Am. Soc. Hortic. Sci. 115, 962–968. doi:10.21273/JASHS.115.6.962
Gombas, D., Luo, Y., Brennan, J., Shergill, G., Petran, R., Walsh, R., et al. (2017). Guidelines to validate control of cross-contamination during washing of fresh-cut leafy vegetables. J. Food Prot. 80 (2), 312–330. doi:10.4315/0362-028X.JFP-16-258
Gómez, P. A., Robles, P. A., Tomás-Callejas, A., Otón, M., Artés, F., and Artés-Hernández, F. (2017). UV-C light preserves quality of minimally processed watermelon cylinders. Acta Hortic. (1151), 279–286. doi:10.17660/ActaHortic.2017.1151.43
Gong, C., Diao, W., Zhu, H., Umer, M. J., Zhao, S., He, N., et al. (2021). Metabolome and transcriptome integration reveals insights into flavor formation of “crimson” watermelon flesh during fruit development. Front. Plant Sci. 12, 629361. doi:10.3389/fpls.2021.629361
Gong, T., Li, C., Bian, B., Wu, Y., Dawuda, M. M., and Liao, W. (2018). Advances in application of small molecule compounds for extending the shelf life of perishable horticultural products: a review. Sci. Hortic. 230, 25–34. doi:10.1016/j.scienta.2017.11.013
Gorny, J. R. (2003). A summary of Ca and ma requirements and recommendations for fresh-cut (minimally processed) fruits and vegetables. Acta Hortic. (600), 609–614. doi:10.17660/ActaHortic.2003.600.92
Guo, S., Sun, H., Zhang, H., Liu, J., Ren, Y., Gong, G., et al. (2015). Comparative transcriptome analysis of cultivated and wild watermelon during fruit development. PloS One 10 (6), e0130267. doi:10.1371/journal.pone.0130267
Habibi, F., Boakye, D. A., Chang, Y., Casorzo, G., Hallman, L. M., Madison, M., et al. (2024). Molecular mechanisms underlying postharvest physiology and metabolism of fruit and vegetables through multi-omics technologies. Sci. Hortic. 324, 112562. doi:10.1016/j.scienta.2023.112562
Han, J. H. (2014). “Chapter 9 - edible films and coatings: a review,” in Innovations in food packaging. Editor J. H. Han Second Edition (San Diego: Academic Press), 213–255. Food Science and Technology). doi:10.1016/B978-0-12-394601-0.00009-6
Henning, A. (1997). Cutting with high-pressure jet in the food industry. Available at: https://publica.fraunhofer.de/handle/publica/190100 (Accessed September 9, 2024).
Hodges, D. M., and Toivonen, P. M. A. (2008). Quality of fresh-cut fruits and vegetables as affected by exposure to abiotic stress. Postharvest Biol. Technol. 48 (2), 155–162. doi:10.1016/j.postharvbio.2007.10.016
Hong, M. Y., Hartig, N., Kaufman, K., Hooshmand, S., Figueroa, A., and Kern, M. (2015). Watermelon consumption improves inflammation and antioxidant capacity in rats fed an atherogenic diet. Nutr. Res. 35 (3), 251–258. doi:10.1016/j.nutres.2014.12.005
Hu, H., Li, P., Wang, Y., and Gu, R. (2014). Hydrogen-rich water delays postharvest ripening and senescence of kiwifruit. Food Chem. 156, 100–109. doi:10.1016/j.foodchem.2014.01.067
Hu, W., Guan, Y., Ji, Y., and Yang, X. (2021). Effect of cutting styles on quality, antioxidant activity, membrane lipid peroxidation, and browning in fresh-cut potatoes. Food Biosci. 44, 101435. doi:10.1016/j.fbio.2021.101435
Hu, W., and Jiang, Y. (2007). Quality attributes and control of fresh-cut produce. Stewart Postharvest Rev. 3 (2), 1–9. doi:10.2212/spr.2007.2.3
Hu, Y., Cai, Y., Wang, H., Xiong, Y., Zhang, X., Wei, L., et al. (2022). Systematic study of the sensory quality, metabolomics, and microbial community of fresh-cut watermelon provides new clues for its quality control and preservation. Foods 11 (21), 3423. doi:10.3390/foods11213423
Huang, J., Zou, T., Hu, H., Xiao, X., Wang, Z., Li, M., et al. (2022). Automatic brix measurement for watermelon breeding. Appl. Sci. 12 (23), 12227. doi:10.3390/app122312227
Huang, Y.-C., Chiang, C.-H., Li, C.-M., and Yu, T.-A. (2011). Transgenic watermelon lines expressing the nucleocapsid gene of Watermelon silver mottle virus and the role of thiamine in reducing hyperhydricity in regenerated shoots. Plant Cell, Tissue Organ Cult. (PCTOC) 106 (1), 21–29. doi:10.1007/s11240-010-9889-z
Ilahy, R., Tlili, I., Siddiqui, M. W., Hdider, C., and Lenucci, M. S. (2019). Inside and beyond color: comparative Overview of functional quality of tomato and watermelon fruits. Front. Plant Sci. 10, 769. doi:10.3389/fpls.2019.00769
Incardona, A., Fatchurrahman, D., Amodio, M. L., Peruzzi, A., and Colelli, G. (2024). Effect of cutting blade sharpness on physical and nutritional quality of fresh-cut ’golden delicious apples. Horticulturae 10 (9), 955. doi:10.3390/horticulturae10090955
Iturralde-García, R. D., Cinco-Moroyoqui, F. J., Martínez-Cruz, O., Ruiz-Cruz, S., Wong-Corral, F. J., Borboa-Flores, J., et al. (2022). Emerging technologies for prolonging fresh-cut fruits’ quality and safety during storage. Horticulturae 8 (8), 731. doi:10.3390/horticulturae8080731
Jacuinde-Guzmán, J. K., Escalona-Buendía, H. B., Barbosa-Martínez, C., Rivera-Cabrera, F., Raddatz-Mota, D., Soriano-Melgar, L., et al. (2024). The potential of calcium nanoparticles in posthaverst conservation of fresh-cut seedless watermelon (Citrullus lanatus). Postharvest Biol. Technol. 216, 113069. doi:10.1016/j.postharvbio.2024.113069
Jaiswal, M., and Srivastava, B. (2024). Evaluating the impact of pulsed light treatment on microbial and enzyme inactivation and quality attributes in fresh-cut watermelon. J. Food Process Eng. 47 (5), e14626. doi:10.1111/jfpe.14626
Jing-Hua, Y., Yuan, G., Yan-Man, L., Xiao-Hua, Q., and Zhang, M.-F. (2008). Salicylic acid-induced enhancement of cold tolerance through activation of antioxidative capacity in watermelon. Sci. Hortic. 118 (3), 200–205. doi:10.1016/j.scienta.2008.06.015
Kader, A. A. (2002). “Postharvest biology and technology: an Overview,” in Postharvest technology of horticultural crops. Third Edition (University of California Agriculture and Natural Resources), 39–47.
Kader, A. A., and Watkins, C. B. (2000). Modified atmosphere packaging—toward 2000 and beyond. Horttechnology 10, 483–486. doi:10.21273/HORTTECH.10.3.483
Kahan, D. (2013). Economics for farm management extension. FAO. Available at: https://openknowledge.fao.org/handle/20.500.14283/i3228e (Accessed September 30, 2024).
Karakurt, Y., and Huber, D. J. (2003). Activities of several membrane and cell-wall hydrolases, ethylene biosynthetic enzymes, and cell wall polyuronide degradation during low-temperature storage of intact and fresh-cut papaya (Carica papaya) fruit. Postharvest Biol. Technol. 28 (2), 219–229. doi:10.1016/S0925-5214(02)00177-1
Karakurt, Y., and Huber, D. J. (2004). Ethylene-induced gene expression, enzyme activities, and water soaking in immature and ripe watermelon (Citrullus lanatus) fruit. J. Plant Physiology 161 (4), 381–388. doi:10.1078/0176-1617-01221
Kaveh, H. (2016). Effect of saffron petal extract on retention quality of fresh-cut watermelon cubes. saffron Agron. Technol. 4. doi:10.22048/jsat.2016.38667
Kyriacou, M. C., Leskovar, D. I., Colla, G., and Rouphael, Y. (2018). Watermelon and melon fruit quality: the genotypic and agro-environmental factors implicated. Sci. Hortic. 234, 393–408. doi:10.1016/j.scienta.2018.01.032
Landgraf, M., Goularte, L., Martins, C., Cestari, A., Nunes, T., Aragonalegro, L., et al. (2006). Use of irradiation to improve the microbiological safety of minimally processed fruits and vegetables’, Use of irradiation to ensure hygienic quality of fresh, pre-cut fruits and vegetables and other minimally processed food of plant origin. Vienna: IAEA, 41–59.
Lee, L., Arul, J., Lencki, R., and Castaigne, F. (1995). A review on modified atmosphere packaging and preservation of fresh fruits and vegetables: physiological basis and practical aspects—Part I. Packag. Technol. Sci. 8 (6), 315–331. doi:10.1002/pts.2770080605
Lee, Y. Z. (2021). Improving quality maintenance of fresh-cut watermelons. Doctoral Dissertation. Tasmania: University of Tasmania. doi:10.25959/23250422.v1
Lee, Y. Z., Wilson, M., Ross, T., Davies, N., and Stanley, R. A. (2018). Effects of pre- and post-cut storage temperatures on fresh-cut watermelon quality and shelf-life. Acta Hortic., 249–256. doi:10.17660/ActaHortic.2018.1210.35
Lewandowski, J. (2001). “Physical control methods in plant protection”, in Physical Control Methods in Plant Protection (Springer). 1st Edn., 111–124.
Li, D., Friedrich, L. M., Danyluk, M. D., Harris, L. J., and Schaffner, D. W. (2013). Development and validation of a mathematical model for growth of pathogens in cut melons. J. Food Prot. 76 (6), 953–958. doi:10.4315/0362-028X.JFP-12-398
Li, Na, Shang, J., Wang, J., Zhou, D., Li, N., and Ma, S. (2020). Discovery of the genomic region and candidate genes of the scarlet red flesh color (scr) locus in watermelon (Citrullus lanatus L.). Front. Plant Sci. 11, 116. doi:10.3389/fpls.2020.00116
Li, P., Yan, J., Cong, F., Zhang, X., and Cui, J. (2019). Effects of cinnamon oil and its inclusion complex with β-cyclodextrin on preserving the quality of fresh-cut watermelon. Food Sci. 40 (9), 276–282. doi:10.7506/spkx1002-6630-20180416-217
Li, X., Li, B., Li, M., Fu, X., Zhao, X., Min, D., et al. (2022). Ethylene pretreatment induces phenolic biosynthesis of fresh-cut pitaya fruit by regulating ethylene signaling pathway. Postharvest Biol. Technol. 192, 112028. doi:10.1016/j.postharvbio.2022.112028
Lichanporn, I., Nanthachai, N., Tanganurat, P., and Singkhum, U. (2014). Effect of calcium ascorbate treatments on juice leakage of fresh cut watermelon (Citrullus lanatus). Int. J. Environ. Rural Dev. 5 (1), 171–175. doi:10.32115/ijerd.5.1_171
Liu, D., Zhou, W., Zhong, Y., Xie, X., Liu, H., Huang, H., et al. (2023). Involvement of branched RG-I pectin with hemicellulose in cell-cell adhesion of tomato during fruit softening. Food Chem. 413, 135574. doi:10.1016/j.foodchem.2023.135574
Liu, L., Gu, Q., Ijaz, R., Zhang, J., and Ye, Z. (2016). Generation of transgenic watermelon resistance to Cucumber mosaic virus facilitated by an effective Agrobacterium-mediated transformation method. Sci. Hortic. 205, 32–38. doi:10.1016/j.scienta.2016.04.013
Liu, S., Liu, Y., Wang, T., and Zhang, J. (2022). The effects of different light storage conditions on volatile flavor compounds and sensory properties of melon fruit. Food Biosci. 48, 101826. doi:10.1016/j.fbio.2022.101826
Liu, Y., He, C., and Song, H. (2018). Comparison of fresh watermelon juice aroma characteristics of five varieties based on gas chromatography-olfactometry-mass spectrometry. Food Res. Int. 107, 119–129. doi:10.1016/j.foodres.2018.02.022
Liu, Y., Keefer, H., Watson, M., and Drake, M. (2024). Consumer perception of whole watermelons. J. Food Sci. 89 (1), 625–639. doi:10.1111/1750-3841.16843
Liu, Xin, Q., Zhang, M., Chen, J., Lu, Q., Zhou, X., Li, X., et al. (2023). Research progress on mango post-harvest ripening physiology and the regulatory technologies. Foods 12 (1), 173. doi:10.3390/foods12010173
Lund, B. M., Baird-Parker, T. C., and Gould, G. W. (2000). Microbiological safety and quality of food. Springer Science and Business Media.
Mahendran, R., Ramanan, K. R., Barba, F. J., Lorenzo, J. M., López-Fernández, O., Munekata, P. E. S., et al. (2019). Recent advances in the application of pulsed light processing for improving food safety and increasing shelf life. Trends Food Sci. and Technol. 88, 67–79. doi:10.1016/j.tifs.2019.03.010
Man, L. V. V., and Huy, T. Q. (2008). Study on prolongation of watermelon fresh-cut shelf-life by ozone treatment. Sci. Technol. Dev. J. 11 (9), 77–82. doi:10.32508/stdj.v11i9.2690
Manivannan, A., Lee, E.-S., Han, K., Lee, H.-E., and Kim, D.-S. (2020). Versatile nutraceutical potentials of watermelon—a modest fruit loaded with pharmaceutically valuable phytochemicals. Molecules 25 (22), 5258. doi:10.3390/molecules25225258
Mao, L., Jeong, J., Que, F., and Huber, D. J. (2006). Physiological properties of fresh-cut watermelon (Citrullus lanatus) in response to 1-methylcyclopropene and post-processing calcium application. J. Sci. Food Agric. 86 (1), 46–53. doi:10.1002/jsfa.2297
Mao, L., Karakurt, Y., and Huber, D. J. (2004). Incidence of water-soaking and phospholipid catabolism in ripe watermelon (Citrullus lanatus) fruit: induction by ethylene and prophylactic effects of 1-methylcyclopropene. Postharvest Biol. Technol. 33 (1), 1–9. doi:10.1016/j.postharvbio.2003.12.007
Mashilo, J., Shimelis, H., Maja, D., and Ngwepe, R. M. (2022). Retrospective genetic analysis of qualitative and quantitative traits in sweet watermelon (Citrullus lanatus var. lanatus): a review. Agronomy 12 (7), 1633. doi:10.3390/agronomy12071633
Mashilo, J., Shimelis, H., Maja, D., and Ngwepe, R. M. (2023). Meta-analysis of qualitative and quantitative trait variation in sweet watermelon and citron watermelon genetic resources. Genet. Resour. Crop Evol. 70 (1), 13–35. doi:10.1007/s10722-022-01466-8
Mcglynn, W. G., Bellmer, D. D., and Reilly, S. S. (2003). Effect of precut sanitizing dip and water jet cutting on quality and shelf-life of fresh-cut watermelon. J. Food Qual. 26 (6), 489–498. doi:10.1111/j.1745-4557.2003.tb00263.x
Meghwar, P., Ghufran Saeed, S. M., Ullah, A., Nikolakakis, E., Panagopoulou, E., Tsoupras, A., et al. (2024). Nutritional benefits of bioactive compounds from watermelon: a comprehensive review. Food Biosci. 61, 104609. doi:10.1016/j.fbio.2024.104609
Mendoza-Enano, M. L., Stanley, R., and Frank, D. (2019). Linking consumer sensory acceptability to volatile composition for improved shelf-life: a case study of fresh-cut watermelon (Citrullus lanatus). Postharvest Biol. Technol. 154, 137–147. doi:10.1016/j.postharvbio.2019.03.018
Mohacsi-Farkas, C., Brueckner, A., Kisko, G., Agoston, R., Farkas, J., Andrassy, E., et al. (2006). Improving the microbiological safety of some fresh pre-cut and pre-packaged chilled produce by low-dose gamma irradiation. 1011–4289. International Atomic Energy Agency IAEA, 130–169. Available at: http://inis.iaea.org/search/search.aspx?orig_q=RN:38024794.
Mohamad Salin, N. S., Md Saad, W. M., Abdul Razak, H. R., and Salim, F. (2022). Effect of storage temperatures on physico-chemicals, phytochemicals and antioxidant properties of watermelon juice (Citrullus lanatus). Metabolites 12 (1), 75. doi:10.3390/metabo12010075
More, A. S., Ranadheera, C. S., Fang, Z., Warner, R., and Ajlouni, S. (2020). Biomarkers associated with quality and safety of fresh-cut produce. Food Biosci. 34, 100524. doi:10.1016/j.fbio.2019.100524
Moreira, J., Mera, E., Singh Chhetri, V., King, J. M., Gentimis, T., and Adhikari, A. (2023). Effect of storage temperature and produce type on the survival or growth of Listeria monocytogenes on peeled rinds and fresh-cut produce. Front. Microbiol. 14, 1151819. doi:10.3389/fmicb.2023.1151819
Mphahlele, R. R., Caleb, O. J., and Ngcobo, M. E. (2020). Effects of packaging and duration on quality of minimally processed and unpitted litchi cv. “Mauritius” under low storage temperature. Heliyon 6 (1), e03229. doi:10.1016/j.heliyon.2020.e03229
Myriah Mason, A., Meijun, Y., Kong, J., Ricaille, M., Yang, X., and Yu, Z. (2017). Physio-biochemical, quality and microbial characteristics: an analysis of watermelon cultivars for the fresh-cut industry. Int. J. Nutr. Food Sci. 6 (4), 158. doi:10.11648/j.ijnfs.20170604.12
Nadeem, M., Navida, M., Ameer, K., Siddique, F., Iqbal, A., Malik, F., et al. (2022). Watermelon nutrition profile, antioxidant activity, and processing. Food Sci. Preserv. 29 (4), 531–545. doi:10.11002/kjfp.2022.29.4.531
Nam, H.-A., Ramakrishnan, S. R., and Kwon, J.-H. (2019). Effects of electron-beam irradiation on the quality characteristics of Mandarin oranges (Citrus unshiu (Swingle) Marcov) during storage. Food Chem. 286, 338–345. doi:10.1016/j.foodchem.2019.02.009
National Watermelon Promotion Board (2024). Retail scan data. Florida. Natl. Watermelon Promot. Board, 1–7. Available at: https://www.watermelon.org/audiences/industry/research/retail-research/(Accessed September 6, 2024).
Nelson, J., Kuehl, C., and Prather, K. (2022). An economic analysis of market impacts of the national watermelon promotion board: 2017-2021. Florida: National Watermelon Promotion Board, 1–25.
Nie, H., Kim, M., Lee, S., Lim, S., Lee, M. S., Kim, J. H., et al. (2023). High-quality genome assembly and genetic mapping reveal a gene regulating flesh color in watermelon (Citrullus lanatus). Front. Plant Sci. 14, 1142856. doi:10.3389/fpls.2023.1142856
Nketiah, A., Quansah, J. K., and Kunadu, A.P.-H. (2024). Presence of carbapenem resistance in hybrid Escherichia coli pathovars from ready-to-eat fresh-cut fruits in Accra, Ghana. J. Appl. Microbiol. 135 (9), lxae239. doi:10.1093/jambio/lxae239
Oluka, N., Kugbenu, G., and Obire, O. (2024). Microbial quality of ready-to-eat pineapple (ananas comosus) and watermelon (Citrullus lanatus). Int. J. Microbiol. Appl. Sci. 3 (1), 362–374.
Pan, W., Cheng, Z., Han, Z., Yang, H., Zhang, W., and Zhang, H. (2022). Efficient genetic transformation and CRISPR/Cas9-mediated genome editing of watermelon assisted by genes encoding developmental regulators. J. Zhejiang Univ. Sci. B 23 (4), 339–344. doi:10.1631/jzus.B2200119
Pelloux, J., Rustérucci, C., and Mellerowicz, E. J. (2007). New insights into pectin methylesterase structure and function. Trends Plant Sci. 12 (6), 267–277. doi:10.1016/j.tplants.2007.04.001
Peng, Z., Song, S., Fu, D., Zhou, J., Chang, H., Wang, B., et al. (2025). Combined transcriptome and metabolome analysis reveals the mechanism of fruit quality formation in different watermelon (Citrullus lanatus) cultivars. Sci. Hortic. 339, 113797. doi:10.1016/j.scienta.2024.113797
Perkins-Veazie, P., Beaulieu, J. C., and Siddiq, M. (2012a). “Watermelon, cantaloupe and honeydew,” in Tropical and subtropical fruits; tropical and subtropical fruits: postharvest physiology, processing and packaging (John Wiley and Sons, Ltd), 549–568. doi:10.1002/9781118324097.ch28
Perkins-Veazie, P., and Collins, J. K. (2004). Flesh quality and lycopene stability of fresh-cut watermelon. Postharvest Biol. Technol. 31 (2), 159–166. doi:10.1016/j.postharvbio.2003.08.005
Perkins-Veazie, P., Collins, J. K., Pair, S. D., and Roberts, W. (2001). Lycopene content differs among red-fleshed watermelon cultivars. J. Sci. Food Agric. 81 (10), 983–987. doi:10.1002/jsfa.880
Perkins-Veazie, P., Davis, A., and Collins, J. K. (2012b) Watermelon: from dessert to functional food. doi:10.1560/IJPS.60.1.402
Petrou, P., Soteriou, G., Schouten, R. E., and Kyriacou, M. C. (2013). Effects of rind removal on physicochemical quality characteristics of fresh-cut watermelon [C (Thunb) Matsum and Nakai] during cold storage. Int. J. Food Sci. and Technol. 48 (2), 357–362. doi:10.1111/j.1365-2621.2012.03195.x
Portela, S., and Cantwell, M. (2001). Cutting blade sharpness affects appearance and other quality attributes of fresh-cut cantaloupe melon. J. Food Sci. 66, 1265–1270. doi:10.1111/j.1365-2621.2001.tb15199.x
Ragaert, P., Devlieghere, F., and Debevere, J. (2007). Role of microbiological and physiological spoilage mechanisms during storage of minimally processed vegetables. Postharvest Biol. Technol. 44 (3), 185–194. doi:10.1016/j.postharvbio.2007.01.001
Ramirez, J. L., Du, X., and Wallace, R. W. (2020). Investigating sensory properties of seven watermelon varieties and factors impacting refreshing perception using quantitative descriptive analysis. Food Res. Int. 138, 109681. doi:10.1016/j.foodres.2020.109681
Ramos-Villarroel, A. Y., Aron-Maftei, N., Martín-Belloso, O., and Soliva-Fortuny, R. (2012). Influence of spectral distribution on bacterial inactivation and quality changes of fresh-cut watermelon treated with intense light pulses. Postharvest Biol. Technol. 69, 32–39. doi:10.1016/j.postharvbio.2012.03.002
Ramos-Villarroel, A. Y., Martín-Belloso, O., and Soliva-Fortuny, R. (2015). Combined effects of malic acid dip and pulsed light treatments on the inactivation of Listeria innocua and Escherichia coli on fresh-cut produce. Food control. 52, 112–118. doi:10.1016/j.foodcont.2014.12.020
Rhim, J. W., Gennadios, A., Fu, D., Weller, C. L., and Hanna, M. A. (1999). Properties of ultraviolet irradiated protein films. LWT - Food Sci. Technol. 32 (3), 129–133. doi:10.1006/fstl.1998.0516
Roberts, W., Bruton, B. D., Popham, T. W., and Fish, W. W. (2005). Improving the quality of fresh-cut watermelon through grafting and rootstock selection. HortScience 40, 871d–871d. doi:10.21273/HORTSCI.40.3.871d
Saftner, R., Luo, Y., McEvoy, J., Abbott, J. A., and Vinyard, B. (2007). Quality characteristics of fresh-cut watermelon slices from non-treated and 1-methylcyclopropene- and/or ethylene-treated whole fruit. Postharvest Biol. Technol. 44 (1), 71–79. doi:10.1016/j.postharvbio.2006.11.002
Saltveit, M. E. (2002). “Fresh-cut vegetables,” in Postharvest physiology and pathology of vegetables (New York, NY: CRC Press). 2nd Edn., 779–803.
Sargent, S. (1998). Fresh-cut watermelon: new guidelines take cutting out of the produce department’s backroom’, Citrus and vegetable magazine, 26–36.
Shahwar, D., Park, G., Jang, G., Shin, J., Park, S., Kwon, G., et al. (2024). Genome-wide association study and quantitative trait loci mapping of fruit-related traits using recombinant inbred lines in watermelon. Sci. Hortic. 338, 113826. doi:10.1016/j.scienta.2024.113826
Shewfelt, R. L., and Rosario, B.A. del (2000). The role of lipid peroxidation in storage disorders of fresh fruits and vegetables. HortScience 35 (4), 575–579. doi:10.21273/HORTSCI.35.4.575
Shi, Y., Wang, Y., Ma, Y., Xu, Y., Zhao, X., and Zhang, C. (2020). “Red light exposure delays appearance and aroma deterioration of fresh-cut watermelon during retail display,”. Editor A. Hernández, 2020, 1–9. doi:10.1155/2020/1840648J. Food Qual.
Shipman, E. N., Yu, J., Zhou, J., Albornoz, K., and Beckles, D. M. (2021). Can gene editing reduce postharvest waste and loss of fruit, vegetables, and ornamentals? Hortic. Res. 8, 1. doi:10.1038/s41438-020-00428-4
Šimović, M., Delaš, F., Gradvol, V., Kocevski, D., and Pavlović, H. (2014). Antifungal effect of eugenol and carvacrol against foodborne pathogens Aspergillus carbonarius and Penicillium roqueforti in improving safety of fresh-cut watermelon. J. Intercult. Ethnopharmacol. 3 (3), 91–96. doi:10.5455/jice.20140503090524
Sipahi, R. E., Castell-Perez, M. E., Moreira, R. G., Gomes, C., and Castillo, A. (2013). Improved multilayered antimicrobial alginate-based edible coating extends the shelf life of fresh-cut watermelon (Citrullus lanatus). LWT - Food Sci. Technol. 51 (1), 9–15. doi:10.1016/j.lwt.2012.11.013
Smith, B., Ortega, A., Shayanfar, S., and Pillai, S. D. (2017). Preserving quality of fresh cut watermelon cubes for vending distribution by low-dose electron beam processing. Food control. 72, 367–371. doi:10.1016/j.foodcont.2016.02.017
Soliva-Fortuny, R. C., and Martı́n-Belloso, O. (2003). New advances in extending the shelf-life of fresh-cut fruits: a review. Trends Food Sci. and Technol. 14 (9), 341–353. doi:10.1016/S0924-2244(03)00054-2
Song, X., Wang, X., Zhang, H., Zhang, D., Li, Z., Wang, H. jiang, et al. (2023). Characterization of polysaccharide-based antibacterial films properties of loaded with Nisin and preservation of fresh-cut watermelon. Food Sci. Technol. 43, e127522. doi:10.1590/fst.127522
Sorokina, M., McCaffrey, K. S., Deaton, E. E., Ma, G., Ordovás, J. M., Perkins-Veazie, P. M., et al. (2021). A catalog of natural products occurring in watermelon—Citrullus lanatus. Front. Nutr. 8, 729822. doi:10.3389/fnut.2021.729822
Spadafora, N. D., Cocetta, G., Cavaiuolo, M., Bulgari, R., Dhorajiwala, R., Ferrante, A., et al. (2019). A complex interaction between pre-harvest and post-harvest factors determines fresh-cut melon quality and aroma. Sci. Rep. 9, 2745. doi:10.1038/s41598-019-39196-0
Sun, L., Zhang, Y., Cui, H., Zhang, L., Sha, T., Wang, C., et al. (2020). Linkage mapping and comparative transcriptome analysis of firmness in watermelon (Citrullus lanatus). Front. Plant Sci. 11, 831. doi:10.3389/fpls.2020.00831
Tadmor, Y., King, S., Levi, A., Davis, A., Meir, A., Wasserman, B., et al. (2005). Comparative fruit colouration in watermelon and tomato. Food Res. Int. 38 (8), 837–841. doi:10.1016/j.foodres.2004.07.011
Tarazona-Díaz, M. P., Viegas, J., Moldao-Martins, M., and Aguayo, E. (2011). Bioactive compounds from flesh and by-product of fresh-cut watermelon cultivars. J. Sci. Food Agric. 91 (5), 805–812. doi:10.1002/jsfa.4250
Tian, S., Jiang, L., Gao, Q., Zhang, J., Zong, M., Zhang, H., et al. (2017). Efficient CRISPR/Cas9-based gene knockout in watermelon. Plant Cell Rep. 36 (3), 399–406. doi:10.1007/s00299-016-2089-5
Toivonen, P. M. A., and Brummell, D. A. (2008). Biochemical bases of appearance and texture changes in fresh-cut fruit and vegetables. Postharvest Biol. Technol. 48 (1), 1–14. doi:10.1016/j.postharvbio.2007.09.004
Trajkovska Petkoska, A., Daniloski, D., D’Cunha, N. M., Naumovski, N., and Broach, A. T. (2021). Edible packaging: sustainable solutions and novel trends in food packaging. Food Res. Int. 140, 109981. doi:10.1016/j.foodres.2020.109981
Trigo, M., Ferreira, A., Sapata, M., Sousa, M., Curado, T., Andrada, L., et al. (2006). “Improving quality and safety of minimally processed fruits and vegetables by gamma irradiation,” in Use of Irradiation to Ensure the hygienic Quality of fresh, pre-cut Fruits and Vegetables and other minimally processed Food of plant origin. IAEA-TECDOC-1530 (Vienna: IAEA), 229–239.
Ukuku, D. O., and Sapers, G. M. (2007). Effect of time before storage and storage temperature on survival of Salmonella inoculated on fresh-cut melons. Food Microbiol. 24 (3), 288–295. doi:10.1016/j.fm.2006.04.007
Vicente, A. R., Saladié, M., Rose, J. K., and Labavitch, J. M. (2007). The linkage between cell wall metabolism and fruit softening: looking to the future. J. Sci. Food Agric. 87 (8), 1435–1448. doi:10.1002/jsfa.2837
Wang, C., Qiao, A., Fang, X., Sun, L., Gao, P., Davis, A. R., et al. (2019). Fine mapping of lycopene content and flesh color related gene and development of molecular marker–assisted selection for flesh color in watermelon (Citrullus lanatus). Front. Plant Sci. 10, 1240. doi:10.3389/fpls.2019.01240
Wang, H., Chen, J., Yi, Y., Wang, L., Hou, W., Ai, Y., et al. (2023). Regulation and mechanism of ethylene treatment on storage quality of fresh-cut lotus (Nelumbo nucifera Gaertn.) root slices. Sci. Hortic. 313, 111900. doi:10.1016/j.scienta.2023.111900
Wang, Y., Li, W., Cai, W., Ma, Y., Xu, Y., Zhao, X., et al. (2018). Visible light exposure reduces the drip loss of fresh-cut watermelon. J. Food Sci. Technol. 55 (5), 1816–1822. doi:10.1007/s13197-018-3096-z
Wang, Z., Wan, L., Ren, J., Zhang, N., Zeng, H., Wei, J., et al. (2024). Improving the genome editing efficiency of CRISPR/Cas9 in melon and watermelon. Cells 13 (21), 1782. doi:10.3390/cells13211782
Watada, A. E., and Qi, L. (1999). Quality of fresh-cut produce. Postharvest Biol. Technol. 15 (3), 201–205. doi:10.1016/S0925-5214(98)00085-4
Weber, C., Wechsler, S., and Wakefield, H. (2023). U.S. per capita availability of melons, harvested area, production, cash receipts, shipments, price, supply and availability, and trade. Available at: http://www.ers.usda.gov/data-products/chart-gallery/gallery/chart-detail/?chartId=107025 (Accessed September 30, 2024).
Wilson, M. D., Stanley, R. A., Eyles, A., and Ross, T. (2019). Innovative processes and technologies for modified atmosphere packaging of fresh and fresh-cut fruits and vegetables. Crit. Rev. Food Sci. Nutr. 59 (3), 411–422. doi:10.1080/10408398.2017.1375892
Wu, Z., Shi, Z., Yang, X., Xie, C., Xu, J., and Yu, Z. (2022). Comparative metabolomics profiling reveals the molecular information of whole and fresh-cut melon fruit (cv. Xizhoumi-17) during storage. Sci. Hortic. 296, 110914. doi:10.1016/j.scienta.2022.110914
Xiaowei, T., Hongju, H., Ye, L., and Xiaoyan, Z. (2012). Identification of aroma compounds in watermelon juice by SPME-GCMS. Acta Hortic. 944, 183–191. doi:10.17660/actahortic.2012.944.24
Xisto, A. L. R. P., Boas, E. V. D. B. V., Nunes, E. E., Federal, B. M. V. B., and Guerreiro, M. C. (2012). Volatile profile and physical, chemical, and biochemical changes in fresh cut watermelon during storage. Food Sci. Technol. 32 (1), 173–178. doi:10.1590/S0101-20612012005000020
Yang, W., Zhou, J., Yu, R., Du, H., Tian, M., Guo, S., et al. (2024). Assessment of fruit quality and volatile profiles in watermelons grafted onto various rootstocks. Veg. Res. 4 (1), 0. doi:10.48130/vegres-0024-0034
Yao, J., Chen, W., and Fan, K. (2023). Recent advances in light irradiation for improving the preservation of fruits and vegetables: a review. Food Biosci. 56, 103206. doi:10.1016/j.fbio.2023.103206
Yousuf, B., Deshi, V., Ozturk, B., and Siddiqui, M. W. (2020). “Fresh-cut fruits and vegetables: quality issues and safety concerns,” in Fresh-cut fruits and vegetables (Elsevier), 1–15. doi:10.1016/B978-0-12-816184-5.00001-X
Zaaroor-Presman, M., Alkalai-Tuvia, S., Chalupowicz, D., Beniches, M., Gamliel, A., and Fallik, E. (2020). Watermelon rootstock/scion relationships and the effects of fruit-thinning and stem-pruning on yield and postharvest fruit quality. Agriculture 10 (9), 366. doi:10.3390/agriculture10090366
Zhang, J., Sun, H., Guo, S., Ren, Y., Li, M., Wang, J., et al. (2020). Decreased protein abundance of lycopene β-cyclase contributes to red flesh in domesticated watermelon. Plant Physiol. 183 (3), 1171–1183. doi:10.1104/pp.19.01409
Zhao, W., Lv, P., and Gu, H. (2013). Studies on carotenoids in watermelon flesh. Agric. Sci. 4 (7), 13–20. doi:10.4236/as.2013.47A003
Zhong, Z., Wang, X., Yin, X., Tian, J., and Komatsu, S. (2021). Morphophysiological and proteomic responses on plants of irradiation with electromagnetic waves. Int. J. Mol. Sci. 22 (22), 12239. doi:10.3390/ijms222212239
Zhou, B., McEvoy, J. L., Luo, Y., Saftner, R. A., Feng, H., and Beltran, T. (2006). 1-Methylcyclopropene counteracts ethylene-induced microbial growth on fresh-cut watermelon. J. Food Sci. 71 (6), M180–M184. doi:10.1111/j.1750-3841.2006.00081.x
Zhou, Y., He, J., Liu, Y., Liu, H., Wang, T., Liu, Y., et al. (2023). Aerated drip irrigation improves watermelon yield, quality, water and fertilizer use efficiency by changing plant biomass and nutrient partitioning. Irrigation Sci. 41 (6), 739–748. doi:10.1007/s00271-023-00853-y
Keywords: Citrullus lanatus, fresh-cut processing, juice leakage, quality deterioration, cell wall, cell membrane
Citation: Quandoh E and Albornoz K (2025) Fresh-cut watermelon: postharvest physiology, technology, and opportunities for quality improvement. Front. Genet. 16:1523240. doi: 10.3389/fgene.2025.1523240
Received: 05 November 2024; Accepted: 13 January 2025;
Published: 03 February 2025.
Edited by:
Zhongqi Fan, Fujian Agriculture and Forestry University, ChinaReviewed by:
Ruiqing Li, Anhui Agricultural University, ChinaCopyright © 2025 Quandoh and Albornoz. This is an open-access article distributed under the terms of the Creative Commons Attribution License (CC BY). The use, distribution or reproduction in other forums is permitted, provided the original author(s) and the copyright owner(s) are credited and that the original publication in this journal is cited, in accordance with accepted academic practice. No use, distribution or reproduction is permitted which does not comply with these terms.
*Correspondence: Karin Albornoz, a3BhbGJvckBjbGVtc29uLmVkdQ==
Disclaimer: All claims expressed in this article are solely those of the authors and do not necessarily represent those of their affiliated organizations, or those of the publisher, the editors and the reviewers. Any product that may be evaluated in this article or claim that may be made by its manufacturer is not guaranteed or endorsed by the publisher.
Research integrity at Frontiers
Learn more about the work of our research integrity team to safeguard the quality of each article we publish.