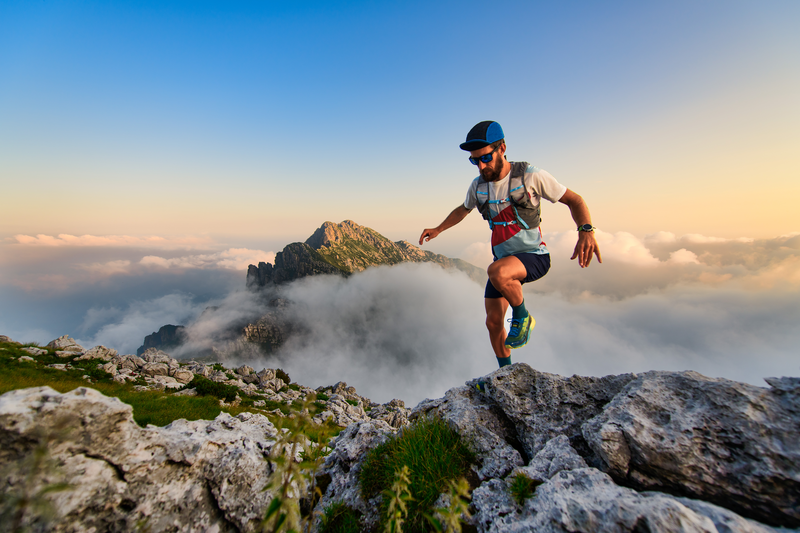
94% of researchers rate our articles as excellent or good
Learn more about the work of our research integrity team to safeguard the quality of each article we publish.
Find out more
ORIGINAL RESEARCH article
Front. Genet.
Sec. Genetics of Common and Rare Diseases
Volume 16 - 2025 | doi: 10.3389/fgene.2025.1518392
The final, formatted version of the article will be published soon.
You have multiple emails registered with Frontiers:
Please enter your email address:
If you already have an account, please login
You don't have a Frontiers account ? You can register here
Objective: To describe the characterization of a novel deletion causing α-thalassemia.Methods:The proband was a 4-year-old male who presented with abnormal hematological parameters identified during routine blood tests conducted for a cold. Three common α-globin gene deletions, three mutations, and 17 mutations in the β-globin gene were detected using PCR-flow fluorescence hybridization. Next-generation sequencing (NGS) and CNVplex technologies were employed to identify potential rare pathogenic mutation types. The CNVplex technology leverages variations in the lengths of linkage sequences of differential sequences at the same locus to produce linkage products of varying lengths, thereby enabling the detection of multiple loci within the same system. The newly identified deletions were further validated using customized third-generation sequencing (TGS) and Sanger sequencing.Conclusions:In this study, hematological analysis indicated a potential diagnosis of thalassemia in the proband, characterized by typical microcytic hypodermic features. A novel 134-kb deletion in the α-globin gene cluster was identified in this proband using CNVplex technology. This deletion encompasses the genes HBZ, HBM, HBA2, HBA1, and HBQ1. Furthermore, we confirmed the gene deletion through customized TGS testing and Sanger sequencing, allowing us to determine the size of the deletion. The results suggest that this represents a new deletion of 146 kb that has not been previously reported, and we hypothesize that this deletion is likely the primary cause of the α-thalassemia trait observed in the proband.
Keywords: Thalassemia, α-Globin gene, CNVplex, single-molecule real-time sequencing, deletion
Received: 28 Oct 2024; Accepted: 20 Feb 2025.
Copyright: © 2025 Xu, Hu, Wen, Cao, Xu, Luo, Long, Yu, Sun and Wei. This is an open-access article distributed under the terms of the Creative Commons Attribution License (CC BY). The use, distribution or reproduction in other forums is permitted, provided the original author(s) or licensor are credited and that the original publication in this journal is cited, in accordance with accepted academic practice. No use, distribution or reproduction is permitted which does not comply with these terms.
* Correspondence:
Fengxiang Wei, Shenzhen Longgang District Maternal and Child Health Care Hospital, Shenzhen, China
Disclaimer: All claims expressed in this article are solely those of the authors and do not necessarily represent those of their affiliated organizations, or those of the publisher, the editors and the reviewers. Any product that may be evaluated in this article or claim that may be made by its manufacturer is not guaranteed or endorsed by the publisher.
Research integrity at Frontiers
Learn more about the work of our research integrity team to safeguard the quality of each article we publish.