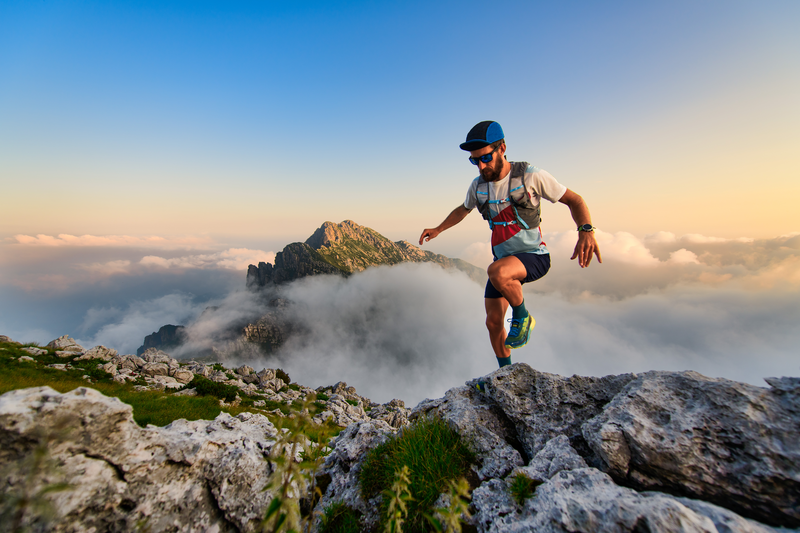
95% of researchers rate our articles as excellent or good
Learn more about the work of our research integrity team to safeguard the quality of each article we publish.
Find out more
ORIGINAL RESEARCH article
Front. Genet.
Sec. Genomics of Plants and the Phytoecosystem
Volume 16 - 2025 | doi: 10.3389/fgene.2025.1496262
This article is part of the Research Topic Plant Plasticity and Epigenetics View all 7 articles
The final, formatted version of the article will be published soon.
You have multiple emails registered with Frontiers:
Please enter your email address:
If you already have an account, please login
You don't have a Frontiers account ? You can register here
Many species in the Araceae have extremely high medicinal value, while the chloroplast genome is relatively conserved, and the encoded and expressed bioactive substances are also abundant. Therefore, chloroplast genomes can serve as one of the basis for species evolution and are extremely important for individual material accumulation.To study the relationship between the chloroplast genome and morphology of target species, this study selected three Araceae species for chloroplast genome sequencing assembly, downloaded the complete chloroplast genomes sequences of another 11 Araceae species. Grouping based on genetic distance, we analyze the association between chloroplast genome structure and morphology. The results showed that there were significant differences in genome size among the three species, but Relative Synonymous Codon Usage (RSCU) exhibited high similarity; Based on the phylogenetic tree, these 14 species can be divided into three branches, with differences in genes such as rrn4, rrn5, rrn23, and trnN among species within each branch; Morphologically, the length of the male inflorescence in BranchⅢ is significantly greater than that in BranchⅡ; There is a strong positive correlation between the length of the plant stem and the three parameters (Length of LSC, Length of SSC and Length of chloroplast genome) of the genome. This study conducted correlation research from the perspective of chloroplast genome and species morphology. On the one hand, the genetic distance and chloroplast genome structure differences between the target species were determined, and on the other hand, explored the correlation between chloroplast genome and species morphology, providing a theoretical basis for the study of phylogenetic relationships and morphology of Araceae species.
Keywords: Araceae, Genetic distance, Chloroplast genomes, morphology, Species morphology
Received: 14 Sep 2024; Accepted: 25 Mar 2025.
Copyright: © 2025 Wen Gang, Jingru, Siqin, Ying, Lulu, Yingxian, Ke and Lili. This is an open-access article distributed under the terms of the Creative Commons Attribution License (CC BY). The use, distribution or reproduction in other forums is permitted, provided the original author(s) or licensor are credited and that the original publication in this journal is cited, in accordance with accepted academic practice. No use, distribution or reproduction is permitted which does not comply with these terms.
* Correspondence:
Luo Lili, Department of Cardiology, Anhui Province Hospital of Traditional Chinese Medicine, Hefei, Anhui Province, China
Disclaimer: All claims expressed in this article are solely those of the authors and do not necessarily represent those of their affiliated organizations, or those of the publisher, the editors and the reviewers. Any product that may be evaluated in this article or claim that may be made by its manufacturer is not guaranteed or endorsed by the publisher.
Research integrity at Frontiers
Learn more about the work of our research integrity team to safeguard the quality of each article we publish.