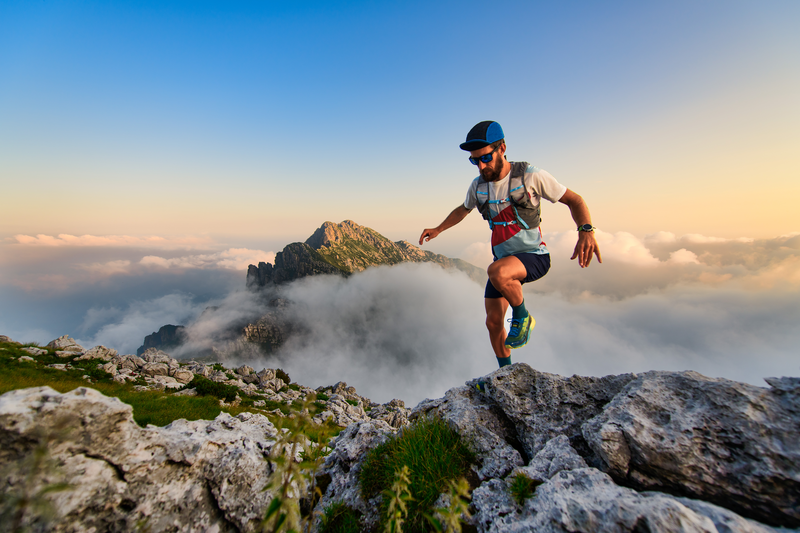
94% of researchers rate our articles as excellent or good
Learn more about the work of our research integrity team to safeguard the quality of each article we publish.
Find out more
ORIGINAL RESEARCH article
Front. Genet.
Sec. RNA
Volume 16 - 2025 | doi: 10.3389/fgene.2025.1474223
This article is part of the Research Topic Recent Advancements in RNA-based and targeted Therapeutics View all 5 articles
The final, formatted version of the article will be published soon.
You have multiple emails registered with Frontiers:
Please enter your email address:
If you already have an account, please login
You don't have a Frontiers account ? You can register here
Background: MicroRNAs (miRNAs) represent a series of non-coding small RNAs and are involved in the processes of many diseases. However, the role of microRNA in obstructive sleep apnea (OSA)-induced white adipose tissue (WAT) dysfunction remains to be elaborated. Taking advantage of miRNA sequencing (miRNA-seq), we uncovered the miRNA expression profiles in chronic intermittent hypoxia (CIH)-induced WAT dysfunction mice.We established apolipoprotein deficient (ApoE-/-) mice CIH model and traced differential expressed miRNAs (DEmiRs) through miRNA-seq technology. With the help of Gene Ontology (GO) functional enrichment and the Kyoto Encyclopedia of Genes and Genomes (KEGG) pathway analyses, we revealed the biological functions of these DEmiRs. In addition, RT-qPCR was used for further evaluation of the sequencing data. Ultimately, we designed a CNC network to expound the relationship between miRNA and target genes.In general, 13 miRNAs manifested as upregulated while 18 miRNAs as downregulated in a CIH mouse model of WAT dysfunction. KEGG results indicated that the Lysosome pathway participated in CIH-induced WAT dysfunction. Then, we selected eight miRNAs for RT-qPCR validation. Based on the data, we chose these DEmiRs to construct a miRNA-mRNA network.To sum up, we discovered 31 DEmiRs in the ApoE-/-mice model of CIH. Our finding may play a major role in explaining the pathophysiology mechanisms of WAT dysfunction induced by obstructive sleep apnea.
Keywords: microRNA, chronic intermittent hypoxia, white adipose tissue dysfunction, miRNA sequencing, Bioinformatics analysis
Received: 01 Aug 2024; Accepted: 26 Feb 2025.
Copyright: © 2025 Zhang, Chen, Guo, Ji, Lin and Liu. This is an open-access article distributed under the terms of the Creative Commons Attribution License (CC BY). The use, distribution or reproduction in other forums is permitted, provided the original author(s) or licensor are credited and that the original publication in this journal is cited, in accordance with accepted academic practice. No use, distribution or reproduction is permitted which does not comply with these terms.
* Correspondence:
Qingshi Chen, The Second Affiliated Hospital of Fujian Medical University, Quanzhou, China
Dexin Liu, The Second Affiliated Hospital of Fujian Medical University, Quanzhou, China
Disclaimer: All claims expressed in this article are solely those of the authors and do not necessarily represent those of their affiliated organizations, or those of the publisher, the editors and the reviewers. Any product that may be evaluated in this article or claim that may be made by its manufacturer is not guaranteed or endorsed by the publisher.
Research integrity at Frontiers
Learn more about the work of our research integrity team to safeguard the quality of each article we publish.