- 1Department of Oncology and Hematology, Beilun District People’s Hospital, Ningbo, China
- 2Department of Biochemistry and Molecular Biology, Zhejiang Key Laboratory of Pathphysiology, Medical School of Ningbo University, Ningbo, China
TRIM28 (tripartite motif protein 28) was initially believed to be a transcription inhibitor that plays an important role in DNA damage repair (DDR) and in maintaining cancer cellular stemness. As research has continued to deepen, several studies have found that TRIM28 not only has ubiquitin E3 ligase activity to promote degradation of substrates, but also can promote SUMOylation of substrates. Although TRIM28 is highly expressed in various cancer tissues and has oncogenic effects, there are still a few studies indicating that TRIM28 has certain anticancer effects. Additionally, TRIM28 is subject to complex upstream regulation. In this review, we have elaborated on the structure and regulation of TRIM28. At the same time, highlighting the functional role of TRIM28 in tumor development and emphasizing its impact on cancer treatment provides a new direction for future clinical antitumor treatment.
1 Introduction
PTMs (Post-translational modifications) play a crucial role in controlling the activity and stability of proteins in response to intracellular and extracellular stimuli (Venne et al., 2014). These modifications involve the cleavage of precursor proteins or covalent addition of modified groups (Venne et al., 2014; Ramazi and Zahiri, 2021). Ubiquitination is a prominent PTM that transfers ubiquitin to substrates through multiple enzymatic reactions, thereby promoting protein degradation and maintaining intracellular protein homeostasis (Sheng et al., 2021). Mechanistically, ubiquitin is activated by E1, coupled with E2, and then transferred to substrates with the help of E3 ligase (Sheng et al., 2021). Substrates labeled with ubiquitin are typically degraded by proteasomes (Sheng et al., 2021). Ubiquitin E3 ligase plays a crucial role in recognizing substrates and maintaining the ubiquitin processes. Dysfunction of ubiquitin E3 ligase can lead to abnormal accumulation or excessive degradation of substrates, which is closely related to the onset and progression of cancer (Sheng et al., 2021). SUMOylation is another important PTM, which is a small ubiquitin-like modification (Zhang and Zeng, 2020; Sheng et al., 2021). Similar to ubiquitination, SUMOylation requires E1 activation, E2 coupling, and E3 ligation to attach SUMO molecules to substrates (Sheng et al., 2021). Although SUMO E3 ligase is not necessary for SUMOylation, the dysregulation of SUMOylation E3 ligases also greatly affects the SUMOylation of substrates, promoting the occurrence of cancer (Li et al., 2023).
TRIM28, also known as KAP1 (Krüppel-Associated Box (KRAB)-Associated Protein 1) and transcription intermediary factor-β (TIF1β), is a RING (really interesting new gene)-type ubiquitin E3 ligase (Czerwińska et al., 2017a). Notably, TRIM28 catalyzes the poly-ubiquitinylation and degradation of various substrates, such as AMPK (AMP-activated protein kinase) AMPK) and RING finger LIM-domain-interacting protein (RLIM), also known as RNF12), by cooperating with the melanoma-associated antigen-encoding gene (MAGE), which plays an important role in the occurrence and development of tumors (Doyle et al., 2010; Pineda and Potts, 2015; Jin et al., 2021). Moreover, under certain conditions, TRIM28 also promotes SUMOylation of target proteins such as PD-L1 (programmed cell death ligand 1) (Ma et al., 2023). In addition, TRIM28 can serve as a skeletal protein that is recruited to chromosomes by Krüppel-related cassette zinc finger protein (KRAB-ZFP) and exerts a certain transcriptional inhibitory effect by binding to various chromatin remodeling factors (Sun et al., 2019). Emerging evidence suggests that inhibiting TRIM28 may enhance the efficacy of antitumor treatments (Tan et al., 2022; Han et al., 2023; Ma et al., 2023). In this review, we have elaborated on the structure and regulation of TRIM28. At the same time, highlighting the critical role of TRIM28 in cancer development and treatment provides new directions for future clinical antitumor therapies.
2 Structure feature and regulation of TRIM28
The human TRIM28 gene is located on chromosome 19 and contains 6,652 bases (International Human Genome Sequencing Consortium, 2004). TRIM28 is a nuclear protein with 835 amino acids that is composed of multiple domains (Figure 1A) (Peng et al., 2000). TRIM28 belongs to the TRIM family of proteins (Hatakeyama, 2017). Like other TRIM family proteins, the N-terminus of TRIM28 has a TRIM domain (also known as the RBCC domain) composed of an RNG domain, B-boxes (B1 and B2), and leucine zipper curled helical region (CC) (Peng et al., 2000; Hatakeyama, 2017). The B-box domain may be an important structure for the formation of TRIM28 polymers (Sun et al., 2019). In addition, the interaction between the RBCC region and the KRAB-ZFP transcription factor exerts the transcriptional inhibitory function of TRIM28 (Peng et al., 2007). Moreover, the RBCC region can bind to MAGE, thereby exerting the ubiquitination activity of TRIM28 (Xiao et al., 2014). Meanwhile, the MAGE-TRIM28 complex can ubiquitinate and degrade KRAB-ZFP family proteins, thus promoting downstream target gene transcription. However, since both MAGE and KRAB-ZFP bind to the RBCC domain of TRIM28 protein, KRAB-ZFP may competitively bind to TRIM28 protein, thereby inhibiting the ubiquitination activity of TRIM28 and subsequently inhibiting downstream gene transcription (Xiao et al., 2014).
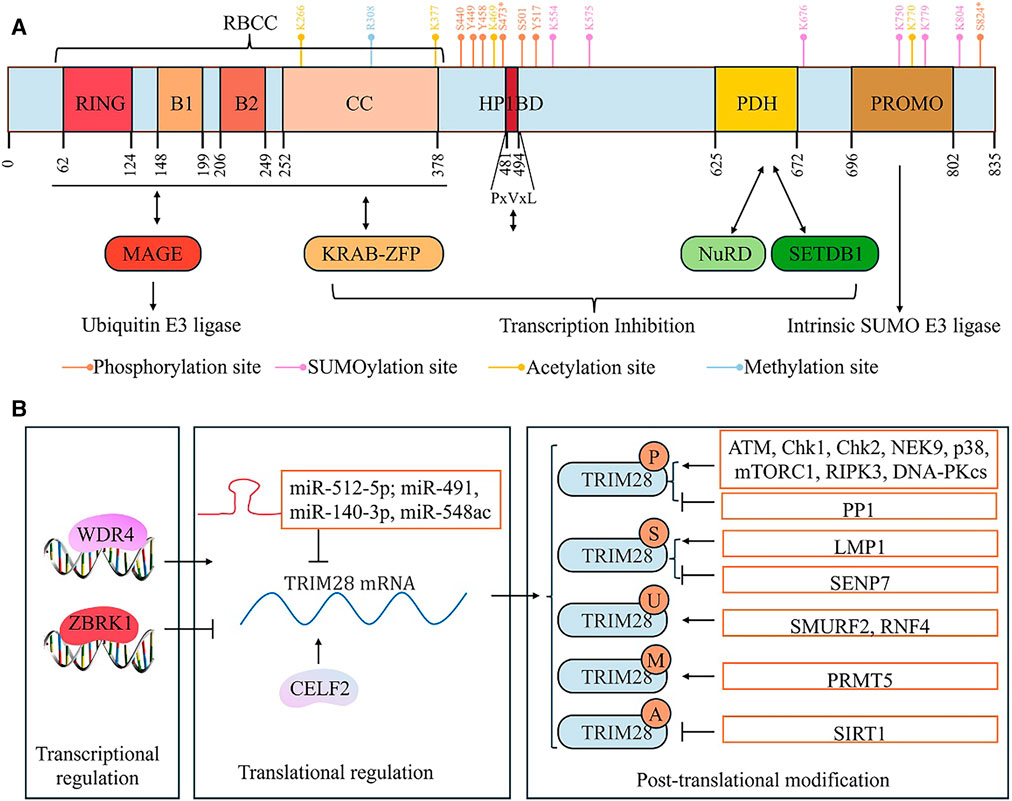
Figure 1. The structure and regulation of TRIM28. (A) The structure of TRIM28: TRIM28 consists of an N-terminal RING domain, two B-boxes (B1 and B2), a leucine zipper coiled-coil region (CC), an intermediate PxVxL pentapeptide domain, and a C-terminal plant homeodomain (PHD) and bromodomain. The RBCC domain, composed of the RING domain, B1 and B2 boxes, and CC domain, not only binds to the MAGE protein and functions as a ubiquitinated E3 ligase but also binds to the KRAB domain of the KRAB-ZNP protein. The PHD domain recruits NuRD and SETDB1 and the central PxVxL pentapeptide region binds to HP1. The transcriptional inhibitory activity of TRIM28 is closely related to its binding to SETDB1, NuRD, and HP1 proteins, but it is also influenced by SUMOylation modification of TRIM28 itself. The PHD domain has intrinsic E3 SUMO ligase activity. (B) The regulation of TRIM28.
The C-termini of TRIM family proteins have different structural domains, which is the basis for their protein specificity and classification of TRIM subfamilies (Hatakeyama, 2017). In the TRIM28 protein, the domains located at its C-terminus are the plant homeodomain (PHD) and bromodomain (Peng et al., 2000). Furthermore, the core of TRIM28 consists of a PxVxL pentapeptide domain (Peng et al., 2000). The PHD domain is a region rich in cysteine/histidine, which can recruit Nucleosome Remodeling Deacetylase (NuRD) and SET domain forked histone lysine methyltransferase 1 (SETDB1), thereby exerting chromosomal cohesion. The central PxVxL region binds to HP1 (Heterochromatin protein 1) (Sripathy et al., 2006). Currently, it is believed that PHD, bromodomain, and PxVxL regions synergistically form concentrated heterochromatin, leading to transcriptional inhibition (Sripathy et al., 2006; Xiao et al., 2014).
TRIM28 is regulated at several levels, including gene transcription, post-transcriptional translation, and PTM (Table 1) (Figure 1B). For example, WDR4 (WD repeat domain 4) can induce transcription of the TRIM28 gene (Han et al., 2023). ZBRK1/ZNF350 (zinc finger and BRCA1-interacting protein with KRAB domain-1, also known as zinc finger protein 350) represses TRIM28 transcription by suppressing its promoter activity (Lin et al., 2013). MicroRNAs (miRNAs) inhibit the expression of TRIM28 by binding to TRIM28 mRNA. miR-512-5p, miR-491, miR-140-3p, and miR-548ac have been found to reduce the levels of endogenous TRIM28 in gastric cancer (GC), glioblastoma, breast cancer (BC), and acute myeloid leukemia (AML) cells (Qi Z. et al., 2016; Zhou et al., 2019; Zou et al., 2021; Zhao et al., 2022). The RNA-binding protein CELF2 binds to TRIM28 mRNA, thereby promoting TRIM28 protein expression (Turchi et al., 2023).
The PTMs of TRIM28 are particularly important for their molecular functions, especially phosphorylation. Currently, research has found that TRIM28 is phosphorylated at multiple serine and threonine sites, such as S440, S473, S501, S824, Y44, Y458, and Y517, inhibiting its gene transcriptional inhibitory activity (Czerwińska et al., 2017a). Phosphorylation of S473 and S824 (pS473 and pS824) is particularly important. DNA damage activates Ataxia-Telangiectasia Mutated (ATM) kinase, which promotes DDR by phosphorylating several specific substrates to trigger damage response pathways (Li and Cucinotta, 2020). ATM promotes the phosphorylation of TRIM28 at S824 and S473, disrupting the interaction between TRIM28 and chromatin remodeling factors, thereby promoting DDR (Ziv et al., 2006; Goodarzi et al., 2011). Multiple studies have shown that pS824-TRIM28 causes chromosome relaxation, thereby promoting DDR and downstream gene transcription (Ziv et al., 2006). Interestingly, pS824-TRIM28 promotes cancer cell growth by regulating chromatin relaxation (Bhatia et al., 2013). In melanoma cancer cells, the MAGE protein upregulates ATM-dependent pS824-TRIM28 by promoting the binding of TRIM28 to ATM, thereby enhancing DDR and promoting tumor progression (Bhatia et al., 2013). pS473-TRIM28 is also involved in effective DDR and cell survival (Chang et al., 2008; Blasius et al., 2011; Magni et al., 2015). Depending on the type of DNA damage that occurs, TRIM28 is mediated by different phosphorylation kinases at the S473 site. In etoposide- or IR-induced stress responses, Chk2 (Checkpoint kinase 2) is the main kinase responsible for TRIM28 phosphorylation at the S473 site, while Chk1 (Checkpoint kinase 1) is an essential kinase responsible for TRIM28 phosphorylation at the S473 site induced by UV radiation (Blasius et al., 2011; Magni et al., 2015). Moreover, pS473-TRIM28 induced by DNA damage also promotes the interaction between TRIM28 and E2F1 (E2 promoter binding factor 1), thereby inhibiting the ability of E2F1 to activate apoptosis (Hu et al., 2012). Additionally, many other kinases have been shown to phosphorylate TRIM28. These include serine/threonine kinases p38MAPK, RIPK3 (receptor interacting serine/threonine kinase 3), NEK9 (NIMA related kinase 9), mTORC1 (mechanistic target of rapamycin complex 1) and DNA-dependent protein kinase catalytic subunits) (Shen et al., 2017; Agarwal et al., 2021; Park et al., 2021; Yang et al., 2022; Lu et al., 2023). Furthermore, PP2A dephosphorylates TRIM28 at S824 in prostate cancer (PCa) cells (Mita et al., 2016).
Moreover, the transcriptional inhibition activity of TRIM28 also depends on SUMOylation of at least three lysine residues (K554, K779, and K804). Interestingly, PHD and bromodomain serve as SUMO E3 ligases within the TRIM28 molecule, where PHD binds to UBC9 (SUMO E2 enzyme) and synergistically promotes the SUMOylation of TRIM28 (Ivanov et al., 2007). LMP1, the main viral oncoprotein of the Epstein–Barr virus (EBV), promotes SUMOylation of TRIM28 by binding to TRIM28 protein, thereby inhibiting EBV gene transcription and helping to maintain stable EBV latency (Bentz et al., 2015). Garvin et al. found that SENP7 (SUMO protease 7) promotes the deSUMOylation of TRIM28, thereby promoting chromosome relaxation under DDR (Garvin et al., 2013).
In addition, Lin et al. discovered four potential acetylation sites of TRIM28 through mass spectrometry analysis: K266, K377, K469, and K770 (Lin et al., 2015). During DDR, SIRT1 (Sirtuin 1) promotes the deacetylation of TRIM28, thereby stabilizing its interaction with 53BP1, leading to an increase in the formation of 53BP1 focal points responsive to DNA damage and promoting non-homologous end joining (NHEJ)-mediated DNA repair (Lin et al., 2015). Furthermore, PRMT5 (Protein arginine methyltransferase 5) promotes the methylation modification of TRIM28 at the R308 site, thereby preventing the interaction between TRIM28 and the KRAB-ZFP protein, ZNF224 (di Caprio et al., 2015).
SMURF2 (SMAD-specific E3 ubiquitin ligase 2) directly binds to TRIM28 to promote its ubiquitination (Shah et al., 2022). Interestingly, in normal cells, SMURF2 has a negative impact on TRIM28 expression, whereas SMURF2 stabilizes TRIM28 in tumor cells (Shah et al., 2022). Current research indicates that the relationship between ubiquitination and SUMOylation is highly complex and interactive (Li et al., 2023). RNF4 (Ring finger protein 4), a SUMO-targeted ubiquitin ligase, stabilizes the abundance of TRIM28 in cells by promoting the ubiquitination degradation of SUMOylated TRIM28 (Kuo et al., 2014).
3 Roles of TRIM28 in cancers
To investigate the role of TRIM28 in cancer, we utilized a web platform (http://solvinglab.com.cn/) to study TCGA (The Cancer Genome Atlas) data and found that TRIM28 mRNA levels were highly expressed in multiple cancers (Figure 2A). We found significant mutations in TRIM28 in multiple tumor tissues, especially in endometrial cancer (EC), with a mutation probability of 6.25% (Table 2). Moreover, we used the cBioPortal platform (https://www.cbioportal.org/) to statistically analyze all point mutation of TRIM28 in cancer (Table 2) (Figure 2B). In addition, TRIM28 has different mutations in various types of cancers, mainly missense mutations. Therefore, we believe that it is necessary to study cancer-related mutations of TRIM28, which can further clarify its clinical significance.
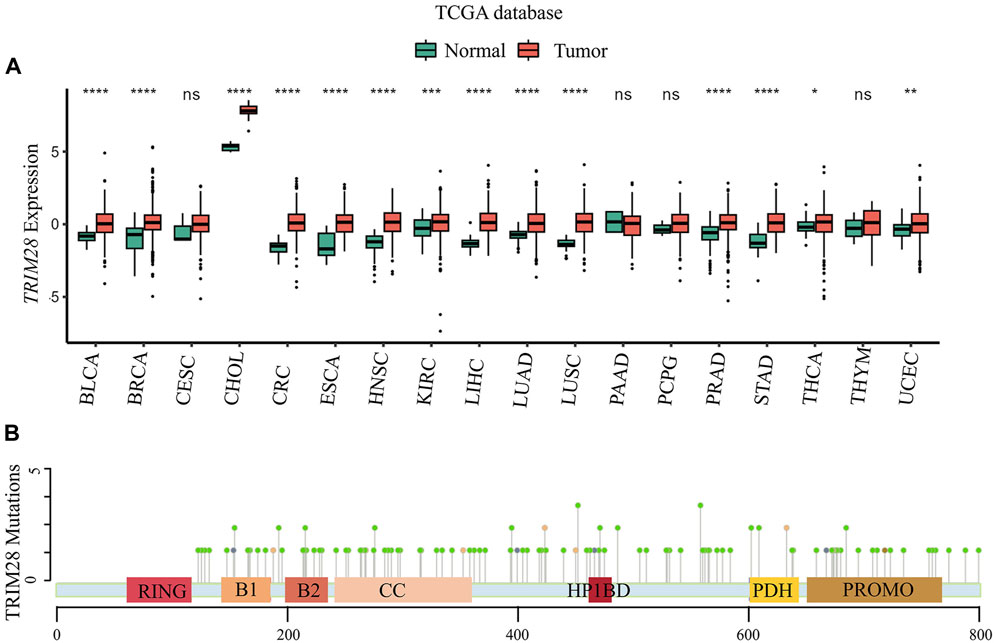
Figure 2. The expression and mutation of the TRIM28 in human cancer (A) The expression of the TRIM28 in human cancer: The TRIM28 gene expression dataset from The Cancer Genome Atlas (TCGA) database was extracted to explore its differential expression between tumors and adjacent normal tissues (data from the TIMER2.0 database http://timer.cistrome. org). Boxplots represent the distribution of gene expression levels and the statistical significance of differential expression was assessed using the Wilcoxon test. An asterisk indicates a p-value less than 0.05, two asterisks indicate a p-value less than 0.01, and three asterisks indicate a p-value less than 0.001. As illustrated in the figure, TRIM28 was significantly overexpressed in BLCA, BRCA, CHOL, CRC, ESCA, HNSC, KIRC, LIHC, LUAD, LUSC, PRAD, STAD, THCA, and UCEC. THCA, thyroid carcinoma; LUSC: Lung squamous cell carcinoma; BLCA: Bladder urothelial carcinoma; LIHC: Liver hepatocellular carcinoma; PRAD: Prostate adenocarcinoma; KIRC: Kidney renal clear cell carcinoma; UCEC: Uterine corpus endometrial carcinoma; LUAD: Lung adenocarcinoma; STAD: Stomach adenocarcinoma; BRCA: Breast invasive carcinoma; CESC: Cervical squamous cell carcinoma and endocervical adenocarcinoma; CHOL: Cholangiocarcinoma; ESCA: Esophageal carcinoma; HNSC: Head and Neck squamous cell carcinoma; PAAD: Pancreatic adenocarcinoma; PCPG: Pheochromocytoma and Paraganglioma; THYM: Thymoma; CRC: Colorectal Cancer. (B) The point mutation of TRIM28 in cancer: Point mutation data of TRIM28 protein displayed on the cBioPortal platform (https://www.cbioportal.org/) in all cancers.
In addition, TRIM28 protein, a ubiquitin E3 ligase, SUMO E3 ligase, or skeletal protein also plays a key role in the development of tumors and tumorigenesis (Table 3) (Figure 3). The following are examples of different subtypes of cancer in which TRIM28 is considered to play a key role.
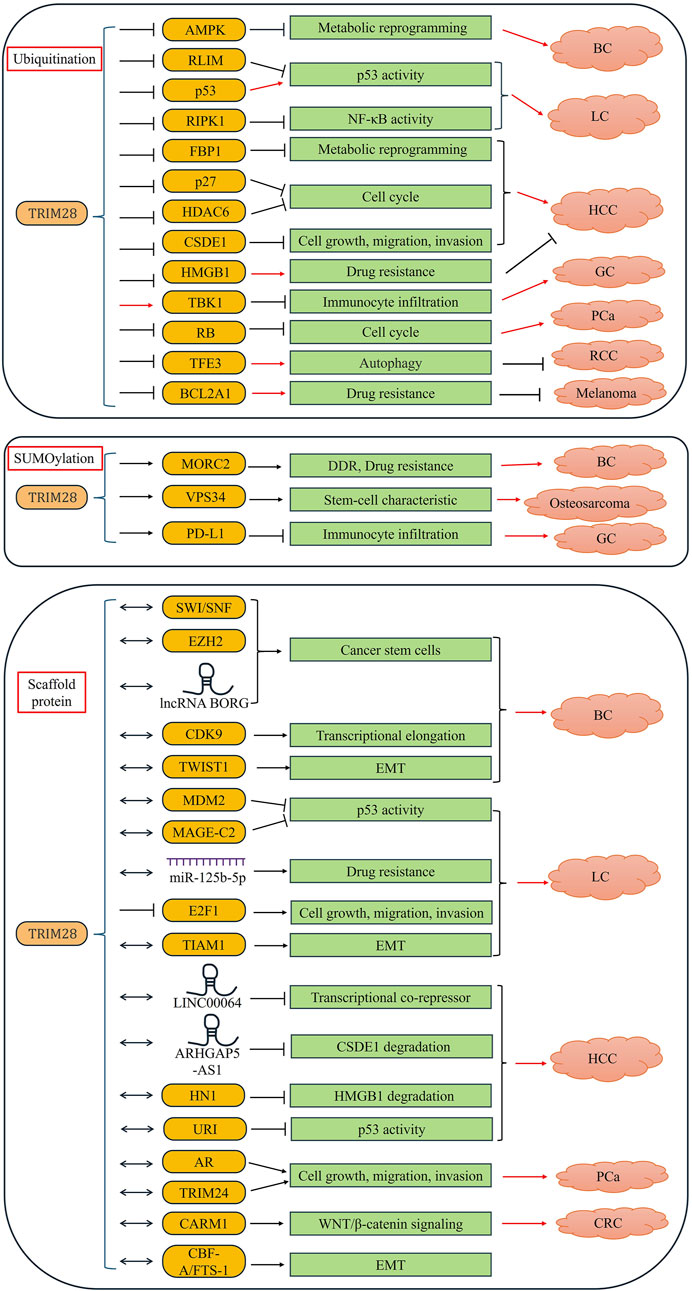
Figure 3. The role of TRIM28 in cancer TRIM28 protein, a ubiquitin E3 ligase, SUMO E3 ligase, or skeletal protein, plays an important role in the occurrence and development of tumors (including breast cancer, lung cancer, stomach cancer, liver cancer, colorectal cancer, prostate cancer, renal clear cell carcinoma, osteosarcoma melanoma, and neuroblastoma).
3.1 The carcinogenic role of TRIM28
3.1.1 TRIM28 in BC
BC is the most common malignancy in women, which seriously damages their health (Sung et al., 2021). Although various antitumor treatments have, to some extent, slowed down the progression of BC, certain subtypes of BC are not able to achieve good therapeutic effects due to the high heterogeneity of pathological features (Sung et al., 2021). The expression of TRIM28 at mRNA and protein level have been found to be significantly higher in BC tissues (Hao et al., 2017; Zhang W. et al., 2021). Furthermore, immunohistochemistry (IHC) staining confirmed higher expression of TRIM28 and pS824-TRIM28 in highly invasive cancer subtypes (HER2, Basal-like) (Addison et al., 2015). In addition, TRIM28 depletion reduced BC formation in mouse tumor models (Czerwińska et al., 2017b).
The tumor suppressor AMPK is a master sensor and regulator of the cellular energy status (Chaube and Bhat, 2016). Pineda et al. demonstrated that TRIM28 forms a cancer-specific ubiquitinase with MAGE protein, which ubiquitinates and degrades AMPK (Pineda and Potts, 2015). CzerwinSka et al. found a significant increase in AMPK protein levels in BC allografts with TRIM28 deletion (Czerwińska et al., 2017b).
DNA damage is caused by a variety of factors, including chemical carcinogens, radiation, and the subsequent initiation of DDR (Jackson and Bartek, 2009). MORC2 (Microrchidia CW-type zinc finger 2) plays a central role in DDR (Li et al., 2012). Notably, under the action of the DNA-damaging agent Dox (doxorubicin), TRIM28 promotes SUMOylation of MORC2 at the K827 site, thereby recruiting CSK21 (casein kinase II subunit alpha) and inducing the activation of DNA-PKcs, promoting DDR (Zhang FL. et al., 2023). At the same time, Zhang et al. used a xenograft transistor model to discover that the SUMOylation-deficient mutant MORC2 enhances the sensitivity of BC cells to DNA-damaging chemotherapeutic drugs (Zhang FL. et al., 2023). Interestingly, Lee et al. found that DOX was able to inhibit the SUMOylation of TRIM28 itself, thereby promoting the transcription of p21 and, thus, the growth of the BC cell line MCF-7 (Lee et al., 2007).
Cancer stem cells (CSCs) are present in various types of cancer. Studies have shown that tumor growth is driven by a small number of CSC (Batlle and Clevers, 2017). TRIM28 plays an important role in BC stem cells (BCSCs) (Czerwińska et al., 2017b). Downregulation of TRIM28 expression reduces BCSCs’ ability of BCSCs to self-renew and inhibits tumor growth (Czerwińska et al., 2017b). In MCF-7 cells, TRIM28 interacts with EZH2 (Enhancer of Zeste Homolog 2) and SWI/SNF (ATP-dependent chromatin remodeling complex, also called BAF) to promote BCSC enrichment and maintenance (Li et al., 2017). TRIM28 binds to lncRNA BORG (long non-coding RNA BMP/OP-Responsive Gene) to form the lncRNA BORG/TRIM28 complex, which induces self-renewal and expansion of BCSCs (Parker et al., 2021). Hypoxia is a prominent feature of the tumor microenvironment and a hallmark of many solid tumors (Harris, 2002). HIF-1α binds to the hypoxia-responsive elements (HREs) of the target gene, recruiting Pol II (RNA polymerase II) to stimulate transcription of the target gene under hypoxia (Galbraith et al., 2013; Semenza, 2014). Although Pol II initiates transcription, it pauses approximately 30–60 nucleotides downstream of the transcription start site (Bunch et al., 2014). In BC cells, DNA-PKcs are recruited into the HRE by HIF-1α and promote the phosphorylation of TRIM28 at the S824 site. Phosphorylated TRIM28 recruits CDK9 (Yang et al., 2022). CDK9 deactivates Pol II by phosphorylation, thereby stimulating efficient transcriptional elongation (Yang et al., 2022). Deregulation of cellular energy, a characteristic feature of cancer, is primarily caused by mitochondrial dysfunction (Gomes et al., 2011). TRIM28 is phosphorylated by ROS-p38MAPK at the S473 site in BC cells that have been starved for a long time (Cheng et al., 2016). Moreover, pS473-TRIM28 downregulates MFN2 (Mitofusin-2), thus reducing the excessive fusion of mitochondria, enabling BC cells to survive in the changes in tumor microenvironment (TME), thus promoting tumor growth (Cheng et al., 2016). TWIST1 (Twist related protein 1) is a tumor protein that plays an important role in tumor metastasis and drug resistance (Yang et al., 2004). Mechanistically, TWIST1 enhances metastasis by promoting epithelial-to-mesenchymal transition (EMT) (Kang and Massagué, 2004). TRIM28 can stabilize TWIST1 by combining with it and promoting BC metastasis (Wei et al., 2016). Moreover, TRIM28 upregulates intracellular TWIST1 protein levels, but does not affect TWIST1 mRNA levels (Wei et al., 2016). In conclusion, these findings support a key role for TRIM28 in regulating BC tumorigenesis and progression.
3.1.2 TRIM28 in GC
GC is one of the deadliest malignant tumors, with a 5-year survival rate of approximately 20% (Sung et al., 2021). Upregulation of TRIM28 protein and mRNA levels has been detected in GC tissues compared to normal tissues (Yokoe et al., 2010). Simultaneous survival analysis showed that patients with high TRIM28 expression had poorer prognosis (Yokoe et al., 2010).
EBV is a pathogenic virus found in many malignant tumors (Farrell, 2019). EBV has been detected in 10% of GC tissues (Burke et al., 1990). SNHG8, a member of the small nucleolar RNA host gene (SNHG) family, is an oncogene in GC (Zhang et al., 2020). SNHG8 is significantly overexpressed in EBV-associated GC (EBVaGC) tissue and promotes the proliferation and migration of EBVaGC cells (Zou et al., 2021). Further research has found that TRIM28 is crucial for SNHG8 mediated malignant behavior (Zou et al., 2021). Mechanistically, SNHG8 sponges have miR-512-5p and upregulate TRIM28, thereby exerting a cancer-promoting effect (Zou et al., 2021). Cancer associated fibroblasts (CAFs) directly or indirectly affect cancer progression and tumor immunity by secreting various proteins (Chen et al., 2021). SLIT2 (Slit guidance ligand 2) is an axon-guiding protein secreted by CAFs, which promotes the kinase activity of NEK9 in GC cells by binding to the surface receptor ROBO1 (Roundabout guidance receptor 1) of GC cells (Lu et al., 2023). As mentioned earlier, TRIM28 is phosphorylated by NEK9 (Lu et al., 2023). Phosphorylation downregulates the transcriptional inhibition function of TRIM28 and promotes gene transcription, thus enabling GC cells to obtain excessive migration ability (Lu et al., 2023).
Targeted immune checkpoints have recently been used in GC therapy (Xie et al., 2021). Recent studies have shown that the response to PD-1/PD-L1 treatment may be related to the expression level of PD-L1 in tumor cells (Zhang et al., 2018; Shen et al., 2022). TRIM28 is an important regulatory factor for PD-L1 expression in GC cells (Ma et al., 2023). Mechanistically, TRIM28 inhibits the ubiquitination and degradation of PD-L1 by promoting PD-L1 SUMOylation, thereby stabilizing PD-L1 within cells. In addition, TRIM28 promotes non-degradable polyubiquitination of TBK1 (TANK-binding kinase 1), activating the TBK1-mTOR pathway, thereby enhancing PD-L1 transcription (Ma et al., 2023). Simultaneously, ectopic TRIM28 expression promotes tumor growth in mice and inhibits T-cell activation (Ma et al., 2023). Interestingly, TRIM28 promotes drug resistance in GC cells by influencing Wnt/β-catenin signaling, indicating that TRIM28 is a promising drug target and potential prognostic factor (Ning et al., 2023).
3.1.3 TRIM28 in PCa
PCa is the second most common cancer in men and the eighth leading cause of cancer death (Rawla, 2019). Androgen deprivation therapy (ADT) has shown promising results in the treatment of PCa (Dai et al., 2017). However, most patients eventually relapse because of castration-resistant PCa (CRPC) (Dai et al., 2017). TRIM28 is significantly upregulated in CRPC, and TRIM28 knockdown inhibits the growth of PCa xenografts (Fong et al., 2018). Further mechanistic studies have shown that TRIM28 enhances AR signaling in PCa cells and that TRIM28 inhibits the degradation of TRIM24 by SPOP, further promoting the transcriptional activity of AR (Van Tilborgh et al., 2013; Fong et al., 2018). Yende et al. showed that TRIM28 promotes the expression of proximal luminal lineage cell markers in PCa cells (Yende et al., 2023). RB (Retinoblastoma) is an important tumor suppressor protein that inhibits cell cycle (Engeland, 2022). RB phosphorylation plays a crucial role in mediating the inhibitory effect of RB (Engeland, 2022). Huang et al. found that TRIM28 can bind and promote polyubiquitination and degradation of phosphorylated RB by CDK4/6, thereby promoting the progression of PCa (Huang et al., 2023). Overall, these studies indicate that TRIM28 may be a potential tumor promoter in PCa.
3.1.4 TRIM28 in CC (cervical cancer) and OC (ovarian cancer)
At present, the incidence of CC and OC is still rising worldwide, posing a serious threat to women’s health (Lajer et al., 2010). However, research on TRIM28 expression in CC and OC remains limited. Studies have shown that TRIM28 is highly expressed in CC and OC tissues (Cui et al., 2014; Li et al., 2018). TRIM28 promotes CC cell proliferation by activating the mTOR signaling pathway (Li et al., 2018). In OC, TRIM28 recruits E2F1 to activate the PI3K/AKT/mTOR signaling pathway and promote OC development (Zhang F. et al., 2023). Deng et al. found that knocking down TRIM28 downregulated the Wnt/β-catenin signaling pathway, thereby inhibiting the migration, invasion, and EMT of OC cells (Deng et al., 2017). Moreover, TRIM28 inhibits the antitumor immune microenvironment by binding to SETDB1 (Lin et al., 2021). However, these studies have not focused on the ubiquitination E3 function of TRIM28 and have not fully revealed the important role of TRIM28 in CC and OC. Further research should be conducted to elucidate the carcinogenic role of TRIM28.
3.1.5 TRIM28 in other cancers
Pancreatic cancer (PC) is one of the deadliest malignant tumors (Cai et al., 2021). Yu et al. found that TRIM28 is highly expressed in PC tissues and is associated with poor prognosis (Yu et al., 2014). The overexpression of TRIM28 promotes EMT and increases the migration and invasion of PC cells in vitro and in vivo (Yu et al., 2014). Neuroblastoma (NB) is the most common extracranial solid tumor in childhood (Qiu and Matthay, 2022). MYCN amplification is characteristic of high-risk NB (Otte et al., 2020). TRIM28 is highly expressed in NB (Yang et al., 2024). Moreover, TRIM28 promotes m6A modification of MYCN mRNA by binding to METTL3 (Methyltransferase-like 3), thereby upregulating the stability of MYCN mRNA (Yang et al., 2024). Glioma is the most common intracranial tumor and is characterized by high malignancy and poor prognosis (Ostrom et al., 2015). Molecular studies have found that TRIM28 is significantly elevated in glioma samples compared to normal brain tissue and is positively correlated with tumor malignancy (Qi ZX. et al., 2016; Su et al., 2018). High TRIM28 expression may indicate poor prognosis in patients with glioma (Qi ZX. et al., 2016). TRIM28 knockout inhibits autophagy in glioblastoma cells and increases p21 expression, inducing tumor cell cycle arrest (Peng et al., 2019). TRIM28 has also been reported to function in B-cell non-Hodgkin lymphoma (B-NHL). B-NHL is progressive lymphadenopathy (Moleti et al., 2020). Zhang et.al. found that high TRIM28 expression was positively associated with poor survival in patients with B-NHL (Mohan et al., 2016). In addition, TRIM28 promotes the proliferation of B-NHL cells by modulating cell cycle progression (Mohan et al., 2016). Osteosarcoma (OS) is a primary bone malignancy (Liu et al., 2021). Vps34, an E3 ubiquitination ligase, can promote ubiquitination degradation of TSC2 (Tuberous sclerosis complex 2), thereby promoting carcinogenic transformation (Mohan et al., 2016). In osteosarcoma, TRIM28 promotes the SUMOylation modification of VPS34 by forming a complex with PVT-1 (Plasmacytoma variant translocation-1), further enhancing the ubiquitination and degradation of TSC2, thereby enhancing the self-renewal and stem cell phenotype of osteosarcoma cells (Tsang et al., 2022).
3.2 The anticancer effect of TRIM28
3.2.1 TRIM28 in KC (Kidney cancer)
In contrast to the oncogenic role of TRIM28 protein in many human cancers described above, the tumor-suppressive function of TRIM28 has been confirmed in KC. Research has shown that overexpression of TRIM28 inhibits the growth of renal cell carcinoma (RCC) cells, and high TRIM28 protein and mRNA expression is associated with better OS in RCC patients (Song et al., 2023). Biochemically, TRIM28 promotes ubiquitination and degradation of TFE3 and inhibits RCC cell proliferation (Song et al., 2023). Moreover, TRIM28 has been identified as a susceptibility gene for Wilms’ tumor (WT), with germline pathogenic variants identified in approximately 1% of isolated and 8% of familial WT cases (Hol et al., 2021). Moreover, Wegert et al. used immunohistochemical techniques and found that 44.4% (56/126) of WT cases exhibited TRIM28 deficiency (Wegert et al., 2024). This indicates that the precise detection of TRIM28 may contribute to the early detection of WT (Hol et al., 2021; Wegert et al., 2024). Taken together, these findings suggest that TRIM28 has an antitumor effect in kC cells.
3.3 The role of TRIM28 remains to be determined
3.3.1 TRIM28 in lung cancer (LC)
LC is one of the leading causes of cancer-related deaths worldwide (Sung et al., 2021). TRIM28 protein and mRNA are highly expressed in LC tissues (Liu et al., 2018). Although Chen et al. found that high TRIM28 expression was associated with increased overall survival in early LC and reduced cell proliferation in model LC cell lines (Chen et al., 2012), another study showed that TRIM28 knockdown inhibited LC cell proliferation, promoted cell apoptosis, and inhibited the growth of subcutaneous LC grafts in mice (Liu et al., 2018).
P53 is the most important tumor suppressor factor involved in various cellular signaling processes (Bykov et al., 2018). In human cells, MDM2 (mouse double minute 2) is the main regulatory factor of p53 (Haupt et al., 1997). MDM2 regulates the abundance of p53 by directly binding to p53 and promoting its ubiquitination and degradation (Haupt et al., 1997). Wang et al. found that TRIM28 can bind to MDM2 and synergistically promote p53 ubiquitination and degradation (Wang et al., 2005). Meanwhile, TRIM28 as a MAGE, activates ubiquitin E3 ligase and independently ubiquitinates p53 (Doyle et al., 2010). In addition, Liu et al. found that MAGE also inhibits the ubiquitination degradation of p53 by directly binding to MDM2 (Liu Y. et al., 2022). TRIM28 overexpression competitively binds to MAGE, thereby promoting p53 ubiquitination, degradation, and cell proliferation (Liu Y. et al., 2022). Moreover, in vitro experiments have shown that TRIM28 can also stabilize the protein level of MDM2 by promoting the ubiquitination degradation of RLIM, a ubiquitin E3 ligase of MDM2, thereby further downregulating the low expression level of p53 and ultimately promoting the proliferation, migration, and invasion of LC cells (Jin et al., 2021). Avoiding immune responses and stimulating inflammation caused by tumors are among several strategies adopted by cancer cells to maintain proliferation and progression (Whiteside, 2008; Schreiber et al., 2011). Tumor cells escape the immune system by promoting the activation of tumor suppressive cells, such as regulatory cells (Tregs) and myeloid-derived suppressor cells (MDSCs) (Whiteside, 2008; Schreiber et al., 2011; Zhao et al., 2021). Bioinformatic studies have shown a negative correlation between TRIM28 and immune infiltration (Liu et al., 2020). Liang et al. found that the expression of TRIM28 is positively correlated with MDSC infiltration in LC (Liang et al., 2023). In addition, silencing TRIM28 can enhance the efficacy of anti-PD-1 immunotherapy by reshaping the inflammatory TME (Liang et al., 2023). Mechanistically, TRIM28 directly binds to RIPK1 (Receptor-interacting protein kinase 1) and promotes K63-linked ubiquitination of RIPK1, thereby activating the NF-κB (nuclear factor NF-Kappa-B) pathway (Liang et al., 2023). Further experiments revealed that TRIM28 activates the NF-κB pathway and upregulates CXCL1 (C-X-C motif ligand 1) expression (Liang et al., 2023). CXCL1 can bind to CXCR2 (C-X-C chemokine receptor 1) on MDSCs, promoting their migration into the TME (Liang et al., 2023). TRIM28 knockout increased the responsiveness of anti-PD-1 therapy in immunocompetent mice (Liang et al., 2023).
Cisplatin (DDP) is a commonly used chemotherapeutic drug for NSCLC, which promotes cell death by inducing DNA damage in tumor cells (Kryczka et al., 2021). However, long-term DDP treatment can increase the drug resistance of LC cells, leading to tumor progression (Kryczka et al., 2021). Tan et al. found that miR-125b-5p induced resistance to DDP in LC cells (Tan et al., 2022). Further research has found that TRIM28 can promote miR-125b-5p and plays an important role in DDP resistance in LC patients (Tan et al., 2022). In addition, TRIM28 knockdown induces cell apoptosis by increasing E2F1 inactivation and downregulating the sensitivity of LC cells to etoposide therapy (Liu et al., 2017). Similar to BC, TRIM28 is also associated with EMT activation. In NSCLC cells, TRIM28 promotes TGF-β-induced EMT by silencing E-cadherin expression, thereby promoting tumor cell migration and invasion (Chen et al., 2014). Moreover, TRIM28 can form a complex with TIAM1 (T-cell invasion and metastasis-inducing protein 1) to silence E-cadherin expression and promote EMT in LC cells (Ginn et al., 2023). In summary, these findings support the crucial role of TRIM28 in the different stages of LC development, and TRIM28 may be a potential clinical therapeutic target.
3.3.2 TRIM28 in HCC (hepatocellular carcinoma)
HCC is one of the most common cancers (Sung et al., 2021). TRIM28 plays a dual role in HCC, being associated with distant metastasis in HCC patients and is closely related to poor prognosis in HCC patients, while in a mouse HCC model, it acts as a tumor suppressor to prevent tumorigenic transformation (Bojkowska et al., 2012; Wang et al., 2016).
FBP1 (Fructose-1,6-diphosphatase) has recently been identified as a tumor suppressor in HCC (Yang et al., 2017). TRIM28 induces metabolic reprogramming in HCC cells by binding to FBP1 and promoting its ubiquitination degradation, thereby promoting the occurrence and development of HCC (Jin et al., 2017). HDAC6 (Histone deacetylase 6) is a transcriptional regulatory factor, playing a crucial role in transcriptional regulation and cell cycle progression (Pulya et al., 2021). HDAC6 inhibits the progression of HCC by forming a transcriptional inhibition complex with TRIM28 (Oo et al., 2020; Li et al., 2021). Moreover, TRIM28 maintains the intracellular abundance of HDAC6 by promoting the ubiquitination degradation of HDAC6 (Li et al., 2021). LINC00064, an antisense lncRNA, enhances the interaction between HDAC6 and TRIM28, thereby accelerating the degradation of HDAC6 (Li et al., 2021). In contrast, LINC00064 binds to the RBCC domain of TRIM28, inhibiting the formation of the HDAC6-TRIM28 transcriptional co-repressor protein complex and promoting HCC progression (Li et al., 2021). CSDE1 (Cold shock domain-containing protein E1) is an RNA-binding protein that plays a vital role in tumorigenesis by coordinating oncogenic RNA regulators such as VIM and RAC1 genes (Wurth et al., 2016; Fabbri et al., 2021). Notably, TRIM28 catalyzes the poly-ubiquitination and degradation of CSDE1 (Liu J. et al., 2022). Interestingly, ARHGAP5-AS1, an lncRNA, attenuates the interaction between CSDE1 and TRIM28, which prevents the degradation of CSDE1, contributing to the progression of HCC (Liu J. et al., 2022).
Similar to LC, TRIM28 can also promote HCC progression by affecting the abundance of p53 protein in cells. Moreover, in HCC cells, URI activity and MUFA (Monounsaturated fatty acid) accumulation, and subsequently promoting cancer cell resistance to TKIs (Tyrosine kinase inhibitors) (Ding et al., 2023). Lenvatinib, a TKI, is the first-line treatment for HCC (Kudo et al., 2018). Current research indicates that TRIM28 is a target gene for WDR4, and high TRIM28 expression is significantly associated with cell-acquired stemness and lenvatinib resistance in HCC (Han et al., 2023). Bortezomib (BTZ) is a selective proteasome inhibitor that has shown promising results in the treatment of HCC (Saeki et al., 2013). The proteasome is composed of a 20S subunit and one or two 19S regulatory subunits (Saeki et al., 2013). Under the action of BTZ, TRIM28 significantly upregulates the expression of the 20s subunit (Zhang et al., 2022). Further research has shown that, under the action of BTZ, TRIM28 enters the nucleus and activates the expression of various proteasome subunits, thereby making HCC cells resistant to BTZ (Zhang et al., 2022). Moreover, nuclear TRIM28 supports the proliferation and migration of HCC cells, partially by promoting the ubiquitination degradation of p27 to promote cell cycle progression (Zhang RY. et al., 2021). Therefore, TRIM28 may be a viable target for novel anticancer agents aimed at HCC inhibition.
Platinum-based drugs have significant inhibitory effects on human HCC cells. Oxaliplatin is an important drug for the clinical chemotherapy of HCC (Li et al., 2022). Unlike in LC cells, where TRIM28 promotes cisplatin resistance, in HCC cells, TRIM28 promotes sensitivity to oxaliplatin treatment by binding to HMGB1 (high mobility group protein B1) and promoting ubiquitination-mediated lysosomal degradation (Wang et al., 2022). However, HN1 (Hematological and neurological expressed 1) can competitively bind to TRIM28, thereby inhibiting the degradation of HMGB1, promoting the proliferation and metastasis of HCC, and reducing chemotherapy sensitivity to oxaliplatin (Wang et al., 2022).
3.3.3 TRIM28 in CRC (colorectal cancer)
CRC is the second leading cause of cancer-related death (Sung et al., 2021). TRIM28 is upregulated in the interstitial tissue of patients with CRC, and its increased expression is associated with poor prognosis (Fitzgerald et al., 2013). SIK2 (Salt induced kinase 2) is an oncoprotein that is upregulated in CRC, and knocking down SIK2 can weaken the proliferation, invasion, and glycolysis of CRC cells (Ni et al., 2021). The overexpression of TRIM28 can reverse the effects of silencing SIK2 (Ni et al., 2021). Similar to BC, the phosphorylation of TRIM28 at the S473 site induced by ROS-p38MAPK effectively promotes DDR and helps CRC cells fight exogenous ROS (Shen et al., 2017). However, Cui et.al found that TRIM28 protein levels were downregulated in CRC tissues and were associated with good prognosis (Cui et al., 2019). Interestingly, TRIM28 protein was significantly overexpressed in CRC exosomes. Mechanistically, TRIM28 interacts with CARM1 (Co-activator-associated arginine methyltransferase1) and protects CARM1 from degradation, thereby inhibiting WNT/β-catenin signaling to inhibit the migration and invasion of CRC cells (Cui et al., 2019). These results indicate that TRIM28 may exert certain antitumor effects in CRC, which remains to be elucidated in the future.
3.3.4 TRIM28 in melanoma
Melanoma is a skin cancer caused by malignant melanoma, and its incidence is increasing rapidly worldwide. High TRIM28 mRNA expression is associated with the stem cell-like phenotype of melanoma cells and poor prognosis in melanoma patients (Czerwinska et al., 2020). Further research has found that TRIM28 knockdown induces the transition of melanoma from invasiveness to tumor growth (Nyberg et al., 2023). Mechanistically, TRIM28 promotes the invasiveness of melanoma cells and inhibits tumor growth by negatively regulating the expression of Jun B proto-oncogene (JUNB) (Nyberg et al., 2023). TRIM28 often forms complexes with MAGE to exert its ubiquitination E3 function. However, TRIM28 can positively regulate the abundance of MAGE proteins in melanoma cells (Song et al., 2018). BCL2A1 (B-cell lymphoma 2-related protein A1) is an anti-apoptotic member of the BCL-2 family and is associated with the resistance of melanoma cells to BRAF-targeted therapy (Haq et al., 2013). Lionnard et al. found that TRIM28 binds to BCL2A1 on the mitochondria and promotes its ubiquitination degradation, thereby upregulating the sensitivity of melanoma cells to BRAF-targeted therapy (Lionnard et al., 2019). These studies suggest that TRIM28 plays an important role in melanoma and may be a new therapeutic target for melanoma.
4 TRIM28 as a therapeutic target
As discussed, TRIM28 can regulate various biological functions, including DDR (Ziv et al., 2006; Goodarzi et al., 2011), EMT (Venkov et al., 2007; Wei et al., 2016; Ginn et al., 2023), maintaining cellular stemness (Czerwińska et al., 2017b; Li et al., 2017; Han et al., 2023). Thus, TRIM28 is a potential target for cancer treatment. In addition, the expression level of TRIM28 affects the drug resistance of tumor cells, which seriously affects the anticancer effects of treatments, such as chemotherapy, targeted therapy, and immunotherapy.
As mentioned earlier, TRIM28 may inhibit the sensitivity of tumor cells to chemotherapy. Knockdown of TRIM28 expression in LC cells promotes sensitivity to 5-FU, etoposide, and cisplatin (Damineni et al., 2017). TRIM28 weakens the sensitivity of HCC cells to BTZ by enhancing proteasome expression (Zhang et al., 2022). Bladder cancer (BCa) has a 70% prevalence of telomerase reverse transcriptase (TERT) promoter mutation, which is associated with poor patient prognosis (Kinde et al., 2013). Phosphorylation of TRIM28 by mTORC1 activates TERT transcription of mutant promoter alleles and promotes BCa cell growth (Agarwal et al., 2021). Ridaforolimus, an mTORC1 inhibitor, suppresses TRIM28 phosphorylation, hTERT expression, and cell viability (Agarwal et al., 2021). However, TRIM28 can enhance the sensitivity of HCC cells to oxaliplatin therapy by promoting the ubiquitination degradation of HMGB1 (Wang et al., 2022).
Targeted therapy is becoming increasingly important for the treatment of tumors. High TRIM28 expression promotes resistance of HCC to TKI therapy (Han et al., 2023). However, in melanoma, TRIM28 upregulates the sensitivity of melanoma cells to targeted BRAF therapy by promoting the degradation of BCL2A1 (Lionnard et al., 2019). In TRIM28 deficient cells, actinomycin D promoted p53 signal activation, indicating that TRIM28 is a probable target for p53 signal activation (Okamoto et al., 2006). JQ1 is one of the most extensively studied BET protein-selective inhibitors (Wang et al., 2018). Overexpression of TRIM28 leads to increased degradation of FBP1, which in turn inhibits the degradation of c-Myc, leading to increased resistance of PC cells to JQ1 (Wang et al., 2018).
Immunotherapy is becoming increasingly important for tumor treatment. As mentioned earlier, TRIM28 knockdown can enhance the efficacy of immunotherapy (Liang et al., 2023; Ma et al., 2023). Verteporfin is a small-molecule inhibitor of PD-L1 expression (Liang et al., 2020). Liang et al. found that verteporfin might inhibit PD-L1 expression by interfering with the interaction of TRIM28-IRF1 (Liang et al., 2020). TRIM28 is also involved in the development and activation of T cells (Tanaka et al., 2018). Gehrmann et al. found that TRIM28 can promote the differentiation of immature T cells into Tregs (Gehrmann et al., 2019). TRIM28 can inhibit the antitumor immune microenvironment (Liu et al., 2020). These studies suggest that the targeted inhibition of TRIM28 may effectively enhance the efficacy of tumor immunotherapy.
Radiation therapy can cause DNA breakage in tumor cells, thereby killing them (Moding et al., 2013). Downregulating the abundance of TRIM28 in tumor cells can increase the efficacy of radiotherapy, and ATM inhibitor (ATMi) drugs promote the sensitivity of glioma cells to radiotherapy by inhibiting TRIM28 phosphorylation (Golding et al., 2012; Lee et al., 2020).
Nanomaterials have been used in clinical trials (Kijanka et al., 2015). NB237, an anti-TRIM28 nanoparticle, significantly inhibits the invasion and growth of glioblastoma cells (Porčnik et al., 2021). However, there is currently a lack of small-molecule inhibitors targeting TRIM28, which may be an important direction for future research.
5 Discussion
Hanahan and Weinberg described ten characteristics of cancer, which are strategies that cancer cells acquire to enable survival, growth, and metastasis (Hanahan and Weinberg, 2011). Numerous studies suggest that TRIM28 is involved in cancer signaling pathways, particularly in promoting cell proliferation, immune evasion, inflammation, invasion and migration, and evasion of apoptosis. TRIM28 is not only highly expressed in multiple cancer tissues, but also promotes the degradation of multiple tumor suppressor proteins, such as p53 and AMPK, TRIM28 can upregulate the expression of oncogenes, such as AR, which promotes the development of most tumors. However, in RCC and early LC, TRIM28 plays an anti-cancer role, indicate that TRIM28 plays a dual role in the occurrence and development of tumors and is related to the background of the tumor, which requires further research. Moreover, TRIM28 knockdown can enhance the efficacy of chemotherapy, targeted therapy, and immunotherapy in cancer treatment. Meanwhile, the PTMs of TRIM28, particularly phosphorylation and SUMOylation, are crucial for its transcriptional inhibition, but their impact on its ubiquitin E3 activity is currently unclear. In addition, TRIM28 acts as both a SUMO and ubiquitin E3 ligase, cascading two different PTMs, and plays important roles in protein homeostasis and signal transduction. However, the reason for TRIM28’s selective ubiquitination or SUMOylation of substrates is currently unclear. However, this requires further investigation.
Author contributions
KL: Writing–original draft, Writing–review and editing. HW: Writing–original draft. BJ: Writing–original draft. XJ: Writing–review and editing.
Funding
The author(s) declare that financial support was received for the research, authorship, and/or publication of this article. This research was funded by the Internal Medicine Research Fund Project of Beilun District People’s Hospital (2024KYJJYB01), the National Natural Science Foundation of China (No. 32270821), and the Basic Research Funds for Universities in Zhejiang Province (No. SJLZ2022004), and Natural Science Foundation of Ningbo (No. 2021J065).
Conflict of interest
The authors declare that the research was conducted in the absence of any commercial or financial relationships that could be construed as a potential conflict of interest.
Publisher’s note
All claims expressed in this article are solely those of the authors and do not necessarily represent those of their affiliated organizations, or those of the publisher, the editors and the reviewers. Any product that may be evaluated in this article, or claim that may be made by its manufacturer, is not guaranteed or endorsed by the publisher.
References
Addison, J. B., Koontz, C., Fugett, J. H., Creighton, C. J., Chen, D., Farrugia, M. K., et al. (2015). KAP1 promotes proliferation and metastatic progression of breast cancer cells. Cancer Res. 75 (2), 344–355. doi:10.1158/0008-5472.CAN-14-1561
Agarwal, N., Rinaldetti, S., Cheikh, B. B., Zhou, Q., Hass, E. P., Jones, R. T., et al. (2021). TRIM28 is a transcriptional activator of the mutant TERT promoter in human bladder cancer. Proc. Natl. Acad. Sci. U. S. A. 118 (38), e2102423118. doi:10.1073/pnas.2102423118
Batlle, E., and Clevers, H. (2017). Cancer stem cells revisited. Nat. Med. 23 (10), 1124–1134. doi:10.1038/nm.4409
Bentz, G. L., Moss, C. R., Whitehurst, C. B., Moody, C. A., and Pagano, J. S. (2015). LMP1-Induced sumoylation influences the maintenance of epstein-barr virus latency through KAP1. J. virology 89 (15), 7465–7477. doi:10.1128/JVI.00711-15
Bhatia, N., Xiao, T. Z., Rosenthal, K. A., Siddiqui, I. A., Thiyagarajan, S., Smart, B., et al. (2013). MAGE-C2 promotes growth and tumorigenicity of melanoma cells, phosphorylation of KAP1, and DNA damage repair. J. investigative dermatology 133 (3), 759–767. doi:10.1038/jid.2012.355
Blasius, M., Forment, J. V., Thakkar, N., Wagner, S. A., Choudhary, C., and Jackson, S. P. (2011). A phospho-proteomic screen identifies substrates of the checkpoint kinase Chk1. Genome Biol. 12 (8), R78. doi:10.1186/gb-2011-12-8-r78
Bojkowska, K., Aloisio, F., Cassano, M., Kapopoulou, A., Santoni de Sio, F., Zangger, N., et al. (2012). Liver-specific ablation of Krüppel-associated box-associated protein 1 in mice leads to male-predominant hepatosteatosis and development of liver adenoma. Hepatol. Baltim. Md 56 (4), 1279–1290. doi:10.1002/hep.25767
Bunch, H., Zheng, X., Burkholder, A., Dillon, S. T., Motola, S., Birrane, G., et al. (2014). TRIM28 regulates RNA polymerase II promoter-proximal pausing and pause release. Nat. Struct. Mol. Biol. 21 (10), 876–883. doi:10.1038/nsmb.2878
Burke, A. P., Yen, T. S., Shekitka, K. M., and Sobin, L. H. (1990). Lymphoepithelial carcinoma of the stomach with Epstein-Barr virus demonstrated by polymerase chain reaction. Mod. Pathol. 3 (3), 377–380.
Bykov, V. J. N., Eriksson, S. E., Bianchi, J., and Wiman, K. G. (2018). Targeting mutant p53 for efficient cancer therapy. Nat. Rev. Cancer 18 (2), 89–102. doi:10.1038/nrc.2017.109
Cai, J., Chen, H., Lu, M., Zhang, Y., Lu, B., You, L., et al. (2021). Advances in the epidemiology of pancreatic cancer: trends, risk factors, screening, and prognosis. Cancer Lett. 520, 1–11. doi:10.1016/j.canlet.2021.06.027
Chang, C. W., Chou, H. Y., Lin, Y. S., Huang, K. H., Chang, C. J., Hsu, T. C., et al. (2008). Phosphorylation at Ser473 regulates heterochromatin protein 1 binding and corepressor function of TIF1beta/KAP1. BMC Mol. Biol. 9, 61. doi:10.1186/1471-2199-9-61
Chaube, B., and Bhat, M. K. (2016). AMPK, a key regulator of metabolic/energy homeostasis and mitochondrial biogenesis in cancer cells. Cell Death Dis. 7 (1), e2044. doi:10.1038/cddis.2015.404
Chen, L., Chen, D. T., Kurtyka, C., Rawal, B., Fulp, W. J., Haura, E. B., et al. (2012). Tripartite motif containing 28 (Trim28) can regulate cell proliferation by bridging HDAC1/E2F interactions. J. Biol. Chem. 287 (48), 40106–40118. doi:10.1074/jbc.M112.380865
Chen, L., Muñoz-Antonia, T., and Cress, W. D. (2014). Trim28 contributes to EMT via regulation of E-cadherin and N-cadherin in lung cancer cell lines. PloS one 9 (7), e101040. doi:10.1371/journal.pone.0101040
Chen, Y., McAndrews, K. M., and Kalluri, R. (2021). Clinical and therapeutic relevance of cancer-associated fibroblasts. Nat. Rev. Clin. Oncol. 18 (12), 792–804. doi:10.1038/s41571-021-00546-5
Cheng, C. T., Kuo, C. Y., Ouyang, C., Li, C. F., Chung, Y., Chan, D. C., et al. (2016). Metabolic stress-induced phosphorylation of KAP1 Ser473 blocks mitochondrial fusion in breast cancer cells. Cancer Res. 76 (17), 5006–5018. doi:10.1158/0008-5472.CAN-15-2921
Cui, J., Hu, J., Ye, Z., Fan, Y., Li, Y., Wang, G., et al. (2019). TRIM28 protects CARM1 from proteasome-mediated degradation to prevent colorectal cancer metastasis. Sci. Bull. 64 (14), 986–997. doi:10.1016/j.scib.2019.05.024
Cui, Y., Yang, S., Fu, X., Feng, J., Xu, S., and Ying, G. (2014). High levels of KAP1 expression are associated with aggressive clinical features in ovarian cancer. Int. J. Mol. Sci. 16 (1), 363–377. doi:10.3390/ijms16010363
Czerwinska, P., Jaworska, A. M., Wlodarczyk, N. A., and Mackiewicz, A. A. (2020). Melanoma stem cell-like phenotype and significant suppression of immune response within a tumor are regulated by TRIM28 protein. Cancers 12 (10), 2998. doi:10.3390/cancers12102998
Czerwińska, P., Mazurek, S., and Wiznerowicz, M. (2017a). The complexity of TRIM28 contribution to cancer. J. Biomed. Sci. 24 (1), 63. doi:10.1186/s12929-017-0374-4
Czerwińska, P., Shah, P. K., Tomczak, K., Klimczak, M., Mazurek, S., Sozańska, B., et al. (2017b). TRIM28 multi-domain protein regulates cancer stem cell population in breast tumor development. Oncotarget 8 (1), 863–882. doi:10.18632/oncotarget.13273
Dai, C., Heemers, H., and Sharifi, N. (2017). Androgen signaling in prostate cancer. Cold Spring Harb. Perspect. Med. 7 (9), a030452. doi:10.1101/cshperspect.a030452
Damineni, S., Balaji, S. A., Shettar, A., Nayanala, S., Kumar, N., Kruthika, B. S., et al. (2017). Expression of tripartite motif-containing protein 28 in primary breast carcinoma predicts metastasis and is involved in the stemness, chemoresistance, and tumor growth. Tumour Biol. 39 (4), 1010428317695919. doi:10.1177/1010428317695919
Deng, B., Zhang, S., Zhang, Y., Miao, Y., Meng, X., and Guo, K. (2017). Knockdown of Tripartite Motif Containing 28 suppresses the migration, invasion and epithelial-mesenchymal transition in ovarian carcinoma cells through down-regulation of Wnt/β-catenin signaling pathway. Neoplasma 64 (6), 893–900. doi:10.4149/neo_2017_611
di Caprio, R., Ciano, M., Montano, G., Costanzo, P., and Cesaro, E. (2015). KAP1 is a novel substrate for the arginine methyltransferase PRMT5. Biol. (Basel) 4 (1), 41–49. doi:10.3390/biology4010041
Ding, Z., Pan, Y., Shang, T., Jiang, T., Lin, Y., Yang, C., et al. (2023). URI alleviates tyrosine kinase inhibitors-induced ferroptosis by reprogramming lipid metabolism in p53 wild-type liver cancers. Nat. Commun. 14 (1), 6269. doi:10.1038/s41467-023-41852-z
Doyle, J. M., Gao, J., Wang, J., Yang, M., and Potts, P. R. (2010). MAGE-RING protein complexes comprise a family of E3 ubiquitin ligases. Mol. Cell 39 (6), 963–974. doi:10.1016/j.molcel.2010.08.029
Engeland, K. (2022). Cell cycle regulation: p53-p21-RB signaling. Cell death Differ. 29 (5), 946–960. doi:10.1038/s41418-022-00988-z
Fabbri, L., Chakraborty, A., Robert, C., and Vagner, S. (2021). The plasticity of mRNA translation during cancer progression and therapy resistance. Nat. Rev. Cancer 21 (9), 558–577. doi:10.1038/s41568-021-00380-y
Farrell, P. J. (2019). Epstein-barr virus and cancer. Annu. Rev. pathology 14, 29–53. doi:10.1146/annurev-pathmechdis-012418-013023
Fitzgerald, S., Sheehan, K. M., O'Grady, A., Kenny, D., O'Kennedy, R., Kay, E. W., et al. (2013). Relationship between epithelial and stromal TRIM28 expression predicts survival in colorectal cancer patients. J. gastroenterology hepatology 28 (6), 967–974. doi:10.1111/jgh.12157
Fong, K. W., Zhao, J. C., Song, B., Zheng, B., and Yu, J. (2018). TRIM28 protects TRIM24 from SPOP-mediated degradation and promotes prostate cancer progression. Nat. Commun. 9 (1), 5007. doi:10.1038/s41467-018-07475-5
Galbraith, M. D., Allen, M. A., Bensard, C. L., Wang, X., Schwinn, M. K., Qin, B., et al. (2013). HIF1A employs CDK8-mediator to stimulate RNAPII elongation in response to hypoxia. Cell 153 (6), 1327–1339. doi:10.1016/j.cell.2013.04.048
Garvin, A. J., Densham, R. M., Blair-Reid, S. A., Pratt, K. M., Stone, H. R., Weekes, D., et al. (2013). The deSUMOylase SENP7 promotes chromatin relaxation for homologous recombination DNA repair. EMBO Rep. 14 (11), 975–983. doi:10.1038/embor.2013.141
Gehrmann, U., Burbage, M., Zueva, E., Goudot, C., Esnault, C., Ye, M., et al. (2019). Critical role for TRIM28 and HP1β/γ in the epigenetic control of T cell metabolic reprograming and effector differentiation. Proc. Natl. Acad. Sci. U. S. A. 116 (51), 25839–25849. doi:10.1073/pnas.1901639116
Ginn, L., Maltas, J., Baker, M. J., Chaturvedi, A., Wilson, L., Guilbert, R., et al. (2023). A TIAM1-TRIM28 complex mediates epigenetic silencing of protocadherins to promote migration of lung cancer cells. Proc. Natl. Acad. Sci. U. S. A. 120 (40), e2300489120. doi:10.1073/pnas.2300489120
Golding, S. E., Rosenberg, E., Adams, B. R., Wignarajah, S., Beckta, J. M., O'Connor, M. J., et al. (2012). Dynamic inhibition of ATM kinase provides a strategy for glioblastoma multiforme radiosensitization and growth control. Cell cycleGeorget. Tex 11 (6), 1167–1173. doi:10.4161/cc.11.6.19576
Gomes, L. C., Di Benedetto, G., and Scorrano, L. (2011). During autophagy mitochondria elongate, are spared from degradation and sustain cell viability. Nat. Cell Biol. 13 (5), 589–598. doi:10.1038/ncb2220
Goodarzi, A. A., Kurka, T., and Jeggo, P. A. (2011). KAP-1 phosphorylation regulates CHD3 nucleosome remodeling during the DNA double-strand break response. Nat. Struct. Mol. Biol. 18 (7), 831–839. doi:10.1038/nsmb.2077
Han, W. Y., Wang, J., Zhao, J., Zheng, Y. M., Chai, X. Q., Gao, C., et al. (2023). WDR4/TRIM28 is a novel molecular target linked to lenvatinib resistance that helps retain the stem characteristics in hepatocellular carcinomas. Cancer Lett. 568, 216259. doi:10.1016/j.canlet.2023.216259
Hanahan, D., and Weinberg, R. A. (2011). Hallmarks of cancer: the next generation. Cell 144 (5), 646–674. doi:10.1016/j.cell.2011.02.013
Hao, L., Leng, J., Xiao, R., Kingsley, T., Li, X., Tu, Z., et al. (2017). Bioinformatics analysis of the prognostic value of Tripartite Motif 28 in breast cancer. Oncol. Lett. 13 (4), 2670–2678. doi:10.3892/ol.2017.5764
Haq, R., Yokoyama, S., Hawryluk, E. B., Jönsson, G. B., Frederick, D. T., McHenry, K., et al. (2013). BCL2A1 is a lineage-specific antiapoptotic melanoma oncogene that confers resistance to BRAF inhibition. Proc. Natl. Acad. Sci. U. S. A. 110 (11), 4321–4326. doi:10.1073/pnas.1205575110
Harris, A. L. (2002). Hypoxia--a key regulatory factor in tumour growth. Nat. Rev. Cancer 2 (1), 38–47. doi:10.1038/nrc704
Hatakeyama, S. (2017). TRIM family proteins: roles in autophagy, immunity, and carcinogenesis. Trends Biochem. Sci. 42 (4), 297–311. doi:10.1016/j.tibs.2017.01.002
Haupt, Y., Maya, R., Kazaz, A., and Oren, M. (1997). Mdm2 promotes the rapid degradation of p53. Nature 387 (6630), 296–299. doi:10.1038/387296a0
Hol, J. A., Diets, I. J., de Krijger, R. R., van den Heuvel-Eibrink, M. M., Jongmans, M. C., and Kuiper, R. P. (2021). TRIM28 variants and Wilms' tumour predisposition. J. pathology 254 (4), 494–504. doi:10.1002/path.5639
Hu, C., Zhang, S., Gao, X., Gao, X., Xu, X., Lv, Y., et al. (2012). Roles of kruppel-associated box (KRAB)-associated Co-repressor KAP1 ser-473 phosphorylation in DNA damage response. J. Biol. Chem. 287 (23), 18937–18952. doi:10.1074/jbc.M111.313262
Huang, Z., Li, X., Tang, B., Li, H., Zhang, J., Sun, R., et al. (2023). SETDB1 modulates degradation of phosphorylated RB and anticancer efficacy of CDK4/6 inhibitors. Cancer Res. 83 (6), 875–889. doi:10.1158/0008-5472.CAN-22-0264
International Human Genome Sequencing Consortium (2004). Finishing the euchromatic sequence of the human genome. Nature 431 (7011), 931–945. doi:10.1038/nature03001
Ivanov, A. V., Peng, H., Yurchenko, V., Yap, K. L., Negorev, D. G., Schultz, D. C., et al. (2007). PHD domain-mediated E3 ligase activity directs intramolecular sumoylation of an adjacent bromodomain required for gene silencing. Mol. Cell 28 (5), 823–837. doi:10.1016/j.molcel.2007.11.012
Jackson, S. P., and Bartek, J. (2009). The DNA-damage response in human biology and disease. Nature 461 (7267), 1071–1078. doi:10.1038/nature08467
Jin, J. O., Lee, G. D., Nam, S. H., Lee, T. H., Kang, D. H., Yun, J. K., et al. (2021). Sequential ubiquitination of p53 by TRIM28, RLIM, and MDM2 in lung tumorigenesis. Cell death Differ. 28 (6), 1790–1803. doi:10.1038/s41418-020-00701-y
Jin, X., Pan, Y., Wang, L., Zhang, L., Ravichandran, R., Potts, P. R., et al. (2017). MAGE-TRIM28 complex promotes the Warburg effect and hepatocellular carcinoma progression by targeting FBP1 for degradation. Oncogenesis 6 (4), e312. doi:10.1038/oncsis.2017.21
Kang, Y., and Massagué, J. (2004). Epithelial-mesenchymal transitions: twist in development and metastasis. Cell 118 (3), 277–279. doi:10.1016/j.cell.2004.07.011
Kijanka, M., Dorresteijn, B., Oliveira, S., and van Bergen en Henegouwen, P. M. (2015). Nanobody-based cancer therapy of solid tumors. Nanomedicine Lond. Engl. 10 (1), 161–174. doi:10.2217/nnm.14.178
Kinde, I., Munari, E., Faraj, S. F., Hruban, R. H., Schoenberg, M., Bivalacqua, T., et al. (2013). TERT promoter mutations occur early in urothelial neoplasia and are biomarkers of early disease and disease recurrence in urine. Cancer Res. 73 (24), 7162–7167. doi:10.1158/0008-5472.CAN-13-2498
Kryczka, J., Kryczka, J., Czarnecka-Chrebelska, K. H., and Brzeziańska-Lasota, E. (2021). Molecular mechanisms of chemoresistance induced by cisplatin in NSCLC cancer therapy. Int. J. Mol. Sci. 22 (16), 8885. doi:10.3390/ijms22168885
Kudo, M., Finn, R. S., Qin, S., Han, K. H., Ikeda, K., Piscaglia, F., et al. (2018). Lenvatinib versus sorafenib in first-line treatment of patients with unresectable hepatocellular carcinoma: a randomised phase 3 non-inferiority trial. Lancet London, Engl. 391 (10126), 1163–1173. doi:10.1016/S0140-6736(18)30207-1
Kuo, C. Y., Li, X., Kong, X. Q., Luo, C., Chang, C. C., Chung, Y., et al. (2014). An arginine-rich motif of ring finger protein 4 (RNF4) oversees the recruitment and degradation of the phosphorylated and SUMOylated Krüppel-associated box domain-associated protein 1 (KAP1)/TRIM28 protein during genotoxic stress. J. Biol. Chem. 289 (30), 20757–20772. doi:10.1074/jbc.M114.555672
Lajer, H., Jensen, M. B., Kilsmark, J., Albæk, J., Svane, D., Mirza, M. R., et al. (2010). The value of gynecologic cancer follow-up: evidence-based ignorance? Int. J. Gynecol. cancer 20 (8), 1307–1320. doi:10.1111/IGC.0b013e3181f3bee0
Lee, A. K., Pan, D., Bao, X., Hu, M., Li, F., and Li, C. Y. (2020). Endogenous retrovirus activation as a key mechanism of anti-tumor immune response in radiotherapy. Radiat. Res. 193 (4), 305–317. doi:10.1667/RADE-20-00013
Lee, Y. K., Thomas, S. N., Yang, A. J., and Ann, D. K. (2007). Doxorubicin down-regulates Kruppel-associated box domain-associated protein 1 sumoylation that relieves its transcription repression on p21WAF1/CIP1 in breast cancer MCF-7 cells. J. Biol. Chem. 282 (3), 1595–1606. doi:10.1074/jbc.M606306200
Li, D. Q., Nair, S. S., Ohshiro, K., Kumar, A., Nair, V. S., Pakala, S. B., et al. (2012). MORC2 signaling integrates phosphorylation-dependent, ATPase-coupled chromatin remodeling during the DNA damage response. Cell Rep. 2 (6), 1657–1669. doi:10.1016/j.celrep.2012.11.018
Li, F., Wang, Z., and Lu, G. (2018). TRIM28 promotes cervical cancer growth through the mTOR signaling pathway. Oncol. Rep. 39 (4), 1860–1866. doi:10.3892/or.2018.6235
Li, J., Xi, Y., Li, W., McCarthy, R. L., Stratton, S. A., Zou, W., et al. (2017). TRIM28 interacts with EZH2 and SWI/SNF to activate genes that promote mammosphere formation. Oncogene 36 (21), 2991–3001. doi:10.1038/onc.2016.453
Li, K., Xia, Y., He, J., Wang, J., Li, J., Ye, M., et al. (2023). The SUMOylation and ubiquitination crosstalk in cancer. J. cancer Res. Clin. Oncol. 149 (17), 16123–16146. doi:10.1007/s00432-023-05310-z
Li, Q. J., He, M. K., Chen, H. W., Fang, W. Q., Zhou, Y. M., Xu, L., et al. (2022). Hepatic arterial infusion of oxaliplatin, fluorouracil, and leucovorin versus transarterial chemoembolization for large hepatocellular carcinoma: a randomized phase III trial. J. Clin. Oncol. 40 (2), 150–160. doi:10.1200/JCO.21.00608
Li, Y., and Cucinotta, F. A. (2020). Mathematical model of ATM activation and chromatin relaxation by ionizing radiation. Int. J. Mol. Sci. 21 (4), 1214. doi:10.3390/ijms21041214
Li, Z., Lu, X., Liu, Y., Zhao, J., Ma, S., Yin, H., et al. (2021). Gain of LINC00624 enhances liver cancer progression by disrupting the histone deacetylase 6/tripartite motif containing 28/zinc finger protein 354C corepressor complex. Hepatol. Baltim. Md 73 (5), 1764–1782. doi:10.1002/hep.31530
Liang, J., Wang, L., Wang, C., Shen, J., Su, B., Marisetty, A. L., et al. (2020). Verteporfin inhibits PD-L1 through autophagy and the STAT1-IRF1-TRIM28 signaling Axis, exerting antitumor efficacy. Cancer Immunol. Res. 8 (7), 952–965. doi:10.1158/2326-6066.CIR-19-0159
Liang, M., Sun, Z., Chen, X., Wang, L., Wang, H., Qin, L., et al. (2023). E3 ligase TRIM28 promotes anti-PD-1 resistance in non-small cell lung cancer by enhancing the recruitment of myeloid-derived suppressor cells. J. Exp. Clin. cancer Res. 42 (1), 275. doi:10.1186/s13046-023-02862-3
Lin, J., Guo, D., Liu, H., Zhou, W., Wang, C., Müller, I., et al. (2021). The SETDB1-TRIM28 complex suppresses antitumor immunity. Cancer Immunol. Res. 9 (12), 1413–1424. doi:10.1158/2326-6066.CIR-21-0754
Lin, L. F., Li, C. F., Wang, W. J., Yang, W. M., Wang, D. D., Chang, W. C., et al. (2013). Loss of ZBRK1 contributes to the increase of KAP1 and promotes KAP1-mediated metastasis and invasion in cervical cancer. PloS one 8 (8), e73033. doi:10.1371/journal.pone.0073033
Lin, Y. H., Yuan, J., Pei, H., Liu, T., Ann, D. K., and Lou, Z. (2015). KAP1 deacetylation by SIRT1 promotes non-homologous end-joining repair. PloS one 10 (4), e0123935. doi:10.1371/journal.pone.0123935
Lionnard, L., Duc, P., Brennan, M. S., Kueh, A. J., Pal, M., Guardia, F., et al. (2019). TRIM17 and TRIM28 antagonistically regulate the ubiquitination and anti-apoptotic activity of BCL2A1. Cell death Differ. 26 (5), 902–917. doi:10.1038/s41418-018-0169-5
Liu, J., Han, X., Chen, L., Han, D., Mu, X., Hu, X., et al. (2020). TRIM28 is a distinct prognostic biomarker that worsens the tumor immune microenvironment in lung adenocarcinoma. Aging (Albany NY) 12 (20), 20308–20331. doi:10.18632/aging.103804
Liu, J., Zhang, N., Zeng, J., Wang, T., Shen, Y., Ma, C., et al. (2022b). N(6) -methyladenosine-modified lncRNA ARHGAP5-AS1 stabilises CSDE1 and coordinates oncogenic RNA regulons in hepatocellular carcinoma. Clin. Transl. Med. 12 (11), e1107. doi:10.1002/ctm2.1107
Liu, L., Xiao, L., Liang, X., Chen, L., Cheng, L., Zhang, L., et al. (2017). TRIM28 knockdown increases sensitivity to etoposide by upregulating E2F1 in non-small cell lung cancer. Oncol. Rep. 37 (6), 3597–3605. doi:10.3892/or.2017.5638
Liu, L., Zhang, L., Wang, J., Zhao, X., Xu, Q., Lu, Y., et al. (2018). Downregulation of TRIM28 inhibits growth and increases apoptosis of nude mice with non-small cell lung cancer xenografts. Mol. Med. Rep. 17 (1), 835–842. doi:10.3892/mmr.2017.7955
Liu, R., Hu, Y., Liu, T., and Wang, Y. (2021). Profiles of immune cell infiltration and immune-related genes in the tumor microenvironment of osteosarcoma cancer. BMC cancer 21 (1), 1345. doi:10.1186/s12885-021-09042-6
Liu, Y., Cao, B., Hu, L., Ye, J., Tian, W., and He, X. (2022a). The dual roles of MAGE-C2 in p53 ubiquitination and cell proliferation through E3 ligases MDM2 and TRIM28. Front. Cell Dev. Biol. 10, 922675. doi:10.3389/fcell.2022.922675
Lu, G., Du, R., Dong, J., Sun, Y., Zhou, F., Feng, F., et al. (2023). Cancer associated fibroblast derived SLIT2 drives gastric cancer cell metastasis by activating NEK9. Cell Death Dis. 14 (7), 421. doi:10.1038/s41419-023-05965-z
Ma, X., Jia, S., Wang, G., Liang, M., Guo, T., Du, H., et al. (2023). TRIM28 promotes the escape of gastric cancer cells from immune surveillance by increasing PD-L1 abundance. Signal Transduct. Target. Ther. 8 (1), 246. doi:10.1038/s41392-023-01450-3
Magni, M., Ruscica, V., Restelli, M., Fontanella, E., Buscemi, G., and Zannini, L. (2015). CCAR2/DBC1 is required for Chk2-dependent KAP1 phosphorylation and repair of DNA damage. Oncotarget 6 (19), 17817–17831. doi:10.18632/oncotarget.4417
Mita, P., Savas, J. N., Briggs, E. M., Ha, S., Gnanakkan, V., Yates, J. R., et al. (2016). URI regulates KAP1 phosphorylation and transcriptional repression via PP2A phosphatase in prostate cancer cells. J. Biol. Chem. 291 (49), 25516–25528. doi:10.1074/jbc.M116.741660
Moding, E. J., Kastan, M. B., and Kirsch, D. G. (2013). Strategies for optimizing the response of cancer and normal tissues to radiation. Nat. Rev. Drug Discov. 12 (7), 526–542. doi:10.1038/nrd4003
Mohan, N., Shen, Y., Dokmanovic, M., Endo, Y., Hirsch, D. S., and Wu, W. J. (2016). VPS34 regulates TSC1/TSC2 heterodimer to mediate RheB and mTORC1/S6K1 activation and cellular transformation. Oncotarget 7 (32), 52239–52254. doi:10.18632/oncotarget.10469
Moleti, M. L., Testi, A. M., and Foà, R. (2020). Treatment of relapsed/refractory paediatric aggressive B-cell non-Hodgkin lymphoma. Br. J. Haematol. 189 (5), 826–843. doi:10.1111/bjh.16461
Ni, X., Feng, Y., and Fu, X. (2021). Role of salt-inducible kinase 2 in the malignant behavior and glycolysis of colorectal cancer cells. Mol. Med. Rep. 24 (5), 822. doi:10.3892/mmr.2021.12460
Ning, T., Zhao, M., Zhang, N., Wang, Z., Zhang, S., Liu, M., et al. (2023). TRIM28 suppresses cancer stem-like characteristics in gastric cancer cells through Wnt/β-catenin signaling pathways. Exp. Biol. Med. (Maywood, NJ) 248 (23), 2210–2218. doi:10.1177/15353702231211970
Nyberg, W. A., Velasquez-Pulgarin, D. A., He, T., Sjöstrand, M., Pellé, L., Covacu, R., et al. (2023). The bromodomain protein TRIM28 controls the balance between growth and invasiveness in melanoma. EMBO Rep. 24 (1), e54944. doi:10.15252/embr.202254944
Okamoto, K., Kitabayashi, I., and Taya, Y. (2006). KAP1 dictates p53 response induced by chemotherapeutic agents via Mdm2 interaction. Biochem. Biophys. Res. Commun. 351 (1), 216–222. doi:10.1016/j.bbrc.2006.10.022
Oo, J. A., Irmer, B., Günther, S., Warwick, T., Pálfi, K., Izquierdo Ponce, J., et al. (2020). ZNF354C is a transcriptional repressor that inhibits endothelial angiogenic sprouting. Sci. Rep. 10 (1), 19079. doi:10.1038/s41598-020-76193-0
Ostrom, Q. T., Gittleman, H., Stetson, L., Virk, S. M., and Barnholtz-Sloan, J. S. (2015). Epidemiology of gliomas. Cancer Treat. Res. 163, 1–14. doi:10.1007/978-3-319-12048-5_1
Otte, J., Dyberg, C., Pepich, A., and Johnsen, J. I. (2020). MYCN function in neuroblastoma development. Front. Oncol. 10, 624079. doi:10.3389/fonc.2020.624079
Park, H. H., Kim, H. R., Park, S. Y., Hwang, S. M., Hong, S. M., Park, S., et al. (2021). RIPK3 activation induces TRIM28 derepression in cancer cells and enhances the anti-tumor microenvironment. Mol. Cancer 20 (1), 107. doi:10.1186/s12943-021-01399-3
Parker, K. A., Gooding, A. J., Valadkhan, S., and Schiemann, W. P. (2021). lncRNA BORG:TRIM28 complexes drive metastatic progression by inducing α6 integrin/CD49f expression in breast cancer stem cells. Mol. cancer Res. 19 (12), 2068–2080. doi:10.1158/1541-7786.MCR-21-0137
Peng, H., Begg, G. E., Schultz, D. C., Friedman, J. R., Jensen, D. E., Speicher, D. W., et al. (2000). Reconstitution of the KRAB-KAP-1 repressor complex: a model system for defining the molecular anatomy of RING-B box-coiled-coil domain-mediated protein-protein interactions. J. Mol. Biol. 295 (5), 1139–1162. doi:10.1006/jmbi.1999.3402
Peng, H., Gibson, L. C., Capili, A. D., Borden, K. L., Osborne, M. J., Harper, S. L., et al. (2007). The structurally disordered KRAB repression domain is incorporated into a protease resistant core upon binding to KAP-1-RBCC domain. J. Mol. Biol. 370 (2), 269–289. doi:10.1016/j.jmb.2007.03.047
Peng, Y., Zhang, M., Jiang, Z., and Jiang, Y. (2019). TRIM28 activates autophagy and promotes cell proliferation in glioblastoma. OncoTargets Ther. 12, 397–404. doi:10.2147/OTT.S188101
Pineda, C. T., and Potts, P. R. (2015). Oncogenic MAGEA-TRIM28 ubiquitin ligase downregulates autophagy by ubiquitinating and degrading AMPK in cancer. Autophagy 11 (5), 844–846. doi:10.1080/15548627.2015.1034420
Porčnik, A., Novak, M., Breznik, B., Majc, B., Hrastar, B., Šamec, N., et al. (2021). TRIM28 selective nanobody reduces glioblastoma stem cell invasion. Mol. Basel, Switz. 26 (17), 5141. doi:10.3390/molecules26175141
Pulya, S., Amin, S. A., Adhikari, N., Biswas, S., Jha, T., and Ghosh, B. (2021). HDAC6 as privileged target in drug discovery: a perspective. Pharmacol. Res. 163, 105274. doi:10.1016/j.phrs.2020.105274
Qi, Z., Cai, S., Cai, J., Chen, L., Yao, Y., Chen, L., et al. (2016a). miR-491 regulates glioma cells proliferation by targeting TRIM28 in vitro. BMC Neurol. 16 (1), 248. doi:10.1186/s12883-016-0769-y
Qi, Z. X., Cai, J. J., Chen, L. C., Yue, Q., Gong, Y., Yao, Y., et al. (2016b). TRIM28 as an independent prognostic marker plays critical roles in glioma progression. J. neuro-oncology 126 (1), 19–26. doi:10.1007/s11060-015-1897-8
Qiu, B., and Matthay, K. K. (2022). Advancing therapy for neuroblastoma. Nat. Rev. Clin. Oncol. 19 (8), 515–533. doi:10.1038/s41571-022-00643-z
Ramazi, S., and Zahiri, J. (2021). Posttranslational modifications in proteins: resources, tools and prediction methods. Database (Oxford) 2021, baab012. doi:10.1093/database/baab012
Rawla, P. (2019). Epidemiology of prostate cancer. World J. Oncol. 10 (2), 63–89. doi:10.14740/wjon1191
Saeki, I., Terai, S., Fujisawa, K., Takami, T., Yamamoto, N., Matsumoto, T., et al. (2013). Bortezomib induces tumor-specific cell death and growth inhibition in hepatocellular carcinoma and improves liver fibrosis. J. gastroenterology 48 (6), 738–750. doi:10.1007/s00535-012-0675-z
Schreiber, R. D., Old, L. J., and Smyth, M. J. (2011). Cancer immunoediting: integrating immunity's roles in cancer suppression and promotion. Science 331 (6024), 1565–1570. doi:10.1126/science.1203486
Semenza, G. L. (2014). Hypoxia-inducible factor 1 and cardiovascular disease. Annu. Rev. physiology 76, 39–56. doi:10.1146/annurev-physiol-021113-170322
Shah, P. A., Boutros-Suleiman, S., Emanuelli, A., Paolini, B., Levy-Cohen, G., and Blank, M. (2022). The emerging role of E3 ubiquitin ligase SMURF2 in the regulation of transcriptional Co-repressor KAP1 in untransformed and cancer cells and tissues. Cancers 14 (7), 1607. doi:10.3390/cancers14071607
Shen, D. D., Pang, J. R., Bi, Y. P., Zhao, L. F., Li, Y. R., Zhao, L. J., et al. (2022). LSD1 deletion decreases exosomal PD-L1 and restores T-cell response in gastric cancer. Mol. Cancer 21 (1), 75. doi:10.1186/s12943-022-01557-1
Shen, L. T., Chou, H. E., and Kato, M. (2017). TIF1β is phosphorylated at serine 473 in colorectal tumor cells through p38 mitogen-activated protein kinase as an oxidative defense mechanism. Biochem. Biophys. Res. Commun. 492 (3), 310–315. doi:10.1016/j.bbrc.2017.08.117
Sheng, Z., Zhu, J., Deng, Y. N., Gao, S., and Liang, S. (2021). SUMOylation modification-mediated cell death. Open Biol. 11 (7), 210050. doi:10.1098/rsob.210050
Song, T., Lv, S., Ma, X., Zhao, X., Fan, L., Zou, Q., et al. (2023). TRIM28 represses renal cell carcinoma cell proliferation by inhibiting TFE3/KDM6A-regulated autophagy. J. Biol. Chem. 299 (5), 104621. doi:10.1016/j.jbc.2023.104621
Song, X., Guo, C., Zheng, Y., Wang, Y., Jin, Z., and Yin, Y. (2018). Post-transcriptional regulation of cancer/testis antigen MAGEC2 expression by TRIM28 in tumor cells. BMC cancer 18 (1), 971. doi:10.1186/s12885-018-4844-1
Sripathy, S. P., Stevens, J., and Schultz, D. C. (2006). The KAP1 corepressor functions to coordinate the assembly of de novo HP1-demarcated microenvironments of heterochromatin required for KRAB zinc finger protein-mediated transcriptional repression. Mol. Cell. Biol. 26 (22), 8623–8638. doi:10.1128/MCB.00487-06
Su, C., Li, H., and Gao, W. (2018). TRIM28 is overexpressed in glioma and associated with tumor progression. OncoTargets Ther. 11, 6447–6458. doi:10.2147/OTT.S168630
Sun, Y., Keown, J. R., Black, M. M., Raclot, C., Demarais, N., Trono, D., et al. (2019). A dissection of oligomerization by the TRIM28 tripartite motif and the interaction with members of the krab-ZFP family. J. Mol. Biol. 431 (14), 2511–2527. doi:10.1016/j.jmb.2019.05.002
Sung, H., Ferlay, J., Siegel, R. L., Laversanne, M., Soerjomataram, I., Jemal, A., et al. (2021). Global cancer statistics 2020: GLOBOCAN estimates of incidence and mortality worldwide for 36 cancers in 185 countries. CA a cancer J. Clin. 71 (3), 209–249. doi:10.3322/caac.21660
Tan, Q., Ma, J., Zhang, H., Wu, X., Li, Q., Zuo, X., et al. (2022). miR-125b-5p upregulation by TRIM28 induces cisplatin resistance in non-small cell lung cancer through CREB1 inhibition. BMC Pulm. Med. 22 (1), 469. doi:10.1186/s12890-022-02272-9
Tanaka, S., Pfleger, C., Lai, J. F., Roan, F., Sun, S. C., and Ziegler, S. F. (2018). KAP1 regulates regulatory T cell function and proliferation in both foxp3-dependent and -independent manners. Cell Rep. 23 (3), 796–807. doi:10.1016/j.celrep.2018.03.099
Tsang, S. V., Rainusso, N., Liu, M., Nomura, M., Patel, T. D., Nakahata, K., et al. (2022). LncRNA PVT-1 promotes osteosarcoma cancer stem-like properties through direct interaction with TRIM28 and TSC2 ubiquitination. Oncogene 41 (50), 5373–5384. doi:10.1038/s41388-022-02538-w
Turchi, L., Sakakini, N., Saviane, G., Polo, B., Saurty-Seerunghen, M. S., Gabut, M., et al. (2023). CELF2 sustains a proliferating/olig2+ glioblastoma cell phenotype via the epigenetic repression of SOX3. Cancers 15 (20), 5038. doi:10.3390/cancers15205038
Van Tilborgh, N., Spans, L., Helsen, C., Clinckemalie, L., Dubois, V., Lerut, E., et al. (2013). The transcription intermediary factor 1β coactivates the androgen receptor. J. Endocrinol. investigation 36 (9), 699–706. doi:10.3275/8927
Venkov, C. D., Link, A. J., Jennings, J. L., Plieth, D., Inoue, T., Nagai, K., et al. (2007). A proximal activator of transcription in epithelial-mesenchymal transition. J. Clin. Invest. 117 (2), 482–491. doi:10.1172/JCI29544
Venne, A. S., Kollipara, L., and Zahedi, R. P. (2014). The next level of complexity: crosstalk of posttranslational modifications. Proteomics 14 (4-5), 513–524. doi:10.1002/pmic.201300344
Wang, B., Fan, P., Zhao, J., Wu, H., Jin, X., and Wu, H. (2018). FBP1 loss contributes to BET inhibitors resistance by undermining c-Myc expression in pancreatic ductal adenocarcinoma. J. Exp. Clin. cancer Res. 37 (1), 224. doi:10.1186/s13046-018-0888-y
Wang, C., Ivanov, A., Chen, L., Fredericks, W. J., Seto, E., Rauscher, F. J., et al. (2005). MDM2 interaction with nuclear corepressor KAP1 contributes to p53 inactivation. Embo J. 24 (18), 3279–3290. doi:10.1038/sj.emboj.7600791
Wang, R., Fu, Y., Yao, M., Cui, X., Zhao, Y., Lu, X., et al. (2022). The HN1/HMGB1 axis promotes the proliferation and metastasis of hepatocellular carcinoma and attenuates the chemosensitivity to oxaliplatin. FEBS J. 289 (20), 6400–6419. doi:10.1111/febs.16531
Wang, Y., Jiang, J., Li, Q., Ma, H., Xu, Z., and Gao, Y. (2016). KAP1 is overexpressed in hepatocellular carcinoma and its clinical significance. Int. J. Clin. Oncol. 21 (5), 927–933. doi:10.1007/s10147-016-0979-8
Wegert, J., Fischer, A. K., Palhazi, B., Treger, T. D., Hilgers, C., Ziegler, B., et al. (2024). TRIM28 inactivation in epithelial nephroblastoma is frequent and often associated with predisposing TRIM28 germline variants. J. pathology 262 (1), 10–21. doi:10.1002/path.6206
Wei, C., Cheng, J., Zhou, B., Zhu, L., Khan, M. A., He, T., et al. (2016). Tripartite motif containing 28 (TRIM28) promotes breast cancer metastasis by stabilizing TWIST1 protein. Sci. Rep. 6, 29822. doi:10.1038/srep29822
Whiteside, T. L. (2008). The tumor microenvironment and its role in promoting tumor growth. Oncogene 27 (45), 5904–5912. doi:10.1038/onc.2008.271
Wurth, L., Papasaikas, P., Olmeda, D., Bley, N., Calvo, G. T., Guerrero, S., et al. (2016). UNR/CSDE1 drives a post-transcriptional program to promote melanoma invasion and metastasis. Cancer Cell 30 (5), 694–707. doi:10.1016/j.ccell.2016.10.004
Xiao, T. Z., Suh, Y., and Longley, B. J. (2014). MAGE proteins regulate KRAB zinc finger transcription factors and KAP1 E3 ligase activity. Archives Biochem. biophysics 563, 136–144. doi:10.1016/j.abb.2014.07.026
Xie, J., Fu, L., and Jin, L. (2021). Immunotherapy of gastric cancer: past, future perspective and challenges. Pathology, Res. Pract. 218, 153322. doi:10.1016/j.prp.2020.153322
Yang, J., Mani, S. A., Donaher, J. L., Ramaswamy, S., Itzykson, R. A., Come, C., et al. (2004). Twist, a master regulator of morphogenesis, plays an essential role in tumor metastasis. Cell 117 (7), 927–939. doi:10.1016/j.cell.2004.06.006
Yang, J., Wang, C., Zhao, F., Luo, X., Qin, M., Arunachalam, E., et al. (2017). Loss of FBP1 facilitates aggressive features of hepatocellular carcinoma cells through the Warburg effect. Carcinogenesis 38 (2), 134–143. doi:10.1093/carcin/bgw109
Yang, Y., Lu, H., Chen, C., Lyu, Y., Cole, R. N., and Semenza, G. L. (2022). HIF-1 Interacts with TRIM28 and DNA-PK to release paused RNA polymerase II and activate target gene transcription in response to hypoxia. Nat. Commun. 13 (1), 316. doi:10.1038/s41467-021-27944-8
Yang, Y., Zhang, Y., Chen, G., Sun, B., Luo, F., Gao, Y., et al. (2024). KAP1 stabilizes MYCN mRNA and promotes neuroblastoma tumorigenicity by protecting the RNA m(6)A reader YTHDC1 protein degradation. J. Exp. Clin. cancer Res. 43 (1), 141. doi:10.1186/s13046-024-03040-9
Yende, A. S., Williams, E. C., Pletcher, A., Helfand, A., Ibeawuchi, H., North, T. M., et al. (2023). TRIM28 promotes luminal cell plasticity in a mouse model of prostate cancer. Oncogene 42 (17), 1347–1359. doi:10.1038/s41388-023-02655-0
Yokoe, T., Toiyama, Y., Okugawa, Y., Tanaka, K., Ohi, M., Inoue, Y., et al. (2010). KAP1 is associated with peritoneal carcinomatosis in gastric cancer. Ann. Surg. Oncol. 17 (3), 821–828. doi:10.1245/s10434-009-0795-8
Yu, C., Zhan, L., Jiang, J., Pan, Y., Zhang, H., Li, X., et al. (2014). KAP-1 is overexpressed and correlates with increased metastatic ability and tumorigenicity in pancreatic cancer. Med. Oncol. 31 (7), 25. doi:10.1007/s12032-014-0025-5
Zhang, F., Zhu, T., Wu, C., Shen, D., Liu, L., Chen, X., et al. (2023b). TRIM28 recruits E2F1 to regulate CBX8-mediated cell proliferation and tumor metastasis of ovarian cancer. Hum. Cell 36 (6), 2113–2128. doi:10.1007/s13577-023-00983-7
Zhang, F. L., Yang, S. Y., Liao, L., Zhang, T. M., Zhang, Y. L., Hu, S. Y., et al. (2023a). Dynamic SUMOylation of MORC2 orchestrates chromatin remodelling and DNA repair in response to DNA damage and drives chemoresistance in breast cancer. Theranostics 13 (3), 973–990. doi:10.7150/thno.79688
Zhang, J., Bu, X., Wang, H., Zhu, Y., Geng, Y., Nihira, N. T., et al. (2018). Cyclin D-CDK4 kinase destabilizes PD-L1 via cullin 3-SPOP to control cancer immune surveillance. Nature 553 (7686), 91–95. doi:10.1038/nature25015
Zhang, J., Fan, X., Liao, L., Zhu, Y., Wan, X., Rao, H., et al. (2022). TRIM28 attenuates Bortezomib sensitivity of hepatocellular carcinoma cells through enhanced proteasome expression. Clin. Transl. Med. 12 (1), e603. doi:10.1002/ctm2.603
Zhang, P., Li, S., Chen, Z., Lu, Y., and Zhang, H. (2020). LncRNA SNHG8 promotes proliferation and invasion of gastric cancer cells by targeting the miR-491/PDGFRA axis. Hum. Cell 33 (1), 123–130. doi:10.1007/s13577-019-00290-0
Zhang, R. Y., Liu, Z. K., Wei, D., Yong, Y. L., Lin, P., Li, H., et al. (2021b). UBE2S interacting with TRIM28 in the nucleus accelerates cell cycle by ubiquitination of p27 to promote hepatocellular carcinoma development. Signal Transduct. Target. Ther. 6 (1), 64. doi:10.1038/s41392-020-00432-z
Zhang, W., Cai, Z., Kong, M., Wu, A., Hu, Z., Wang, F., et al. (2021a). Prognostic significance of TRIM28 expression in patients with breast carcinoma. Open Med. Wars. Pol. 16 (1), 472–480. doi:10.1515/med-2021-0263
Zhang, Y., and Zeng, L. (2020). Crosstalk between ubiquitination and other post-translational protein modifications in plant immunity. Plant Commun. 1 (4), 100041. doi:10.1016/j.xplc.2020.100041
Zhao, C., Zhao, Y., Zhao, J., Meng, G., Huang, S., Liu, Y., et al. (2022). Acute myeloid leukemia cell-derived extracellular vesicles carrying microRNA-548ac regulate hematopoietic function via the TRIM28/STAT3 pathway. Cancer gene Ther. 29 (7), 918–929. doi:10.1038/s41417-021-00378-6
Zhao, H., Wu, L., Yan, G., Chen, Y., Zhou, M., Wu, Y., et al. (2021). Inflammation and tumor progression: signaling pathways and targeted intervention. Signal Transduct. Target. Ther. 6 (1), 263. doi:10.1038/s41392-021-00658-5
Zhou, Y., Wang, B., Wang, Y., Chen, G., Lian, Q., and Wang, H. (2019). miR-140-3p inhibits breast cancer proliferation and migration by directly regulating the expression of tripartite motif 28. Oncol. Lett. 17 (4), 3835–3841. doi:10.3892/ol.2019.10038
Ziv, Y., Bielopolski, D., Galanty, Y., Lukas, C., Taya, Y., Schultz, D. C., et al. (2006). Chromatin relaxation in response to DNA double-strand breaks is modulated by a novel ATM- and KAP-1 dependent pathway. Nat. Cell Biol. 8 (8), 870–876. doi:10.1038/ncb1446
Keywords: TRIM28, ubiquitination, SUMOylation, transcription inhibitor, tumorigenesis, cancer therapy
Citation: Li K, Wang H, Jiang B and Jin X (2024) TRIM28 in cancer and cancer therapy. Front. Genet. 15:1431564. doi: 10.3389/fgene.2024.1431564
Received: 12 May 2024; Accepted: 01 July 2024;
Published: 19 July 2024.
Edited by:
Yadi Wu, University of Kentucky, United StatesReviewed by:
Donglin Ding, Mayo Clinic, United StatesNaresh Poondla, Icahn School of Medicine at Mount Sinai, United States
Copyright © 2024 Li, Wang, Jiang and Jin. This is an open-access article distributed under the terms of the Creative Commons Attribution License (CC BY). The use, distribution or reproduction in other forums is permitted, provided the original author(s) and the copyright owner(s) are credited and that the original publication in this journal is cited, in accordance with accepted academic practice. No use, distribution or reproduction is permitted which does not comply with these terms.
*Correspondence: Bitao Jiang, jiangbitao@163.com; Xiaofeng Jin, jinxiaofeng@nbu.edu.cn