- 1College of Life Sciences, University of Chinese Academy of Sciences, Beijing, China
- 2Shenzhen Key Lab of Marine Genomics, Guangdong Provincial Key Lab of Molecular Breeding in Marine Economic Animals, BGI Academy of Marine Sciences, BGI Marine, Shenzhen, China
- 3Laboratory of Aquatic Genomics, College of Life Sciences and Oceanography, Shenzhen University, Shenzhen, China
- 4Key Laboratory of Prevention and Control for Aquatic Invasive Alien Species, Ministry of Agriculture and Rural Affairs, Guangdong Modern Recreational Fisheries Engineering Technology Center, Pearl River Fisheries Research Institute, Chinese Academy of Fishery Sciences, Guangzhou, China
Asia arowana (Scleropages formosus) is an ornamental fish with high economic value, while its sex determination mechanism is still poorly understood. By far, no morphological evidence or molecular marker has been developed for effective distinguishment of genders, which poses a critical challenge to our captive breeding efforts. In this study, we sequenced gonadal transcriptomes of adult Asian arowanas and revealed differential expression profiling of sex-related genes. Based on the comparative transcriptomics analysis of testes (n = 3) and ovaries (n = 3), we identified a total of 8,872 differentially expressed genes (DEGs) and 18,490 differentially expressed transposable elements (TEs) between male and female individuals. Interestingly, the expression of TEs usually has been more significantly testis-biased than related coding genes. As expected, several genes related to females (such as foxl2 and cyp19a1a) are significantly transcribed in the ovary, and some genes related to male gonad development (such as dmrt1, gsdf and amh) are highly expressed in the testis. This sexual dimorphism is valuable for ascertaining the differential expression patterns of sex-related genes and enriching the genetic resources of this economically important species. These valuable genetic materials thereby provide instructive references for gender identification and one-to-one breeding practices so as to expand fish numbers for a rapid elevation of economic value.
Introduction
Teleost exhibit remarkable diversity of sex determination mechanisms, including genetic sex determination (GSD; Myosho et al., 2012), environmental sex determination (ESD; Li et al., 2023), and various combinations of these two modes. In the GSD system (such as XX/XY and ZW/ZZ), the expression of sex-determining genes initiates a series of cascadic signaling pathways of sex determination and differentiation, thereby inducing the primordial gonads to develop into ovaries or testes (Li and Gui, 2018). Many master sex-determining genes or sex differentiation-related genes have been reported to play a main role in regulation of sex development in various fishes, such as dmrt1 (doublesex and mab-3 related transcription factor 1), sox9 (SRY-box transcription factor 9), and foxl2 (forkhead box protein L2). Some transcription factors, involved in regulating gene expression, play a crucial role in the development of male or female differentiation (Cocquet et al., 2002; Cui et al., 2017; Hu et al., 2021).
In fish, dmrt1 is the master sex-determining gene in half-smooth tongue sole (Cui et al., 2017), and its homologous gene dmy (the dmrt1 paralogous located in Y-chromosome) was verified to be the sex-determining gene in medaka (Nanda et al., 2002; Kobayashi et al., 2004). Nearly all polypeptide chains encoded by dmrt genes contain a highly conserved zinc finger DNA-binding and transcription regulating motif (known as DM domain), which consists of six conserved cysteines and two histidines for binding into the minor groove of any target DNA. High expression of dmrt1 in males activates genetic programs to promote testicular differentiation. There is only one copy of the dmrt2 gene in mammals, but two copies (dmrt2a and dmrt2b) are commonly identified in teleost fishes. It has been reported that the dmrt2 (a/b) genes are involved in determination of the body axis and segment differentiation of vertebrates and are also related to the gonadal sex differentiation process (Han et al., 2021). In bony fishes, dmrt3 is usually highly expressed in the testis and nervous system, which presumably plays an important role in the differentiation and development of nerve and germ cells as well as testes (Li et al., 2008; Dong et al., 2010). In contrast, in females, high expression of foxl2 inhibits the expression of dmrt1 while upregulates cyp19a1, thereby promoting the development of ovarian tissues (Zhang et al., 2017). The transforming growth factor-β (TGF-β) signaling pathway mainly participates in mediating the formation of tissues and organs as well as reproductive development by regulating cell growth, proliferation, differentiation and other processes (Li and Gui, 2018; Pan et al., 2021). Many previous studies have proved that this signaling pathway is related to sex determination and differentiation in various fishes. Meanwhile, some members of the TGF-β superfamily have been considered as initiators or key regulators of sexual differentiation, such as amh (anti-Müllerian hormone), amhr2 (anti-Müllerian hormone receptor type 2; (Kamiya et al., 2012), and gsdf (gonadal soma-derived factor; (Myosho et al., 2012).
Transposable elements (TEs) have been integral parts of vertebrate genomes throughout the evolution process and play important roles in promoting genetic innovation, sex determination and reproduction (Biémont and Vieira, 2006). Recent bursts of TEs and simple repeat accumulations were observed around young sex determination loci, indicating the strong association between TE amplification and the formation of sex determination regions on sex chromosomes (Chalopin et al., 2015). TEs could carry regulatory elements and modify the expression of neighboring genes, thereby participating in the evolution of germ cells and gonadal regulatory networks. The expression and transposition of TEs are critical for vertical transmission to progeny and persistence in lineages (Dechaud et al., 2019). Interestingly, TEs are particularly prone to being recruited in sexual development, since they are typically expressed in the gonads (Brunet et al., 2018). For instance, in Oryzias latipes approximately 1.2% and 3.5% of ovarian and testicular transcripts were reported to be attributed to TE expression, respectively (Dechaud et al., 2019).
Asian arowana (Scleropages formosus, also known as dragonfish) belongs to the ancient family Osteoglossidae, and it is a freshwater species with a native distribution in Southeast Asia. It has become a well-known ornamental fish for its vibrant colors and cultural significance. Mainly due to habitat destruction and overfishing, arowanas have been classified as endangered species in Appendix I by the Convention on International Trade in Endangered Species of Wild Fauna and Flora (CITES). In recent years, significant progress has been made in the genetics research and breeding techniques of arowanas (Chang, 2009; Mu et al., 2012; Shen et al., 2014; Austin et al., 2015; Bian et al., 2016; Mu et al., 2022).
Asian arowana often reaches sexual maturity at 2–4 years of age (Chang, 2009), and several previous studies proposed that the genetic sex determination system of this species may be ZW/ZZ (Shen et al., 2014; Bian et al., 2016). Because there are no obvious morphological differences during all life stages of the Asian arowana, even after sexual maturity, it is very difficult to phenotypically differentiate the sex of individuals. One recent report showed that its putative ZW system is more likely a polymorphic pattern that occurs in the 18th chromosome pair, including the accumulation of constitutive heterochromatin and 18S rDNA (Toma et al., 2023). Meanwhile, although its genomes of both sexes have been published by us (Mu et al., 2022), our in-depth comparative genomics between males and females has failed to identified any significant region with obvious genomic differences. Therefore, we performed this study to identify coding and non-coding regions that are differentially expressed between ovaries and testes of adult Asian arowanas through transcriptome sequencing, which may become differential signatures between both sexes for practical gender identification and one-to-one captive breeding.
Materials and methods
Sample collection
Three female and three male individuals of Asian arowana, around 5-year-old, were obtained from Pearl River Fisheries Research Institute, Chinese Academy of Fishery Sciences (Guangzhou, Guangdong, China). After dissection, the sex of these fishes was determined based on morphological inspection of the gonads. A total of six gonad tissues, including three ovaries and three testes, were frozen in liquid nitrogen immediately and stored at −80°C until use.
RNA isolation, library construction, and transcriptome sequencing
Total RNAs were extracted from the ovary and testis tissues by using a TRIZOL Kit (Invitrogen, Carlsbad, CA, United States of America) following the manufacturer’s instructions. The extracted RNAs were then digested by DNase I to eliminate genomic DNA’s contamination. Purified RNA integrity and quality were assessed with an Agilent 2,100 Bioanalyzer System (Agilent Technologies, Santa Clara, CA, United States of America). Only those RNA samples with RIN (RNA integrity number) > 7.0 were utilized for library construction. A total of six cDNA libraries with insert sizes of 300–400 bp were generated in DNA nanoballs (DNBs) according to the manufacturer’s protocol of DNBSEQ sequencing platform, and then sequenced on a MGISEQ-2000 platform (MGI, BGI Shenzhen, China) to obtain 150-bp paired-end reads.
Screening and functional analysis of sex-biased differentially expressed genes (DEGs)
Raw reads with low quality, adapter sequences, and/or highly unknown N bases were filtered by using SOAPnuke v1.5.6 with optimized parameters “filter -n 0.01 -L 15 -q 0.4 -G -Q 2” (Chen et al., 2017). The clean RNA reads from six samples were subsequently aligned onto the previously published female genome assembly (Mu et al., 2022) by using STAR v2.5.3 (Dobin et al., 2013), and then RSEM v1.2.8 (Li and Dewey, 2011) was applied to quantify transcription levels of genes and transcripts. The Fragments Per Kilobase of exon model per Million mapped fragments (FPKM) algorithms were used to normalize the mRNA expression levels. Based on the quantitative data, we utilized the DESeq2 R package (Love et al., 2014) to characterize DEGs between ovaries and testes. Adjusted p-value (false discovery rate, FDR) < 0.05 and absolute value of log2 (fold change) > 1.5 were assigned as the stringent threshold for significant DEGs (Stachowiak et al., 2024).
Functional annotations of the DEGs were performed via the NCBI NR database, Gene Ontology (GO) (Ashburner et al., 2000) enrichment analysis and Kyoto Encyclopedia of Genes and Genomes (KEGG) (Kanehisa and Goto, 2000) pathway enrichment analysis. In addition, we compared all genes to the AnimalTFDB2.0 (Zhang et al., 2015) database to obtain transcription factor (TF) families. We subsequently applied DIAMOND (Buchfink et al., 2015) to align the DEGs with the sequences of Asia arowana in the STRING v12.0 database (Szklarczyk et al., 2023), constructed a potential protein-protein interaction (PPI) network among potential sex-related genes, and visualized the results using Cytoscape v3.10.1 (Su et al., 2014).
Quantification of TE expression
To analyze the expression of TEs, we applied TEtranscripts v2.2.3 (Jin et al., 2015) to estimate TE expression at a copy-level resolution by using sorted bam files from STAR. TE quantification was performed using RepeatMasker (Tarailo-Graovac and Chen, 2009) TE annotation. TE transcripts were used for a differential expression analysis between ovary and testis tissues with DESeq2 (Love et al., 2014) to generate a normalized count matrix, variance-stable count matrix, and matrix of differential gene expression. We further classified TE categories into LTRs, LINEs, SINEs, DNA TEs, and unknowns as provided in the published TE annotation of arowana (Mu et al., 2022). The numbers of differentially expressed TEs by sex within each category were reported for ovaries and testes.
To characterize the distributions of sex-biased genes and TEs across the arowana genome, we examined whether sex-biased genes clustered on the genome according to a published pipeline (https://gitlab.com/Corend/gene_clusters_pyth) (Dechaud et al., 2021; Toubiana et al., 2021), which calculated the local average log2FC of transcripts in a sliding window of 1 Mb with a 50-kb step size and applied a bootstrap method to detect regions of significant deviation (p < 0.05). We then counted the TE copies expressed in the testes and ovaries according to 500-kb windows and visualized the distributions of co-expression clusters and sex-biased TEs by RectChr (v1.36; https://github.com/BGI-shenzhen/RectChr).
Identification of dmrt family genes for phylogenetic analysis
We applied two strategies to obtain the protein sequences of dmrt family genes in one mammal (Homo sapiens, Hs), one cartilaginous fish (Amblyraja radiata, Ara), 18 representative teleost fishes, including Danio rerio (Dre), O. latipes (Ola), Gasterosteus aculeatus (Gac), Lepisosteus oculatus (Loc), Albula glossodonta (Agl), Anguilla japonica (Aja), Arapaima gigas (Agi), Clarias batrachus (Cba), Clupea harengus (Cha), Gambusia affinis (Gaf), Heterotis niloticus (Hni), Ictalurus punctatus (Ipu), Mastacembelus armatus (Mar), Megalops atlanticus (Mat), Megalops cyprinoides (Mcy), Oreochromis aureus (Oau), Scophthalmus maximus (Sma) and arowana (Sfo) (Supplementary Table S1).
For those species with public annotations, we downloaded related gene sequences from the NCBI (Supplementary Table S2) or extracted the sequences through BLASTP (Ye et al., 2006) for using as the reference sequences. Then we employed GeneWise (Birney et al., 2004) to predict related protein-coding sequences in the arowana genome using the reference protein sequences, and obtained neighboring genes from the genome annotation or using BLAST with an E-value of 1e-5 against the arowana genome in order to validate the synteny of dmrt genes. We converted coding sequences (CDS) to protein sequences and used MUSCLE v3.8 (Edgar, 2004) to perform global alignments. RaxML (Stamatakis, 2006) was employed to construct a gene-family phylogenetic tree with the PROTGAMMAAUTO model. We also searched the domains of these protein sequences by using NCBI Batch CD-Search and generated visualizations via iTOL (Letunic and Bork, 2021) and IBS 2.0 (Xie et al., 2022).
Results
Summary of the sequencing data and quality analysis
Transcriptome sequencing (RNA-Seq) of the six libraries, constructed in triplicates from ovaries and testes, yielded 32.16 Gb and 29.76 Gb of raw reads, respectively. After data filtering, we obtained 29.43 Gb of clean reads for three ovarian samples and 27.72 Gb of clean reads for three testis samples. In addition, the average percentages of bases with quality values greater than 20 (Q20) and 30 (Q30) in the six samples accounted for 97.51% and 90.45% of the total bases, respectively. The average mapping rates of transcriptome reads were 95.91% per ovary sample and 94.86% per testis sample, independently (Table 1).
Identification and enrichment of DEGs in ovaries and testes
A total of 23,009 expressed genes were detected in our research. Among the expressed genes, a total of 20,715 genes are expressed in both males and females, while 500 and 1,794 genes are specifically expressed in males and females respectively (Figure 1A). In total, 8,872 expressed genes (38.56%) were found to be sex-biased, including 5,153 (22.40%) upregulated DEGs and 3,719 (16.16%) downregulated DEGs in testes compared with ovaries (Figures 1B, C). The remaining 14,137 genes were expressed without significant difference between genders.
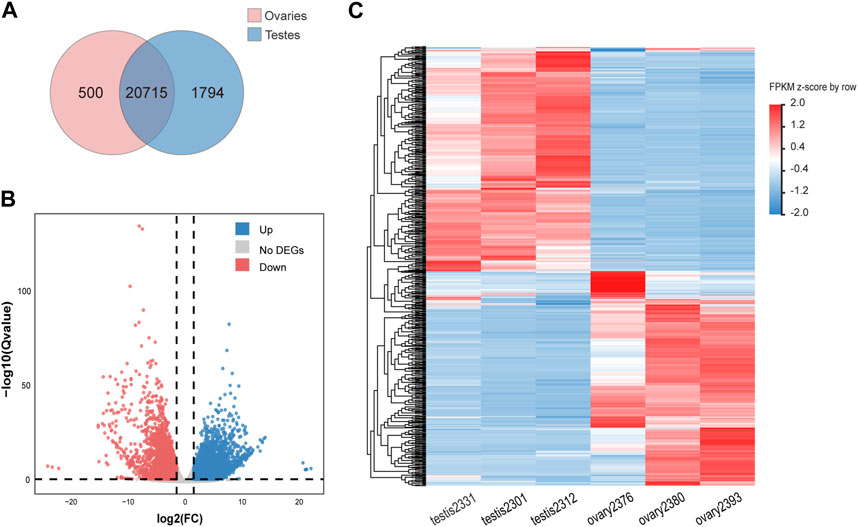
Figure 1. Differentially expressed genes (DEGs) obtained from the RNA-seq data of ovaries and testes. (A) A Venn diagram showing both expressed, testis-specific expressed, and ovarian-specific expressed genes. (B) A volcano plot showing number of up-/down-expressed DEGs in testes versus ovaries. Significantly upregulated (FDR < 0.05) genes in females and males are indicated by red and blue dots respectively, while genes without significant difference between sexes (FDR >0.05) are represented by gray dots. (C) A heatmap depicting hierarchical clustering of DEGs in gonads of Asia arowana. Here we chose the top 500 highest expressed genes in testes and ovaries, respectively; each column represents an individual, and each row represents a gene. The color scale indicates relative expression.
GO functional annotation and KEGG pathway annotation were further performed on the DEGs. A total of 5,651 DEGs (2,612 female-biased genes and 3,039 male-biased genes) were assigned to GO terms (Supplementary Figure S1), and 3,479 DEGs were annotated to KEGG pathways (Supplementary Figure S2). GO enrichment analysis showed that ovary-biased DEGs were enriched in GO terms such as cell cycle (GO:0007049), egg coat (GO:0035805) and single fertilization (GO:0007338) (Figure 2A; Supplementary Table S3), while testis-biased DEGs were enriched in GO terms such as RNA-directed DNA polymerase activity (GO:0003964), motile cilium (GO:0031514) and regulation of cellular process (GO:0050794) (Figure 2B; Supplementary Table S4). Through KEGG pathway enrichment analysis, we observed that upregulated DEGs in ovaries were significantly enriched in cell cycle (ko04110), oocyte meiosis (ko04114), progesterone-mediated oocyte maturation pathways (ko04914) and TGF-β signaling pathway (ko04350) (Figure 2C; Supplementary Table S5), while upregulated DEGs in testes were enriched in some pathways including oxytocin signaling pathway (ko04921), focal adhesion (ko04510), cell adhesion molecules (ko04514), calcium signaling pathway (ko04020) (Figure 2D; Supplementary Table S6).
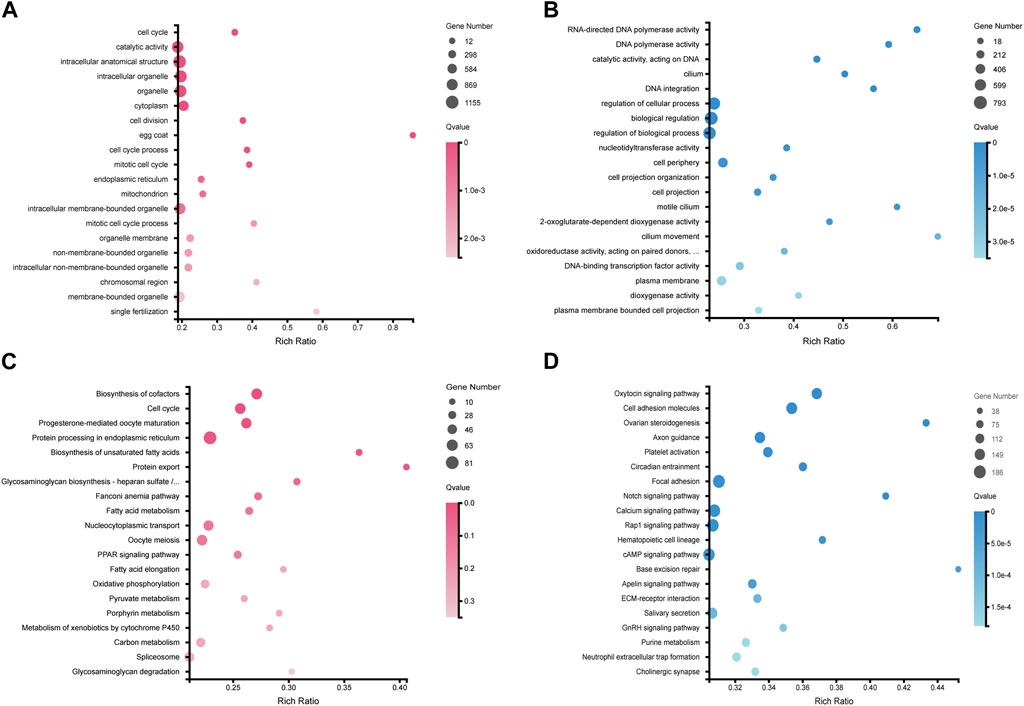
Figure 2. Top20 GO and KEGG pathway enrichment of DEGs in ovaries and testes. (A) GO enrichment of upregulated DEGs in ovaries. (B) GO enrichment of upregulated DEGs in testes. (C) KEGG pathway enrichment of upregulated DEGs in ovaries. (D) KEGG pathway enrichment of upregulated DEGs in testes.
Expression patterns of sex determination and sexual differentiation related genes
Based on the functional annotations results of NCBI NR database as well as KEGG Orthology and enrichment analyses, we performed a further comparative analysis to detect that some well-known candidate sex determination or sexual differentiation-related genes and members of the TGF-β signaling pathway showed significant sexual dimorphism in gonadal expression. Those genes related to testis differentiation as well as sperm maturation and maintenance of teleost, such as dmrt1, dmrtB1, sox9, cyp11a (cholesterol side-chain cleavage enzyme), cyp17a (steroid 17-alpha-hydroxylase/17,20 lyase-like), amh and its receptor amhr2 were detected with high transcription in testes; foxl2, figla (factor in the germline alpha), cyp19a1 (aromatase), and gdf9, related to ovary differentiation, were significantly expressed in ovaries compared with testes (Figure 3A). Several members of wnt family also showed sex-biased in gonads. For example, wnt4a and wnt11 showed a female bias, but wnt5b, wnt6, wnt7b, wnt8b, and wnt10b upregulated in testes; however, wnt4b was expressed without differential difference in these samples (Table 2; Figure 3A).
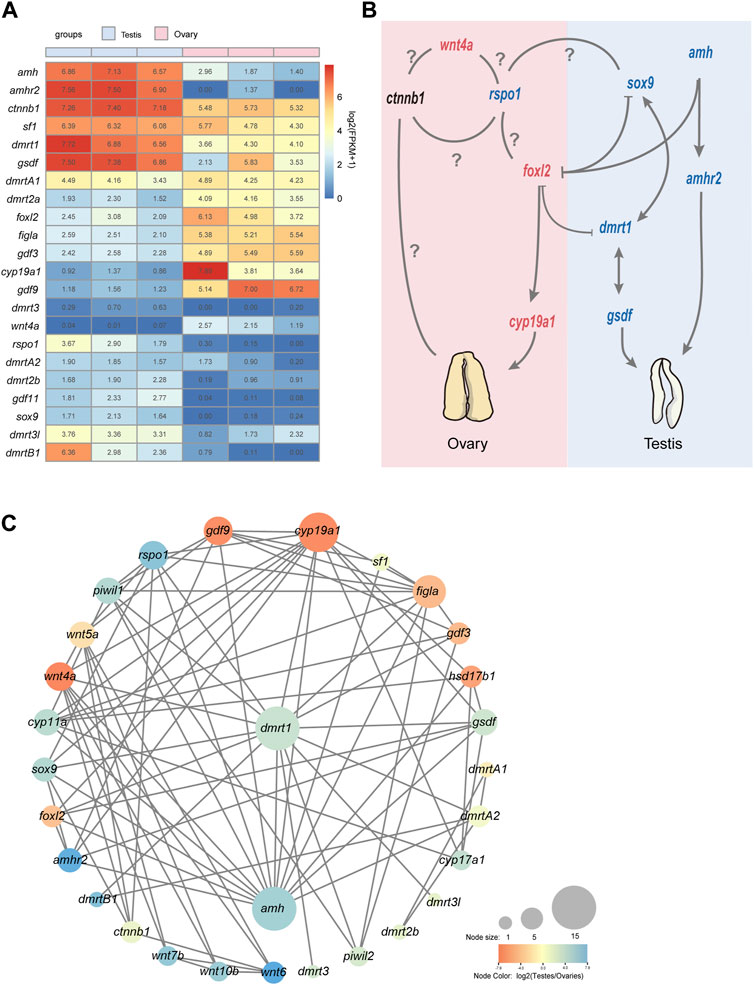
Figure 3. An overview of sex-related genes and a predicted regulatory network in Asia arowana. (A) The expression profiles of sex determination or differentiation-related genes in gonads of Asia arowana. (B) A predicted regulatory network for sex development in Asia arowana. Genes with upregulated expression in females and males are marked in red and blue, respectively. Note that dmrt1 and amh are the key male sex regulators, while foxl2 and cyp19a1a can induce female development. Symbols: ⊥: inhibition; →: stimulation; ?: unclearly. (C) PPI network analysis of sex determination or differentiation-related genes. Each node size reflects the number of interacting proteins, and various colors represent the differential expression profiles.
The STRING database was utilized to construct a PPI network for potential sex-related genes among the DEGs. It was revealed that 31 potential sex-related genes exhibited intricate interaction relationships. Notably, dmrt1 and amh were associated with 15 sex-related genes, cyp19a1 collaborated with 13 genes, and Foxl2 interacted with five genes. Dmrt1 not only interacted closely with testis-upregulated genes (such as amh, sox9, dmrtB1, and rspo1), but also with female sex-related genes (like wnt4a, foxl2, figla, and cyp19a1). Furthermore, foxl2 interacted with its downstream functional gene cyp19a1, as well as with dmrt1, amh, and amhr2 (Figures 3B, C).
Identification of TE copies and families with sex-biased expression
We characterized gonadal expression of TEs in order to analyze TE expression relative to gene expression. Unlike protein-coding genes that have an approximately equal proportions of expression in both sexes, TEs appeared to have a significant testicular bias. In brief, 18,490 TE copies from the transcriptomes were detected with significantly differential expression in the gonads, of which 15,930 (86.15%) were testis-biased, while 2,560 (13.84%) were ovarian biased. We classified these testis- or ovary-biased TEs into five subfamilies by TE annotation subsequently (Figure 4A). Interestingly, we observed that sex-biased expressed genes often clustered in a genome-wide manner. And in some chromosomes, TE copies located near ovary-biased gene regions are still showing testis expression (such as the testis-biased TE is located between the two copies of the ovary-biased rapunzel-like on Chr23; Figure 4B, C-2), which is consistent with the trend that the overall expression of TEs in the genome is more testis-biased (Figure 4A). Nevertheless, we also found some TE copies expressed near female related genes, such as an expressed LTR retrotransposon and an expressed DNA transposon at the upstream of the foxl2 (Figure 4C-1).
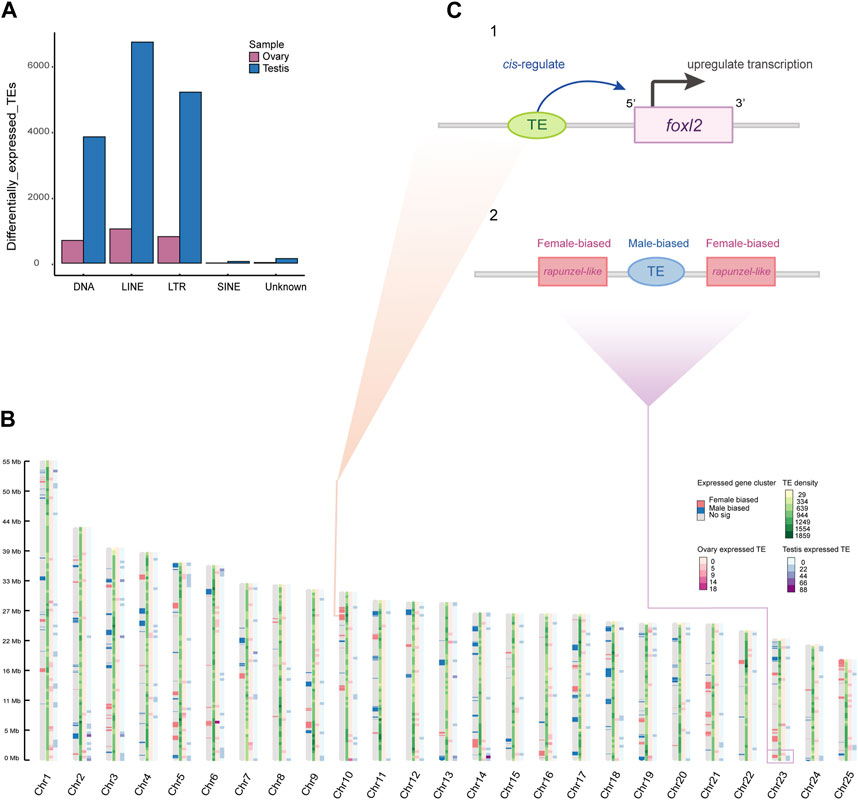
Figure 4. Sex-biased expressed TEs and gene expression clusters. (A) A bar plot of differentially expressed TEs in ovaries and testes (categorized by TE family). (B) Genome-wide sex-biased gene clusters and TEs density across sliding windows. The color pattern on each chromosome represents gene expression cluster, TE density, ovary-biased expressed TE density, and testis-biased expressed TE density, respectively. (C) The predicted regulative pattern of expressed TE at upstream of the foxl2 (1). The testis-biased TE located in neighborhood of ovary-biased expressed genes (2).
Summary of dmrt family genes and related phylogenetic tree
Since multiple copies of dmrt family genes were detected by the transcriptome sequencing, we scanned the dmrt gene family in many representative genomes of arowana and some other bony fishes. A total of nine members from the dmrt gene family were identified in arowana (Figure 5A) and named based on the nomenclature of teleost dmrt genes. All of these identified DMRT proteins contain a conserved DM (Doublesex and Mab-3) domain (near the N-terminus in Figure 5B). Meanwhile, Dmrt1 has an additional DMRT1 domain, as well as Dmrt3, Dmrt3L, DmrtA1 and DmrtA2 own a special DAM domain (middle in Figure 5B). Furthermore, we observed a somehow conserved dmrt1-dmrt3-dmrt2 cluster in the examined 20 species (see the middle panel in Figure 5C), while those species of Osteoglossus and Elopomorpha have another dmrt2-dmrt3-dmrt1 gene cluster (see the left panel in Figure 5C). Based on the neighboring genes of both gene clusters, we infer that the gene clusters of these two groups are dmrt1-dmrt3l-dmrt2l and dmrt3-dmrt2a, respectively (Figure 5C).
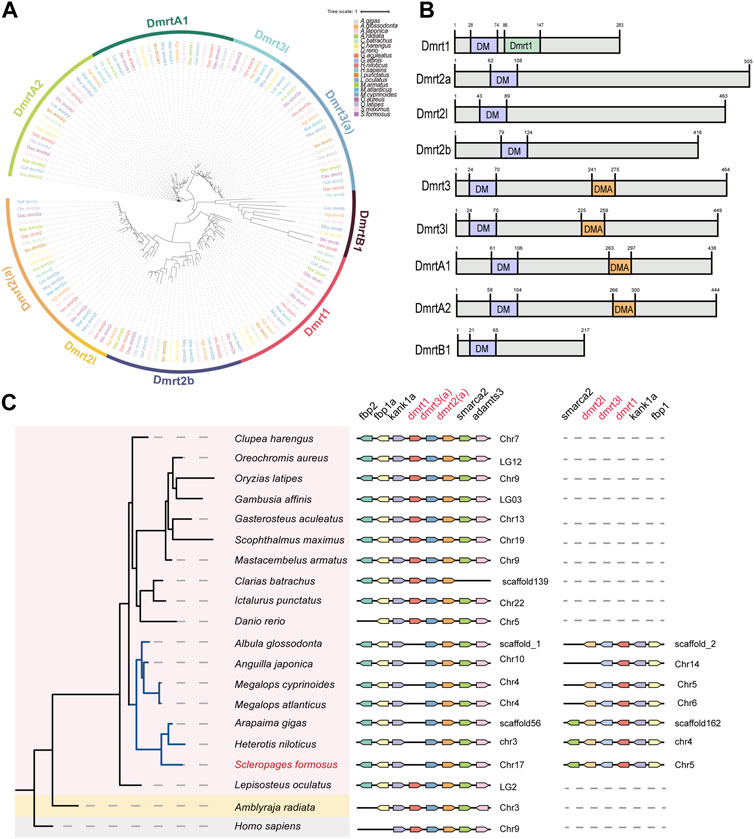
Figure 5. The phylogeny of vertebrate dmrt gene family and a synteny analysis of the conserved dmrt1-dmrt3-dmrt2 gene clusters. (A) A phylogenetic tree of the dmrt gene family in 20 representative species (constructed by the maximum likelihood method). (B) A schematic diagram of conserved domain structures in various DMRT proteins. (C) The synteny of dmrt1-dmrt3-dmrt2 gene clusters.
The expression patterns of dmrt genes were investigated among testes and ovaries. Obviously, the transcription levels of dmrt1 and dmrtB1 in the testes were significantly higher than those in ovaries, dmrt3l was upregulated in the testes, while dmrt2l was not detectable in transcription (Figure 3A). Surprisingly, in the dmrt3-dmrt2a gene cluster (middle panel of Figure 5C), the dmrt3 is slightly expressed in the testis but undetectable in the ovary, while dmrt2a is highly transcribed in the ovary (Figure 3A).
Discussion
Transcriptomics is an effective method to obtain gene regulatory networks among different individuals. In this study, we employed the RNA-Seq technology for transcriptomics analysis, and many genes related to gonad development were identified and characterized to improve our understanding of their sex-related functions from a molecular perspective. Generally speaking, the fish sex determination mechanism usually involves complex biological processes, including a series of genes that promote or maintain the development of gonads into testes or ovaries. The sexual dimorphism in expression identified in this study, especially for those previously reported candidate genes that are related to sex determination and sexual differentiation (Kitano et al., 2023), may be effective indicators for sex prediction in Asian arowana.
The Wnt signaling is a crucial pathway to regulate cell proliferation, differentiation, embryonic development, and folliculogenesis. Recent genetic research has identified the wnt4 as a vital regulator of the Wnt/β-catenin signaling pathway during sex determination (Kossack et al., 2018; Farhadi et al., 2021). The most important member wnt4 gene has been identified in zebrafish (Kossack et al., 2018), medaka (LI et al., 2012) and various other teleost fishes. Due to the teleost-specific whole-genome duplication (WGD) events, there are two paralogs in most teleost, wnt4a and wnt4b, both of which play essential roles in the development of female gonads, ovarian growth, early male development, and sex transition (Hu et al., 2014). We found that several wnt genes showed sex-biased expression patterns in arowana. For example, the expression of wnt4a (log2FC = −7.26) and wnt11 (log2FC = −5.46) that are related to ovary differentiation were upregulated in ovaries; the expression of wnt6 (log2FC = 7.95), which could initiate Wnt/β-catenin signaling to control the proliferation of undifferentiated spermatogonia in mouse (Takase and Nusse, 2016), was also testis-biased. rspo1 showed a sexually dimorphic expression pattern with significantly higher expression in testes over ovaries in our present study (log2FC = 5.41). It is a potential female-determining gene in mammals that can regulate the important Wnt/β-catenin signaling pathway; loss of rspo1 can lead to female sex reversal. However, the expression pattern of rspo1 in fish seems not very conservative. For instance, in many teleost fishes such as zebrafish, medaka, and half-smooth tongue, rspo1 shows a female bias, while in some ancient fishes (like Acipenser stephensi, coelacanth, and lungfish) and some sex-changed teleost fishes (like Notolabrus celidotus) shows a male bias(Biscotti et al., 2018; Zhang et al., 2020; Muncaster et al., 2023), implying that rspo1 may have other gender-related functions in various fishes.
The TGF-β signaling pathway is a large family comprising many members responsible for regulation of various processes including tissue and organ formation, reproductive development, cell growth, proliferation, and differentiation (Pan et al., 2021). In fish, this critical pathway often plays a key role in regulating important physiological processes, such as embryonic development, tissue regeneration, immune regulation, chondrogenesis, as well as sex determination and sexual differentiation (Pan et al., 2021; Kitano et al., 2023). Some members belonged to the TGF-β superfamily, such as amh, amhr2, and gsdf were highly expressed in males (Figure 3A), which indicates that these pathways should play a significant role in sexual differentiation of Asian arowana. During ovarian development, the interaction among granulosa cells, theca cells, and oocytes is crucial for follicular development, steroidogenesis, and female oogenesis (Richards, 2018). The forkhead-box L2 (foxl2) transcription factor is one of the earliest known markers of granulosa cell differentiation (Cocquet et al., 2002), and it has been determined to be a major gene of sex determination and maintenance in various teleost (Cui et al., 2017; Zhang et al., 2017; Yuan et al., 2021). In addition, the foxl2 can directly bind to the promoter region of the aromatase gene cyp19a1a to promote its expression, or indirectly regulated expression of cyp19a1a through interaction with sf1 (splicing factor 1), resulting in estrogen (E2) production to promote ovarian differentiation (Wang et al., 2007; Zhang et al., 2017; Yuan et al., 2021). In our present study, folx2 (log2FC = −3.67) and cyp19a1 (log2FC = −6.97) were found to be highly upregulated in the ovary compared with the testis (Figure 3A), showing a similar expression pattern to other fish species (Zhang et al., 2019; Mustapha et al., 2022). Through a PPI analysis, we found that most of the potential sex-related genes among the DEGs exhibit interaction relationships, suggesting that these genes may play functional roles in sex differentiation and development, potentially being directly or indirectly involved in gonadal development or differentiation. Notably, dmrt1, amh, as well as cyp19a1 (and its upstream regulator foxl2) interact with multiple detected potential sex-related genes, indicating that these gene play central regulatory roles in sex differentiation in Asia arowana. In mammals, Dmrt1 directly represses Foxl2 expression in the testis, and Foxl2 is required for repressing Dmrt1 expression in the ovary (Matson et al., 2011). Previous studies on tilapia and zebrafish found that dmrt1 has an antagonistic effect on the expression of foxl2 and cyp19a1 (Wang et al., 2010). Dmrt1 can directly repress the expression of foxl2 and cyp19a1 in somatic cells of tilapia, while in females foxl2 activates the expression of cyp19a1 and inhibits expression of dmrt1. In addition, studies have found that foxl2 has ovary-enriched expression in zebrafish, just like in mammals, and dmrt1 is required for its downregulation. Therefore, it is speculated that the antagonistic relationship between foxl2 and dmrt1 in the sex determination process may be conserved among vertebrates (Webster et al., 2017). We have observed that dmrt1 not only interacts with male-biased gene, such as amh and sox9, but also engages with cyp19a1 and foxl2, suggesting that the antagonism may also exist in Asian arowana. However, due to limited research on Asian arowana, some protein-protein interactions may require further experimental validation (like Dmrt2a). According to the sex-biased DEGs detected in our present study and the sex relevant pathways in amphioxus and vertebrates (Pan et al., 2021; Curzon et al., 2023; Huang et al., 2023), we infer that wnt4a, foxl2 and cyp19a1 may play the major role in promoting ovarian differentiation of Asia arowana, while the expression and regulation of sox9, dmrt1, gsdf, amh and amhr2 possibly play an important role in development and differentiation of testis. Based on this inference, a potential regulatory network of Asian arowana sexual development was proposed (Figure 3B).
It is well known that TEs could regulate gene expression (Feschotte, 2008; Sundaram and Wysocka, 2020; Gebrie, 2023), and previous studies reported that the closer TE copy to the target gene, the higher correlation of their expressions (Dechaud et al., 2021). In mammalian genomes, TEs are an important source of various cis-regulatory sequences; 20% of the cis-regulatory elements (CREs) in the human genome may have been taken from TEs (Sundaram and Wysocka, 2020), and TEs often contribute to zebrafish cis-regulatory elements, tissue-specific expression and alternative promoters (Lee et al., 2022). Researchers have found that the fourth exon (Ex4) of the sex-determining gene dm-W in the African clawed frog (Xenopus laevis) originated from a non-coding fragment of the hAT-10 family of DNA transposons (Hayashi et al., 2022). An experimental evidence from sablefish (Anoplopoma fimbria) demonstrated that a TE insertion in the promoter region of gsdfY produced allelic diversification by bringing a cis-regulatory module, leading to transcriptional reprofiling and generating a new sex-determined gene for this species (Herpin et al., 2021). The regulatory elements of many genes contain TE sequences, which are involved in the regulation of gene expression. Some studies have reported that TEs can be expressed in multiple tissues (Brunet et al., 2018; Chang et al., 2022; Xu et al., 2023). However, because they align to multiple genomic loci, many RNA-seq reads derived from TEs are often discarded before data analysis. To resolve this problem, several computational tools have been developed (Jin et al., 2015; Jeong et al., 2017; Yang et al., 2019; Lanciano and Cristofari, 2020). We therefore observed a DNA transposon and an LTR transposon located at the promoter region of foxl2 expressed in the arowana ovary (Figure 4C), suggesting co-regulation of the cis-regulatory elements to enhance the neighboring sex-biased gene(s) and/or sex-biased TEs themselves on these two types of sequences, as well as the foxl2 may play a significant functional role in ovarian differentiation of arowana.
Dmrt1, an important member of the dmrt gene family, is essential for maintaining male-specific germ cells and testis differentiation (Matson and Zarkower, 2012; Cui et al., 2017). It not only participates in sex regulation in mammals, but also acts as a ubiquitous conserved sex regulation factor in other non-mammalian vertebrates including fishes. A recently published paper reported no orthologous gene of dmrt1 in amphioxus (Huang et al., 2023), while previous studies on the dmrt family of diverse animals found that dmrt1 and dmrtB1 are vertebrate-specific genes, and they may have arisen in vertebrates through WGD events (Mawaribuchi et al., 2019). Various studies on non-mammalian vertebrates have proved that dmrt1 or its paralogous genes control gonadal sex determination and differentiation with different mechanisms (Smith et al., 2009; Yoshimoto et al., 2010; Cui et al., 2017; Mawaribuchi et al., 2019). From the TF annotation of arowana transcriptomes, we identified multiple copies of dmrt, such as dmrt3 and dmrt2a. Through gene annotation and genome scanning of one elasmobranch and 18 bony fishes, we observed the conserved dmrt1-dmrt3-dmrt2 gene cluster among various vertebrates, and some species of the order Osteoglossus and Elopomorpha also have another dmrt3-dmrt2 gene cluster (Figure 5C). Previous studies have shown that dmrt1 may have emerged after a WGD event in vertebrates, and based on our findings we speculate that the dmrt gene clusters in these two basal teleost species are likely to come from the teleost-specific WGD event (3R-WGD).
It seems that the expanded dmrt gene cluster remains in this clade for continuous function after the 3R-WGD. This confirms that the two groups are sister branch to each other, and this branch forms as sister groups to all the other teleost (Parey et al., 2023). The gene cluster identified in the Asian arowana was named as dmrt1-dmrt3l-dmrt2l, based on the names of related genes published in the NCBI, and another cluster is named as dmrt3-dmrt2a (Figure 5C). Interestingly, dmrt1-dmrt3l in the dmrt1-dmrt3l-dmrt2l gene cluster was upregulated in the arowana testis (log2FC = 2.50, log2FC = 1.06), while dmrt2l was undetectable in both testes and ovaries; in the dmrt3-dmrt2a gene cluster, the dmrt3 gene is slightly expressed in the testes but without expression in the ovary, while dmrt2a is highly expressed in the ovary (log2FC = −3.39) (Figure 3A). These expression trends are very similar to those in other teleost fishes, indicating that dmrt1 and dmrt2 located in different clusters may be critical players in the sexual differentiation of Asian arowana: one of the gene clusters may be associated with male development, while another is potentially associated with female development.
Conclusion
Based on gonadal transcriptome sequencing and transcriptomic comparisons, we characterized a set of differentially expressed genes and pathways for potential involvement in sex determination or differentiation in Asia arowana. This sexual dimorphism is valuable for ascertaining the differential expression patterns of sex-related genes and enriching the genetic resources of this ornamental fish species. Our transcriptome data on sex-related genes can also promote the exploration of molecular mechanisms of gonadal development and sex determination in Asian arowana, which provides valuable references for practical gender identification and one-to-one breeding programs so as to expand fish number for a rapid elevation of worldwide economic value.
Data availability statement
The datasets presented in this study can be found in online repositories. The names of the repository/repositories and accession number(s) can be found below: NCBI under accession numbers: SRR20631749-SRR20631754.
Ethics statement
The animal study was approved by the Animal Care and Use Committee of BGI. The study was conducted in accordance with the local legislation and institutional requirements.
Author contributions
CZ: Writing–original draft, Writing–review and editing, Formal Analysis, Methodology. CB: Writing–original draft, Writing–review and editing, Conceptualization, Methodology. XM: Investigation, Resources, Writing–review and editing, Conceptualization, Supervision. XZ: Methodology, Writing–review and editing. QS: Conceptualization, Writing–original draft, Writing–review and editing, Supervision.
Funding
The author(s) declare that financial support was received for the research, authorship, and/or publication of this article. This work was supported by National Key Research and Development Program of China (no. 2022YFE0139700).
Conflict of interest
The authors declare that the research was conducted in the absence of any commercial or financial relationships that could be construed as a potential conflict of interest.
Publisher’s note
All claims expressed in this article are solely those of the authors and do not necessarily represent those of their affiliated organizations, or those of the publisher, the editors and the reviewers. Any product that may be evaluated in this article, or claim that may be made by its manufacturer, is not guaranteed or endorsed by the publisher.
Supplementary material
The Supplementary Material for this article can be found online at: https://www.frontiersin.org/articles/10.3389/fgene.2024.1381832/full#supplementary-material
References
Ashburner, M., Ball, C. A., Blake, J. A., Botstein, D., Butler, H., Cherry, J. M., et al. (2000). Gene ontology: tool for the unification of biology. The Gene Ontology Consortium. Nat. Genet. 25 (1), 25–29. doi:10.1038/75556
Austin, C. M., Tan, M. H., Croft, L. J., Hammer, M. P., and Gan, H. M. (2015). Whole genome sequencing of the Asian arowana (Scleropages formosus) provides insights into the evolution of ray-finned fishes. Genome Biol. Evol. 7 (10), 2885–2895. doi:10.1093/gbe/evv186
Bian, C., Hu, Y., Ravi, V., Kuznetsova, I. S., Shen, X., Mu, X., et al. (2016). The Asian arowana (Scleropages formosus) genome provides new insights into the evolution of an early lineage of teleosts. Sci. Rep. 6 (1), 24501. doi:10.1038/srep24501
Biémont, C., and Vieira, C. (2006). Genetics: junk DNA as an evolutionary force. Nature 443 (7111), 521–524. doi:10.1038/443521a
Birney, E., Clamp, M., and Durbin, R. (2004). GeneWise and genomewise. Genome Res. 14 (5), 988–995. doi:10.1101/gr.1865504
Biscotti, M. A., Adolfi, M. C., Barucca, M., Forconi, M., Pallavicini, A., Gerdol, M., et al. (2018). A comparative view on sex differentiation and gametogenesis genes in lungfish and coelacanths. Genome Biol. Evol. 10 (6), 1430–1444. doi:10.1093/gbe/evy101
Brunet, F., Roche, A., Chalopin, D., Naville, M., Klopp, C., Vizziano-Cantonnet, D., et al. (2018). Analysis of transposable elements expressed in the gonads of the siberian sturgeon. Sib. Sturgeon (Acipenser baerii, Brandt, 1869) Volume 1-Biology, 115–130.
Buchfink, B., Xie, C., and Huson, D. H. (2015). Fast and sensitive protein alignment using DIAMOND. Nat. Methods 12 (1), 59–60. doi:10.1038/nmeth.3176
Chalopin, D., Volff, J.-N., Galiana, D., Anderson, J. L., and Schartl, M. (2015). Transposable elements and early evolution of sex chromosomes in fish. Chromosome Res. 23, 545–560. doi:10.1007/s10577-015-9490-8
Chang, K. (2009). “Molecular analysis of the breeding biology of the Asian arowana (Scleropages formosus),” (Singapore: National University of Singapore). [PhD thesis].
Chang, N.-C., Rovira, Q., Wells, J., Feschotte, C., and Vaquerizas, J. M. (2022). Zebrafish transposable elements show extensive diversification in age, genomic distribution, and developmental expression. Genome Res. 32 (7), 1408–1423. doi:10.1101/gr.275655.121
Chen, Y., Chen, Y., Shi, C., Huang, Z., Zhang, Y., Li, S., et al. (2017). SOAPnuke: a MapReduce acceleration-supported software for integrated quality control and preprocessing of high-throughput sequencing data. GigaScience 7 (1), 1–6. doi:10.1093/gigascience/gix120
Cocquet, J., Pailhoux, E., Jaubert, F., Servel, N., Xia, X., Pannetier, M., et al. (2002). Evolution and expression of FOXL2. J. Med. Genet. 39 (12), 916–921. doi:10.1136/jmg.39.12.916
Cui, Z., Liu, Y., Wang, W., Wang, Q., Zhang, N., Lin, F., et al. (2017). Genome editing reveals dmrt1 as an essential male sex-determining gene in Chinese tongue sole (Cynoglossus semilaevis). Sci. Rep. 7 (1), 42213. doi:10.1038/srep42213
Curzon, A. Y., Shirak, A., Ron, M., and Seroussi, E. (2023). Master-key regulators of sex determination in fish and other vertebrates—a review. Int. J. Mol. Sci. 24 (3), 2468. [Online]. doi:10.3390/ijms24032468
Dechaud, C., Miyake, S., Martinez-Bengochea, A., Schartl, M., Volff, J.-N., and Naville, M. (2021). Clustering of sex-biased genes and transposable elements in the genome of the medaka fish Oryzias latipes. Genome Biol. Evol. 13 (11), evab230. doi:10.1093/gbe/evab230
Dechaud, C., Volff, J.-N., Schartl, M., and Naville, M. (2019). Sex and the TEs: transposable elements in sexual development and function in animals. Mob. DNA 10, 42–15. doi:10.1186/s13100-019-0185-0
Dobin, A., Davis, C. A., Schlesinger, F., Drenkow, J., Zaleski, C., Jha, S., et al. (2013). STAR: ultrafast universal RNA-seq aligner. Bioinformatics 29 (1), 15–21. doi:10.1093/bioinformatics/bts635
Dong, X., Chen, S., and Ji, X. (2010). Molecular cloning and expression analysis of Dmrt3 gene in half-smooth tongue sole (Cynoglossus semilaevis). J. Fish. China 34 (6), 649–655.
Edgar, R. C. (2004). MUSCLE: multiple sequence alignment with high accuracy and high throughput. Nucleic Acids Res. 32 (5), 1792–1797. doi:10.1093/nar/gkh340
Farhadi, A., Fang, S., Zhang, Y., Cui, W., Fang, H., Ikhwanuddin, M., et al. (2021). The significant sex-biased expression pattern of Sp-Wnt4 provides novel insights into the ovarian development of mud crab (Scylla Paramamosain). Int. J. Biol. Macromol. 183, 490–501. doi:10.1016/j.ijbiomac.2021.04.186
Feschotte, C. (2008). Transposable elements and the evolution of regulatory networks. Nat. Rev. Genet. 9 (5), 397–405. doi:10.1038/nrg2337
Gebrie, A. (2023). Transposable elements as essential elements in the control of gene expression. Mob. DNA 14 (1), 9. doi:10.1186/s13100-023-00297-3
Han, C., Wang, C., Ouyang, H., Zhu, Q., Huang, J., Han, L., et al. (2021). Characterization of dmrts and their potential role in gonadal development of Mandarin fish (Siniperca chuatsi). Aquacult. Rep. 21, 100802. doi:10.1016/j.aqrep.2021.100802
Hayashi, S., Suda, K., Fujimura, F., Fujikawa, M., Tamura, K., Tsukamoto, D., et al. (2022). Neofunctionalization of a noncoding portion of a DNA transposon in the coding region of the chimerical sex-determining gene dm-W in Xenopus frogs. Mol. Biol. Evol. 39 (7), msac138. doi:10.1093/molbev/msac138
Herpin, A., Schartl, M., Depincé, A., Guiguen, Y., Bobe, J., Hua-Van, A., et al. (2021). Allelic diversification after transposable element exaptation promoted gsdf as the master sex determining gene of sablefish. Genome Res. 31 (8), 1366–1380. doi:10.1101/gr.274266.120
Hu, Q., Zhu, Y., Liu, Y., Wang, N., and Chen, S. (2014). Cloning and characterization of wnt4a gene and evidence for positive selection in half-smooth tongue sole (Cynoglossus semilaevis). Sci. Rep. 4 (1), 7167. doi:10.1038/srep07167
Hu, Y., Wang, B., and Du, H. (2021). A review on sox genes in fish. Rev. Aquacult. 13 (4), 1986–2003. doi:10.1111/raq.12554
Huang, Z., Xu, L., Cai, C., Zhou, Y., Liu, J., Xu, Z., et al. (2023). Three amphioxus reference genomes reveal gene and chromosome evolution of chordates. Proc. Natl. Acad. Sci. 120 (10), e2201504120. doi:10.1073/pnas.2201504120
Jeong, H.-H., Yalamanchili, H. K., Guo, C., Shulman, J. M., and Liu, Z. (2017). “An ultra-fast and scalable quantification pipeline for transposable elements from next generation sequencing data,” in Biocomputing 2018 (Singapore: World Scientific), 168–179.
Jin, Y., Tam, O. H., Paniagua, E., and Hammell, M. (2015). TEtranscripts: a package for including transposable elements in differential expression analysis of RNA-seq datasets. Bioinformatics 31 (22), 3593–3599. doi:10.1093/bioinformatics/btv422
Kamiya, T., Kai, W., Tasumi, S., Oka, A., Matsunaga, T., Mizuno, N., et al. (2012). A trans-species missense SNP in Amhr2 is associated with sex determination in the tiger pufferfish, Takifugu rubripes (fugu). PLos Genet. 8 (7), e1002798. doi:10.1371/journal.pgen.1002798
Kanehisa, M., and Goto, S. (2000). KEGG: kyoto encyclopedia of genes and genomes. Nucleic Acids Res. 28 (1), 27–30. doi:10.1093/nar/28.1.27
Kitano, J., Ansai, S., Takehana, Y., and Yamamoto, Y. (2023). Diversity and convergence of sex determination mechanisms in teleost fish. Annu. Rev. Anim. Biosci. 12, 233–259. doi:10.1146/annurev-animal-021122-113935
Kobayashi, T., Matsuda, M., Kajiura-Kobayashi, H., Suzuki, A., Saito, N., Nakamoto, M., et al. (2004). Two DM domain genes, DMY and DMRT1, involved in testicular differentiation and development in the medaka, Oryzias latipes. Dev. Dyn. 231 (3), 518–526. doi:10.1002/dvdy.20158
Kossack, M. E., High, S. K., Hopton, R. E., Yan, Y.-l., Postlethwait, J. H., and Draper, B. W. (2018). Female sex development and reproductive duct formation depend on Wnt4a in zebrafish. Genetics 211 (1), 219–233. doi:10.1534/genetics.118.301620
Lanciano, S., and Cristofari, G. (2020). Measuring and interpreting transposable element expression. Nat. Rev. Genet. 21 (12), 721–736. doi:10.1038/s41576-020-0251-y
Lee, H. J., Hou, Y., Maeng, J. H., Shah, N. M., Chen, Y., Lawson, H. A., et al. (2022). Epigenomic analysis reveals prevalent contribution of transposable elements to cis-regulatory elements, tissue-specific expression, and alternative promoters in zebrafish. Genome Res. 32 (7), 1424–1436. doi:10.1101/gr.276052.121
Letunic, I., and Bork, P. (2021). Interactive Tree of Life (iTOL) v5: an online tool for phylogenetic tree display and annotation. Nucleic Acids Res. 49 (W1), W293–W296. doi:10.1093/nar/gkab301
Li, B., and Dewey, C. N. (2011). RSEM: accurate transcript quantification from RNA-Seq data with or without a reference genome. BMC Bioinf 12, 323–416. doi:10.1186/1471-2105-12-323
Li, J.-Z., Liu, Q., Wang, D.-S., Zhou, L.-Y., Sakai, F., and Nagahama, Y. (2012). Molecular cloning and identification of two Wnt4 genes from the medaka (Oryzias latipes). Acta Hydrobiol. Sin. 36 (5), 983–986. doi:10.3724/SP.J.1035.2012.00983
Li, Q., Zhou, X., Guo, Y., Shang, X., Chen, H., Lu, H., et al. (2008). Nuclear localization, DNA binding and restricted expression in neural and germ cells of zebrafish Dmrt3. Biol. Cell 100 (8), 453–463. doi:10.1042/BC20070114
Li, S., Li, W., Jiang, S., Jing, Y., Xiao, L., Yu, Y., et al. (2023). Mechanisms of sex differentiation and sex reversal in hermaphrodite fish as revealed by the Epinephelus coioides genome. Mol. Ecol. Resour. 23 (4), 920–932. doi:10.1111/1755-0998.13753
Li, X.-Y., and Gui, J.-F. (2018). Diverse and variable sex determination mechanisms in vertebrates. Sci. China Life Sci. 61, 1503–1514. doi:10.1007/s11427-018-9415-7
Love, M. I., Huber, W., and Anders, S. (2014). Moderated estimation of fold change and dispersion for RNA-seq data with DESeq2. Genome Biol. 15 (12), 550–621. doi:10.1186/s13059-014-0550-8
Matson, C. K., Murphy, M. W., Sarver, A. L., Griswold, M. D., Bardwell, V. J., and Zarkower, D. (2011). DMRT1 prevents female reprogramming in the postnatal mammalian testis. Nature 476 (7358), 101–104. doi:10.1038/nature10239
Matson, C. K., and Zarkower, D. (2012). Sex and the singular DM domain: insights into sexual regulation, evolution and plasticity. Nat. Rev. Genet. 13 (3), 163–174. doi:10.1038/nrg3161
Mawaribuchi, S., Ito, Y., and Ito, M. (2019). Independent evolution for sex determination and differentiation in the DMRT family in animals. Biol. Open 8 (8), bio041962. doi:10.1242/bio.041962
Mu, X., Liu, Y., Liu, C., Zhao, C., Li, R., You, X., et al. (2022). Identification of candidate sex-specific genomic regions in male and female Asian arowana genomes. GigaScience 11, giac085. doi:10.1093/gigascience/giac085
Mu, X., Wang, X., Song, H., Yang, Y., Luo, D., Gu, D., et al. (2012). Mitochondrial DNA as effective molecular markers for the genetic variation and phylogeny of the family Osteoglossidae. Gene 511 (2), 320–325. doi:10.1016/j.gene.2012.09.087
Muncaster, S., Goikoetxea, A., Lokman, P. M., De Farias e Moraes, C. E., Damsteegt, E. L., Edgecombe, J., et al. (2023). Genes involved in sex differentiation, epigenetic reprogramming, and cell fate regulate sex change in a wrasse. Rev. Fish. Biol. Fish. 33 (1), 281–294. doi:10.1007/s11160-022-09755-2
Mustapha, U. F., Peng, Y.-X., Huang, Y.-Q., Assan, D., Zhi, F., Shi, G., et al. (2022). Comparative transcriptome analysis of the differentiating gonads in Scatophagus argus. Front. Mar. Sci. 9, 962534. doi:10.3389/fmars.2022.962534
Myosho, T., Otake, H., Masuyama, H., Matsuda, M., Kuroki, Y., Fujiyama, A., et al. (2012). Tracing the emergence of a novel sex-determining gene in medaka, Oryzias luzonensis. Genetics 191 (1), 163–170. doi:10.1534/genetics.111.137497
Nanda, I., Kondo, M., Hornung, U., Asakawa, S., Winkler, C., Shimizu, A., et al. (2002). A duplicated copy of DMRT1 in the sex-determining region of the Y chromosome of the medaka, Oryzias latipes. Proc. Natl. Acad. Sci. 99(18), 11778–11783. doi:10.1073/pnas.182314699
Pan, Q., Kay, T., Depincé, A., Adolfi, M., Schartl, M., Guiguen, Y., et al. (2021). Evolution of master sex determiners: TGF-β signalling pathways at regulatory crossroads. Phil. Trans. R. Soc. B 376 (1832), 20200091. doi:10.1098/rstb.2020.0091
Parey, E., Louis, A., Montfort, J., Bouchez, O., Roques, C., Iampietro, C., et al. (2023). Genome structures resolve the early diversification of teleost fishes. Science 379(6632), 572–575. doi:10.1126/science.abq4257
Richards, J. S. (2018). “Chapter one - the ovarian cycle,” in Vitamins and hormones. Editor G. Litwack (United States: Academic Press), 1–25.
Shen, X., Kwan, H., Thevasagayam, N., Prakki, S., Kuznetsova, I., Ngoh, S., et al. (2014). The first transcriptome and genetic linkage map for Asian arowana. Mol. Ecol. Resour. 14 (3), 622–635. doi:10.1111/1755-0998.12212
Smith, C. A., Roeszler, K. N., Ohnesorg, T., Cummins, D. M., Farlie, P. G., Doran, T. J., et al. (2009). The avian Z-linked gene DMRT1 is required for male sex determination in the chicken. Nature 461 (7261), 267–271. doi:10.1038/nature08298
Stachowiak, M., Nowacka-Woszuk, J., Szabelska-Beresewicz, A., Zyprych-Walczak, J., Krzeminska, P., Sosinski, O., et al. (2024). A massive alteration of gene expression in undescended testicles of dogs and the association of KAT6A variants with cryptorchidism. Proc. Natl. Acad. Sci. 121 (7), e2312724121. doi:10.1073/pnas.2312724121
Stamatakis, A. (2006). RAxML-VI-HPC: maximum likelihood-based phylogenetic analyses with thousands of taxa and mixed models. Bioinformatics 22 (21), 2688–2690. doi:10.1093/bioinformatics/btl446
Su, G., Morris, J. H., Demchak, B., and Bader, G. D. (2014). Biological network exploration with Cytoscape 3. Curr. Protoc. Bioinforma. 47 (1), 8.13.11–24. doi:10.1002/0471250953.bi0813s47
Sundaram, V., and Wysocka, J. (2020). Transposable elements as a potent source of diverse cis-regulatory sequences in mammalian genomes. Phil. Trans. R. Soc. B 375 (1795), 20190347. doi:10.1098/rstb.2019.0347
Szklarczyk, D., Kirsch, R., Koutrouli, M., Nastou, K., Mehryary, F., Hachilif, R., et al. (2023). The STRING database in 2023: protein–protein association networks and functional enrichment analyses for any sequenced genome of interest. Nucleic Acids Res. 51 (D1), D638–D646. doi:10.1093/nar/gkac1000
Takase, H. M., and Nusse, R. (2016). Paracrine Wnt/β-catenin signaling mediates proliferation of undifferentiated spermatogonia in the adult mouse testis. Proc. Natl. Acad. Sci. 113 (11), E1489–E1497. doi:10.1073/pnas.1601461113
Tarailo-Graovac, M., and Chen, N. (2009). Using RepeatMasker to identify repetitive elements in genomic sequences. Curr. Protoc. Bioinforma. 25 (1), Unit 4.10–14.10.14. doi:10.1002/0471250953.bi0410s25
Toma, G. A., dos Santos, N., dos Santos, R., Rab, P., Kretschmer, R., Ezaz, T., et al. (2023). Cytogenetics meets genomics: cytotaxonomy and genomic relationships among color variants of the Asian arowana Scleropages formosus. Int. J. Mol. Sci. 24 (10), 9005. doi:10.3390/ijms24109005
Toubiana, W., Armisén, D., Dechaud, C., Arbore, R., and Khila, A. (2021). Impact of male trait exaggeration on sex-biased gene expression and genome architecture in a water strider. BMC Biol. 19 (1), 89–17. doi:10.1186/s12915-021-01021-4
Wang, D.-S., Kobayashi, T., Zhou, L.-Y., Paul-Prasanth, B., Ijiri, S., Sakai, F., et al. (2007). Foxl2 up-regulates aromatase gene transcription in a female-specific manner by binding to the promoter as well as interacting with Ad4 binding protein/steroidogenic factor 1. Mol. Endocrinol. 21 (3), 712–725. doi:10.1210/me.2006-0248
Wang, D.-S., Zhou, L.-Y., Kobayashi, T., Matsuda, M., Shibata, Y., Sakai, F., et al. (2010). Doublesex- and mab-3-related transcription factor-1 repression of aromatase transcription, a possible mechanism favoring the male pathway in Tilapia. Endocrinology 151 (3), 1331–1340. doi:10.1210/en.2009-0999
Webster, K. A., Schach, U., Ordaz, A., Steinfeld, J. S., Draper, B. W., and Siegfried, K. R. (2017). Dmrt1 is necessary for male sexual development in zebrafish. Dev. Biol. 422 (1), 33–46. doi:10.1016/j.ydbio.2016.12.008
Xie, Y., Li, H., Luo, X., Li, H., Gao, Q., Zhang, L., et al. (2022). IBS 2.0: an upgraded illustrator for the visualization of biological sequences. Nucleic Acids Res. 50 (W1), W420–W426. doi:10.1093/nar/gkac373
Xu, A., Teefy, B. B., Lu, R. J., Nozownik, S., Tyers, A. M., Valenzano, D. R., et al. (2023). Transcriptomes of aging brain, heart, muscle, and spleen from female and male African turquoise killifish. Sci. Data 10 (1), 695. doi:10.1038/s41597-023-02609-x
Yang, W. R., Ardeljan, D., Pacyna, C. N., Payer, L. M., and Burns, K. H. (2019). SQuIRE reveals locus-specific regulation of interspersed repeat expression. Nucleic Acids Res. 47 (5), e27. doi:10.1093/nar/gky1301
Ye, J., McGinnis, S., and Madden, T. L. (2006). BLAST: improvements for better sequence analysis. Nucleic Acids Res. 34 (Web Server issue), W6–W9. doi:10.1093/nar/gkl164
Yoshimoto, S., Ikeda, N., Izutsu, Y., Shiba, T., Takamatsu, N., and Ito, M. (2010). Opposite roles of DMRT1 and its W-linked paralogue, DM-W, in sexual dimorphism of Xenopus laevis: implications of a ZZ/ZW-type sex-determining system. Development 137 (15), 2519–2526. doi:10.1242/dev.048751
Yuan, Z., Shen, X., Yan, H., Jiang, J., Liu, B., Zhang, L., et al. (2021). Effects of the thyroid endocrine system on gonadal sex ratios and sex-related gene expression in the pufferfish Takifugu rubripes. Front. Endocrinol. 12, 674954. doi:10.3389/fendo.2021.674954
Zhang, H.-M., Liu, T., Liu, C.-J., Song, S., Zhang, X., Liu, W., et al. (2015). AnimalTFDB 2.0: a resource for expression, prediction and functional study of animal transcription factors. Nucleic Acids Res. 43 (D1), D76–D81. doi:10.1093/nar/gku887
Zhang, K., Xu, J., Zhang, Z., Huang, Y., Ruan, Z., Chen, S., et al. (2019). A comparative transcriptomic study on developmental gonads provides novel insights into sex change in the protandrous black porgy (Acanthopagrus schlegelii). Genomics 111 (3), 277–283. doi:10.1016/j.ygeno.2018.11.006
Zhang, X., Li, M., Ma, H., Liu, X., Shi, H., Li, M., et al. (2017). Mutation of foxl2 or cyp19a1a results in female to male sex reversal in XX nile Tilapia. Endocrinology 158 (8), 2634–2647. doi:10.1210/en.2017-00127
Zhang, X., Zhou, J., Li, L., Huang, W., Ahmad, H. I., Li, H., et al. (2020). Full-length transcriptome sequencing and comparative transcriptomic analysis to uncover genes involved in early gametogenesis in the gonads of Amur sturgeon (Acipenser schrenckii). Front. Zool. 17 (1), 11–21. doi:10.1186/s12983-020-00355-z
Keywords: Asia arowana, gonad transcriptome, sex-biased, foxl2, dmrt gene family
Citation: Zhao C, Bian C, Mu X, Zhang X and Shi Q (2024) Gonadal transcriptome sequencing reveals sexual dimorphism in expression profiling of sex-related genes in Asian arowana (Scleropages formosus). Front. Genet. 15:1381832. doi: 10.3389/fgene.2024.1381832
Received: 05 February 2024; Accepted: 03 April 2024;
Published: 11 April 2024.
Edited by:
Xu Wang, Auburn University, United StatesReviewed by:
Qing-Ping Xie, Zhejiang Academy of Agricultural Sciences, ChinaXiaoli Ma, Jiangsu Normal University, China
Zhiyuan Ruan, Merck, United States
Copyright © 2024 Zhao, Bian, Mu, Zhang and Shi. This is an open-access article distributed under the terms of the Creative Commons Attribution License (CC BY). The use, distribution or reproduction in other forums is permitted, provided the original author(s) and the copyright owner(s) are credited and that the original publication in this journal is cited, in accordance with accepted academic practice. No use, distribution or reproduction is permitted which does not comply with these terms.
*Correspondence: Chao Bian, bianchao@szu.edu.cn; Qiong Shi, shiqiong@szu.edu.cn, shiqiong@genomics.cn
†These authors have contributed equally to this work