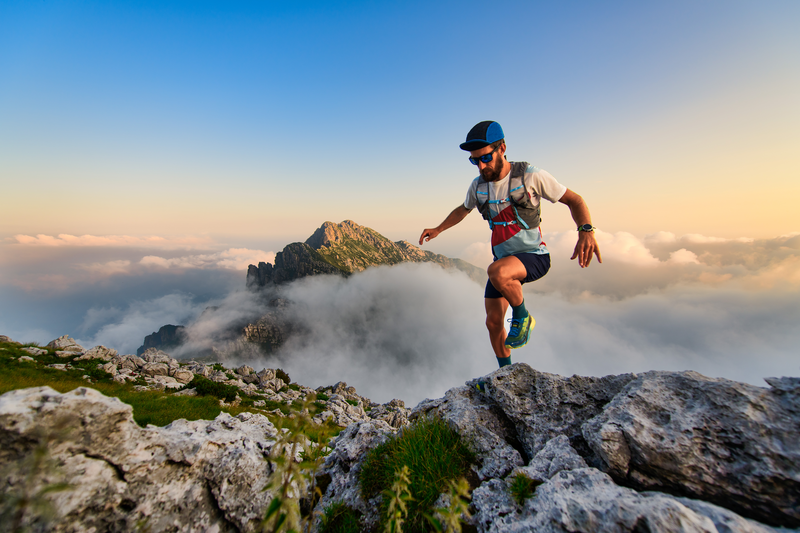
95% of researchers rate our articles as excellent or good
Learn more about the work of our research integrity team to safeguard the quality of each article we publish.
Find out more
ORIGINAL RESEARCH article
Front. Genet. , 08 April 2024
Sec. Genomics of Plants and the Phytoecosystem
Volume 15 - 2024 | https://doi.org/10.3389/fgene.2024.1381690
This article is part of the Research Topic Multi-omics and molecular biology studies on abiotic stress in crops View all 6 articles
A correction has been applied to this article in:
Corrigendum: Expression profiling of ALOG family genes during inflorescence development and abiotic stress responses in rice (Oryza sativa L.)
The ALOG (Arabidopsis LSH1 and Oryza G1) family proteins, namely, DUF640 domain-containing proteins, have been reported to function as transcription factors in various plants. However, the understanding of the response and function of ALOG family genes during reproductive development and under abiotic stress is still largely limited. In this study, we comprehensively analyzed the structural characteristics of ALOG family proteins and their expression profiles during inflorescence development and under abiotic stress in rice. The results showed that OsG1/OsG1L1/2/3/4/5/6/7/8/9 all had four conserved helical structures and an inserted Zinc-Ribbon (ZnR), the other four proteins OsG1L10/11/12/13 lacked complete Helix-1 and Helix-2. In the ALOG gene promoters, there were abundant cis-acting elements, including ABA, MeJA, and drought-responsive elements. Most ALOG genes show a decrease in expression levels within 24 h under ABA and drought treatments, while OsG1L2 expression levels show an upregulated trend under ABA and drought treatments. The expression analysis at different stages of inflorescence development indicated that OsG1L1/2/3/8/11 were mainly expressed in the P1 stage; in the P4 stage, OsG1/OsG1L4/5/9/12 had a higher expression level. These results lay a good foundation for further studying the expression of rice ALOG family genes under abiotic stresses, and provide important experimental support for their functional research.
Rice (Oryza sativa) is one of the most important food crops in the world and an ideal model plant for genomics research and monocotyledonous plant evolutionary lineage research (Chen et al., 2022). Some studies had reported that ALOG genes involved in the regulation of lemma, palea and inflorescence development in rice. For example, OsG1 inhibits the homologous transformation of sterile lemma to normal lemma, and TH1/BH1/AFD1 affects the development of rice lemma/palea, spikelet morphogenesis, and grain shape. It has been reported that both genes belong to the ALOG family (Li et al., 2019). The characteristic of members of the Arabidopsis LSH1 and Oryza G1 (ALOG) gene family is the conserved DUF640 domain which is considered specific transcription factor in plants (Yoshida et al., 2009; Chen et al., 2019). The ALOG domain is suggested to originate from the N-terminal DNA-binding domains of the XerC/D-like tyrosine recombinases encoded by a novel category of DIRS1-like LTR (long terminal repeat) retrotransposon found in several eukaryotes. The N-terminus of the ALOG domain fused with a specific but weakly catalytic N6-adenine methylase active region, while the C-terminus fused with a tyrosine recombinase catalytic active region. These two regions are prominent features of DIRS1-like retrotransposons in eukaryote (Poulter and Goodwin, 2005). The secondary structure predictions showed that the ALOG domain consisted of four conserved α-helices, and an additional Zinc-Ribbon (ZnR) was inserted between helix-2 and helix-3 with “HxxxC” and “CxC” motifs, making this domain more flexible and facilitating binding to more DNA (Iyer and Aravind, 2012).
Current researches indicate that the ALOG genes play an important role in seedling growth, floral organ development and inflorescence meristem differentiation (Li et al., 2019). LIGHT-DEPENDENT SHORT HYPOCOTYLS 1 (LSH1) is the first ALOG gene identified from Arabidopsis, and mainly expressed in hypocotyls, shoot tips, and lateral root primordia. The functional analysis suggested that AtLSH1 might play a role as a transcriptional regulatory protein to control seedling development in light-dependent manner (Zhao et al., 2004). Overexpression of AtLSH1 and AtLSH2 significantly inhibit the hypocotyls elongation and reproductive growth of Arabidopsis (Lee et al., 2020). LSH4 and its homologous gene LSH3 are expressed in the boundary cells of various bud organs, and inhibit bud organ differentiation in boundary region during plant development (Takeda et al., 2011). Moreover, the expression of LSH4 in the shoot apex inhibits leaf growth during the vegetative period and the formation of additional shoot organs within the flower during the reproductive period. LSH8 participates as a positive regulatory factor in the ABA signaling pathway during seed germination and seedling growth in Arabidopsis (Zou et al., 2021). LSH9 regulates hypocotyl elongation by interacting with temperature receptor ELF3 (Press and Queitsch, 2017). LSH10 is found to interacts with OTLD1 in plant cells to form an OTLD1-LSH10 co-repressor protein complex that deubiquitinates histone 2B and inhibits the expression of genes involved in growth, cell expansion, and hormone signaling (Vo Phan et al., 2023). These researches indicated that the ALOG genes in Arabidopsis were involved in vegetative and reproductive development.
OsG1, the first gene reported in the rice ALOG family, is mainly expressed in the sterile lemma primordia during their development, and the sterile lemma in the g1 mutant is larger than WT. The further analysis reveals that OsG1 may serve as a transcription inhibitor and specify the identity of sterile lemma by regulating downstream hormone signal transduction (Yoshida et al., 2009). Similarly, OsG1L6 regulates the development of rice lemma and palea by controlling cell division and expansion, thereby affecting grain shape and size (Yan et al., 2013). In addition, OsG1L6 acts as a transcription inhibitor and regulates cell expansion during lateral development of spikelets (Ren et al., 2016; Peng et al., 2017). OsG1L5, as known as TAW1 (TAWAWA1), is highly expressed in shoot apical meristem, and regulates inflorescence development by maintaining inflorescence meristem (IM) activity and inhibiting the phase transitions of spikelet meristem (SM) identity (Yoshida et al., 2013). Similar to OsG1L5, OsG1L1 and OsG1L2 are also expressed at high levels in the IM tissues, and regulate inflorescence branching in rice. Compared with the WT, osg1l1 and osg1l2 had shorter panicles, fewer spikelets, and smaller grains, farther the inflorescence structure of the mutants’ phenotype are similar to that of the TAW1 (Beretta et al., 2023). TtTAW1-1A, the homologous gene of OsG1L5 in Triticum turgidum participates in regulating the development of branching and meridians in wheat (Nan et al., 2018). In sorghum, DOMINANT AWN INHIBITOR (DAI), derived from gene replication, encodes an ALOG protein, which may inhibit awn elongation by acting as a transcription factor to repress cell proliferation and elongation in sorghum (Takanashi et al., 2022). Overexpression of PhLSH7a and PhLSH7b in Arabidopsis resulted in rounded leaves, late flowers and deformed flowers, indicating that these two genes significantly affected the vegetative and reproductive growth (Chen et al., 2019). An ALOG protein in Solanum lycopersicum, Terminating Flower (TMF), affects panicle architecture by inhibiting inflorescence meristem tissue (MacAlister et al., 2012). These research results indicated that the ALOG family genes involved in regulation of the reproductive and developmental processes of plants, but its function under abiotic stress was still unclear.
Improving rice yield and tolerance to abiotic stress had always been a research hotspot (Reyes, 2023), and some ALOG family members had been reported to be essential for rice grain development. However, the analysis of ALOG family members was not systematic enough. Therefore, this study conducted amino acid sequence alignment, 3D protein structure prediction, phylogenetic analysis, collinearity analysis, promoter cis-acting element analysis, expression analysis during inflorescence development and under abiotic stresses of the ALOG family in rice. This study is crucial for understanding the evolution and diversity of rice ALOG genes and their potential roles in plant growth and stress response.
In order to analyze the expression profile of the ALOG genes during vegetative and reproductive development, the rice plants of O. sativa japonica cv. Zhonghua 11 (ZH11) were grown under normal field conditions in summer at Anhui Science and Technology University. The different tissues were used, including 7-day-old seedlings (YS), 80-day-old roots (R), 80-day-old stems (St), 80-day-old leaves (YL), seeds at 3 DAP (day after pollination, S2) and at 5 DAP (S3), different stages of panicle development: P1, 0–1 cm; P2, 1–3 cm; P3, 3–5 cm; P4, 5–10 cm; P5, 10–15 cm; P6, 15–22 cm.
For expression analysis of ALOG genes in response to ABA and drought treatments, the rice seeds of ZH11 were germinated and sown in the 96 well boxes with nutrient solution in the greenhouse at 25°C–30°C and 60% humidity with a photoperiod of 16 h/8 h (light/dark). The 14-day-old seedlings were placed in nutrient solution containing 200 μM ABA or 20% PEG6000, respectively. The samples were selected at 0, 3, 6, 12, and 24 h after the treatments. Parallel control samples were prepared by keeping the seedlings in nutrient solution. All materials contained three biological replicates, and were immediately frozen in liquid nitrogen and stored at −80°C for RNA extraction.
For multiple sequence alignments, the full-length protein sequences of the ALOG family members in rice and Arabidopsis were obtained from the NCBI (https://www.ncbi.nlm.nih.gov) and TAIR (https://www.arabidopsis.org) databases, respectively (Supplementary Table S1). The MUSCLE program of MEGA 11 was used to align amino acid sequences of the ALOG domain (about 134 aa) in rice and Arabidopsis. Then, the GeneDoc software was used to color the regions with different degrees of sequence conservation.
The initial 3D protein structure was performed by using the AlphaFold2 program based on amino acid sequences of the ALOG family in rice, and the ALOG domains of the protein 3D structures were colored in the PyMOL software (https://pymol.org/2/).
The ALOG family protein sequences in Brachypodium distachyon, Sorghum bicolor, and S. lycopersicum were obtained from the online database phytozome 13 (https://phytozome-next.jgi.doe.gov/blast-search) (Supplementary Table S1). MEGA 11 was used to align the protein sequences of the ALOG families with the ClustalW program with parameters set to default values. Using the method of Maximum Likelihood, a phylogenetic tree was constructed for the protein sequences of ALOG family members, with a bootstrap repeat value of 1,000, and other parameters were defaulted. The phylogenetic trees were visualized using the Interactive Tree of Life (iTOL) program.
The coding sequences (CDS) (Supplementary Table S2) and genomic sequences (Tbale S3) of the OsG1/OsG1L1-13 were used to analyze the exon-intron structures in the GSDS (Gene Structure Display Server 2.0) website (gsds.gao-lab.org).
The rice ALOG family protein sequences were submitted to the MEME suite (http://meme-suite.org/tools/meme) and processed with a maximum discovery number of motifs set to 10, and then the result file was visualized through the TBtools.
The GFF3 annotation files of rice, Arabidopsis and B. distachyon were sourced from the database ensemblplants (http://plants.ensembl.org/info/data/ftp/index.html). Based on the GFF3 files, the MCScanX program was used to analyze the repeated events of ALOG genes (Wang et al., 2012). The conserved synteny between rice and other two species were analyzed using the Dual Synteny Plotter program in TBtools.
In order to verify the subcellular localization prediction of ALOG members, the CDS sequences of OsG1L3 and OsG1L7 were amplified from ZH11 cDNA using the specific primers (Supplementary Table S4), and fused with vector pCAMBIA2300. The recombinant constructs (35S::OsG1L3/7-GFP) and the empty construct (35S::GFP) were transformed separately into rice protoplasts. The GFP signals were observed by a laser scanning microscope (C2-ER, Nikon, Japan) after culture for 16 h, and the excitation and emission wavelength was 488 and 510 nm, respectively.
The microarray data of ALOG genes in rice were extracted from the Rice Functional Genomic Express Database (http://signal.salk.edu/cgi-bin/RiceGE), and then were used to analyze expression profiles of the genes in the tissues during different development stages (GSE6893, GSE6901, GSE7951). The absolute signal values in the microarray data were respectively divided by the average of all absolute values, and the logarithmic values of the ratios were used to generate hierarchical cluster display using the Heatmap function in software TBtools.
To understand the cis-regulatory elements in the promoter region of ALOG genes, we used TBtools to retrieve the genomic sequences of 3,000 bp upstream of the start codon (ATG), and then submitted them to PlantCARE online website (http://bioinformatics.psb.ugent.be/webtools/plantcare/html) to predict the cis-acting elements, notably ABA and drought stress response elements. The results were visualized using Simple Biosequence Viewer and HeatMap program in the TBtools.
The extraction and quality detection of total RNA from all collected samples were carried out according to the method of Gan et al. (2022). For quantitative real time PCR, 1 μg of RNA was reverse transcribed into cDNA using the MonScriptTM RTIII AII-in-One Mix with dsDNase (Monad, Wuhan) kit according to the instruction manual, and qRT-PCR was performed with the SYBR Green Ⅰ PCR Master Mix system on the ABI ViiA7 real-time fluorescence quantitative PCR instrument (Life Technologies, USA). The gene-specific primers of OsG1/Ls were listed in Supplementary Table S5. The reaction system is 20 μL: 10 μL for ProQTM qPCR EvaGreen Master Mix (Biomed), 2 μL cDNA, 0.5 μL Forward primer and 0.5 μL Reverse primer, ddH2O up to 20 μL. The qRT-PCR program was: 95°C for 2 min; 40 cycles of denaturation at 95°C for 15 s, annealing at 60°C for 15 s and extension at 72°C for 30 s. The Actin gene was used as the internal control in rice. The relative expression levels of the genes examined were evaluated using the 2−ΔCT method (Livak and Schmittgen, 2001). The mean values ±SD (standard deviation) were calculated in the SPSS 26.0 software (p < 0.05), and the software GraphPad Prism 8.0 was used to visualize data.
According to previous reports, there were 14 members in the rice ALOG family (Li et al., 2019), and we collected basic information of 14 ALOG members (Supplementary Table S6). To better understand the sequence characteristics of ALOG domain, the sequences of ALOG proteins from rice and Arabidopsis were aligned and analyzed (Figure 1A). The results showed that most of ALOG proteins contained a conserved domain with 134 aa, which consisted of Helix-1/2/3/4 and a zinc ribbon inserted between Helix-2 and Helix-3. However, 10 out of the 14 ALOG proteins from rice included all conserved domain, of which OsG1L10 had a partial change in its first helical structure Helix-1, OsG1L11/12 lost the Helix-1, and OsG1L13 lost Helix-1 and Helix-2, and part of Helix-4 were only missing in OsG1L12 (Figure 2A). These results were consistent with previous reports (Iyer and Aravind, 2012), and showed that most ALOG proteins were highly conserved.
Figure 1. Multiple sequence alignment and 3D analysis of ALOG proteins. (A) Multiple sequence alignment of the ALOG proteins from rice and Arabidopsis thaliana. Multiple alignments of the highly conserved ALOG domains (134 aa) of ALOG proteins. The conserved ALOG domain included Helix-1/2/3/4 and a zinc ribbon insert structure. (B) Prediction of three-dimensional structure of 14 ALOG proteins from rice. Helix-1, Helix-2, Helix-3 and Helix-4 were marked in red, and the zinc ribbon insert was marked in yellow.
Figure 2. Phylogenetic tree of ALOG domain proteins in five different plant species. On the basis of the full-length sequences of ALOG proteins, a total of 59 proteins were used to construct the phylogenetic tree. Species abbreviations were as follows: Os, Oryza sativa; At: Arabidopsis thaliana; Bd: Brachypodium distachyon; Sl: Solanum lycopersicum; Sb: Sorghum bicolor. Clades A-I were indicated in different colored circles.
Protein conformation is usually related to their function (Varadi et al., 2022). To further explore the conservation and difference of ALOG domains, the three-dimensional (3D) structures of 14 ALOG proteins from rice were predicted through Alphafold2 program (Jumper et al., 2021). The results showed that the secondary structure of all ALOG proteins contained a zinc ribbon insert, and four helix structures existed in OsG1/OsG1L1/2/3/4/5/6/7/8/9/10 (Figure 1B). Among the other three proteins, OsG1L11/12/13, Helix-1 were absent in OsG1L11 and OsG1L12. Both of Helix-1 and Helix-2 were missing in OsG1L13. Moreover, it was noteworthy that four helix structures were parallel to each other in OsG1L10, which was different from the helix structures of conformation in other proteins.
To explore the evolutionary relationship and functional differences among ALOG members, the phylogenetic tree was constructed using the full-length sequences of ALOG proteins from rice and other four species, including Arabidopsis thaliana, B. distachyon, S. lycopersicum, and Sorghum bicolor (Figure 2). On the basis of phylogenetic analysis, 59 ALOG proteins from five species were divided into nine different clades (Clade A-I) in the phylogenetic tree. Among nine clades, the ALOG proteins in clades A, B, C, D, E and I were all from monocotyledons, the ALOG proteins in clade F and G were only from dicotyledons. Differently, the clade H contained not only three proteins from dicotyledons but also 10 proteins from monocotyledons. Particularly, the clade B was only composed of SbG5. 14 ALOG proteins from rice were unevenly distributed on six clades, clade A containing OsG1L2/10/11/12/13, clade C including OsG1L1, clade D including OsG1, clade E including OsG1L3/4/5, clade H containing OsG1L7/8/9 and clade I including OsG1L6.
To further understand the sequence conservation and divergence of rice ALOG members, gene structrue and conserved motifs were identified in the 14 ALOG proteins. Gene structure analysis revealed that half of rice ALOG genes (OsG1L2/3/4/5/6/10/13) contained introns, of which OsG1L2/5/10 had only one intron and OsG1L3/4/6/13 had two introns. The members of the same clade in the phylogenetic tree had similar exon/intron structures, with OsG1L7/8/9 (belong to clade H) not containing introns and OsG1L3/4 containing two introns (Supplementary Figure S1A, B). Motif analysis showed that a total of 10 motifs were present in ALOG proteins and the number of motifs in each protein varies from five to eight (Supplementary Figure S1C). Motifs one to five constituted the ALOG conserved domain and existed in OsG1/OsG1L1/2/3/4/5/6/7/8/9, and motifs 2, three and five existed in all ALOG proteins, while other motifs showed member-specific distribution. For instance, motif six just appeared in OsG1L10 and OsG1L11. Motif nine existed only in OsG1L1 and OsG1L2 and motif eight was specific to OsG1L6. These results indicated that most members of the ALOG family contained conserved motifs and members of the same clade had similar structures.
Gene duplication events, such as tandem and segmental duplication, provide major forces that drive the expansion of gene families and the evolution of the entire genome (Cen et al., 2023). To reveal the origin and evolutionary information of ALOG genes, a collinearity analysis was respectively performed between the genome of rice and two other species, including Arabidopsis (dicotyledon plant) and B. distachyon (monocotyledon plant) (Figure 3A). The results showed that three and 20 collinear gene pairs were found in rice with Arabidopsis and B. distachyon, respectively. Compared with the dicotyledon plant, ALOG genes revealed a high level of identity with the monocotyledon plant with fewer evolutionary separation events.
Figure 3. Collinearity analysis of ALOG genes. (A) Collinearity relationship between ALOG genes from rice with Arabidopsis and Brachypodium distachyon. The chromosomes of rice, Arabidopsis and Brachypodium distachyon were marked with different colors. (B) Collinearity relationship of ALOG genes from rice. The collinear relationship between the ALOG family members of different species were connected by red lines.
To determine the collinear relationships of the ALOG genes from rice, we used the TBtools software to examine the duplication events of ALOG members within the rice genome (Figure 3B). There were five pairs of segmental duplication genes (OsG1L1/2, OsG1L3/4/5, OsG1L7/8) in the ALOG gene family, and these genes were distributed on six chromosomes (chromosome 1, 2,4, 5, 6, and 10). These results suggested that segmental duplication events might be a vital driving force for the expansion of ALOG gene family.
To verify the prediction of subcellular localization, OsG1L3 and OsG1L7 were performed for subcellular localization. The full-length coding sequences of OsG1L3 and OsG1L7 were fused with green fluorescent protein (GFP) to construct the expression vectors of fusion proteins, respectively. Then, the two fusion vectors, OsG1L3-GFP and OsG1L7-GFP were transformed into rice protoplasts, and the fluorescence signals were detected by laser scanning confocal microscopy. As the results were shown in Figure 4, the fluorescence signal from 35S::GFP vector was detected throughout the whole rice protoplasts, and the signals from 35S::OsG1L3/7-GFP vector were observed in the nucleus, indicating that both OsG1L3 and OsG1L7 were localized in the nucleus (Figures 4A,B). The results of subcellular localization were consistent with the predictions (Supplementary Table S6), suggesting that ALOG genes might play crucial roles in the nucleus.
Figure 4. The subcellular localization of OsG1L3 and OsG1L7. (A) The signal of 35S::OsG1L3-GFP fusion protein; (B) The signal of 35S::OsG1L7-GFP fusion protein. The 35S::OsG1L3/7-GFP and control vector (35S::GFP) were transiently expressed in rice protoplasts. Confocal microscopy images were visualized after 16 h transformation. Scale bars = 10 μm.
To explore the expression patterns of ALOG genes in different tissues and organs from rice, the microarray data of 13 ALOG genes (OsG1L3 was not detected) were collected from the Rice Functional Genomic Express Database (http://signal.salk.edu/cgi-bin/RiceGE) (Supplementary Table S7). The various tissues at different developmental stages were examined, including 7-day-old seedlings (YS), young roots (YR), young leaves (YL), mature leaves (ML), shoot apical meristem (SAM), panicles (P1-6), stigma of mature pistil (Sti), mature ovary (OV) and seeds (S1-5). The expression heatmap of ALOG genes were also constructed by TBtools (Figure 5A). The results revealed that ALOG genes mainly display three expression patterns among various tissues. For instance, group Ⅰ (OsG1L5/10/12/13) were highly expressed in seedling tissues (YS, YR, YL) and panicles (P1-6), group Ⅱ (OsG1/OsG1L1/4/6/11) were principally expressed in the middle and late stages of seed development (S3, S4, S5), and group Ⅲ (OsG1L2/7/8/9) were mainly expressed in shoot apical meristem (SAM) and early stages of inflorescence development (P1, P2). Most ALOG genes mainly expressed in early seedling and inflorescence suggest that these genes might paly roles in reproductive development.
Figure 5. Expression profiles of ALOG genes in various tissues. (A) The expression of microarray data of ALOG genes in various organs at different stages. The heat map representing hierarchical cluster was generated by using the average log2 expression values of ALOG genes in various organs, including YS, 7-day-old seedlings; YR, roots from 7-day-old seedlings; YL, leaves from 7-day-old seedlings; ML, mature leaf; SAM, shoot apical meristem; different stages of panicle development: P1, 0–3 cm; P2, 3–5 cm; P3, 5–10 cm; P4, 10–15 cm; P5, 15–22 cm; P6, 22–30 cm; Sti, stigma of mature pistil; OV, mature ovary; different stages of seed development: S1, 0–2 dap (day after pollination); S2, 3–4 dap; S3, 5–10 dap; S4, 11–20 dap; S5, 21–29 dap. The differential expression of ALOG genes in various organs were consistent with the results of qRT-PCR analysis, which were represented by the asterisk (*) on the right. The color scale (representing the average log signal value) was displayed on the right side. (B) The expression of ALOG genes (marked by asterisks in A) in different organs using qRT-PCR, which were consistent with microarray data using qRT PCR. The error bar represented the standard deviation of three independent biological replicates (n = 3). Different letters (a, b, c, d) denoted significant differences at P < 0.05 according to ANOVA in combination with Duncan’s multiple range test.
To validate the expression profiles of ALOG genes in different tissues, the qRT-PCR analysis was performed in rice. The results showed that OsG1 was highly expressed in P1 and P2, and OsG1L5 was predominantly expressed in young seedlings and leaves, which were consistent with results from microarray data (Figure 5B). Moreover, OsG1L1 and OsG1L2 were highly expressed in the early inflorescence (P1), and OsG1L6 and OsG1L13 were specially expressed in P2, whereas, OsG1L3 and OsG1L4, were preferentially expressed in ML, and OsG1L12 were specially expressed in St (Supplementary Figure S2). These data suggested that ALOG genes might play various roles in the specific vegetative and reproductive tissues at different development stages.
To find the key genes of ALOG family that might have functions during the reproductive development, the expression profile analysis of rice ALOG genes in inflorescence at specific developmental stages was performed using qRT-PCR. According to the length, the inflorescences were divided into six developmental stages (P1-6). OsG1L1/2/3/8/11 showed similar expression patterns, which were mainly expressed in the early development stages of inflorescence (P1, panicle length = 0–1 cm, Figure 6A). OsG1/OsG1L4/5/9/12 were principally expressed at the P4 stage (panicle length = 5–10 cm, Figure 6B). In particular, OsG1L6 was highly expressed at the P2 (panicle length = 1–3 cm) and P4 stages, OsG1L7 was specifically expressed at the P6 (panicle length = 15–20 cm) stage, and OsG1L13 was mainly expressed at the P2 stage (Supplementary Figure S3). These data suggested that the ALOG genes might play a variety of roles during inflorescence development in rice.
Figure 6. The expression profiles of ALOG genes in inflorescence. (A) The ALOG genes mainly expressed at P1 stage of inflorescence. (B) The ALOG genes predominately expressed at P4 stage of inflorescence. The stages of P1–P6 were represented by the spikelet length of 0–1, 1–3, 3–5, 5–10, 10–15, 15–22 cm. The y-axis was used to detect the relative expression level of the genes at different stages of inflorescence using qRT-PCR. The error bar represented the standard deviation of three independent biological replicates (n = 3). Different letters (a, b, c, d) denoted significant differences at P <0.05 according to ANOVA in combination with Duncan’s multiple range test.
Cis-acting elements within promoters of ALOG genes were predicted, so as to speculate on the possible factors affecting ALOG gene expression and the regulatory pathways in which ALOG genes might be involved. The 3,000 bp upstream genomic sequences from the start codon of 14 ALOG genes in rice were extracted by TBtools and used to predict the cis-acting elements in the putative promoter regions by PlantCARE (Lescot et al., 2002). A total of 216 cis-acting elements were detected in the putative promoter regions of the ALOG genes, including auxin responsive elements (TGA-element and TGA-box), ABA responsive elements (ABRE), MeJA responsive elements (CGTCA-motif and TGACG-motif), GA responsive elements (TATC-box and P-box), drought responsive elements (MBS), SA responsive elements (TCA-element) and defense and stress responsive elements (TC-rich repeats). The cis-acting elements were mainly associated with phytohormone and stress responses (Figures 7A,B). All the 14 ALOG genes contained more than two ABA responsive elements, and seven of them contained more than five ABA responsive elements. Most of the ALOG genes contained drought responsive elements. The above results imply that ALOG genes might be involved in plant response to abiotic and hormones stresses.
Figure 7. Prediction of cis-acting elements related to hormone and abiotic stress responses in the promoter region and expression analysis of ALOG genes in rice. (A) The number of cis-acting elements detected in the putative promoter region (the sequence was retrieved from the 3 kb region upstream of the start codon). The cis-acting elements were divided into 10 categories. (B) The number, type and location of stress-related and hormone-responsive elements in the putative promoter regions were speculated by the ALOG genes in (A). TGA-element and TGA-box, Auxin responsive elements; ABRE, ABA responsive elements; CGTCA-motif and TGACG-motif, MeJA responsive elements; MBS, drought responsive elements; TATC-box and P-box, GA responsive elements; TCA-element, SA responsive elements; TC-rich repeats, defense and stress responsive elements. Different types of elements were represented by different colors. (C) The expression analysis of ALOG genes under ABA treatment. (D) The expression analysis of ALOG genes under drought treatment. The y axis was the relative expression level of the genes compared to Actin under different treatments using qRT-PCR. The expression level was based on the relative expression level of Actin at 0 h as a reference. The error bar represented the standard deviation of three independent biological replicates (n = 3). Different letters (a, b, c, d) denoted significant differences at P <0.05 according to ANOVA in combination with Duncan’s multiple range test.
To explore the potential involvement of ALOG genes in abiotic stresses, the expression profile of ALOG genes under ABA and drought treatments were evaluated using qRT-PCR. The results showed that 10 genes (OsG1L1/3/4/5/7/8/9/11/12/13) were significantly decreased at 3/6/12/24 h after ABA treatment, and OsG1L2 was increased significantly at 3/6/12 h (Figure 7C; Supplementary Figure S4). Under drought treatment, 11 genes (OsG1L1/3/4/5/6/7/8/9/11/12/13) were significantly decreased at 3/6/12/24 h (Figure 4D; Supplementary Figure S5). Interestingly, most of ALOG genes (OsG1L1/3/4/5/7/8/9/11/12/13) showed similar response patterns under ABA and drought stresses. The trends in expression of ALOG genes suggested that they might be involved in plant early response to ABA and drought stresses.
The ALOG domain had been proposed to be originated from the XerC/D-like recombinases of a new category of DIRS-1-like retroposons and consisted of a full α-helix domain with four conserved helices (Helix-1/2/3/4) and a conserved predicted zinc band (ZnR) insert (Iyer and Aravind, 2012). In our investigation, the prediction of protein 3D structure showed that Helix-1 and Helix-2 were incomplete in OsG1L11/12/13. Helix-1/2 were also missing in the amino acid sequence alignment of OsG1L10, but the 3D structure of the protein showed that its four helix structures were parallel to each other. Iyer and Aravind (2012) inferred that the helix-1 and helix-3 of the ALOG domains, which were orthogonally positioned with respect to each other, were likely to make key backbone and based contacts in the major groove. The change of helix structures might be one of the reasons for the functional diversity of ALOG members.
Based on previous studies, it has been understood that ALOG genes played an important role in plant morphogenesis and organ development (Turchetto et al., 2023). Which organs the ALOG family played roles in, however, still remained largely unclear. Currently, the systematic phylogenetic analysis of ALOG family members suggested that rice ALOG members were unevenly distributed among four clades (Li et al., 2019). In this study, the phylogenetic analysis showed that rice ALOG members could be divided into six clades (A, C, D, E, H and I): the numbers in clade D, and H were consistent with the results of Li et al. (Li et al., 2019), including OsG1 and OsG1L7/8/9, respectively; clade A included OsG1L2/10/11/12/13, clade C included only OsG1L1, clade E included OsG1L3/4/5 and clade I included OsG1L6, which were different from the previous findings. The different distribution of rice ALOG members in the phylogenetic trees might be caused by the different plant species. Among the members of the ALOG family in rice, OsG1L1/2/5/6 had been reported to be involved in the regulation of rice reproductive development. The mutant plants of OsG1L1/2, osg1l1/osg1l2, developed panicles that were shorter, with fewer primary branch meristems (PBMs), extremely reduced secondary branch meristems (SBMs) and fewer spikelets than the wild-type plants (Beretta et al., 2023). In the dominant gain-of-function mutant tawawa1-D (the mutant plant of OsG1L5), the activity of the inflorescence meristem (IM) was extended and spikelet specification was delayed, resulting in prolonged branch formation and increased numbers of spikelets (Yoshida et al., 2013). Abnormal floral organs were observed in the afd1 (the mutant plant of OsG1L6), including slender and thick hulls, and hull-like lodicules (Ren et al., 2016). It was especially noteworthy that OsG1L1/2/5/6 were distributed in four different clades in the phylogenetic tree, suggesting that they might be conservative in function.
In order to further explore the conservatism of the members of the ALOG family, collinearity analysis was performed on 14 rice ALOG members. Intraspecific collinearity analysis showed that half of the members of the rice ALOG family (OsG1L1/2, OsG1L3/4/5, OsG1L7/8) had collinearity. Although OsG1L1/2 did not belong to the same clade, they had a collinear relationship, further confirming that rice ALOG family members might be functionally conserved. OsG1L3/4/5 had collinear relationships and were also in the same clade in the phylogenetic tree, hinting that OsG1L3/4 might have similar functions to OsG1L5 in rice.
ALOG family members had been reported in a few plants and played important roles in plant growth and development, particularly in reproductive development (Chen et al., 2019). The analysis on spatial and temporal expression patterns of genes might provide useful information for establishing their putative functions (Zhiguo et al., 2015). In our study, the analysis of microarray data showed that 10 of the 13 genes were specifically expressed during the shoot apical meristem (SAM) period (Figure 5A). Previous studies had shown that OsG1L1/2/5 were preferentially expressed in the IM tissues, indicating that they play an important role in reproductive meristems, especially in inflorescences (Beretta et al., 2023). The qRT-PCR data showed that OsG1L1/2/5 were specifically expressed in the early stage of inflorescence development (P1, P2) (Figure 5B). These results suggested that they might regulate inflorescence development from the SAM period to the early IM period. Similarly, the microarray data showed that OsG1L6 was preferentially expressed at the SAM stage, and qRT-PCR data showed that it was preferentially expressed at the P2 stage. According to recent studies, OsG1L6 was expressed in the 4–5 cm period of panicle length, and its pleiotropic effect affects the development of spikelets (Peng et al., 2017). These data indicated that OsG1L6 might regulated spikelet development similar to OsG1L1/2/5. Most ALOG genes were specifically expressed in the SAM stage, and half of the genes were highly expressed in the early stage of inflorescence development, suggesting that these genes also played a role in the inflorescence development.
Inflorescence development is a relatively long and complex process. We performed expression analysis for a more comprehensive inflorescence development period (P1∼6). The results showed that OsG1L1/2 were highly expressed at the P1 stage, OsG1L5 was specifically expressed at the P4 stage, and OsG1L6 was specifically expressed at the P2 and P4 stages (Figures 6A,B). According to previous studies, OsG1L6 not only regulates inflorescence development, but also affects grain size (Peng et al., 2017). The research proposed that OsG1L5 regulates the inflorescence architecture of rice through the promotion of IM activity and suppression of the phase change to SM identity. The similar expression pattern of OsG1L5 and OsG1L6 produced functional differentiation, indicating that they might regulate inflorescence development in different ways.
Drought is a major environmental factor that affects the development of plants, in addition, abscisic acid (ABA), which is the central regulator of abiotic stress resistance in plants, coordinates an array of functions enabling plants to cope with different stresses. Abiotic stresses, especially drought, induce ABA accumulation, which triggers rapid biochemical and physiological responses that enhance stress adaptation. At present, there were still limited reports on abiotic stress of ALOG family members in rice (Xu et al., 2020).
The regulation of gene expression by cis-elements in the promoter region had become the important adaptive mechanism for organisms to respond to environmental changes (Walther et al., 2007; Lai et al., 2022). We conducted in-depth analysis of the upstream promoter region of the ALOG genes in rice. The ABA response elements (ABRE) were abundant in the promoter region of the ALOG genes, and half of the ALOG members contained at least 5 ABRE elements, of which the most contained 12 ABRE elements. Drought responsive elements (MBS) closely related to ABA also existed in the promoter regions of most ALOG members. (Figure 7A). The expression analysis using real-time PCR data revealed that most ALOG genes showed downregulated expression levels under ABA and drought stresses (Figures 7C,D). A recent research had shown that the expression level of LSH8 decreased under ABA treatment. LSH8 mutant lines lsh8-1 and lsh8-2 showed ABA-insensitive phenotypes during seed germination, primary root and lateral root development. The ABA response elements (ABRE) were also identified in the LSH8 promoter region and the protein localized in the nucleus (Zou et al., 2021). In this study, OsG1L7 also contained a large number of ABRE elements (9), and subcellular localization results also showed that it was located in the nucleus (Figure 4B). Similarly, OsG1L7 was significantly downregulate under ABA and drought treatment, suggesting that OsG1L7 might have similar functions to LSH8 and might also be an important factor in the ABA signaling pathway. Interestingly, OsGlL2 and OsGlL7 had similar cis-element combination. OsG1L2 had the most 12 ABRE elements, and OsG1L7 had 9 ABRE elements next to OsG1L2. However, OsGlL2 and OsGlL7 showed opposite expression patterns under ABA and drought stresses. These results suggested that they might respond to aboitic stresses in different ways. It was also worth noting that the expression levels of most ALOG genes were significantly decreased under ABA and drought treatments, indicating that rice ALOG family genes might be involved in the regulation of abiotic stress. However, the molecular mechanism of ALOG family genes involved in abiotic stress regulation is still unclear.
ALOG is an important family that plays a role in plant growth and development. In this study, based on the analysis of phylogenetic relationship, protein 3D structure, collinearity, cis-acting elements, and expression profile, it is concluded that the ALOG family in rice have conserved domain, the cis-acting elements in the promoters are mainly involved in the response to hormone and abiotic stresses, and the most genes are predominantly expressed in vegetative tissues and early inflorescences. These data could provide important insights for further understanding the functions of ALOG family genes in rice growth and development, and offer valuable information for the selecting candidate genes and functional validation studies of rice ALOG members.
The datasets presented in this study can be found in online repositories. The names of the repository/repositories and accession number(s) can be found in the article/Supplementary Material.
HeZ: Conceptualization, Funding acquisition, Project administration, Visualization, Writing–review and editing. ZhL: Data curation, Visualization, Writing–original draft. ZF: Data curation, Writing–original draft. LW: Data curation, Writing–original draft. SZ: Data curation, Writing–original draft. WX: Data curation, Writing–original draft. SZ: Data curation, Writing–original draft. SF: Data curation, Writing–original draft. ML: Data curation, Writing–original draft. SK: Data curation, Writing–original draft. ShZ: Data curation, Writing–original draft. NK: Data curation, Writing–original draft. HA: Supervision, Writing–review and editing. RL: Supervision, Writing–review and editing. TF: Supervision, Writing–review and editing. SW: Conceptualization, Project administration, Writing–review and editing.
The author(s) declare that financial support was received for the research, authorship, and/or publication of this article. This study was supported by the Natural Science Foundation of Anhui Province (2308085MC70); the key project of the Education Department of Anhui Province (2023AH051850); the Excellent Scientific Research and Innovation team of the Education Department of Anhui Province (2022AH010087); the Natural Science Foundation of Hubei Province - General Project (2019CFB625); the Talent Introduction Project of Anhui Science and Technology University (NXYJ202104); the National College Student Innovation and Entrepreneurship Training Program Project of China (202210879058, 202310879076); the College Student Innovation and Entrepreneurship Training Program Project of Anhui Province (S202310879168). The funding institution was not involved in the design of the study, collection, analysis, and interpretation of data, and in writing the manuscript.
The authors declare that the research was conducted in the absence of any commercial or financial relationships that could be construed as a potential conflict of interest.
All claims expressed in this article are solely those of the authors and do not necessarily represent those of their affiliated organizations, or those of the publisher, the editors and the reviewers. Any product that may be evaluated in this article, or claim that may be made by its manufacturer, is not guaranteed or endorsed by the publisher.
The Supplementary Material for this article can be found online at: https://www.frontiersin.org/articles/10.3389/fgene.2024.1381690/full#supplementary-material
Beretta, V. M., Franchini, E., Din, I. U., Lacchini, E., Broeck, L. V. D., Sozzani, R., et al. (2023). The ALOG family members OsG1L1 and OsG1L2 regulate inflorescence branching in rice. Plant J. 115 (2), 351–368. doi:10.1111/tpj.16229
Cen, Q. W., Kang, L. H., Zhou, D. N., Zhang, X., Tian, Q., Zhang, X., et al. (2023). Genome-Wide identification and expression analysis of RCC1 gene family under abiotic stresses in rice (Oryza sativa L). Agronomy 13, 703. doi:10.3390/agronomy13030703
Chen, F., Zhou, Q., Wu, L., Li, F., Liu, B. J., Zhang, S. T., et al. (2019). Genome-wide identification and characterization of the ALOG gene family in Petunia. BMC Plant Biol. 19 (1), 600. doi:10.1186/s12870-019-2127-x
Chen, R. Z., Deng, Y. W., Ding, Y. L., Guo, J. X., Qiu, J., Wang, B., et al. (2022). Rice functional genomics: decades' efforts and roads ahead. Sci. China Life Sci. 65 (1), 33–92. doi:10.1007/s11427-021-2024-0
Gan, Z. C., Wu, X. X., Biahomba, S. A. M., Feng, T. T., Lu, X. M., Hu, N. B., et al. (2022). Genome-Wide identification, evolution, and expression characterization of the pepper (capsicum spp.) MADS-box gene family. Genes 13 (11), 2047. doi:10.3390/genes13112047
Iyer, L. M., and Aravind, L. (2012). ALOG domains: provenance of plant homeotic and developmental regulators from the DNA-binding domain of a novel class of DIRS1-type retroposons. Biol. Direct. 7, 39. doi:10.1186/1745-6150-7-39
Jumper, J., Evans, R., Pritzel, A., Green, T., Figurnov, M., Ronneberger, O., et al. (2021). Highly accurate protein structure prediction with AlphaFold. Nature 596 (7873), 583–589. doi:10.1038/s41586-021-03819-2
Lai, D. L., Fan, Y., Xue, G. X., He, A. L., Yang, H., He, C. L., et al. (2022). Genome-wide identification and characterization of the SPL gene family and its expression in the various developmental stages and stress conditions in foxtail millet (Setaria italica). BMC Genomics 23 (1), 389. doi:10.1186/s12864-022-08633-2
Lee, M., Dong, X. S., Song, H. Y., Yang, J. Y., Kim, S., and Hur, Y. (2020). Molecular characterization of Arabidopsis thaliana LSH1 and LSH2 genes. Genes Genomics 42 (10), 1151–1162. doi:10.1007/s13258-020-00985-x
Lescot, M., Déhais, P., Thijs, G., Marchal, K., Moreau, Y., Van de Peer, Y., et al. (2002). PlantCARE, a database of plant cis-acting regulatory elements and a portal to tools for in silico analysis of promoter sequences. Nucleic Acids Res. 30 (1), 325–327. doi:10.1093/nar/30.1.325
Li, N., Wang, Y., Lu, J., and Liu, C. (2019). Genome-Wide identification and characterization of the ALOG domain genes in rice. Int. J. Genomics 2019, 2146391. doi:10.1155/2019/2146391
Livak, K. J., and Schmittgen, T. D. (2001). Analysis of relative gene expression data using real-time quantitative PCR and the 2(-Delta Delta C(T)) Method. Methods 25 (4), 402–408. doi:10.1006/meth.2001.1262
MacAlister, C. A., Park, S. J., Jiang, K., Marcel, F., Bendahmane, A., Izkovich, Y., et al. (2012). Synchronization of the flowering transition by the tomato TERMINATING FLOWER gene. Nat. Genet. 44 (12), 1393–1398. doi:10.1038/ng.2465
Nan, W. Z., Shi, S. D., Jeewani, D. C., Quan, L., Shi, X., and Wang, Z. H. (2018). Genome-Wide identification and characterization of wALOG family genes involved in branch meristem development of branching head wheat. Genes 9 (10), 510. doi:10.3390/genes9100510
Peng, P., Liu, L. H., Fang, J. J., Zhao, J. F., Yuan, S. J., and Li, X. Y. (2017). The rice TRIANGULAR HULL1 protein acts as a transcriptional repressor in regulating lateral development of spikelet. Sci. Rep. 7 (1), 13712. doi:10.1038/s41598-017-14146-w
Poulter, R. T., and Goodwin, T. J. (2005). DIRS-1 and the other tyrosine recombinase retrotransposons. Genome Res. 110 (1-4), 575–588. doi:10.1159/000084991
Press, M. O., and Queitsch, C. (2017). Variability in a short tandem repeat mediates complex epistatic interactions in Arabidopsis thaliana. Genetics 205 (1), 455–464. doi:10.1534/genetics.116.193359
Ren, D. Y., Rao, Y. C., Wu, L. W., Xu, Q. K., Li, Z. Z., Yu, H. P., et al. (2016). The pleiotropic abnormal flower and DWARF1 affects plant height, floral development and grain yield in rice. J. Integr. Plant Biol. 58 (6), 529–539. doi:10.1111/jipb.12441
Reyes, V. P. (2023). Fantastic genes: where and how to find them? Exploiting rice genetic resources for the improvement of yield, tolerance, and resistance to a wide array of stresses in rice. Funct. Integr. Genomics 23 (3), 238. doi:10.1007/s10142-023-01159-0
Takanashi, H., Kajiya-Kanegae, H., Nishimura, A., Yamada, J., Ishimori, M., Kobayashi, M., et al. (2022). Dominant awn inhibitor encodes the ALOG protein originating from gene duplication and inhibits AWN elongation by suppressing cell proliferation and elongation in sorghum. Plant Cell Physiol. 63 (7), 901–918. doi:10.1093/pcp/pcac057
Takeda, S., Hanano, K., Kariya, A., Shimizu, S., Zhao, L., Matsui, M., et al. (2011). CUP-SHAPED COTYLEDON1 transcription factor activates the expression of LSH4 and LSH3, two members of the ALOG gene family, in shoot organ boundary cells. Plant J. 66 (6), 1066–1077. doi:10.1111/j.1365-313X.2011.04571.x
Turchetto, C., Silvério, A. D. C., Waschburger, E. L., Lacerda, M. E. G., Quintana, I. V., and Turchetto-Zolet, A. C. (2023). Genome-wide identification and evolutionary view of ALOG gene family in Solanaceae. Genet. Mol. Biol. 46 (3), e20230142. doi:10.1590/1415-4757-GMB-2023-0142
Varadi, M., Anyango, S., Deshpande, M., Nair, S., Natassia, C., Yordanova, G., et al. (2022). AlphaFold Protein Structure Database: massively expanding the structural coverage of protein-sequence space with high-accuracy models. Nucleic Acids Res. 50 (1), D439–D444. doi:10.1093/nar/gkab1061
Vo Phan, M. S., Keren, I., Tran, P. T., Lapidot, M., and Citovsky, V. (2023). Arabidopsis LSH10 transcription factor and OTLD1 histone deubiquitinase interact and transcriptionally regulate the same target genes. Commun. Biol. 6 (1), 58. doi:10.1038/s42003-023-04424-x
Walther, D., Brunnemann, R., and Selbig, J. (2007). The regulatory code for transcriptional response diversity and its relation to genome structural properties in A. thaliana. PLoS Genet. 3 (2), e11. doi:10.1371/journal.pgen.0030011
Wang, Y. P., Tang, H. B., Debarry, J. D., Tan, X., Li, J. P., Wang, X. Y., et al. (2012). MCScanX: a toolkit for detection and evolutionary analysis of gene synteny and collinearity. Nucleic Acids Res. 40 (7), e49. doi:10.1093/nar/gkr1293
Xu, W., Tang, W., Wang, C., Ge, L., Sun, J., Qi, X., et al. (2020). SiMYB56 confers drought stress tolerance in transgenic rice by regulating lignin biosynthesis and ABA signaling pathway. Front. Plant Sci. 11, 785. doi:10.3389/fpls.2020.00785
Yan, D. W., Zhou, Y., Ye, S. H., Zeng, L. J., Zhang, X. M., and He, Z. H. (2013). Beak-shaped grain 1/TRIANGULAR HULL 1, a DUF640 gene, is associated with grain shape, size and weight in rice. Sci. China Life Sci. 56 (3), 275–283. doi:10.1007/s11427-013-4449-5
Yoshida, A., Sasao, M., Yasuno, N., Takagi, K., Daimon, Y., Chen, R. H., et al. (2013). TAWAWA1, a regulator of rice inflorescence architecture, functions through the suppression of meristem phase transition. Proc. Natl. Acad. Sci. U. S. A. 110 (2), 767–772. doi:10.1073/pnas.1216151110
Yoshida, A., Suzaki, T., Tanaka, W., and Hirano, H. Y. (2009). The homeotic gene long sterile lemma (G1) specifies sterile lemma identity in the rice spikelet. Proc. Natl. Acad. Sci. U. S. A. 106 (47), 20103–20108. doi:10.1073/pnas.0907896106
Zhao, L., Nakazawa, M., Takase, T., Manabe, K., Kobayashi, M., Seki, M., et al. (2004). Overexpression of LSH1, a member of an uncharacterised gene family, causes enhanced light regulation of seedling development. Plant J. 37 (5), 694–706. doi:10.1111/j.1365-313x.2003.01993.x
Zhiguo, E., Zhang, Y. P., Li, T. T., Wang, L., and Zhao, H. M. (2015). Characterization of the ubiquitin-conjugating enzyme gene family in rice and evaluation of expression profiles under abiotic stresses and hormone treatments. PLoS One 10 (4), e0122621. doi:10.1371/journal.pone.0122621
Keywords: rice, ALOG family, expression analysis, inflorescence development, abiotic stress
Citation: Liu Z, Fan Z, Wang L, Zhang S, Xu W, Zhao S, Fang S, Liu M, Kofi SM, Zhang S, Kang N, Ai H, Li R, Feng T, Wei S and Zhao H (2024) Expression profiling of ALOG family genes during inflorescence development and abiotic stress responses in rice (Oryza sativa L.). Front. Genet. 15:1381690. doi: 10.3389/fgene.2024.1381690
Received: 04 February 2024; Accepted: 25 March 2024;
Published: 08 April 2024.
Edited by:
Mintao Sun, Chinese Academy of Agricultural Sciences (CAAS), ChinaReviewed by:
Min Tu, Wuhan Polytechnic University, ChinaCopyright © 2024 Liu, Fan, Wang, Zhang, Xu, Zhao, Fang, Liu, Kofi, Zhang, Kang, Ai, Li, Feng, Wei and Zhao. This is an open-access article distributed under the terms of the Creative Commons Attribution License (CC BY). The use, distribution or reproduction in other forums is permitted, provided the original author(s) and the copyright owner(s) are credited and that the original publication in this journal is cited, in accordance with accepted academic practice. No use, distribution or reproduction is permitted which does not comply with these terms.
*Correspondence: Heming Zhao, emhhb2htQGFoc3R1LmVkdS5jbg==; Shuya Wei, d2Vpc2h1eWExMDExQDEyNi5jb20=
Disclaimer: All claims expressed in this article are solely those of the authors and do not necessarily represent those of their affiliated organizations, or those of the publisher, the editors and the reviewers. Any product that may be evaluated in this article or claim that may be made by its manufacturer is not guaranteed or endorsed by the publisher.
Research integrity at Frontiers
Learn more about the work of our research integrity team to safeguard the quality of each article we publish.