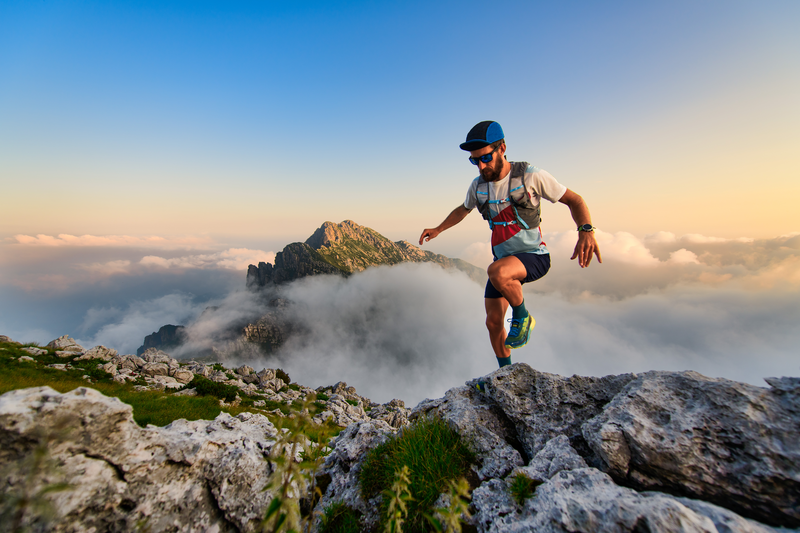
95% of researchers rate our articles as excellent or good
Learn more about the work of our research integrity team to safeguard the quality of each article we publish.
Find out more
ORIGINAL RESEARCH article
Front. Genet. , 20 November 2023
Sec. Genomics of Plants and the Phytoecosystem
Volume 14 - 2023 | https://doi.org/10.3389/fgene.2023.1274288
This article is part of the Research Topic Genetic Advancements for Improving the Plant Tolerance to Biotic and Abiotic Stresses View all 13 articles
Introduction: Soil salinization poses a significant challenge to plant growth and vitality. Plants like Tamarix ramosissima Ledeb (T. ramosissima), which are halophytes, are often integrated into planting schemes tailored for saline environments. Yet, the role of WRKY transcription factors in T. ramosissima, especially under sodium chloride (NaCl) stress mitigated by exogenous K+ application, is not well-understood. This research endeavors to bridge this knowledge gap.
Methods: Using Pfam protein domain prediction and physicochemical property analysis, we delved into the WRKY genes in T. ramosissima roots that are implicated in counteracting NaCl stress when aided by exogenous K+ applications. By observing shifts in the expression levels of WRKY genes annotated to the KEGG pathway under NaCl stress at 0, 48, and 168 h, we aimed to identify potential key WRKY genes.
Results: We found that the expression of 56 WRKY genes in T. ramosissima roots responded to exogenous K+ application during NaCl stress at the indicated time points. Particularly, the expression levels of these genes were primarily upregulated within 168 h. From these, 10 WRKY genes were found to be relevant in the KEGG pathways. Moreover, six genes, namely Unigene0024962, Unigene0024963, Unigene0010090, Unigene0007135, Unigene0070215, and Unigene0077293, were annotated to the Plant-pathogen interaction pathway or the MAPK signaling pathway in plants. These genes exhibited dynamic expression regulation at 48 h with the application of exogenous K+ under NaCl stress.
Discussion: Our research highlights that WRKY transcription factors can modulate the activation or inhibition of related genes during NaCl stress with the application of exogenous K+. This regulation enhances the plant’s adaptability to saline environments and mitigates the damage induced by NaCl. These findings provide valuable gene resources for future salt-tolerant Tamarix breeding and expand our understanding of the molecular mechanisms of WRKY transcription factors in alleviating NaCl toxicity.
Soil salinization poses a significant environmental issue worldwide. Reports indicate that approximately 8.31 × 106 km2 of the earth’s soil is affected by salinity (Li et al., 2014), and projections warn that due to escalating soil salinity, as much as 50% of the world’s arable land could be lost by mid-century. This exacerbation is predominantly caused by environmental degradation, global warming, and inappropriate irrigation practices (Ludwig et al., 2018). High salt levels are known to inhibit plant growth severely, a phenomenon first highlighted in Boyer’s 1984 study (Boyer, 1982). The ramifications on global agriculture are considerable, with an estimated economic toll exceeding 27 billion US dollars annually due to loss in productivity (Qadir et al., 2014). Therefore, devising strategies to utilize saline-alkaline land efficiently and promoting the development and cultivation of salt-resistant crops is of paramount importance (Savary et al., 2020).
Salt stress, the detrimental impact of highly saline soils on plant growth, affects many stages of a plant’s life, from seed germination to flowering and fruiting (Park et al., 2013). This often results in reduced germination rates, slower growth, decreased plant height, and leaf wilting (Hao et al., 2021). High Na+ concentrations in saline-alkali soils cause osmotic stress, obstructing the absorption of water and nutrients, leading to a nutrient-water imbalance (Mauro et al., 2022). Potassium (K+) is a key cation in plant cells, improving plant resilience to both abiotic stresses, such as salt, drought, and heavy metals, and biotic stresses like fungi (Leigh and Jones, 1984; Amtmann et al., 2008). High salt content in soil hinders K+ absorption due to competition with Na+, leading to a decrease in the K+/Na+ ratio, which results in excessive Na+ in plants, inhibiting growth and potentially causing plant death (Shabala and Cuin, 2008; Wang et al., 2019). Plant enrichment with K+ can enhance their water status, biomass, and salt tolerance (Tittal et al., 2021; Johnson et al., 2022). Under salt stress, the Na+ content in plants greatly exceeds K+ levels (Chakraborty et al., 2016). To prevent Na+ toxicity, plants must maintain a high K+/Na+ ratio to regulate the Na+/K+ balance (Chakraborty et al., 2016). Thus, boosting K+ absorption and minimizing Na+ accumulation in plants is a prime strategy against salt stress (Munns and Tester, 2008; Shabala and Cuin, 2008; Chen et al., 2022a).
Transcription factors (TFs) are also known as trans-acting factors and are specialized proteins that can regulate plant growth and development. Transcription factors can bind to specific DNA sequences (cis-acting elements) in the promoter region of target genes through their DNA-binding domains (DBD). This interaction allows them to control the specific expression of target genes in different tissues, cells, or under various environmental conditions in plants (Singh et al., 2002). Transcription factor families such as WRKY, V-myb avian myeloblastosis viral oncogene homolog (MYB), NAM, ATAF1/2 and CUC2 (NAC), Basic leucine zipper (bZIP), APETALA2/ethylene responsive factor (AP2/ERF), and Basic helix-loop-helix (bHLH) play pivotal regulatory and molecular switch roles in the intricate salt stress signaling network. By activating or suppressing the expression of specific genes or a set of genes, these transcription factors ensure their targeted expression. The products resulting from this gene expression further control the expression of downstream genes or directly act to protect plants from the damage induced by salt stress (Khan et al., 2018). It’s worth noting that WRKY transcription factors have been recognized over the past two decades as instrumental in regulating plant responses to biotic and abiotic stresses (Schluttenhofer and Yuan, 2015).
WRKY transcription factors are a plant-specific transcription factor family, which is one of the largest transcription factor families in higher plants (Ulker and Somssich, 2004; Cheng et al., 2021). These factors regulate gene transcription by identifying and binding to the W-box, a conserved DNA binding site. As a result, they play roles in various plant functions, including growth, development, defense against pathogens, responses to both biological and environmental stress, hormone signal processing, secondary metabolism, and other processes (Li and Zhou, 2014; Wei et al., 2022). WRKY transcription factors can also regulate the expression of related genes by binding cis-elements or interacting with other regulatory factors to play an important role in plant growth and development and resist abiotic stress (Wei et al., 2022). In particular, WRKY transcription factors can positively or negatively regulate the expression of related genes when plants are subjected to salt stress, thus making plants tolerant to salt. Dai et al. reported that Fortunella crassifolia FcWRKY40 can directly activate the expression of serine/threonine protein kinase gene FcSOS2 in SOS pathway, indirectly regulate the expression of FcSOS1 and FcSOS3 genes, promote Na+ effection, and positive response to salt stress (Dai et al., 2018). Research by Song and colleagues revealed that SbWRKY50, found in Sorghum, had a negative impact on the salt response by down-regulating the expression of AtSOS1, a Na+/H+ reverse transporter gene in Arabidopsis (Song et al., 2020). The overexpression of OsWRKY40 in rice decreased the tolerance of rice to high salt (Tao et al., 2011). GhWRKY25 is overexpressed and its tolerance to salt stress is enhanced, while in transgenic tobacco, it has relatively weak tolerance to drought stress (Liu et al., 2016).
As halophytes, Tamarix plants utilize their innate physiological and metabolic mechanisms to enhance their resistance to saline conditions (Gao et al., 2011; Gupta et al., 2014; Che et al., 2019; Bahramsoltani et al., 2020). Plants of the Tamarix plants have remarkable resilience to high salt environments, making them essential for restoring arid, desert, and saline regions (Yang et al., 2021; Sun et al., 2022). Recognizing their value, China has significantly invested in Tamarix cultivation as part of its ecological conservation initiatives. Additionally, Tamarix plants play a vital role in Chinese herbal medicine due to their medicinal properties (Duan et al., 2022). Studies by Flowers and Colmer indicate that halophytes can successfully complete their life cycle even when subjected to NaCl concentrations exceeding 200 mM (Flowers and Colmer, 2008; Liu et al., 2022). Typically, Tamarix ramosissima Ledeb (T. ramosissima), a representative of the Tamarix plants, is used as a restoration species in saline-alkali areas (Duan et al., 2022). T. ramosissima can sustain growth under NaCl stress up to concentrations of 100 mM, beyond which the plant’s growth is negatively impacted. Interestingly, NaCl concentrations exceeding 200 mM do not result in the plant’s death but significantly reduce its growth rate (Chen et al., 2023). However, halophytes like T. ramosissima have the ability to increase K+ retention when under salt stress, facilitating their adaptation to a range of saline and alkaline conditions (Garthwaite et al., 2005). Studies have discovered that the roots of T. ramosissima can resist NaCl stress using 10 mM KCl and can continue to grow even under 200 mM NaCl stress (Chen et al., 2022b; Chen et al., 2022c). In this manuscript, T. ramosissima was used as the research subject. We studied and analyzed the role of WRKY genes in T. ramosissima in response to NaCl stress when exogenous K+ is applied. By delving into the transcriptome, we identified key candidate WRKY genes and their essential metabolic pathways. This provides a scientific theoretical foundation for the selection of salt-tolerant varieties and the involvement of WRKY transcription factors in alleviating the toxic effects of NaCl.
Throughout this study, root samples of T. ramosissima under the stress of 200 mM NaCl with the application of exogenous K+ at 0, 48, and 168 h were collected for transcriptome sequencing. The data obtained underwent processing, including the screening of Differential expressed genes (DEGs) and database annotation, then identifing the WRKY-related genes. Using Pfam_Scan, protein domains of these genes were predicted (Finn et al., 2016). Protparam (https://web.expasy.org/protparam/) provided predictions on the basic physicochemical properties of the WRKY gene family members (Gasteiger et al., 2003), while CELLOv.2.5 (http://cello.life.nctu.edu.tw/) was employed for their subcellular localization. Subsequent analysis focused on the KEGG pathways annotated by the identified WRKY genes. A phylogenetic tree of the key WRKY candidate genes was then constructed. Lastly, the accuracy of the transcriptome data for these genes was validated using Quantitative Real-Time PCR (qRT-PCR) (Supplementary Figure S1).
The T. ramosissima with similar growth (seedling age: 5 months) were selected. They were cultivated in a 24-well hydroponic box with 1/2 Hoagland nutrient solution (replaced every 3 days), and the experimental treatments were applied 2 months later. The group with 1/2 Hoagland nutrient solution was set as the control group. The seedlings cultivated in 200 mM NaCl 1/2 Hoagland nutrient solution and 200 mM NaCl + 10 mM KCl 1/2 Hoagland nutrient solution were the treatment groups, with 8 plants per group (repeated 3 times). The nutrient solution was replaced every 3 days, with 24 seedlings for all treatments. At the same time, root samples were collected at 0, 48, and 168 h after treatment and stored in a −80°C freezer for transcriptome sequencing.
The collected T. ramosissima root samples were entrusted to biotechnology company (Gene Denovo, Guangzhou, China) for transcriptome sequencing. During the experiment, ultrasound was used to break down the mRNA. (Note: mRNA is Oligo (dT) magnetic beads enriched to eukaryotic mRNA with a polyA tail). The mRNA (fragmentation) is then used as the basis to synthesize the double strand of cDNA. The purified double-stranded cDNA was screened out using about 200bp and PCR amplification was performed. Finally, the database was constructed using PCR products, which were purified by AMPure XP beads Chen et al., 2022b). The raw data obtained (WRKY genes in the roots of T. ramosissima - NCBI Short Reads Archive database: SRP356215) was analyzed. DEGs were identified based on the criteria of FDR < 0.05 (corrected p-value) and |log2FC| > 1. The DEGs were annotated to the Gene Ontology (GO) (Conesa et al., 2005) and Kyoto Encyclopedia of Genes and Genomes (KEGG) (Michael et al., 2000) databases.
Through transcriptome sequencing results, members of the WRKY family annotated in the NCBI database were obtained from the roots of the T. ramosissima. Then, the obtained WRKY proteins were matched in the Pfam database using the Pfam_Scan program, yielding protein structure-related annotation information for the WRKY genes (Finn et al., 2016), and the final members of the WRKY gene family were obtained. The basic physicochemical properties of the members of the WRKY gene family were analyzed and predicted online using Protparam (https://web.expasy.org/protparam/), including Molecular weight, Theoretical pI, Grand average of hydropathicity (GRAVY), Instability index, and Aliphatic index, etc. Finally, the obtained WRKY proteins were subjected to subcellular localization prediction analysis using the online website CELLOv.2.5 (http://cello.life.nctu.edu.tw/).
Seven WRKY candidate genes were randomly chosen to validate the transcriptome sequencing accuracy. Total RNA from T. ramosissima root samples was extracted using the RNAprep pure kit (Tiangeng, Beijing, China), and cDNA was synthesized with the Prime Script™ DEG II 1st Strand cDNA Synthesis Kit (Takara, Beijing, China). Primers were designed for the above WRKY genes (Supplementary Table S1). However, after diluting the cDNA template 8 times, qRT-PCR experiments were conducted according to the instructions of the TB Green Premix ExTaq (Tli RNaseH Plus) kit (Takara, RR420A) by Takara company. The reaction system was set to 20 μL, including 10 μL TB Green Premix Ex Taq, 0.8 μL upstream primer (10 μmol L−1), 0.8 μL downstream primer (10 μmol L−1), 1 μL DNA template, and 7.4 μL dd H2O. Three replicates were set for each sample, with all operations performed on ice. Amplification of each sample was executed using the ABI ViiA™ 7 Real-time PCR system (ABI, California, United States). The PCR amplification protocol included an initial denaturation at 95°C for 30 s, followed by 40 cycles of denaturation at 95°C for 5 s and annealing at 60°C for 30 s. The melting curve process involved heating at 95°C for 5 s, then 60°C for 1 min, gradually increasing to 95°C, and finally cooling to 50°C for 30 s (Yang et al., 2022). Each gene was biologically replicated 3 times, with Tubulin as the internal reference gene. The 2−ΔΔCT method was used to calculate the relative expression levels (Livak and Schmittgen, 2001).
Data computations such as mean and standard deviations were performed using Microsoft Excel (Microsoft, Washington, United States). The prediction of Pfam protein structural domains was made through the Pfam_Scan program. The construction of graphs was facilitated by the Origin 2019 software (OriginLab, Massachusetts, United States). Phylogenetic trees were developed utilizing the MEGA 11 software (MEGA software, Pennsylvania, United States). Lastly, LSD analysis was executed using the ANOVA tool in the SPSS 26.0 software (SPSS, New York, United States).
The transcript data results revealed 61 WRKY-related genes. Subsequent predictions of these 61 WRKY gene protein sequences in the Pfam database (Supplementary Table S3) showed that 5 WRKY genes (Unigene0009588, Unigene0016969, Unigene0023528, Unigene0052366, and Unigene0062497) were not present in the Pfam database The results show that the start-to-end positions of the structure domains of the Unigene-encoded protein sequences predicted by the HMM models of these 56 WRKY genes are 1-489, the HMM length is all 21-59, the bit score is between 23.7-96.4, the HMM acc is PF03106.15, the clan is CL0274, and the PfamA_definition is WRKY DNA-binding domain. Finally, there are 56 WRKY genes present in the roots of the T. ramosissima under the exogenous NaCl stress.
The Protparam online tool was used to analyze the physicochemical properties of the 56 WRKY genes, ande the 56 WRKY genes were analyzed by clustering heat map with each sample (Figure 1). The results show (Table 1) that the number of amino acids ranges from 39 to 728 aa, indicating a large variation in the number of amino acids; the molecular weight ranges from 4385.91 (Unigene0075549) to 69860.14 (Unigene0032744) Da, which is proportional to the amino acid content; Theoretical pI ranges from 4.70 (Unigene0077293) to 10.26 (Unigene0041792), among which 27 members are basic proteins (pI > 7), and 29 members are acidic proteins (pI < 7); only 4 WRKY proteins have an Instability index less than 40, indicating that most of them are unstable proteins. The results of hydrophilicity/hydrophobicity of the WRKY proteins obtained are all less than 0, indicating that these 56 WRKY proteins are hydrophilic. The subcellular prediction results show that all WRKY proteins are located in the nuclear.
FIGURE 1. Clustering heatmap of the WRKY candidate genes (A heatmap clustering analysis was performed on the 56 identified WRKY candidate genes across various samples).
According to our focus on the expression levels of 56 WRKY genes (Supplementary Figure S2), five genes (Unigene0066131, Unigene0061329, Unigene0053897, Unigene0025290, and Unigene0101998) showed an initial upregulation followed by downregulation after 48 and 168 h of NaCl stress. However, their expression levels were first downregulated and then upregulated after 48 and 168 h of exogenous K+ application under NaCl stress. Another ten genes (Unigene0024962, Unigene00249623, Unigene0079543, Unigene0104866, Unigene0094842, Unigene0078949, Unigene0078678, Unigene0075549, Unigene0065429, and Unigene0033084) showed initial upregulation followed by downregulation under NaCl stress at 48 and 168 h. However, they consistently exhibited an upregulated trend in their expression levels after 48 and 168 h of exogenous K+ application under NaCl stress. Notably, Unigene0090616 showed an upregulated trend in expression levels, whether it was under NaCl stress at 48 and 168 h or under NaCl stress with exogenous K+ application at 48 and 168 h.
Ten DEGs of the 56 WRKY genes found in the roots of T. ramosissima were annotated in the related KEGG pathway (Supplementary Table S2) when exogenous K+ was applied at 0, 48 and 168 h under NaCl stress. 6 WRKY genes (Unigene0007135, Unigene0010090, Unigene0014405, Unigene0052215, Unigene0070215 and Unigene0077293) were annotated in the plant-interaction pathway (ko04626) and the MAPK signaling pathway-plant pathway (ko04016), The 6 WRKY genes were classified into Organismal Systems, and the Environmental Information Processing in KEGG A. KEGG B classified Environmental adaptation and Signal transduction. Unigene0024962, Unigene0024963, Unigene0079542 and Unigene0079543 are annotated for the Plant-pathogen interaction pathway (ko04626). In addition, these 4 WRKY genes were classified into Organismal Systems in KEGG A and Environmental adaptation in KEGG B. According to the expression levels of the 10 WRKY genes at 0, 48 and 168 h under NaCl stress (Supplementary Figure S2), The expression levels of 3 WRKY genes (Unigene0024962, Unigene0024963, and Unigene0079543) increased at 48 h and 168 h when applying exogenous K+ under NaCl stress. Notably, the expression levels of Unigene0024962 and Unigene0024963 consistently rose at both 48 and 168 h with exogenous K+ under NaCl stress. However, under NaCl stress alone, their expression levels first increased at 48 h and then decreased at 168 h.
As inferred from the analysis of the Plant-pathogen interaction pathway (Table 2), In the 200 mM NaCl-48 h vs. 200 mM NaCl + 10 mM KCl-48 h (N-48 h vs. N + K-48 h) comparison group, 99 DEGs were significantly enriched into the Plant-pathogen interaction pathway (p < 0.05). Among them, 65 DEGs were upregulated, while 34 were downregulated. The 7 WRKY genes (Unigene0052215, Unigene0010090, Unigene0007135, Unigene0070215 Unigene0077293, Unigene0024962 and Unigene00249623) have been annotated for Plant-pathogen interaction pathway in N-48 h vs. N + K-48 h comparison group, and the expression level changed (Supplementary Figure S3). In addition, Unigene0052215, Unigene0024962, and Unigene00249623 were down-regulated, while Unigene0010090, Unigene0007135, Unigene0070215, and Unigene0077293 were upregulated, suggesting their roles in NaCl stress resistance. In the comparison of the 200 mM NaCl-168 h and 200 mM NaCl + 10 mM KCl-168 h groups, 74 DEGs were identified in the Plant-pathogen interaction pathway. Of these, 21 DEGs were upregulated and 53 were downregulated. Notably, 3 WRKY genes (Unigene0070215, Unigene0024962, and Unigene00249623) were associated with this pathway. The expression level of Unigene0070215 was downregulated, while the expression levels of Unigene0024962 and Unigene00249623 were upregulated.
Based on the MAPK signaling pathway-plant pathway (Table 3), in the 200 mM NaCl-48 h vs. 200 mM NaCl + 10 mM KCl-48 h comparison group, 57 DEGs were enriched to MAPK signaling Pathway-plant pathway. Among them, 37 DEGs showed upregulated expression levels, while 20 DEGs exhibited downregulated expression. Unigene0052215, Unigene0010090, Unigene0007135, Unigene0070215 and Unigene0077293 belong to WRKY genes that have been annotated to MAPK signaling the N-48 h vs. N + K-48 h comparison group Pathway-plant pathway, and the expression level was changed (Supplementary Figure S4). Among them, in addition to the downregulated expression of Unigene0052215, the upregulated expression of Unigene0010090, Unigene0007135, Unigene0070215 and Unigene0077293 actively participate in the resistance to NaCl stress. In the N-168 h vs. N + K-168 h comparison group, 54 DEGs were significantly enriched to MAPK signaling Pathway-plant pathway (p < 0.05). Among them, 19 DEGs showed upregulated expression levels, while 35 DEGs exhibited downregulated expression. Only Unigene0070215 belongs to the WRKY gene and has been annotated to MAPK signaling pathway-plant in N-168 h vs. N + K-168 h comparison group pathway, and its expression level was still downregulated.
Unigene0024962 and Unigene00249623, which belong to the WRKY gene family (WRKY transcription factor 1), have both been annotated in the Plant-pathogen interaction pathway. Under NaCl stress, their expression levels initially increase at 48 h and then decrease at 168 h. However, after exogenous K+ application under NaCl stress, their expression levels continue to show an increasing trend at both 48 and 168 h. Thus, it can be inferred that Unigene0024962 and Unigene00249623 are key candidate genes in the WRKY family. The protein amino acid sequences of Unigene0024962 and Unigene0024963 were compared on the NCBI using Blast, resulting in 15 homologous species were selected (Supplementary Table S4). A phylogenetic tree constructed with MEGA software revealed that Unigene0024962 shares a close phylogenetic relationship with Tamarix hispida (Supplementary Figure S5).
Seven WRKY candidate genes were selected for qRT-PCR validation to verify the accuracy of our transcriptome sequencing outcomes. The patterns observed in qRT-PCR expression closely matched those from the transcriptomic study (Supplementary Figure S6). This affirms the integrity and dependability of the transcriptome data acquired. Hence, this research lays a solid foundation for identifying WRKY genes in T. ramosissima roots that enhance salinity resilience and mitigate the adverse impacts of NaCl stress.
Tamarix plants has developed a complex regulatory network to adapt to abiotic stress (Zhu, 2016). Transcription factors are the most important regulatory factors in all abiotic stress responses (Yao et al., 2016). The WRKY family is large, Arabidopsis thaliana had 74 WRKY genes (Eulgem et al., 2000), cotton had 116 WRKY genes (Dou et al., 2014), rice (Oryza sativa) had 102 WRKY genes (Ross et al., 2007), Solanum Lycopersicum had 81 WRKY genes (Huang et al., 2012), carrot (Daucus carota L.) had 95 WRKY genes (Li et al., 2016), and Castor Bean (Ricinus communis L.) had 58 WRKY genes (Zou et al., 2016). In this study, 56 WRKY genes were found in the roots of T. ramosissima under NaCl stress at 48 h and 168 h. Further analysis of these 56 WRKY genes can help to understand the response mechanism of T. ramosissima to NaCl stress under exogenous K+ application. To identify salt tolerance genes and related metabolic pathways to provide a scientific and theoretical basis for further research on salt tolerance in T. ramosissima.
WRKY transcription factors are essential in plant responses to biotic and abiotic stresses (Jiang et al., 2017). Particularly, it plays a crucial role in responding to salt stress in various plants (Huang et al., 2022). A WRKY transcription factor PbWRKY40 from Pyrus betulaefolia functions positively in salt tolerance and modulating organic acid accumulation by regulating PbVHA-B1 expression (Lin et al., 2022). Ahwrky75 was overexpressed in the M34 mutant of “salt-tolerant” peanut, which improved the salt tolerance of transgenic peanut (Zhu et al., 2021). In transgenic tobacco, overexpression of the WRKY transcription factor gene of Begonia (MbWRKY5) can improve drought tolerance and salt tolerance (Han et al., 2018). in Solanum lycopersicum, SlWRKY8 plants overexpressed showed tolerance to salt stress (Gao et al., 2020). In this study, 37 DEGs were upregulated, and 24 DEGs were downregulated in the N-48 h vs. N + K-48 h comparison group. In the N-168 h vs. N + K-168 h comparison group, 33 DEGs were upregulated, and 28 DEGs were downregulated. Additionally, overexpression of the WRKY gene DgWRKY1 or DgWRKY3 in chrysanthemum plants increased their salt tolerance by dendranthema grandiflorum (Liu et al., 2013). However, K+ can better regulate Na+ uptake under salt conditions, which plays a vital role in regulating plant physiological processes to enable plants to survive under stress conditions, especially in improving their tolerance to salt stress (Çolpan et al., 2013). Furthermore, the introduction of K+ helped mitigate the negative impacts of Na+, enhanced the uptake of K+, and raised the K+/Na+ ratio under conditions of NaCl stress (Carden et al., 2003). It is worth noting that halophytes have a solid competitive advantage in maintaining K+ stability under a high NaCl stress (Ardie et al., 2009). In this study, expression level at 48 and 168 h increased first and then decreased under NaCl stress. However, the expression level of the candidate key WRKY genes (Unigene0024962 and Unigene0024963) were upregulated after the application of exogenous K+ for 48 and 168 h under NaCl stress. Therefore, the increased expression level of Unigene0024962 and Unigene0024963 may be influenced by the addition of exogenous K+. In brief, the results showed that WRKY genes expression in the roots of T. ramosissima was consistently upregulated within 168 h after applying exogenous NaCl stress, which improved the tolerance and resistance of T. ramosissima to NaCl stress.
Under conditions of salt stress in grapes (Vitis vinifera), VvWRKY28 has the ability to boost the accumulation levels of enzymatic antioxidants (superoxide dismutase, peroxidase, and catalase) as well as proline. These changes counteract the over-accumulation of reactive oxygen species and support osmotic balance in cells, thus increasing the plant’s overall salt tolerance (Liu et al., 2022). In T. ramosissima, when exposed to 200 mM NaCl stress for 48 h and 168h, the leaves had more activity of certain helpful enzymes. At the same time, there was more proline in the roots. These changes helped the seeds handle salt better. (Chen et al., 2022b; Chen et al., 2022c). In this study, the expression level of the WRKY gene (Unigene0020906) was upregulated after applying exogenous K+ for 48 and 168 h under 200 mM NaCl stress. Therefore, it may play an essential role in increasing the main antioxidant activities and proline content accumulation of T. ramosissima. Besides, abiotic stresses such as high temperature, salinity and drought affect plant growth. They also affect plant defense response to pathogens through enhancement or inhibition of pathogens, to regulate plant response to pathogen infection (Pandey and Senthil-Kumar, 2019). In particular, one of the earliest signaling events after a plant sense an invading pathogen is the activation of mitogen activated protein kinase (MAPK) signaling cascades. They are able to correlate the perception of external stimuli with cellular responses, and MAPK signaling networks play specific and overlapping roles in controlling the activity of a large number of transcription factors, enzymes, hormones, peptides, and antibacterial chemicals, among others (Taj et al., 2014). WRKY transcription factors have been shown to regulate plant defense responses (Rushton and Somssich, 1998). WRKY transcription factors regulate plant defense responses (Rushton and Somssich, 1998). Their activity is modulated by phosphorylation through mitogen-activated protein kinase (Ishihama and Yoshioka, 2012; Adachi et al., 2015). WRKY protein is involved in Arabidopsis thaliana response to bacterial flagellin flg22 as a transcriptional activator at the end of the PAMP signaling cascade. Flagellin flg22 in Arabidopsis thaliana initiates the entire MEKK1-MKK4/5-MAPK3/6 MAPK cascade pathway, leading to the activation of WRKY22/29 expressions. These expressions function after the flagellin receptor FLS2, a leucine-rich repeat (LRR) receptor kinase, and further stimulate MAPK cascades, enhancing plant resistance (Sheen et al., 2002). Simultaneously, AtMKK1 and AtMKK2 in Arabidopsis Thaliana are also associated with biological and abiotic stress responses as part of a signaling cascade that includes MEKK1 and MPK4 (Teige et al., 2004). Nevertheless, AtMKK2 is also involved in mediating salt signaling (Hadiarto et al., 2006). It is worth noting that Mkk1 is a positive regulator of flg22 activation of MPK3, MPK4, MPK6, and a negative regulator of flg22 activation of gene expression, and its role is very complex (Meszaros et al., 2006). MPK3 and MPK6 are two mitogen-activated protein kinases (MAPK or MPK) that play key roles in plant resistance by regulating various defense responses (Meng et al., 2013). AtWRKY33 mutants in Arabidopsis Thaliana are very sensitive to NaCl, and overexpression of AtWRKY33 can enhance tolerance to NaCl stress (Jiang and Deyholos, 2009; Li et al., 2011). In this study, FLS2 activated downstream mitogen-activated protein kinase (MEKK) in both the Plant-pathogen interaction pathway and the MAPK signaling Pathway-plant pathway, MKK1/MKK2 → MPK4 or MKK4/MKK5 → MPK3/MPK6 (+p is the process of protein phosphorylation). They transmit these signals into the nucleus to transcription factors (WRKY 22/WRKY29 or WRKY25/WRKY33) that activate the transcription of genes associated with immune defense. The expression levels of Unigene0010090, Unigene0007135, Unigene0070215 and Unigene0077293 were upregulated in the N-48 h vs. N + K-48 h comparison group. In the comparison group of N-168 h vs. N + K-168 h, the expression levels of Unigene0024962 and Unigene0024963 were upregulated. Therefore, it is concluded that these genes actively participate in the resistance to NaCl stress when exogenous K+ is applied for 48 h under NaCl stress. However, with the increase of K+ addition time, the damage of NaCl stress was alleviated, T. ramosissima could maintain normal growth, and the genes involved in regulation were reduced.
To conclude, the results of this manuscript suggest that the result of this manuscript suggest that the WRKY transcription factors are involved in T. ramosissima‘s response to external K+ under NaCl stress. A significant number of WRKY-related genes are upregulated and take part in essential metabolic pathways, thereby creating a comprehensive defense mechanism to help T. ramosissima resist NaCl stress. Notably, two WRKY candidate genes, Unigene0024962 and Unigene00249623, play pivotal roles within these pathways. They are identified as key WRKY genes worthy of further investigation.
The WRKY transcription factor family is one of the largest transcriptional regulatory families in higher plants, involved in regulating plant growth and development, and responding to biotic and abiotic stresses. They play an indispensable role in the normal life activities of plants, being involved in various aspects of the plant’s lifecycle. In this study, the roots of T. ramosissima, under exogenous potassium in response to salt stress at 48 h and 168 h, saw the involvement of the WRKY transcription factor family in the growth and development processes of T. ramosissima, as well as the construction of its defense system. A significant number of WRKY genes actively upregulated their expression levels to resist NaCl stress through the plant-pathogen interaction pathway and the MAPK signaling pathway-plant pathway, alleviating the damage caused by NaCl and thus maintaining the normal growth of T. ramosissima. Notably, two key candidate WRKY genes (Unigene0024962 and Unigene00249623) actively upregulated their expression levels in the plant-pathogen interaction pathway after exogenous potassium application under NaCl stress, playing a crucial role in the T. ramosissima root system’s mitigation of NaCl stress. They can serve as key salt tolerance genes in the Tamarix plants for further exploration.
The datasets presented in this study can be found in online repositories. The names of the repository/repositories and accession number(s) can be found below: https://www.ncbi.nlm.nih.gov/, SRP356215.
YC: Data curation, Investigation, Project administration, Resources, Software, Writing–review and editing, Methodology, Validation, Conceptualization, Formal Analysis, Visualization, Writing–original draft. XZ: Data curation, Investigation, Methodology, Writing–original draft. YF: Software, Writing–review and editing, Data curation. DS: Project administration, Writing–review and editing, Funding acquisition. JJ: Data curation, Software, Writing–review and editing, Investigation, Resources, Supervision. LW: Investigation, Project administration, Resources, Validation, Writing–review and editing, Funding acquisition, Supervision.
The authors declare financial support was received for the research, authorship, and/or publication of this article. This work was jointly supported by grants from Jiangsu Coastal Tidal Spartina alterniflora Loisel Comprehensive Management Technology Innovation and Demonstration (Su[2023]TG10).
The authors declare that the research was conducted in the absence of any commercial or financial relationships that could be construed as a potential conflict of interest.
The authors declared that they were an editorial board member of Frontiers, at the time of submission. This had no impact on the peer review process and the final decision
All claims expressed in this article are solely those of the authors and do not necessarily represent those of their affiliated organizations, or those of the publisher, the editors and the reviewers. Any product that may be evaluated in this article, or claim that may be made by its manufacturer, is not guaranteed or endorsed by the publisher.
The Supplementary Material for this article can be found online at: https://www.frontiersin.org/articles/10.3389/fgene.2023.1274288/full#supplementary-material
Adachi, H., Nakano, T., Miyagawa, N., Ishihama, N., Yoshioka, M., Katou, Y., et al. (2015). WRKY transcription factors phosphorylated by MAPK regulate a plant immune NADPH oxidase in Nicotiana benthamiana. Plant Cell 27, 2645–2663. doi:10.1105/tpc.15.00213
Amtmann, A., Troufflard, S., and Armengaud, P. (2008). The effect of potassium nutrition on pest and disease resistance in plants. Physiol. Plant 133, 682–691. doi:10.1111/j.1399-3054.2008.01075.x
Ardie, S. W., Xie, L., Takahashi, R., Liu, S., and Takano, T. (2009). Cloning of a high-affinity K+ transporter gene PutHKT2;1 from Puccinellia tenuiflora and its functional comparison with OsHKT2;1 from rice in yeast and Arabidopsis. J. Exp. Bot. 60, 3491–3502. doi:10.1093/jxb/erp184
Bahramsoltani, R., Kalkhorani, M., Abbas, Z. S., Farzaei, M. H., and Rahimi, R. (2020). The genus Tamarix: traditional uses, phytochemistry, and pharmacology. J. Ethnopharmacol. 246, 112245. doi:10.1016/j.jep.2019.112245
Boyer, J. S. (1982). Plant productivity and environment. Science 218, 443–448. doi:10.1126/science.218.4571.443
Carden, D. E., Walker, D. J., Flowers, T. J., and Miller, A. J. (2003). Single-cell measurements of the contributions of cytosolic Na(+) and K(+) to salt tolerance. Plant Physiol. 131, 676–683. doi:10.1104/pp.011445
Chakraborty, K., Bhaduri, D., Meena, H. N., and Kalariya, K. (2016). External potassium K(+) application improves salinity tolerance by promoting Na(+)-exclusion, K(+)-accumulation and osmotic adjustment in contrasting peanut cultivars. Plant Physiol. Biochem. 103, 143–153. doi:10.1016/j.plaphy.2016.02.039
Che, B., Cheng, C., Fang, J., Liu, Y., Jiang, L., and Yu, B. (2019). The recretohalophyte Tamarix TrSOS1 gene confers enhanced salt tolerance to transgenic hairy root composite cotton seedlings exhibiting Virus-Induced gene silencing of GhSOS1. Int. J. Mol. Sci. 20, 2930. doi:10.3390/ijms20122930
Chen, Y., Li, H., Zhang, S., Du, S., Zhang, J., Song, Z., et al. (2023). Analysis of the main antioxidant enzymes in the roots of Tamarix ramosissima under NaCl stress by applying exogenous potassium (K+). Front. Plant Sci. 14, 1114266. doi:10.3389/fpls.2023.1114266
Chen, Y., Zhang, S., Du, S., Jiang, J., and Wang, G. (2022a). Transcriptome and metabonomic analysis of Tamarix ramosissima potassium (K+) Channels and transporters in response to NaCl Stress. Genes 13, 1313. doi:10.3390/genes13081313
Chen, Y., Zhang, S., Du, S., Zhang, X., Jiang, J., and Wang, G. (2022b). Analysis of amino acids in the roots of Tamarix ramosissima by application of exogenous potassium (K+) under NaCl Stress. Int. J. Mol. Sci. 23, 9331. doi:10.3390/ijms23169331
Chen, Y., Zhang, S., Du, S., Zhang, X., Wang, G., Huang, J., et al. (2022c). Effects of exogenous potassium (K+) application on the antioxidant enzymes activities in leaves of Tamarix ramosissima under NaCl Stress. Genes 13, 1507. doi:10.3390/genes13091507
Cheng, Z., Luan, Y., Meng, J., Sun, J., Tao, J., and Zhao, D. (2021). WRKY transcription factor response to High-Temperature stress. Plants (Basel) 10, 2211. doi:10.3390/plants10102211
Çolpan, E., Zengin, M., and Özbahçe, A. (2013). The effects of potassium on the yield and fruit quality components of stick tomato. Hortic. Environ. Biotechnol. 54, 20–28. doi:10.1007/s13580-013-0080-4
Conesa, A., Gotz, S., Garcia-Gomez, J. M., Terol, J., Talon, M., and Robles, M. (2005). Blast2GO: a universal tool for annotation, visualization and analysis in functional genomics research. Bioinformatics 21, 3674–3676. doi:10.1093/bioinformatics/bti610
Dai, W., Wang, M., Gong, X., and Liu, J. H. (2018). The transcription factor FcWRKY40 of Fortunella crassifolia functions positively in salt tolerance through modulation of ion homeostasis and proline biosynthesis by directly regulating SOS2 and P5CS1 homologs. New Phytol. 219, 972–989. doi:10.1111/nph.15240
Dou, L., Zhang, X., Pang, C., Song, M., Wei, H., Fan, S., et al. (2014). Genome-wide analysis of the WRKY gene family in cotton. Mol. Genet. Genomics 289, 1103–1121. doi:10.1007/s00438-014-0872-y
Duan, Q., Zhu, Z., Wang, B., and Chen, M. (2022). Recent progress on the salt tolerance mechanisms and application of tamarisk. Int. J. Mol. Sci. 23, 3325. doi:10.3390/ijms23063325
Eulgem, T., Rushton, P. J., Robatzek, S., and Somssich, I. E. (2000). The WRKY superfamily of plant transcription factors. Trends Plant Sci. 5, 199–206. doi:10.1016/s1360-1385(00)01600-9
Finn, R. D., Coggill, P., Eberhardt, R. Y., Eddy, S. R., Mistry, J., Mitchell, A. L., et al. (2016). The Pfam protein families database: towards a more sustainable future. Nucleic Acids Res. 44, D279–D285. doi:10.1093/nar/gkv1344
Flowers, T. J., and Colmer, T. D. (2008). Salinity tolerance in halophytes. New Phytologist 179, 945–963. doi:10.1111/j.1469-8137.2008.02531.x
Gao, C., Wang, Y., Jiang, B., Liu, G., Yu, L., Wei, Z., et al. (2011). A novel vacuolar membrane H+-ATPase c subunit gene (ThVHAc1) from Tamarix hispida confers tolerance to several abiotic stresses in Saccharomyces cerevisiae. Mol. Biol. Rep. 38, 957–963. doi:10.1007/s11033-010-0189-9
Gao, Y. F., Liu, J. K., Yang, F. M., Zhang, G. Y., Wang, D., Zhang, L., et al. (2020). The WRKY transcription factor WRKY8 promotes resistance to pathogen infection and mediates drought and salt stress tolerance in Solanum lycopersicum. Physiol. Plant 168, 98–117. doi:10.1111/ppl.12978
Garthwaite, A. J., von Bothmer, R., and Colmer, T. D. (2005). Salt tolerance in wild Hordeum species is associated with restricted entry of Na+ and Cl- into the shoots. J. Exp. Bot. 56, 2365–2378. doi:10.1093/jxb/eri229
Gasteiger, E., Gattiker, A., Hoogland, C., Ivanyi, I., Appel, R. D., and Bairoch, A. (2003). ExPASy: the proteomics server for in-depth protein knowledge and analysis. Nucleic Acids Res. 31, 3784–3788. doi:10.1093/nar/gkg563
Gupta, B., Huang, B., and Catuvelli, L. (2014). Mechanism of salinity tolerance in plants: physiological, biochemical, and molecular characterization. Int. J. Genomics 2014, 1–18. doi:10.1155/2014/701596
Hadiarto, T., Nanmori, T., Matsuoka, D., Iwasaki, T., Sato, K., Fukami, Y., et al. (2006). Activation of Arabidopsis MAPK kinase kinase (AtMEKK1) and induction of AtMEKK1-AtMEK1 pathway by wounding. Planta 223, 708–713. doi:10.1007/s00425-005-0126-7
Han, D., Hou, Y., Wang, Y., Ni, B., Li, Z., and Yang, G. (2018). Overexpression of a Malus baccata WRKY transcription factor gene (MbWRKY5) increases drought and salt tolerance in transgenic tobacco. Can. J. Plant Sci. 99, 173–183. doi:10.1139/cjps-2018-0053
Hao, S., Wang, Y., Yan, Y., Liu, Y., Wang, J., and Chen, S. (2021). A review on plant responses to salt stress and their mechanisms of salt resistance. Horticulturae 7, 132. doi:10.3390/horticulturae7060132
Huang, J., Liu, F., Chao, D., Xin, B., Liu, K., Cao, S., et al. (2022). The WRKY transcription factor OsWRKY54 is involved in salt tolerance in rice. Int. J. Mol. Sci. 23, 11999. doi:10.3390/ijms231911999
Huang, S., Gao, Y., Liu, J., Peng, X., Niu, X., Fei, Z., et al. (2012). Genome-wide analysis of WRKY transcription factors in Solanum lycopersicum. Mol. Genet. Genomics MGG 287, 495–513. doi:10.1007/s00438-012-0696-6
Ishihama, N., and Yoshioka, H. (2012). Post-translational regulation of WRKY transcription factors in plant immunity. Curr. Opin. Plant Biol. 15, 431–437. doi:10.1016/j.pbi.2012.02.003
Jiang, J., Ma, S., Ye, N., Jiang, M., Cao, J., and Zhang, J. (2017). WRKY transcription factors in plant responses to stresses. J. Integr. Plant Biol. 59, 86–101. doi:10.1111/jipb.12513
Jiang, Y., and Deyholos, M. K. (2009). Functional characterization of Arabidopsis NaCl-inducible WRKY25 and WRKY33 transcription factors in abiotic stresses. Plant Mol. Biol. 69, 91–105. doi:10.1007/s11103-008-9408-3
Johnson, R., Vishwakarma, K., Hossen, M. S., Kumar, V., Shackira, A. M., Puthur, J. T., et al. (2022). Potassium in plants: growth regulation, signaling, and environmental stress tolerance. Plant Physiol. Biochem. 172, 56–69. doi:10.1016/j.plaphy.2022.01.001
Khan, S. A., Li, M. Z., Wang, S. M., and Yin, H. J. (2018). Revisiting the role of plant transcription factors in the battle against abiotic stress. Int. J. Mol. Sci. 19, 1634. doi:10.3390/ijms19061634
Leigh, R. A., and Jones, R. G. W. (1984). A hypothesis relating critical potassium concentrations for growth to the distribution and functions of this ion in the plant cell. New Phytol. 97, 1–13. doi:10.1111/j.1469-8137.1984.tb04103.x
Li, J., Pu, L., Han, M., Zhu, M., Zhang, R., and Xiang, Y. (2014). Soil salinization research in China: advances and prospects. J. Geogr. Sci. 24, 943–960. doi:10.1007/s11442-014-1130-2
Li, K., and Zhou, C. J. (2014). Research progress in WRKY transcription factors in plants. Plant Physiology J. 50, 1329–1335. doi:10.13592/j.cnki.ppj.2014.1019
Li, M. Y., Xu, Z. S., Tian, C., Huang, Y., Wang, F., and Xiong, A. S. (2016). Genomic identification of WRKY transcription factors in carrot (Daucus carota) and analysis of evolution and homologous groups for plants. Sci. Rep. 6, 23101–23117. doi:10.1038/srep23101
Li, S., Fu, Q., Chen, L., Huang, W., and Yu, D. (2011). Arabidopsis thaliana WRKY25, WRKY26, and WRKY33 coordinate induction of plant thermotolerance. Planta 233, 1237–1252. doi:10.1007/s00425-011-1375-2
Lin, L., Yuan, K., Huang, Y., Dong, H., Qiao, Q., Xing, C., et al. (2022). A WRKY transcription factor PbWRKY40 from Pyrus betulaefolia functions positively in salt tolerance and modulating organic acid accumulation by regulating PbVHA-B1 expression. Environ. Exp. Bot. 196, 104782. doi:10.1016/j.envexpbot.2022.104782
Liu, J. N., Fang, H., Liang, Q., Dong, Y., Wang, C., Yan, L., et al. (2022a). Genomic analyses provide insights into the evolution and salinity adaptation of halophyte Tamarix chinensis. Gigascience 12, giad053. doi:10.1093/gigascience/giad053
Liu, Q. L., Zhong, M., Li, S., Pan, Y. Z., Jiang, B. B., Jia, Y., et al. (2013). Overexpression of a chrysanthemum transcription factor gene, DgWRKY3, in tobacco enhances tolerance to salt stress. Plant Physiol. Biochem. 69, 27–33. doi:10.1016/j.plaphy.2013.04.016
Liu, W., Liang, X., Cai, W., Wang, H., Liu, X., Cheng, L., et al. (2022b). Isolation and functional analysis of VvWRKY28, a vitis vinifera WRKY transcription factor gene, with functions in tolerance to cold and salt stress in transgenic Arabidopsis thaliana. Int. J. Mol. Sci. 23, 13418. doi:10.3390/ijms232113418
Liu, X., Song, Y., Xing, F., Wang, N., Wen, F., and Zhu, C. (2016). GhWRKY25, a group I WRKY gene from cotton, confers differential tolerance to abiotic and biotic stresses in transgenic Nicotiana benthamiana. Protoplasma 253, 1265–1281. doi:10.1007/s00709-015-0885-3
Livak, K. J., and Schmittgen, T. D. (2001). Analysis of relative gene expression data using Real-Time quantitative PCR and the 2−ΔΔCT method. Methods 25, 402–408. doi:10.1006/meth.2001.1262
Ludwig, M., Wilmes, P., and Schrader, S. (2018). Measuring soil sustainability via soil resilience. Sci. Total Environ. 626, 1484–1493. doi:10.1016/j.scitotenv.2017.10.043
Mauro, R. P., Perez-Alfocea, F., Cookson, S. J., Ollat, N., and Vitale, A. (2022). Editorial: physiological and molecular aspects of plant Rootstock-Scion interactions. Front. Plant Sci. 13, 852518. doi:10.3389/fpls.2022.852518
Meng, X., Xu, J., He, Y., Yang, K., Mordorski, B., Liu, Y., et al. (2013). Phosphorylation of an ERF transcription factor by Arabidopsis MPK3/MPK6 regulates plant defense gene induction and fungal resistance. Plant Cell 25, 1126–1142. doi:10.1105/tpc.112.109074
Meszaros, T., Helfer, A., Hatzimasoura, E., Magyar, Z., Serazetdinova, L., Rios, G., et al. (2006). The Arabidopsis MAP kinase kinase MKK1 participates in defence responses to the bacterial elicitor flagellin. Plant J. 48, 485–498. doi:10.1111/j.1365-313X.2006.02888.x
Michael, A., Catherine, A. B., Judith, A. B., David, B., Heather, B., Cherry, J. M., et al. (2000). Gene ontology: tool for the unification of biology. The Gene Ontology Consortium. Nat. Genet. 25, 25–29. doi:10.1038/75556
Munns, R., and Tester, M. (2008). Mechanisms of salinity tolerance. Annu. Rev. Plant Biol. 59, 651–681. doi:10.1146/annurev.arplant.59.032607.092911
Pandey, P., and Senthil-Kumar, M. (2019). Plant-pathogen interaction in the presence of abiotic stress: what do we know about plant responses? Indian J. Plant Physiology 24, 541–549. doi:10.1007/s40502-019-00483-7
Park, H. J., Kim, W. Y., and Yun, D. J. (2013). A role for GIGANTEA: keeping the balance between flowering and salinity stress tolerance. Plant Signal Behav. 8, e24820. doi:10.4161/psb.24820
Qadir, M., Quillérou, E., Nangia, V., Murtaza, G., Singh, M., Thomas, R., et al. (2014). Economics of salt-induced land degradation and restoration. Nat. Resour. Forum 38, 282–295. doi:10.1111/1477-8947.12054
Ross, C. A., Liu, Y., and Shen, Q. J. (2007). The WRKY gene family in rice (Oryza sativa). J. Integr. Plant Biol. 49, 827–842. doi:10.1111/j.1744-7909.2007.00504.x
Rushton, P. J., and Somssich, I. E. (1998). Transcriptional control of plant genes responsive to pathogens. Curr. Opin. Plant Biol. 1, 311–315. doi:10.1016/1369-5266(88)80052-9
Savary, S., Akter, S., Almekinders, C., Harris, J., Korsten, L., Rötter, R., et al. (2020). Mapping disruption and resilience mechanisms in food systems. Food Secur. 12, 695–717. doi:10.1007/s12571-020-01093-0
Schluttenhofer, C., and Yuan, L. (2015). Regulation of specialized metabolism by WRKY transcription factors. Plant Physiol. (Bethesda) 167, 295–306. doi:10.1104/pp.114.251769
Shabala, S., and Cuin, T. A. (2008). Potassium transport and plant salt tolerance. Physiol. Plant. 133, 651–669. doi:10.1111/j.1399-3054.2007.01008.x
Sheen, J., Asai, T., Tena, G., Plotnikova, J., Willmann, M. R., Gomez-Gomez, L., et al. (2002). MAP kinase signalling cascade in Arabidopsis innate immunity. Nat. Lond. 415, 977–983. doi:10.1038/415977a
Singh, K., Foley, R. C., and Onate-Sanchez, L. (2002). Transcription factors in plant defense and stress responses. Curr. Opin. Plant Biol. 5, 430–436. doi:10.1016/s1369-5266(02)00289-3
Song, Y., Li, J., Sui, Y., Han, G., Zhang, Y., Guo, S., et al. (2020). The sweet sorghum SbWRKY50 is negatively involved in salt response by regulating ion homeostasis. Plant Mol. Biol. 102, 603–614. doi:10.1007/s11103-020-00966-4
Sun, J., Zhao, X., Fang, Y., Xu, W., Gao, F., Zhao, W., et al. (2022). Root growth and architecture of Tamarix chinensis in response to the groundwater level in the Yellow River Delta. Mar. Pollut. Bull. 179, 113717. doi:10.1016/j.marpolbul.2022.113717
Taj, G., Giri, P., Kumar, A., and Tasleem, M. (2014). MAPK signaling cascades and transcriptional reprogramming in plant–pathogen interactions. Approaches Plant Stress their Manag., 297–316. doi:10.1007/978-81-322-1620-9_17
Tao, Z., Kou, Y., Liu, H., Li, X., Xiao, J., and Wang, S. (2011). OsWRKY45 alleles play different roles in abscisic acid signalling and salt stress tolerance but similar roles in drought and cold tolerance in rice. J. Exp. Bot. 62, 4863–4874. doi:10.1093/jxb/err144
Teige, M., Scheikl, E., Eulgem, T., Dóczi, R., Ichimura, K., Shinozaki, K., et al. (2004). The MKK2 pathway mediates cold and salt stress signaling in arabidopsis. Mol. Cell 15, 141–152. doi:10.1016/j.molcel.2004.06.023
Tittal, M., Mir, R. A., Jatav, K. S., and Agarwal, R. M. (2021). Supplementation of potassium alleviates water stress-induced changes in Sorghum bicolor L. Physiol. Plant 172, 1149–1161. doi:10.1111/ppl.13306
Ulker, B., and Somssich, I. E. (2004). WRKY transcription factors: from DNA binding towards biological function. Curr. Opin. Plant Biol. 7, 491–498. doi:10.1016/j.pbi.2004.07.012
Wang, X., Mohamed, I., Ali, M., Abbas, M. H. H., Shah, G. M., and Chen, F. (2019). Potassium distribution in root and non-root zones of two cotton genotypes and its accumulation in their organs as affected by drought and potassium stress conditions. J. Plant Nutr. Soil Sci. 182, 72–81. doi:10.1002/jpln.201800026
Wei, H., Chen, S., Niyitanga, S., Liu, T., Qi, J., and Zhang, L. (2022a). Genome-wide identification and expression analysis response to GA(3) stresses of WRKY gene family in seed hemp (Cannabis sativa L). Gene 822, 146290. doi:10.1016/j.gene.2022.146290
Wei, Y. L., Jin, J. P., Liang, D., Gao, J., Li, J., Xie, Q., et al. (2022b). Genome-wide identification of Cymbidium sinense WRKY gene family and the importance of its Group III members in response to abiotic stress. Front. Plant Sci. 13, 969010. doi:10.3389/fpls.2022.969010
Yang, H., Xia, J., Cui, Q., Liu, J., Wei, S., Feng, L., et al. (2021). Effects of different Tamarix chinensis-grass patterns on the soil quality of coastal saline soil in the Yellow River Delta, China. Sci. Total Environ. 772, 145501. doi:10.1016/j.scitotenv.2021.145501
Yang, Y., Zhao, L., Yang, G., Zang, Y., Fu, P., Hu, J., et al. (2022). Selection and validation of reference genes for leaf color phenotypein 'maiyuanjinqiu', a Catalpa fargesii variety, by qRT-PCR. For. Res. 35 (1), 123–131. doi:10.13275/j.cnki.lykxyj.2022.01.014
Yao, W., Wang, S., Zhou, B., and Jiang, T. (2016). Transgenic poplar overexpressing the endogenous transcription factor ERF76 gene improves salinity tolerance. Tree Physiol. 36, 896–908. doi:10.1093/treephys/tpw004
Zhu, H., Jiang, Y., Guo, Y., Huang, J., Zhou, M., Tang, Y., et al. (2021). A novel salt inducible WRKY transcription factor gene, AhWRKY75, confers salt tolerance in transgenic peanut. Plant Physiol. Biochem. 160, 175–183. doi:10.1016/j.plaphy.2021.01.014
Zhu, J. (2016). Abiotic stress signaling and responses in plants. Cell 167, 313–324. doi:10.1016/j.cell.2016.08.029
Keywords: exogenous potassium (K+), halophyte, NaCl stress, transcriptome, WRKY transcription factor
Citation: Chen Y, Zhang X, Fan Y, Sui D, Jiang J and Wang L (2023) The role of WRKY transcription factors in exogenous potassium (K+) response to NaCl stress in Tamarix ramosissima. Front. Genet. 14:1274288. doi: 10.3389/fgene.2023.1274288
Received: 08 August 2023; Accepted: 30 October 2023;
Published: 20 November 2023.
Edited by:
Rupesh Deshmukh, Central University of Haryana, IndiaReviewed by:
Pandiyan Muthuramalingam, Gyeongsang National University, Republic of KoreaCopyright © 2023 Chen, Zhang, Fan, Sui, Jiang and Wang. This is an open-access article distributed under the terms of the Creative Commons Attribution License (CC BY). The use, distribution or reproduction in other forums is permitted, provided the original author(s) and the copyright owner(s) are credited and that the original publication in this journal is cited, in accordance with accepted academic practice. No use, distribution or reproduction is permitted which does not comply with these terms.
*Correspondence: Yahui Chen, Y2hlbnlhaHVpQG5qZnUuZWR1LmNu; Jiang Jiang, ZWNvbG9neWppYW5nQGdtYWlsLmNvbQ==; Lei Wang, d2wuc3RvbmVAMTYzLmNvbQ==
Disclaimer: All claims expressed in this article are solely those of the authors and do not necessarily represent those of their affiliated organizations, or those of the publisher, the editors and the reviewers. Any product that may be evaluated in this article or claim that may be made by its manufacturer is not guaranteed or endorsed by the publisher.
Research integrity at Frontiers
Learn more about the work of our research integrity team to safeguard the quality of each article we publish.