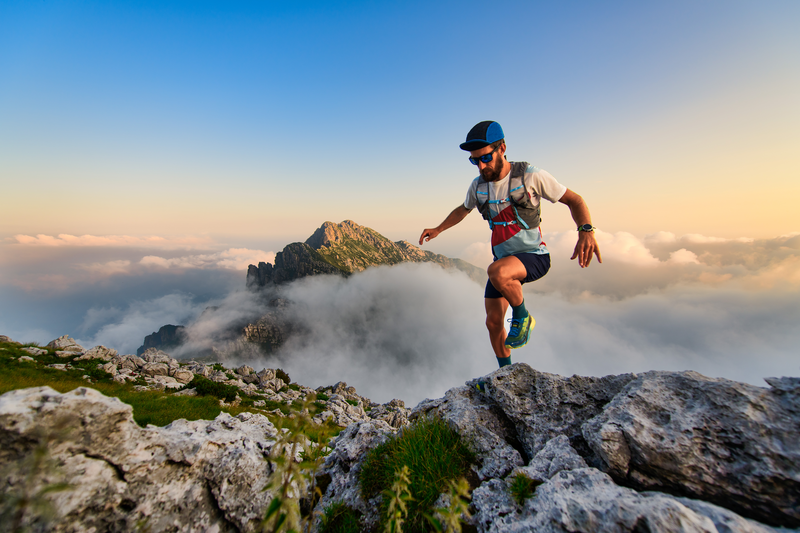
94% of researchers rate our articles as excellent or good
Learn more about the work of our research integrity team to safeguard the quality of each article we publish.
Find out more
ORIGINAL RESEARCH article
Front. Genet. , 03 November 2023
Sec. Human and Medical Genomics
Volume 14 - 2023 | https://doi.org/10.3389/fgene.2023.1249678
This article is part of the Research Topic Liquid Biopsy and New Omics Technologies in Vascular Malformations View all 6 articles
Introduction: Lung cancer is the most frequent cause of cancer-related deaths worldwide. Exosomes are involved in different types of cancer, including lung cancer.
Methods: We collected saliva from patients with (LC) or without (NC) lung cancer and successfully isolated salivary exosomes by ultracentrifugation. MiRNA sequencing was implemented for the exosome samples from NC and LC groups, dgeR was used to determine differentially expressed miRNAs (DE miRNAs), and quantitative real-time polymerase chain reaction (qPCR) was used to verify three differentially expressed microRNAs (miRNAs).
Results: A total of 372 miRNAs were identified based on the sequencing results. Subsequently, 15 DE miRNAs were identified in LC vs. NC, including eight upregulated miRNAs and seven downregulated miRNAs. Some DE miRNAs were validated via qPCR. A total of 488 putative target genes of the upregulated DE miRNAs were found, and the functional analyses indicated that numerous target genes were enriched in the pathways associated with cancer.
Discussion: This suggests that miRNAs of salivary exosomes might have the potential to be used as biomarkers for prediction and diagnosis of lung cancer.
Lung cancer is the leading cause of cancer-related deaths worldwide; two million people are diagnosed with lung cancer, and approximately 1.76 million people die as a result of this disease every year (Hirsch et al., 2017; Thai et al., 2021). Lung cancer is often not diagnosed until it progresses to an advanced stage, and patients have poor treatment outcomes (Nooreldeen and Bach, 2021). Therefore, it is important to explore new ways for diagnosing and treating lung cancer.
Exosomes, secreted by multiple types of cells, are small extracellular vesicles with an average diameter of 30–150 nm, containing RNAs, proteins, and lipids, and play a crucial role in intercellular crosstalk (Kalluri and LeBleu, 2020; Isaac et al., 2021). Previous studies revealed that exosomes were involved in many diseases, including Alzheimer’s disease (Soares Martins et al., 2021), osteoporosis (Shan et al., 2019), and cancer (Yu et al., 2021). In cancer, exosomes are associated with cell proliferation, metastasis, invasion, epithelial–mesenchymal transition (EMT), angiogenesis, and tumor microenvironment (TME) remodeling (Bai et al., 2022). Tumor-derived exosomes contain a variety of stimulatory and inhibitory factors involved in mediating the immune response, which can affect the TME and thus participate in the formation and progression of tumors (Mashouri et al., 2019). Hence, the RNAs, proteins, and lipids from exosomes could be explored as a source of biomarkers for diagnosis and prognosis (Yu et al., 2022). In recent years, significant progress has been made in our understanding of the role of exosomes in the diagnosis, treatment, and prognosis of lung cancer (Li et al., 2021). For example, the deep learning-based spectroscopic analysis of circulating exosomes could be used to diagnose early-stage lung cancer (Shin et al., 2020).
Saliva, an acidic biological fluid composed of secretions from multiple salivary glands, plays a crucial role in numerous biological functions, such as perception of oral sensations, lubrication, chewing, swallowing, and digestion (Kaczor-Urbanowicz et al., 2017). The collection of saliva is convenient for the screening of large populations and provides an alternative for patients whose blood cannot be easily collected or when cooperation is an issue (Chojnowska et al., 2018). In recent years, saliva has been widely used in the diagnosis of many diseases, including Alzheimer’s disease (Ashton et al., 2021), COVID-19 (Fernandes et al., 2020), and cancer (Wang et al., 2017; Li et al., 2022). Saliva-derived exosomal small RNAs have been shown to be useful as biomarkers of esophageal carcinoma (Li et al., 2022), and biomarkers of head and neck cancer were identified through the analysis of cargo and the functional profile of saliva-derived exosomes (Hofmann et al., 2022). In addition, there are studies demonstrating salivary exosomal miRNA-1307-5p is a potential prognosticator for predicting poor survival and poor patient outcome in oral cancers (Patel et al., 2022).
MicroRNAs (miRNAs) derived from exosomes have often been explored as a predictive biomarker for lung cancer, but miRNAs of salivary exosomes remain unexplored. In the current study, we collected saliva from healthy individuals and patients with lung cancer, and salivary exosomes were obtained via a series of centrifugation and ultracentrifugation steps. Subsequently, miRNA sequencing (miRNA-seq), quantitative real-time polymerase chain reaction (qPCR), and bioinformatic analyses were carried out.
In this study, we aimed to investigate the potential biomarkers for early-stage lung adenocarcinoma by analyzing saliva samples. We collected saliva samples from 18 early-stage (I–IIIA) lung adenocarcinoma patients and 18 non-cancer controls, aged between 50 and 75 years, at Harbin Medical University Cancer Hospital, according to the approved protocols by the Ethics Committee of Harbin Medical University (Supplementary Table S1). In general, having oral diseases will affect the expression of miRNA in saliva (Safari et al., 2023). In order to reduce the interference, the participants who did not have oral diseases were included in the study; meanwhile, age, gender, and smoking and drinking status of the participants in the control group were made as consistent as possible with those in the experimental group. In addition, all subjects provided written informed consent. During the collection period, the participants were seated straight up and instructed to refrain from swallowing or speaking, and approximately 5 mL saliva sample was obtained from each individual.
For each set, six samples were mixed to create one biological replicate and centrifuged at 2,000 g for 5 min at 4°C to discard insoluble materials and cell debris. The samples were stored at −80°C for subsequent use.
Saliva samples were centrifuged at 2,500 g for 15 min at 4°C, followed by ultracentrifugation at 120,000 g for 2 h at 4°C to pellet exosomes. Exosome pellets were washed in filtered phosphate-buffered saline (PBS) and re-centrifuged at 120,000 g, the supernatant was removed, and the final pellet was re-suspended in 150 µL of PBS.
Fixation of exosomes was carried out with 2.5% glutaraldehyde for 2 h. Subsequently, the exosomes were purified and suspended in 100 µL serum albumin. Approximately 20 µL of exosomes was overloaded onto a formvar/carbon-coated grid and stained with 3% aqueous phosphor-tungstic acid for 60 s. Exosomes were then examined with transmission electron microscopy (TEM), which indicated their spherical shape.
The supernatants containing particles were analyzed using a NanoSight LM20 instrument equipped with a 640 nm laser (NanoSight, Amesbury, United Kingdom) at 25°C. The movement of particles was tracked using NTA software (version 2.2, NanoSight) with a low refractive index corresponding to cell-derived vesicles. The mean, mode, median particle size, and particle concentration (in millions) for each size were analyzed in each track.
Total RNA was extracted from salivary exosomes with TRIzol reagent (Invitrogen; Thermo Fisher Scientific, Inc.) and purified using two phenol–chloroform extractions followed by treatment with RQ1 DNase (Promega, Madison, WI, United States) to remove DNA. The SmartSpec Plus spectrophotometer (Bio-Rad, Hercules, CA, United States) was used to assess the quality and quantity of the purified RNA. The integrity of RNA was further verified by 1.4% agarose gel electrophoresis.
Total RNAs (3 μg) from each sample were used for small RNA cDNA library preparation using a Balancer NGS Library Preparation kit (Gnomegen, San Diego, CA, United States) according to the manufacturer’s protocol prior to directional RNA-seq library preparation. The whole library was subjected to 10% native polyacrylamide gel electrophoresis. Then, the bands corresponding to miRNA insertion were cut and eluted. Ultimately, the purified libraries were quantified using the Qubit Fluorometer (Invitrogen; Thermo Fisher Scientific, Inc.) after ethanol precipitation and washing. The RNA libraries were subjected to 150 bp pair-end sequencing on the HiSeq 2500 platform (Illumina, Inc., San Diego, CA, United States).
The FASTX-Toolkit (version 0.0.13) (http://hannonlab.cshl.edu/fastx_toolkit/) was used to process raw reads for obtaining reliable clean reads. RNAs <16 or >30 nt in length were also removed from the subsequent analysis according to the length of the adapter and mature miRNA lengths. Subsequently, the clean reads were searched against the Rfam database (version 12.0) using Bowtie (Langmead et al., 2009). Matches to transfer RNAs and ribosomal RNAs were discarded. The remaining unique sequences were aligned using Bowtie against the miRBase database (Kozomara and Griffiths-Jones, 2014), with one mismatch allowed. The aligned small RNA sequences were convinced by conserved miRNAs.
To investigate the expression profiles of identified miRNAs, the frequency of miRNA counts was normalized to transcripts per million (TPM) using the following formula: normalized expression = actual read count/total read count × 106. The edgeR (v3.22) package of Bioconductor software (Robinson et al., 2010) was used to explore the DE miRNAs, and fold change (FC) ≥ 2 or ≤0.5 and p ≤ 0.05 and false discovery rates (FDRs) ≤ 0.05 indicated a statistically significant DE miRNA. The human miRNA and transcript sequences of miRBase were calculated by applying the miRanda algorithm (Krek et al., 2005). Subsequently, TargetScan was used for predicting putative miRNA targets (Lewis et al., 2005). To explore the gene function and analyze the frequency distribution of functional categories, Gene Ontology (GO) and Kyoto Encyclopedia of Genes and Genomes (KEGG) analyses were employed using the R package “clusterProfiler” using the DAVID bioinformatics database (Huang et al., 2009).
Salivary exosome proteins were extracted and separated by denaturing sodium dodecyl sulfate-polyacrylamide gel electrophoresis (SDS-PAGE). Subsequently, they were transferred to a polyvinylidene difluoride (PVDF) membrane and incubated with blocking buffer [5% skimmed milk powder in PBS–Tween-20 (PBST)] for 1 h. After washing with PBST, the membrane was incubated with primary antibodies (CD9 and syntenin; Abcam, MA, United States) according to the manufacturer’s instructions. Then, the PVDF membrane was incubated with the appropriate secondary antibody for 1 h followed by washing. Finally, the PVDF membrane was incubated for 3 min with SuperSignal West Pico Western HRP substrate at room temperature and imaged using a Tanon 5200 multi-imaging system (Tanon, Shanghai, China).
qPCR was carried out to detect the expression levels of miRNAs using primers designed for reverse transcription (RT) and qPCR. The primer sequences used in our study were as follows: miR-92b-5p: 5′-CTC AAC TGG TGT CGT GGA GTC GGC AAT TCA GTT GAG CAC TGC AC-3′ (RT), 5′-ACA CTC CAG CTG GGA GGG ACG GGA CGC GGT-3′ (forward), and 5′-TGG TGT CGT GGA GTC G-3′ (reverse); miR-532-3p: 5′-CTC AAC TGG TGT CGT GGA GTC GGC AAT TCA GTT GAG TGC AAG CC-3′ (RT), 5′-ACA CTC CAG CTG GGC CTC CCA CAC CCA AGG-3′ (forward), and 5′-TGG TGT CGT GGA GTC G-3′ (reverse); miR-135b-5p: 5′-CTC AAC TGG TGT CGT GGA GTC GGC AAT TCA GTT GAG TCA CAT AG-3′ (RT), 5′-ACA CTC CAG CTG GGT ATG GCT TTT CAT TCC T-3′ (forward), and 5′-TGG TGT CGT GGA GTC G-3′ (reverse); and U6: 5′-CTC GCT TCG GCA GCA CA-3′ (forward) and 5′-AAC GCT TCA CGA ATT GTG CGT-3′ (reverse). cDNAs were generated by standard procedures, and real-time PCR was performed on Bio-Rad S1000 (Bio-Rad, Hercules, CA, United States) with Bestar SYBR Green RT-PCR Master Mix (DBI Bioscience, Shanghai, China). The PCR cycling conditions were set as follows: 95°C for 10 min, 38 cycles at 95°C for 15 s, annealing, and extension at 60°C for 1 min. qPCR amplifications were performed in triplicate for each sample. Transcript levels for the genes analyzed were measured in comparison with the housekeeping gene U6 as an internal reference standard using the 2−ΔΔCT method (Livak and Schmittgen, 2001).
The data were analyzed for statistical significance using Microsoft Excel (2012). All data are presented as the mean ± standard deviation (mean ± SD). Student’s t-test (paired) was used to determine the statistical significance when comparing the means of two datasets. A p-value < 0.05 was considered statistically significant.
In this study, a total of 567 samples, including 521 patients with lung cancer and 46 normal tissues, were obtained from The Cancer Genome Atlas (TCGA) database (https://portal.gdc.cancer.gov/).
The datasets obtained in the current study have been deposited in the National Center for Biotechnology Information under accession number PRJNA908267 (https://www.ncbi.nlm.nih.gov/bioproject/PRJNA908267).
Saliva samples from 18 patients with lung cancer (LC) and 18 individuals without lung cancer (NC) were collected (Figure 1A). Six samples for each group were mixed to create one biological replicate, and six samples of salivary exosomes (LC-1, LC-2, and LC-3; NC-1, NC-2, and NC-3) were obtained by a series of differential centrifugation, ultracentrifugation, and filtration steps (Figure 1B).
FIGURE 1. Characterization of salivary exosomes from individuals with (LC) or without lung cancer (NC). (A) Schematic diagram illustrating the experimental design from sample collection to sequencing. (B) Schematic diagram illustrating isolation of salivary exosomes. (C) Exosomes were imaged with transmission electron microscopy (TEM). Electron microscopy allowed visualization of membrane-bound nanovesicles sized ∼100 nm. Scale bar = 100 nm. (D) Analysis of exosomes using a NanoSight LM10-HS instrument. (E) Exosome validation by Western blotting indicating expression of the exosomal markers CD9 and syntenin.
TEM examination indicated that exosomes from two groups showed a characteristic round- or cup-shaped morphology and dimension (Figure 1C). Nanoparticle tracking analysis (NTA) indicated that the average sizes of exosomes from LC and NC groups were 73.34 and 75.68 nm, respectively (Figure 1D). Furthermore, exosomes were validated by Western blotting. Exosomes can be characterized using exosome markers (CD9 and syntenin) expressed on their outer membrane. By analyzing the gray values of marker proteins, it was discovered that the expression level of the CD9 protein within the LC group was higher than that in the NC group. In contrast, the opposite was observed for the expression level of the syntenin protein (Figure 1E). These results indicated that salivary exosomes were isolated successfully and were suitable for further investigation.
Previous studies have indicated that exosomal miRNAs play significant roles in cancer biology and clinical applications (Sun et al., 2018a; Hu et al., 2020). The aim of this study was to investigate the miRNA cargo of salivary exosomes from two groups. Therefore, a total of six small RNA libraries (LC-1, LC-2, and LC-3; NC-1, NC-2, and NC-3) were constructed for miRNA-seq with three biological replicates for each group.
Using Illumina HiSeq 2500, >433.1 million reads were generated, corresponding to ∼73.2 million sequence reads per library; the clean reads accounted for ∼87.7%. Hierarchical clustering heatmap analyses indicated that the global expression pattern of miRNAs differed obviously between the LC and NC groups (Figures 2A, B). The clean reads were matched to Rfam and a human reference genome (Ensembl release 102 GRCh38), and the filtered reads were mapped to miRBase. The read counts were normalized to TPM, and a total of 372 miRNAs were identified (Supplementary Table S2).
FIGURE 2. Salivary exosome miRNAs of the salivary exosomes. (A) Hierarchical clustering heatmap showing the distinct miRNA expression patterns of the six samples (LC-1, LC-2, and LC-3; NC-1, NC-2, and NC-3). (B) Heatmap of the miRNAs identified in the salivary exosomes. (C) Volcano plot indicating the expression of miRNAs in LC vs. NC groups. X-axis represents the log2 fold-changes (FC), while the y-axis represents the −log10 significant difference (p-value). miRNAs with significant differential expression are shown in red (upregulated) or blue (downregulated). miRNAs that are not significantly differentially expressed are shown in black. A FC of log2 ratio ≥2 and p-value < 0.05 were set as the threshold to determine differentially expressed miRNAs. (D) Heatmap of the DE miRNAs.
DE miRNAs were determined using edgeR. The threshold for screening differentially expressed miRNAs was set as follows: a “fold change (FC) ≥ 2 or ≤ 0.5 and p ≤ 0.05 and false discovery rates (FDR) ≤ 0.05.” A total of 15 DE miRNAs were identified in LC vs. NC, including eight upregulated miRNAs and seven downregulated miRNAs, respectively (Figures 2C, D). The highest and lowest FC values were 25.13 and 2−4.89, respectively (Supplementary Table S3). These findings indicated that lung cancer is associated with the expression levels of numerous miRNAs in salivary exosomes.
Three miRNAs (miR-92b-5p, miR-532-3p, and miR-135b-5p) were selected from these identified miRNAs and verified by RT-qPCR (Figure 3A). The RT-qPCR expression results were highly correlated with the RNA-seq results (Figure 3B). Hence, the RNA-seq data were reliable and suitable for further analysis.
FIGURE 3. RT-qPCR validation of three differentially expressed miRNAs. (A) miRNA expression was determined by high-throughput sequencing. Fragments per kilobase per million mapped reads were used to calculate the expression levels of miRNAs. (B) miRNA expression levels of target genes were determined by RT-qPCR and normalized against U6 expression. Statistical analysis was performed by Student’s t-test, and data are presented at the mean ± standard deviation of three replicates. *p < 0.05, **p < 0.01. RT-qPCR, reverse transcription quantitative real-time polymerase chain reaction.
miRNAs commonly exert their functions by binding to complementary target sites in the mRNAs of their target genes. Using the miRanda algorithm on human miRNA and transcript sequences of miRBase and TargetScan, 488 and 161 overlapped target genes were identified for the upregulated and downregulated DE miRNAs in LC vs. NC (Supplementary Table S4), respectively. This suggests that the DE miRNAs of salivary exosomes have the potential to regulate the expression levels of multiple genes.
To identify the pathways associated with the target genes of the upregulated DE miRNAs, GO enrichment analysis was conducted and 54 biological pathways were identified (p < 0.01, FDR < 0.01) (Figure 4A; Supplementary Table S5). A large number of terms were associated with cell proliferation and cycle, including “mitotic cell cycle phase transition” (GO: 0044772), “regulation of cell cycle phase transition” (GO: 1901987), “regulation of mitotic cell cycle phase transition” (GO: 1901990), “G1/S transition of mitotic cell cycle” (GO: 0000082), “negative regulation of cell cycle” (GO: 0045786), “cell cycle G1/S phase transition” (GO: 0044843), and “negative regulation of mitotic cell cycle” (GO: 0045930). These results suggested that deregulated expression of miRNAs might be associated with a disorder of cell proliferation.
FIGURE 4. Functional analysis of target genes of the upregulated and downregulated differentially expressed (DE) miRNAs. (A) GO analysis of upregulated DE miRNA target genes (B) KEGG analysis of upregulated DE miRNA target genes. (C) GO analysis of downregulated DE miRNA target genes. (D) KEGG analysis of downregulated DE miRNA target genes. The top 15 terms are presented. miRNA, microRNA; GO, Gene Ontology; KEGG, Kyoto Encyclopedia of Genes and Genomes. The rich factor indicates the enrichment degree of terms, while y-axis represents the names of the enriched terms. The area of the node indicates the number of genes. The p-value is shown by a color scale with the statistical significance increasing from green to red.
KEGG enrichment analysis revealed that 20 significant functional terms were associated with the target genes of the upregulated DE miRNAs (p < 0.01, FDR < 0.01) (Figure 4B; Supplementary Table S6). Multiple enriched pathways were associated with cancer, including “colorectal cancer” (ID: hsa05210), “hepatocellular carcinoma” (ID: hsa05225), “microRNAs in cancer” (ID: hsa05206), “proteoglycans in cancer” (ID: hsa05205), “gastric cancer” (ID: hsa05226), “endometrial cancer” (ID: hsa05213), “chronic myeloid leukemia” (ID: hsa05220), “chemical carcinogenesis-receptor activation” (ID: hsa05207), “prostate cancer” (ID: hsa05215), “breast cancer” (ID: hsa05224), and “bladder cancer” (ID: hsa05219).
Moreover, GO and KEGG enrichment analyses were carried out for downregulated DE miRNAs (Figures 4C, D; Supplementary Tables S7, S8), resulting in 64 and 16 terms, respectively (p < 0.01, FDR < 0.05). It was found that multiple enriched GO terms were associated with apoptosis of cell, including “intrinsic apoptotic signaling pathway” (GO: 0097193), “negative regulation of apoptotic signaling pathway” (GO: 2001234), “neuron apoptotic process” (GO: 0051402), “regulation of apoptotic signaling pathway” (GO: 2001233), “intrinsic apoptotic signaling pathway in response to DNA damage by p53 class mediator” (GO: 0042771), and “cellular response to abiotic stimulus” (GO: 0071214), and several KEGG terms were involved with cancer, including “viral carcinogenesis” (ID: hsa05203), “prostate cancer” (ID: hsa05215), and “glioma” (ID: hsa05214).
These results suggested that deregulated expression of miRNAs might be involved in the occurrence and development of cancer.
An improved understanding of cancer is important for the improvement of treatment options (Tarighati et al., 2023). Although tissue biopsy and conventional imaging techniques have been widely used for many years, limitations remain unaddressed. For tissue biopsy, limited sampling is often hard to capture the heterogeneity and evolution of tumors (Cheng et al., 2019), while conventional imaging techniques are less sensitive for the early detection of cancer (Loy et al., 2022). In recent years, liquid biopsy, which aims to provide an alternative to invasive tissue biopsy by analyzing biomarkers in biofluids that reflect the nature of cancer (Ignatiadis et al., 2021), has been widely investigated. In the present study, the collection of saliva samples was convenient as all participants were more cooperative. Therefore, we can use saliva samples as liquid biopsy more widely, and putative biomarkers might be discovered by comparative analyses in future.
Exosomes are small extracellular vesicles secreted by various types of cells that play an essential role in intercellular signaling and cellular homeostasis (Stahl and Raposo, 2019) and affect the pathophysiological conditions of recipient cells. Hence, the molecules derived from exosomes could be used as ideal biomarkers in detecting and monitoring cancer for their biological role in cancer pathogenesis (Mashouri et al., 2019). Exosomes are easily isolated from a wide variety of body fluids, including blood, urine, saliva, breast milk, and cerebrospinal fluid; therefore, exploring exosomal biomarkers of cancer is feasible (Nair et al., 2018; Yu et al., 2022). In the present study, salivary exosomes were isolated by a series of centrifugation and ultracentrifugation steps (Figure 1B), and these protocols could be referenced for future studies.
Exosomes contain diverse constituents including nucleic acids, proteins, lipids, amino acids, and metabolites. miRNAs are non-coding RNAs of approximately 21–23 nucleotides in length that can regulate gene expression at the posttranscriptional level. Exosomal miRNAs, which are transferred into extracellular vesicles through unknown mechanisms, are not prone to RNase-mediated degradation (Yu et al., 2016; Garcia-Martin et al., 2022). Hence, miRNAs are more stable and have been intensely investigated compared with other molecules derived from exosomes (Sun et al., 2018b; Lin et al., 2022). In this study, using miRNA-seq and comparative analyses, a total of 372 exosomal miRNAs were identified, and it was also found that eight miRNAs were significantly upregulated in the salivary samples of patients with lung cancer (Figures 2, 3), suggesting exosomal miRNAs might be suitable biomarkers for disease diagnosis.
Functional analyses of these putative targets of the upregulated miRNAs suggested that these miRNAs might regulate pathways associated with cancer (Figure 4). In the present study, miRNA-Seq and RT-qPCR analyses indicated that miR-92b-5p was more highly enriched in salivary exosomes from individuals with lung cancer (Figure 3). Previous studies suggested that miR-92b-5p could be used for predicting the prognosis of gastric cancer (Xu et al., 2021) and biliary tract cancer (Høgdall et al., 2022), suggesting that this exosomal miRNA might have the potential to be used as a diagnostic biomarker of lung cancer. Although other studies have also associated miR-532-3p with cancer (Han et al., 2019; Jiang et al., 2019), salivary exosomal miR-532-3p deserves further investigation.
Moreover, we obtained 567 samples, including 521 patients with lung cancer and 46 normal tissues, from the TCGA-LUAD database (Supplementary Table S9). The expression levels of some miRNAs were analyzed, and the results indicated that three miRNAs (miR-92b-5p, mir-135b-5p, and mir-532-3p) were upregulated in tumor samples (Supplementary Figures S1A–C). Subsequently, logistic regression analysis was used to predict the effect of the model constructed by these three indicators (hsa-mir-92b-5p, hsa-mir-532-3p, and hsa-mir-135-5p) in distinguishing patients with lung adenocarcinoma from normal people; four models [mir-92b-5p (model 1), has-mir-532-3p (model 2), hsa-mir-135b-5p (model 3), and mir-92b-5p + hsa-mir-532-3p + mir-135b-5p (model 4)] were established and analyzed, and the results indicated that AUCs (areas under the curve) of four models were 0.590 (p < 0.001), 0.699 (p < 0.001), 0.906 (p < 0.001) and 0.912 (p < 0.001), respectively (Supplementary Figure S1D). At the same time, it can be seen that the combined use of multiple miRNAs can achieve better results than a single miRNA, suggesting that they might be useful as biomarkers for lung cancer prediction and diagnosis.
The datasets presented in this study can be found in online repositories. The names of the repository/repositories and accession number(s) can be found in the article/Supplementary Material.
ML and CQ conceived the study and participated in its design. XY participated in study design and coordination and drafted the manuscript. JB participated in study design, assessed treatment effectiveness, and collected clinical data. QX participated in study design and performed the statistical analysis. ML and CQ interviewed patients before and after treatment and collected clinical data. SM and NC recruited and screened eligible participants from the outpatient department and assigned patients to either the massage group or control group. ML and CQ participated in trial design and helped prepare the manuscript. All authors contributed to the article and approved the submitted version.
The authors thank members of Wuhan GeneCreate Biological Engineering Limited for fruitful discussions and, in particular, Dr. Zhan for critical reading of the manuscript.
The authors declare that the research was conducted in the absence of any commercial or financial relationships that could be construed as a potential conflict of interest.
All claims expressed in this article are solely those of the authors and do not necessarily represent those of their affiliated organizations, or those of the publisher, the editors, and the reviewers. Any product that may be evaluated in this article, or claim that may be made by its manufacturer, is not guaranteed or endorsed by the publisher.
The Supplementary Material for this article can be found online at: https://www.frontiersin.org/articles/10.3389/fgene.2023.1249678/full#supplementary-material
Ashton, N. J., Blennow, K., and Zetterberg, H. (2021). Spitting image: can saliva biomarkers reflect Alzheimer's disease? EBioMedicine 68, 103437. doi:10.1016/j.ebiom.2021.103437
Bai, S., Wei, Y., Liu, R., Xu, R., Xiang, L., and Du, J. (2022). Role of tumour-derived exosomes in metastasis. Biomed. Pharmacother. 147, 112657. doi:10.1016/j.biopha.2022.112657
Cheng, J., Nonaka, T., and Wong, D. T. W. (2019). Salivary exosomes as nanocarriers for cancer biomarker delivery. Mater. (Basel) 12 (4), 654. doi:10.3390/ma12040654
Chojnowska, S., Baran, T., Wilińska, I., Sienicka, P., Cabaj-Wiater, I., and Knaś, M. (2018). Human saliva as a diagnostic material. Adv. Med. Sci. 63 (1), 185–191. doi:10.1016/j.advms.2017.11.002
Fernandes, L. L., Pacheco, V. B., Borges, L., Athwal, H. K., de Paula Eduardo, F., Bezinelli, L., et al. (2020). Saliva in the diagnosis of COVID-19: a review and new research directions. J. Dent. Res. 99 (13), 1435–1443. doi:10.1177/0022034520960070
Garcia-Martin, R., Wang, G., Brandão, B. B., Zanotto, T. M., Shah, S., Kumar Patel, S., et al. (2022). MicroRNA sequence codes for small extracellular vesicle release and cellular retention. Nature 601 (7893), 446–451. doi:10.1038/s41586-021-04234-3
Han, J., Wang, F., Lan, Y., Wang, J., Nie, C., Liang, Y., et al. (2019). KIFC1 regulated by miR-532-3p promotes epithelial-to-mesenchymal transition and metastasis of hepatocellular carcinoma via gankyrin/AKT signaling. Oncogene 38 (3), 406–420. doi:10.1038/s41388-018-0440-8
Hirsch, F. R., Scagliotti, G. V., Mulshine, J. L., Kwon, R., Curran, W. J., Wu, Y. L., et al. (2017). Lung cancer: current therapies and new targeted treatments. Lancet 389 (10066), 299–311. doi:10.1016/S0140-6736(16)30958-8
Hofmann, L., Medyany, V., Ezić, J., Lotfi, R., Niesler, B., Röth, R., et al. (2022). Cargo and functional profile of saliva-derived exosomes reveal biomarkers specific for head and neck cancer. Front. Med. (Lausanne) 9, 904295. doi:10.3389/fmed.2022.904295
Høgdall, D., O'Rourke, C. J., Larsen, F. O., Zarforoushan, S., Christensen, T. D., Ghazal, A., et al. (2022). Whole blood microRNAs capture systemic reprogramming and have diagnostic potential in patients with biliary tract cancer. J. Hepatol. 77 (4), 1047–1058. doi:10.1016/j.jhep.2022.05.036
Hu, C., Meiners, S., Lukas, C., Stathopoulos, G. T., and Chen, J. (2020). Role of exosomal microRNAs in lung cancer biology and clinical applications. Cell Prolif. 53 (6), e12828. doi:10.1111/cpr.12828
Huang, da W., Sherman, B. T., and Lempicki, R. A. (2009). Systematic and integrative analysis of large gene lists using DAVID bioinformatics resources. Nat. Protoc. 4 (1), 44–57. doi:10.1038/nprot.2008.211
Ignatiadis, M., Sledge, G. W., and Jeffrey, S. S. (2021). Liquid biopsy enters the clinic-implementation issues and future challenges. Nat. Rev. Clin. Oncol. 18 (5), 297–312. doi:10.1038/s41571-020-00457-x
Isaac, R., Reis, F. C. G., Ying, W., and Olefsky, J. M. (2021). Exosomes as mediators of intercellular crosstalk in metabolism. Cell Metab. 33 (9), 1744–1762. doi:10.1016/j.cmet.2021.08.006
Jiang, W., Zheng, L., Yan, Q., Chen, L., and Wang, X. (2019). MiR-532-3p inhibits metastasis and proliferation of non-small cell lung cancer by targeting FOXP3. J. BUON 24 (6), 2287–2293.
Kaczor-Urbanowicz, K. E., Martin Carreras-Presas, C., Aro, K., Tu, M., Garcia-Godoy, F., and Wong, D. T. (2017). Saliva diagnostics-current views and directions. Exp. Biol. Med. (Maywood) 242 (5), 459–472. doi:10.1177/1535370216681550
Kalluri, R., and LeBleu, V. S. (2020). The biology, function, and biomedical applications of exosomes. Science 367 (6478), eaau6977. doi:10.1126/science.aau6977
Kozomara, A., and Griffiths-Jones, S. (2014). MiRBase: annotating high confidence microRNAs using deep sequencing data. Nucleic Acids Res. 42, D68–D73. doi:10.1093/nar/gkt1181
Krek, A., Grun, D., Poy, M. N., Wolf, R., Rosenberg, L., Epstein, E. J., et al. (2005). Combinatorial microRNA target predictions. Nat. Genet. 37, 495–500. doi:10.1038/ng1536
Langmead, B., Trapnell, C., Pop, M., and Salzberg, S. L. (2009). Ultrafast and memory-efficient alignment of short DNA sequences to the human genome. Genome Biol. 10, R25. doi:10.1186/gb-2009-10-3-r25
Lewis, B. P., Burge, C. B., and Bartel, D. P. (2005). Conserved seed pairing, often flanked by adenosines, indicates that thousands of human genes are microRNA targets. Cell 120, 15–20. doi:10.1016/j.cell.2004.12.035
Li, K., Lin, Y., Luo, Y., Xiong, X., Wang, L., Durante, K., et al. (2022). A signature of saliva-derived exosomal small RNAs as predicting biomarker for esophageal carcinoma: a multicenter prospective study. Mol. Cancer 21 (1), 21. doi:10.1186/s12943-022-01499-8
Li, M. Y., Liu, L. Z., and Dong, M. (2021). Progress on pivotal role and application of exosome in lung cancer carcinogenesis, diagnosis, therapy and prognosis. Mol. Cancer 20 (1), 22. doi:10.1186/s12943-021-01312-y
Lin, B., Jiang, J., Jia, J., and Zhou, X. (2022). Recent advances in exosomal miRNA biosensing for liquid biopsy. Molecules 27 (21), 7145. doi:10.3390/molecules27217145
Livak, K. J., and Schmittgen, T. D. (2001). Analysis of relative gene expres-sion data using real-time quantitative PCR and the 2-ΔΔCT method. Methods 25, 402–408. doi:10.1006/meth.2001.1262
Loy, L. M., Aftab, S., Ang, Y. L. S., Ding, S. L. C., and Ho, C. L. (2022). Intracranial "white" epidermoid tumours-an imaging approach and systematic review. Clin. Imaging 91, 1–8. doi:10.1016/j.clinimag.2022.08.002
Mashouri, L., Yousefi, H., Aref, A. R., Ahadi, A. M., Molaei, F., and Alahari, S. K. (2019). Exosomes: composition, biogenesis, and mechanisms in cancer metastasis and drug resistance. Mol. Cancer 18 (1), 75. doi:10.1186/s12943-019-0991-5
Nair, S., Tang, K. D., Kenny, L., and Punyadeera, C. (2018). Salivary exosomes as potential biomarkers in cancer. Oral Oncol. 84, 31–40. doi:10.1016/j.oraloncology.2018.07.001
Nooreldeen, R., and Bach, H. (2021). Current and future development in lung cancer diagnosis. Int. J. Mol. Sci. 22 (16), 8661. doi:10.3390/ijms22168661
Patel, A., Patel, S., Patel, P., Mandlik, D., Patel, K., and Tanavde, V. (2022). Salivary Exosomal miRNA-1307-5p predicts disease aggressiveness and poor prognosis in oral squamous cell carcinoma patients. Int. J. Mol. Sci. 23 (18), 10639. doi:10.3390/ijms231810639
Robinson, M. D., McCarthy, D. J., and Smyth, G. K. (2010). EdgeR: a biocon-ductor package for differential expression analysis of digital gene expression data. Bioinformatics 26, 139–140. doi:10.1093/bioinformatics/btp616
Safari, Z., Firouzi, A., Rezaeikalantari, N., Mohammadi, S., Ranjbar, N., Shahpori, H., et al. (2023). The salivary exosomal microRNA as a potential biomarker in patients with periodontitis and oral cancers. Chem. Biol. Drug Des. 101 (5), 1204–1215. doi:10.1111/cbdd.14159
Shan, S. K., Lin, X., Li, F., Xu, F., Zhong, J. Y., Guo, B., et al. (2019). Exosomes and bone disease. Curr. Pharm. Des. 25 (42), 4536–4549. doi:10.2174/1381612825666191127114054
Shin, H., Oh, S., Hong, S., Kang, M., Kang, D., Ji, Y. G., et al. (2020). Early-stage lung cancer diagnosis by deep learning-based spectroscopic analysis of circulating exosomes. ACS Nano 14 (5), 5435–5444. doi:10.1021/acsnano.9b09119
Soares Martins, T., Trindade, D., Vaz, M., Campelo, I., Almeida, M., Trigo, G., et al. (2021). Diagnostic and therapeutic potential of exosomes in Alzheimer's disease. J. Neurochem. 156 (2), 162–181. doi:10.1111/jnc.15112
Stahl, P. D., and Raposo, G. (2019). Extracellular vesicles: exosomes and microvesicles, integrators of homeostasis. Physiol. (Bethesda) 34 (3), 169–177. doi:10.1152/physiol.00045.2018
Sun, Y., Huo, C., Qiao, Z., Shang, Z., Uzzaman, A., Liu, S., et al. (2018a). Comparative proteomic analysis of exosomes and microvesicles in human saliva for lung cancer. J. Proteome Res. 17 (3), 1101–1107. doi:10.1021/acs.jproteome.7b00770
Sun, Z., Shi, K., Yang, S., Liu, J., Zhou, Q., Wang, G., et al. (2018b). Effect of exosomal miRNA on cancer biology and clinical applications. Mol. Cancer 17 (1), 147. doi:10.1186/s12943-018-0897-7
Tarighati, E., Keivan, H., and Mahani, H. (2023). A review of prognostic and predictive biomarkers in breast cancer. Clin. Exp. Med. 23 (1), 1–16. doi:10.1007/s10238-021-00781-1
Thai, A. A., Solomon, B. J., Sequist, L. V., Gainor, J. F., and Heist, R. S. (2021). Lung cancer. Lancet 398 (10299), 535–554. doi:10.1016/S0140-6736(21)00312-3
Wang, X., Kaczor-Urbanowicz, K. E., and Wong, D. T. (2017). Salivary biomarkers in cancer detection. Med. Oncol. 34 (1), 7. doi:10.1007/s12032-016-0863-4
Xu, J., Wen, J., Li, S., Shen, X., You, T., Huang, Y., et al. (2021). Immune-Related nine-microRNA signature for predicting the prognosis of gastric cancer. Front. Genet. 12, 690598. doi:10.3389/fgene.2021.690598
Yu, D., Li, Y., Wang, M., Gu, J., Xu, W., Cai, H., et al. (2022). Exosomes as a new frontier of cancer liquid biopsy. Mol. Cancer 21 (1), 56. doi:10.1186/s12943-022-01509-9
Yu, W., Hurley, J., Roberts, D., Chakrabortty, S. K., Enderle, D., Noerholm, M., et al. (2021). Exosome-based liquid biopsies in cancer: opportunities and challenges. Ann. Oncol. 32 (4), 466–477. doi:10.1016/j.annonc.2021.01.074
Keywords: lung cancer, saliva, exosome, microRNA, miRNA sequencing, biomarker
Citation: Liu M, Yu X, Bu J, Xiao Q, Ma S, Chen N and Qu C (2023) Comparative analyses of salivary exosomal miRNAs for patients with or without lung cancer. Front. Genet. 14:1249678. doi: 10.3389/fgene.2023.1249678
Received: 29 June 2023; Accepted: 16 October 2023;
Published: 03 November 2023.
Edited by:
Massimo Vaghi, ASST Rhodense, ItalyReviewed by:
Nir Pillar, University of California, Los Angeles, United StatesCopyright © 2023 Liu, Yu, Bu, Xiao, Ma, Chen and Qu. This is an open-access article distributed under the terms of the Creative Commons Attribution License (CC BY). The use, distribution or reproduction in other forums is permitted, provided the original author(s) and the copyright owner(s) are credited and that the original publication in this journal is cited, in accordance with accepted academic practice. No use, distribution or reproduction is permitted which does not comply with these terms.
*Correspondence: Changfa Qu, MjEwNkBocmJtdS5lZHUuY24=
Disclaimer: All claims expressed in this article are solely those of the authors and do not necessarily represent those of their affiliated organizations, or those of the publisher, the editors and the reviewers. Any product that may be evaluated in this article or claim that may be made by its manufacturer is not guaranteed or endorsed by the publisher.
Research integrity at Frontiers
Learn more about the work of our research integrity team to safeguard the quality of each article we publish.