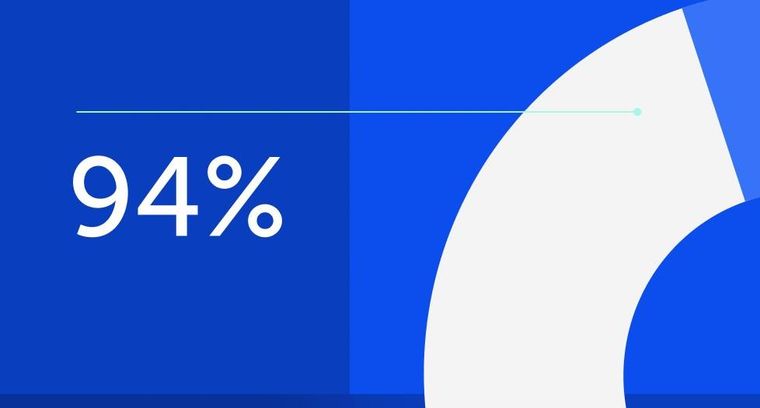
94% of researchers rate our articles as excellent or good
Learn more about the work of our research integrity team to safeguard the quality of each article we publish.
Find out more
EDITORIAL article
Front. Genet., 17 April 2023
Sec. Genetics of Aging
Volume 14 - 2023 | https://doi.org/10.3389/fgene.2023.1199667
This article is part of the Research TopicTelomere Length and Species LifespanView all 5 articles
Editorial on the Research Topic
Telomere length and species lifespan
The extent to which telomeres, the protective caps at the ends of chromosomes, determine lifespan and impact the aging process has been debated for many decades and continues to be debated today. In the late 1800s, the German evolutionary biologist August Weismann was one of the first to propose that aging is caused by the inability of cells to replicate forever (Strehler, 2000). His theory was seemingly disproved when Alexis Carrel published papers claiming that mammalian cells have an infinite capacity for replication (Carrel, 1912). This was the prevailing view for many decades until 1961 when Leonard Hayflick was able to use better techniques to demonstrate that mammalian cells do indeed have a finite replicative capacity (Hayflick and Moorhead, 1961). Through a series of discoveries (Watson, 1972; Szostak and Blackburn, 1982; Greider and Blackburn, 1985; Cooke and Smith, 1986; Greider and Blackburn, 1989), scientists soon worked out that the telomere caps at the ends of chromosomes shortened with each cell division, that cells entered into a state of senescence once telomeres became critically short, and that the enzyme telomerase could lengthen telomeres. There seemed to be a strong link between telomeres and aging. This relationship became more complicated however, when the discovery was made that mice lacking the gene for telomerase did not show dramatically shortened lifespans or defects until the third or fourth generation of breeding after the gene knockout (Blasco et al., 1997; Lee et al., 1998; Rudolph et al., 1999). Additionally, some species of mice have short telomere lengths similar to humans, while others have extremely long telomere lengths, yet they have approximately the same lifespans (Hemann and Greider, 2000). Today there are those who argue that other aspects of biology such as changes of DNA methylation with age are much more important determinants of the aging process. One approach to disentangle the effects of telomeres on the aging process is to study how telomeres affect the lifespan of different species. One recent article has demonstrated that the telomere shortening rate can be used to predict the lifespan of a variety of species (Whittemore et al., 2019), but there are still many additional questions that can be addressed and species that could be investigated.
In this small Research Topic of articles, the effect of telomeres in different species is explored. One of the articles found that there is little correlation between telomere length and lifespan in marine mammals (https://www.frontiersin.org/articles/10.3389/fgene.2021.737860/full). However, there was a strong correlation between body size and lifespan. These findings are in agreement with a previous study which found no correlation between initial species telomere length and lifespan but did find a strong correlation between telomere shortening rate and lifespan (Whittemore et al., 2019). In the marine mammal study, telomere shortening rates could not be determined since the necessary samples were not available. Another article in this Research Topic studied the telomere length of Psittacidae species (parrots) and found that longer living species had longer telomere lengths and greater antioxidant capacity (https://www.frontiersin.org/articles/10.3389/fgene.2023.1156730/full). Interestingly, the study found that breeding shortened telomere length. Another study investigated the paternal effects of telomere length on Passer domesticus (house sparrows) and found that older fathers had daughters with longer telomere lengths (https://www.frontiersin.org/articles/10.3389/fgene.2022.880455/full). A separate study in this Research Topic investigated the effects of the tumor suppressor genes p16 and p21 in a mouse model of Werner syndrome lacking telomerase (https://www.frontiersin.org/articles/10.3389/fgene.2021.597566/full). The study found that p16 and p21 had very different effects: p21 deficiency resulted in a dramatic increase in DNA damage responses, cellular senescence, apoptosis, and proliferation, whereas p16 deficiency showed reduced cellular senescence, apoptosis, increased telomere length, and increased cellular proliferation, ultimately rescuing the aging Werner syndrome phenotype.
The overall trend that species lifespan increases with increasing body mass, as also noted in the marine mammal article in this Research Topic (https://www.frontiersin.org/articles/10.3389/fgene.2021.737860/full), is quite fascinating. One possible explanation for this trend is simply that smaller animals are more likely to be eaten, and therefore, there would be no natural selection pressure to select for genes to allow for long life since the average lifespan would already be short. However, smaller organisms also often have higher heartbeat rates and metabolisms. Why is it that small animals have higher heartbeat rates and metabolisms? One proposal is that a faster metabolism is necessary in small organisms due to their large surface area to volume ratio through which heat can be lost (Levine, 1997). Thus, it is necessary for these species to have a fast metabolism in order to maintain their body temperature. Perhaps the fast metabolism of these species leads to more rapid cell turnover and telomere shortening. Note that within a given species, the opposite trend is observed: instead of large body size being correlated with longer life, smaller breeds within a species tend to live longer than larger breeds. For example, smaller breeds of dogs live longer on average than larger breeds of dogs (Selman et al., 2013), smaller breeds of horses live longer than larger breeds of horses (https://equestrianspace.com/average-lifespan-of-a-horse/), smaller mice live longer (Miller et al., 2000), and there is even a trend for shorter humans to live longer than taller humans (Samaras et al., 2003).
There are many important questions remaining to address telomere length and species lifespans. For example, how can one explain the large variation in mouse species telomere lengths, and the lack of large variation in the lifespan of those species? For example, the Mus musculus castaneus mouse species has a telomere length of 18–20 kb (Hemann and Greider, 2000), and yet the lifespan of Mus musculus castaneous (≈681 days (Hemann and Greider, 2000)) is similar to the lifespan of other mouse species such as C57BL/6J (≈767 days (Hemann and Greider, 2000)) which can have telomere lengths of 40–50 kb (Zijlmans et al., 1997; Hemann and Greider, 2000; Vera et al., 2012; Varela et al., 2016). A thorough investigation comparing initial telomere length, telomere shortening rate, percentage of short telomeres, the length of the shortest telomeres, and other aging markers such as DNA methylation may provide insights. Another caveat is that telomeres are often measured from circulating lymphocytes in the blood, which may not be the best cell type for these measurements (Fossel, 2012). Additional Research Topics of interest are more thorough investigations of outlier species which have much longer lifespans than expected such as the naked mole rat which can live 31 years (Buffenstein, 2005) compared to the mouse which lives about 2 years (Hemann and Greider, 2000), or the bat, which can live up to 37 years in some species (de Magalhães et al., 2007) even though a bat is about the same size as a mouse. Overall, a complete understanding of telomere dynamics and species lifespan requires further studies and discoveries.
KW and MF wrote this editorial.
MF is founder of a biotech company targeting telomerase therapy.
The remaining author declares that the research was conducted in the absence of any commercial or financial relationships that could be construed as a potential conflict of interest.
All claims expressed in this article are solely those of the authors and do not necessarily represent those of their affiliated organizations, or those of the publisher, the editors and the reviewers. Any product that may be evaluated in this article, or claim that may be made by its manufacturer, is not guaranteed or endorsed by the publisher.
Blasco, M. A., Lee, H. W., Hande, M. P., Samper, E., Lansdorp, P. M., DePinho, R. A., et al. (1997). Telomere shortening and tumor formation by mouse cells lacking telomerase RNA. Cell 91, 25–34. doi:10.1016/s0092-8674(01)80006-4
Buffenstein, R. (2005). The naked mole-rat: A new long-living model for human aging research. J. Gerontol. A Biol. Sci. Med. Sci. 60, 1369–1377. doi:10.1093/gerona/60.11.1369
Carrel, A. O. N. (1912). The permanent life of tissues outside of the organism. J. Exp. Med. 15, 516–528. doi:10.1084/jem.15.5.516
Cooke, H. J., and Smith, B. A. (1986). Variability at the telomeres of the human X/Y pseudoautosomal region. Cold Spring Harb. Symp. Quant. Biol. 51, 213–219. doi:10.1101/sqb.1986.051.01.026
de Magalhães, J. P., Costa, J., and Church, G. M. (2007). An analysis of the relationship between metabolism, developmental schedules, and longevity using phylogenetic independent contrasts. J. Gerontol. A Biol. Sci. Med. Sci. 62, 149–160. doi:10.1093/gerona/62.2.149
Fossel, M. (2012). Use of telomere length as a biomarker for aging and age-related disease. Curr. Transl. Geriatr. Exp. Gerontol. Rep. 1, 121–127. doi:10.1007/s13670-012-0013-6
Greider, C. W., and Blackburn, E. H. (1989). A telomeric sequence in the RNA of Tetrahymena telomerase required for telomere repeat synthesis. Nature 337, 331–337. doi:10.1038/337331a0
Greider, C. W., and Blackburn, E. H. (1985). Identification of a specific telomere terminal transferase activity in Tetrahymena extracts. Cell 43, 405–413. doi:10.1016/0092-8674(85)90170-9
Hayflick, L., and Moorhead, P. S. (1961). The serial cultivation of human diploid cell strains. Exp. Cell Res. 25, 585–621. doi:10.1016/0014-4827(61)90192-6
Hemann, M. T., and Greider, C. W. (2000). Wild-derived inbred mouse strains have short telomeres. Nucleic Acids Res. 28, 4474–4478. doi:10.1093/nar/28.22.4474
Lee, H.-W., Blasco, M. A., Gottlieb, G. J., Horner, J. W., Greider, C. W., and DePinho, R. A. (1998). Essential role of mouse telomerase in highly proliferative organs. Nature 392, 569–574. doi:10.1038/33345
Levine, H. J. (1997). Rest heart rate and life expectancy. J. Am. Coll. Cardiol. 30, 1104–1106. doi:10.1016/s0735-1097(97)00246-5
Miller, R. A., Chrisp, C., and Atchley, W. (2000). Differential longevity in mouse stocks selected for early life growth trajectory. J. Gerontol. A Biol. Sci. Med. Sci. 55, B455–B461. doi:10.1093/gerona/55.9.b455
Rudolph, K. L., Chang, S., Lee, H. W., Blasco, M., Gottlieb, G. J., Greider, C., et al. (1999). Longevity, stress response, and cancer in aging telomerase-deficient mice. Cell 96, 701–712. doi:10.1016/s0092-8674(00)80580-2
Samaras, T. T., Elrick, H., and Storms, L. H. (2003). Is height related to longevity? Life Sci. 72, 1781–1802. doi:10.1016/s0024-3205(02)02503-1
Selman, C., Nussey, D. H., and Monaghan, P. (2013). Ageing: It’s a dog’s life. Curr. Biol. 23, R451–R453. doi:10.1016/j.cub.2013.04.005
Strehler, B. L. (2000). Understanding aging. Methods Mol. Med. 38, 1–19. doi:10.1385/1-59259-070-5:1
Szostak, J. W., and Blackburn, E. H. (1982). Cloning yeast telomeres on linear plasmid vectors. Cell 29, 245–255. doi:10.1016/0092-8674(82)90109-x
Varela, E., Muñoz-Lorente, M. A., Tejera, A. M., Ortega, S., and Blasco, M. A. (2016). Generation of mice with longer and better preserved telomeres in the absence of genetic manipulations. Nat. Commun. 7, 11739. doi:10.1038/ncomms11739
Vera, E., Bernardes de Jesus, B., Foronda, M., Flores, J. M., and Blasco, M. A. (2012). The rate of increase of short telomeres predicts longevity in mammals. Cell Rep. 2, 732–737. doi:10.1016/j.celrep.2012.08.023
Watson, J. D. (1972). Origin of concatemeric T7 DNA. Nat. New Biol. 239, 197–201. doi:10.1038/newbio239197a0
Whittemore, K., Vera, E., Martínez-Nevado, E., Sanpera, C., and Blasco, M. A. (2019). Telomere shortening rate predicts species life span. Proc. Natl. Acad. Sci. 116, 15122–15127. doi:10.1073/pnas.1902452116
Keywords: telomeres, species lifespan, body mass, telomere shortening rate, cellular senescence
Citation: Whittemore K and Fossel M (2023) Editorial: Telomere length and species lifespan. Front. Genet. 14:1199667. doi: 10.3389/fgene.2023.1199667
Received: 03 April 2023; Accepted: 04 April 2023;
Published: 17 April 2023.
Edited and reviewed by:
Blanka Rogina, University of Connecticut Health Center, United StatesCopyright © 2023 Whittemore and Fossel. This is an open-access article distributed under the terms of the Creative Commons Attribution License (CC BY). The use, distribution or reproduction in other forums is permitted, provided the original author(s) and the copyright owner(s) are credited and that the original publication in this journal is cited, in accordance with accepted academic practice. No use, distribution or reproduction is permitted which does not comply with these terms.
*Correspondence: Kurt Whittemore, a3VydHdoaXR0ZW1vcmVAZ21haWwuY29t; Michael Fossel, bWljaGFlbC5mb3NzZWxAdGVsb2N5dGUuY29t
Disclaimer: All claims expressed in this article are solely those of the authors and do not necessarily represent those of their affiliated organizations, or those of the publisher, the editors and the reviewers. Any product that may be evaluated in this article or claim that may be made by its manufacturer is not guaranteed or endorsed by the publisher.
Research integrity at Frontiers
Learn more about the work of our research integrity team to safeguard the quality of each article we publish.