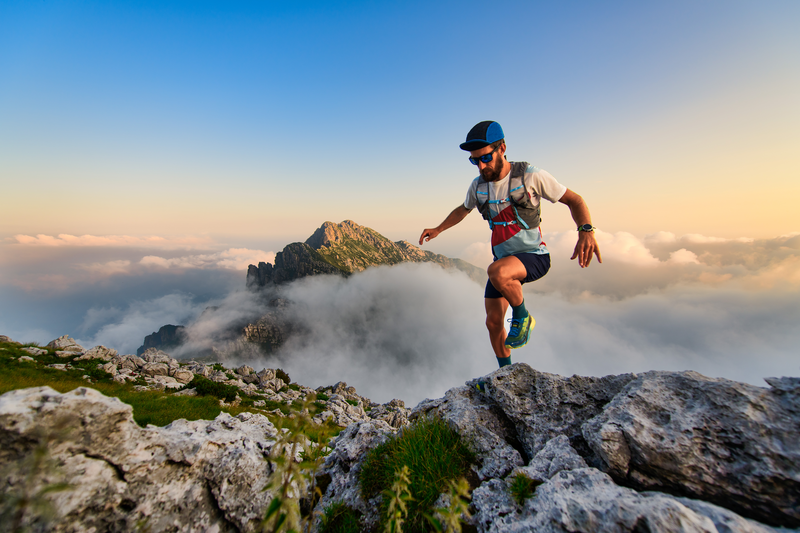
94% of researchers rate our articles as excellent or good
Learn more about the work of our research integrity team to safeguard the quality of each article we publish.
Find out more
EDITORIAL article
Front. Genet. , 21 February 2023
Sec. Livestock Genomics
Volume 14 - 2023 | https://doi.org/10.3389/fgene.2023.1155201
This article is part of the Research Topic Genetic Engineering in Farm Animals View all 7 articles
Editorial on the Research Topic
Genetic engineering in farm animals
Worldwide agriculture faces enormous challenges. Global warming, a growing world population with a constantly growing need for high-quality food, increasing demand for animal welfare, and reducing the ecological footprint represent the greatest challenges for the present and the near future.
Deciphering the function of the CRISPR-Cas9 system and adapting it for genome editing of the mammalian genome has revolutionized the genetic engineering of farm animals and offers novel opportunities to address these challenges (Jinek et al., 2012; Cong et al., 2013; Jinek et al., 2013). Besides their use for food production, livestock serves as an important animal model for human diseases and potential organ donors as they share high similarities with humans regarding physiology, anatomy, genetics, and size. This Frontiers in Genetics Research Topic includes six manuscripts that span from gene-edited pigs that avoid castration to prevent boar taint to basic research to identify potential targets for genetic engineering in chickens to increase the stability of eggshells. The castration of piglets with or without anesthesia is increasingly being criticized as it is associated with pain, stress, and an increased risk of infection. Disrupting porcine Kisspeptin-1 (KISS1) is hypothesized to delay or abolish puberty by inducing variable hypogonadotropism and thus preventing the need for castration. To test this hypothesis, Florez et al. generated the first KISS1-edited large animal using CRISPR/Cas9-ribonucleoproteins and single-stranded donor oligonucleotides. Though follow-on research will be necessary to evaluate the efficacy of this approach, this proof-of-principle study gives a good example of how genetic engineering could be employed to address urgent problems in livestock production. The knockout of Myostatin (MSTN), a negative regulator of muscle growth, is accompanied by a significant increase in skeletal muscle mass and has been discussed to increase the meat yield from livestock species (Crispo et al., 2015; Wang et al., 2015; Yu et al., 2016; Wang and Petersen, 2022). Several MSTN-KO livestock have been produced, but the visceral development remains unclear. Pei et al. provide the first evidence that MSTN-KO pigs show no significant difference in the size and average weight or length of visceral organs in adult pigs. These findings are important for the further application of this technology.
The importance of genetically engineered livestock is further displayed in the article by Davies et al. that generated a unique type I Interferon Receptor knockout sheep model for viral immunology and reproductive signaling. The mini-review by Greising et al. reviews the recent developments and options for the treatment of volumetric muscle loss in gene-edited pigs, while Ruan et al. provide a comprehensive opinion on the recent developments in altering the pig genome.
The establishment and culture of PGCs enabled the targeted genetic modification of the chicken genome to address productive traits such as eggshell strength and thickness which are critical factors in reducing egg breaking and preventing economic losses (Oishi et al., 2016; Atsuta et al., 2022). To identify potential targets for genetic engineering, Wu et al. employed transcriptional sequencing and proteomics to investigate the differences between the uteruses of laying hens with high- and low-breaking eggshells. They identified the KRT14 gene which may promote calcium metabolism and the deposition of calcium carbonate in eggshells.
Since its advent, CRISPR-Cas9 has proven its disruptive potential for animal breeding. A multiverse of different CRISPR variants has been developed in the last 10 years increasing the efficiency of precisely altering the genome of livestock species and reducing the number of unwanted mutations (Cong et al., 2013; Fu et al., 2014; Kleinstiver et al., 2015; Kleinstiver et al., 2016; Anzalone et al., 2019; Walton et al., 2020). Except for the European Union, most countries worldwide follow liberal regulations for novel breeding tools and consider approval of gene-edited livestock to integrate them into breeding programs to produce food for human consumption. A legal and ethical debate is still ongoing, but the assessment of genetically modified farm animals is moving more and more from a technology-based to a product-based risk assessment. With all the uncertainties and risks associated with the use of genetic engineering, we should also consider the risks of not using the new breeding technologies.
BP wrote the article and revised the article.
The author declares that the research was conducted in the absence of any commercial or financial relationships that could be construed as a potential conflict of interest.
All claims expressed in this article are solely those of the authors and do not necessarily represent those of their affiliated organizations, or those of the publisher, the editors and the reviewers. Any product that may be evaluated in this article, or claim that may be made by its manufacturer, is not guaranteed or endorsed by the publisher.
Anzalone, A. V., Randolph, P. B., Davis, J. R., Sousa, A. A., Koblan, L. W., Levy, J. M., et al. (2019). Search-and-replace genome editing without double-strand breaks or donor DNA. Nature 576, 149–157. doi:10.1038/s41586-019-1711-4
Atsuta, Y., Suzuki, K., Iikawa, H., Yaguchi, H., and Saito, D. (2022). Prime editing in chicken fibroblasts and primordial germ cells. Dev. growth & Differ. 64, 548–557. doi:10.1111/dgd.12823
Cong, L., Ran, F. A., Cox, D., Lin, S., Barretto, R., Habib, N., et al. (2013). Multiplex genome engineering using CRISPR/Cas systems. Science 339, 819–823. doi:10.1126/science.1231143
Crispo, M., Mulet, A. P., Tesson, L., Barrera, N., Cuadro, F., dos Santos-Neto, P. C., et al. (2015). Efficient generation of myostatin knock-out sheep using CRISPR/Cas9 technology and microinjection into zygotes. Plos One 10, e0136690. doi:10.1371/journal.pone.0136690
Fu, Y., Sander, J. D., Reyon, D., Cascio, V. M., and Joung, J. K. (2014). Improving CRISPR-Cas nuclease specificity using truncated guide RNAs. Nat. Biotechnol. 32, 279–284. doi:10.1038/nbt.2808
Jinek, M., Chylinski, K., Fonfara, I., Hauer, M., Doudna, J. A., and Charpentier, E. (2012). A programmable dual-RNA-guided DNA endonuclease in adaptive bacterial immunity. Science 337, 816–821. doi:10.1126/science.1225829
Jinek, M., East, A., Cheng, A., Lin, S., Ma, E., and Doudna, J. (2013). RNA-programmed genome editing in human cells. eLife 2, e00471. doi:10.7554/eLife.00471
Kleinstiver, B. P., Pattanayak, V., Prew, M. S., Tsai, S. Q., Nguyen, N. T., Zheng, Z., et al. (2016). High-fidelity CRISPR-Cas9 nucleases with no detectable genome-wide off-target effects. Nature 529, 490–495. doi:10.1038/nature16526
Kleinstiver, B. P., Prew, M. S., Tsai, S. Q., Topkar, V. V., Nguyen, N. T., Zheng, Z., et al. (2015). Engineered CRISPR-Cas9 nucleases with altered PAM specificities. Nature 523, 481–485. doi:10.1038/nature14592
Oishi, I., Yoshii, K., Miyahara, D., Kagami, H., and Tagami, T. (2016). Targeted mutagenesis in chicken using CRISPR/Cas9 system. Sci. Rep. 6, 23980. doi:10.1038/srep23980
Walton, R. T., Christie, K. A., Whittaker, M. N., and Kleinstiver, B. P. (2020). Unconstrained genome targeting with near-PAMless engineered CRISPR-Cas9 variants. Science 368, 290–296. doi:10.1126/science.aba8853
Wang, K. K., Ouyang, H. S., Xie, Z. C., Yao, C. G., Guo, N. N., Li, M. J., et al. (2015). Efficient generation of myostatin mutations in pigs using the CRISPR/Cas9 system. Sci. Rep. 5, 16623. doi:10.1038/srep16623
Wang, X., and Petersen, B. (2022). More abundant and healthier meat: Will the MSTN editing epitome empower the commercialization of gene editing in livestock? Science China. Life Sci. 65, 448–450. doi:10.1007/s11427-021-1980-4
Keywords: genetic engineering, CRISPR-Cas, livestock, gene editing, MSTN, IFNAR, eggshell
Citation: Petersen B (2023) Editorial: Genetic engineering in farm animals. Front. Genet. 14:1155201. doi: 10.3389/fgene.2023.1155201
Received: 31 January 2023; Accepted: 03 February 2023;
Published: 21 February 2023.
Edited and reviewed by:
Martino Cassandro, University of Padua, ItalyCopyright © 2023 Petersen. This is an open-access article distributed under the terms of the Creative Commons Attribution License (CC BY). The use, distribution or reproduction in other forums is permitted, provided the original author(s) and the copyright owner(s) are credited and that the original publication in this journal is cited, in accordance with accepted academic practice. No use, distribution or reproduction is permitted which does not comply with these terms.
*Correspondence: Bjoern Petersen, YmpvZXJuLnBldGVyc2VuQGZsaS5kZQ==
Disclaimer: All claims expressed in this article are solely those of the authors and do not necessarily represent those of their affiliated organizations, or those of the publisher, the editors and the reviewers. Any product that may be evaluated in this article or claim that may be made by its manufacturer is not guaranteed or endorsed by the publisher.
Research integrity at Frontiers
Learn more about the work of our research integrity team to safeguard the quality of each article we publish.