- 1Department of Biomedical Engineering, Osaka Institute of Technology, Osaka, Japan
- 2Department of Biosignaling and Radioisotope Experiment, Interdisciplinary Center for Science Research, Head Office for Research and Academic Information, Shimane University, Izumo, Japan
Tenascin-X (TNX) is an extracellular matrix glycoprotein for which a deficiency results in a recessive form of classical-like Ehlers-Danlos syndrome (clEDS), a heritable connective tissue disorder with hyperextensible skin without atrophic scarring, joint hypermobility, and easy bruising. Notably, patients with clEDS also suffer from not only chronic joint pain and chronic myalgia but also neurological abnormalities such as peripheral paresthesia and axonal polyneuropathy with high frequency. By using TNX-deficient (Tnxb−/−) mice, well-known as a model animal of clEDS, we recently showed that Tnxb−/− mice exhibit hypersensitivity to chemical stimuli and the development of mechanical allodynia due to the hypersensitization of myelinated A-fibers and activation of the spinal dorsal horn. Pain also occurs in other types of EDS. First, we review the underlying molecular mechanisms of pain in EDS, especially that in clEDS. In addition, the roles of TNX as a tumor suppressor protein in cancer progression have been reported. Recent in silico large-scale database analyses have shown that TNX is downregulated in various tumor tissues and that high expression of TNX in tumor cells has a good prognosis. We describe what is so far known about TNX as a tumor suppressor protein. Furthermore, some patients with clEDS show delayed wound healing. Tnxb−/− mice also exhibit impairment of epithelial wound healing in corneas. TNX is also involved in liver fibrosis. We address the molecular mechanism for the induction of COL1A1 by the expression of both a peptide derived from the fibrinogen-related domain of TNX and integrin α11.
Introduction
The Ehlers-Danlos syndromes (EDS) comprise a group of rare heritable connective tissue disorders mainly characterized by a variable degree of joint hypermobility, hyperextensible skin and fragility of connective tissues. Currently, 14 EDS are classified according to typical clinical features, and 20 causal genes that are mainly responsible for collagen and extracellular matrix (ECM) synthesis and maintenance have been identified (Malfait et al., 2020). Among the 14 types of EDS, non-collagenous classical-like EDS (clEDS) is the result of tenascin-X (TNX) deficiency with homozygous or compound heterozygous mutations in its gene (TNXB) (Burch et al., 1997; Schalkwijk et al., 2001; Malfait et al., 2017). The major clinical features of clEDS are generalized joint hypermobility, hyperextensible velvety skin without atrophic scarring, and easy bruising (Malfait et al., 2017) (Figure 1A).
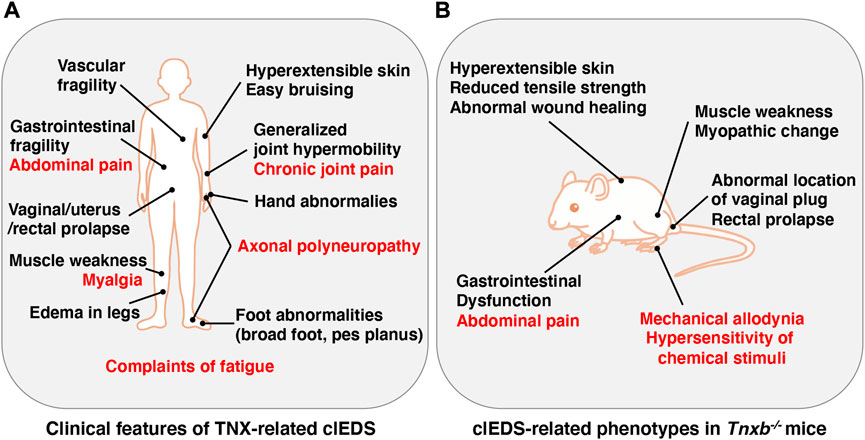
FIGURE 1. Clinical features of TNX-related clEDS (A) and clEDS-related phenotypes of Tnxb−/− mice (B). Symptoms in many patients with TNX-related clEDS are shown in (A) (van Dijk et al., 2022). Major clEDS-related phenotypes exhibited in Tnxb−/− mice are shown in (B) (Matsumoto and Aoki, 2020). Complaints associated with pain are highlighted by red letters in (A) and (B).
A causal gene for clEDS, TNXB, was identified serendipitously as an opposite strand gene (OSG) with its 3′ genomic overlap with the steroid 21-hydroxylase gene (CYP21A2) in the human major histocompatibility complex (MHC) class III region (Morel et al., 1989). Further independent analyses of the MHC class III region revealed a novel gene having the highest homology with tenascin-C (TNC) and the OSG is the portion of the 3’ region of the gene, naming the novel gene TNXB (Matsumoto et al., 1992a; Matsumoto et al., 1992b; Bristow et al., 1993; Erickson, 1993). TNX is the largest glycoprotein in the tenascin family with a size of roughly 450 kDa and is composed of characteristic structural domains with a tenascin assemble region, heptad repeats, epidermal growth factor (EGF)-like repeats, fibronectin type III (FNIII)-like repeats, and a fibrinogen (FBG)-related domain (Bristow et al., 1993; Ikuta et al., 1998).
TNX is expressed prominently in a variety of tissues including the heart, skin, skeletal muscle, peripheral nerves, ligaments, tendons and the digestive tract, while there are very low expression levels in immune tissues such as the thymus, bone marrow and lymphocytes (Matsumoto et al., 1994; Geffrotin et al., 1995). Brain-derived neurotrophic factor (BDNF) has been identified as an up-regulator of TNX expression (Takeda et al., 2005) and glucocorticoids have been identified as a down-regulators of TNX expression (Sakai et al., 1996).
TNX has physiological functions in collagen deposition (Mao et al., 2002; Minamitani et al., 2004a), collagen stability (Mao and Bristow, 2001), physical property of collagen (Margaron et al., 2010) and collagen fibrillogenesis (Minamitani et al., 2004b; Egging et al., 2007). Several phenotypes tied to the function of TNX have been revealed by using Tnxb−/− mice (Matsumoto and Aoki, 2020) (Figure 1B).
In this review, we focus on the function of TNX in pain related to a characteristic of clEDS as well as in tumor suppression and fibrosis.
Clinical characteristics of TNX-related clEDS
TNX-related clEDS was identified in 56 individuals from 44 families so far (van Dijk et al., 2022). The major clinical characteristics of TNX-related clEDS are skin hyperextensibility with velvety skin texture and absence of atrophic scaring (100% of patients), generalized joint hypermobility with or without recurrent dislocations (100%), and easy or spontaneous bruising of the skin including hematomas and ecchymoses (91%), as shown in Figure 1A (Malfait et al., 2017; van Dijk et al., 2022). It has been considered that the absence of atrophic scaring is a characteristic of clEDS, distinguish it from classical EDS, but mild atrophic scarring was observed in seven clEDS patients (Chen et al., 2016; Green et al., 2020). Additional musculoskeletal presentations of TNX-related clEDS are foot abnormalities including broad/plump forefoot, brachydactyly with excessive skin, pes planus, hallux valgus, and painful soles of the feet (81%), edema in the legs in the absence of cardiac failure (25%), hand anomalies (20%), and complaints of fatigue (53%) (van Dijk et al., 2022). Cardiovascular presentations of TNX-related clEDS are vascular fragility (27%), mild valvular abnormality (16%), and cardiomyopathy (5%) (van Dijk et al., 2022). Vascular fragility has been reported to cause major medical events such as rupture of the brachial vein and aneurysmal abdominal arteries (Demirdas et al., 2017; Micale et al., 2019). Neuromuscular presentations of TNX-related clEDS are subjective muscle weakness (37%), axonal polyneuropathy (14%), and atrophy of muscles in the hands and feet (4%) (van Dijk et al., 2022). Voermans et al. (2009) reported that TNX-deficient EDS patients show muscle weakness, myalgia, easy fatigability, and limited walking distance. Physical examination revealed mild-to-moderate muscle weakness, hypotonia, reduction of vibration sense, hyporeflexia, and impairment of mobility. Furthermore, clinical neurological studies showed axonal polyneuropathy and mild abnormal motor unit action potentials, and muscle ultrasound showed increased echo intensity and atrophy (Voermans et al., 2009). Interestingly, neuromuscular features have been observed in adults but not in children (Demirdas et al., 2017). Other presentations of TNX-related clEDS are gastrointestinal fragility including esophageal, small bowel and/or large bowel ruptures (16%), vaginal/uterus/rectal prolapse (21%), and other types of fragility including trachea rupture after intubation and defect of nasal cartilages after nose blowing (4%) (van Dijk et al., 2022).
Pain in clEDS due to TNX deficiency
Pain is a common and severe symptom in patients with various types of EDS (Chopra et al., 2017; Syx et al., 2017; Malfait et al., 2021). Pain initially occurs as acute and localized musculoskeletal nociception in different joints and limbs in relation to hypermobility, subluxations, dislocations, soft-tissue injury, myalgias, and surgery (Chopra et al., 2017). However, pain related to EDS gradually becomes chronic (lasting for longer than 3 months) and assumes a more generalized distribution (Chopra et al., 2017; Syx et al., 2017). Among the various types of EDS, chronic pain is most frequent in hypermobile EDS. Chopra et al. (2017) reported that pain in patients with hypermobile EDS occurs in various forms including generalized body pain (incidence of 90%), soft-tissue pain (90%), dislocations (78%), and joint pain including pain in the shoulders (80%), hands (75%), knees (71%), temporomandibular joints (71%), spine (67%), and elbows (43%). In addition to musculoskeletal pain, patients with hypermobile EDS suffer from chronic fatigue (95%), neuropathic pain (68%), headaches (75%), gastrointestinal pain (86%), dysmenorrhea (73%), and vulvodynia/dyspareunia (42%). Pathological chronic pain is also caused by a lesion or disease of the somatosensory nervous system, and that pain is called neuropathic pain (Jensen et al., 2011). There is a high frequency of neuropathic pain in patients with EDS who have chronic pain (Chopra et al., 2017). Neuropathic pain occurs as spontaneous pain such as shooting, and burning, or stabbing pain, an increased response to normally noxious stimuli (hyperalgesia), and pain due to normally innocuous stimuli (allodynia) (Colloca et al., 2017).
It has been reported that TNX-related clEDS patients complain of chronic pain including joint pain, myalgia, back pain, abdominal pain, and fatigue (Figure 1A) (Schalkwijk et al., 2001; Voermans et al., 2009; Demirdas et al., 2017; Green et al., 2020; van Dijk et al., 2022). We first reported pain responses in a murine TNX-deficient EDS model (Okuda-Ashitaka et al., 2020). Our studies with Tnxb−/− mice showed increased sensitivity to innocuous mechanical stimuli but not to thermal stimuli such as cold and heat, suggesting that TNX deficiency is involved in the development of mechanical allodynia, a major feature of neuropathic pain (Figure 1B) (Okuda-Ashitaka et al., 2020). Furthermore, Tnxb−/− mice also exhibited hypersensitization of myelinated Aδ- and Aβ-fibers, but not unmyelinated C fibers, by using transcutaneous sine wave stimuli (Figure 2). TNX is highly expressed in tendons, ligaments, and peripheral nerves (Geffrotin et al., 1995). TNX exists in the perineurium, endoneurium, and Schwann cells in the sciatic nerve (Matsumoto et al., 2002; Sakai et al., 2017; Okuda-Ashitaka et al., 2020). Electron microscopy analysis of the sciatic nerve showed modestly smaller inner and outer diameters of myelinated fibers and reduced collagen fibril density in the endoneurium in Tnxb−/− mice (Voermans et al., 2011), whereas there was no significant difference in the numbers of axons or thickness of the myelin sheaths in Tnxb−/− mice (Matsumoto et al., 2002). Moderate changes of myelinated fibers and hypersensitization of myelinated Aδ- and Aβ-fibers in Tnxb−/− mice may be correlated to the axonal polyneuropathy in TNX-deficient EDS (Voermans et al., 2009). Axonal polyneuropathy is thought to be one of the mechanisms of neuropathic pain in EDS (Voermans et al., 2011). Furthermore, Tnxb−/− mice showed increased levels of anatomical neuronal activation markers, phosphorylated extracellular signal-regulated kinase and neuronal nitric oxide in the spinal dorsal horn, indicating that TNX deficiency induces spinal central sensitization, namely, another mechanism of neuropathic pain (Okuda-Ashitaka et al., 2020). Similar to pain responses in Tnxb−/− mice, a murine classical EDS model, type V collagen (COL5A1) haploinsufficient (Col5a1+/−) mice, showed mechanical allodynia but not thermal hyperalgesia (Syx et al., 2020). Col5a1+/− mice showed a disorganization of Nav1.8-expressing fibers including above 90% C-fibers, with less fibers crossing the epidermis of footpad glabrous skin. These results indicated that pain in both TNX-related clEDS and COL5A1-related classical EDS corresponds to neuropathic pain associated with hypersensitization of myelinated Aδ- and Aβ-fibers, disorganization of Nav1.8-expressing fibers, and central sensitization of the spinal cord.
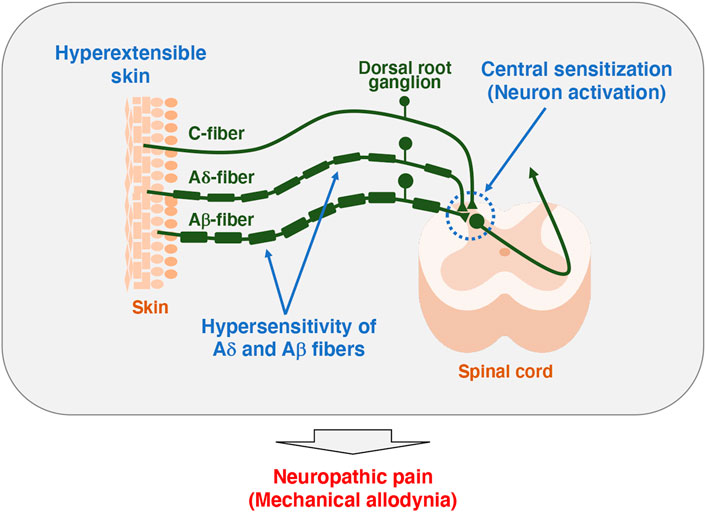
FIGURE 2. Model of pathogenesis for mechanical allodynia in Tnxb−/− mice. Somatosensory information is detected in the primary afferent fibers extending to the skin, which in turn is transmitted to the spinal cord and then to the brain. Unmyelinated C-fibers and lightly myelinated Aδ-fibers conduct noxious and thermal signals, whereas myelinated Aβ-fibers conduct innocuous signals such as touch and pressure (Moehring et al., 2018). Tnxb−/− mice exhibited increased sensitivity to innocuous mechanical stimuli but not thermal stimuli, indicating the induction of mechanical allodynia (Okuda-Ashitaka et al., 2020). Likewise, Tnxb−/− mice showed hypersensitization of myelinated Aδ- and Aβ-fibers but not C-fibers. Furthermore, levels of activated neuron markers, phosphorylation of extracellular signal-related kinase and NADPH-diaphorase activity of neuronal nitric oxide were increased in the spinal dorsal horn of Tnxb−/− mice compared to those in wild-type mice. Thus, TNX deficiency is involved in mechanical allodynia associated with hypersensitization of myelinated Aδ- and Aβ-fibers and central sensitization of the spinal cord.
Additionally, TNX influences neuronal functions in gut tissues including abdominal pain (Figure 1A). Tnxb−/− mice show hypersensitivity of colonic nociceptive afferents and increased sensory neuron sprouting in the mucosa (Aktar et al., 2018). Tnxb−/− mice also exhibited gastric dysfunction associated with accelerated gastric emptying and hypersensitivity of gastric vagal mechanoreceptors (Figure 1B) (Aktar et al., 2019), which are consistent with TNX-related clEDS patients (Schalkwijk et al., 2001; Lindor and Bristow, 2005).
TNX with tumor suppressive function
Previously, we demonstrated that Tnxb−/− mice bearing aggressive B16-BL6 melanoma cells exhibit promotion of tumor invasion and metastasis due to upregulation of matrix metalloproteinases Mmp2 and Mmp9 followed by enhanced activities of the MMPs (Matsumoto et al., 2001; Matsumoto et al., 2004). Conversely, overexpression of TNX in fibroblasts downregulated the expression of Mmp2 (Matsumoto et al., 2004). In addition, silencing of long non-coding RNA (LncRNA) LINC01305 inhibited the progression of lung cancer by activating the TNX-mediated phosphatidylinositol 3-kinase (PI3K)/protein kinase B (Akt) signaling pathway (Yan et al., 2020). Moreover, it has been revealed that a functional variant in the TNXB promoter is associated with risk of esophageal squamous-cell carcinoma (ESCC) in the Chinese population, leading to the expression of TNXB being downregulated in ESCC tissues (Chang et al., 2018; Yang et al., 2020). Knockout of TNXB significantly increased cell proliferation of ESCC cells (Yang et al., 2020). These results suggest that TNX has a tumor suppressor role. Interestingly, when carcinoma cells were transplanted into the skin of nude mice, the expression of TNX was downregulated substantially not only in the transplanted tumor cells themselves but also in the surrounding tumor stroma (Sakai et al., 1996).
In conjunction with a tumor suppressor role of TNX, the expression of TNX was shown to be downregulated in most tumor tissues such as the lung, breast, prostate, colon, stomach, liver, kidney, skin melanoma, and leiomyoma by using in silico large database studies of the Gene Expression Omnibus (GEO) and The Cancer Genomic Atlas (TCGA) (Liot et al., 2020), although there are some discrepancies in the expression pattern of TNX in glioma and ovarian cancer compared with those of previous published data (Hasegawa et al., 1997; Kramer et al., 2015). In another study using the TCGA database for ECM gene dysregulation in cancer, 58 out of 249 ECM genes were identified as cancer-associated ECM genes and TNXB was found to be the most significantly downregulated among those genes in cancers (Chakravarthy et al., 2018). Even more interesting is that TNXB expression is inversely correlated with tumor progression and that a high level of TNX in tumor tissues predicts a good prognosis (Liot et al., 2020).
Meanwhile, as an exception, the expression of TNX is upregulated in malignant mesothelioma (Yuan et al., 2009; Davidson, 2011; Nakayama et al., 2019). This evidence suggests that TNX is applicable as a diagnostic marker of malignant mesothelioma since most other tumors are negative for TNX expression.
Involvement of TNX in fibrosis and wound healing
Alcaraz et al. (2014) showed that a fibrinogen (FBG)-related domain of TNX (TNX-FBG) interacts with small latent TGF-β complex (SLC) and elicits the activation of its latent form into a bioactive form with integrin α11β1, leading to epithelial-to-mesenchymal transition in mammary epithelial cells. On the other hand, Liang et al. (2022) recently demonstrated that TNX-FBG interacts with mature TGF-β and impedes it from binding to its receptor, mediating flow-inducing suppression of endothelial-to-mesenchymal transition and atherosclerosis.
Previously, our group revealed that Tnxb−/− mice fed a high-fat and high-cholesterol diet with high levels of phosphorus and calcium (HFCD) exhibit less fibrotic characteristics in livers than those in wild-type mice, indicating the involvement of TNX in hepatic fibrosis (Yamaguchi et al., 2017). Fibrosis is a pathological sign of wound healing that replaces damaged tissue with collagen-rich scar tissue. We attempted to disclose the molecular mechanism by which TNX induces in vitro fibrosis such as the induction of type I collagen 1α (COL1A1) expression. Initially, we speculated that TGF-β and TNX-FBG with integrin α11β1 are involved in the induction of COL1A1 expression since interaction of the TNX-FBG domain and TGF-β was reported previously (Alcaraz et al., 2014) and TGF-β is a well-known central mediator of fibrosis (Dewidar et al., 2019). However, contrary to our initial expectation, we found that the Yes-associated protein 1 (YAP1) signaling pathway through integrin α11β1 plays a major role in the induction of COL1A1 expression by expression of the TNX-FBG domain in human hepatic stellate LX-2 cells and that the minimum 15-amino acid (aa) sequence derived from the TNX-FBG domain is required for the induction of COL1A1 expression in the LX-2 cells (Matsumoto et al., 2022). Since integrin α11β1 is known to be a receptor for type I collagen (COL1) and type II collagen (Zhang et al., 2003), it is yet to be determined whether interaction of the TNX-FBG domain with integrin α11β1 is direct or indirect for the induction of COL1A1expression.
According to previous reports, 41% of patients with TNX-deficient clEDS showed delayed wound healing (Demirdas et al., 2017). Notably, the corneas of Tnxb−/− mice that underwent epithelium debridement exhibited impairment of epithelial wound healing due to increased neutrophil infiltration and activation of reactive oxygen species (Sumioka et al., 2021). TNX might also be involved in the angiogenetic process during wound healing. Injury-induced corneal stromal angiogenesis in Tnxb−/− mice was impaired (Sumioka et al., 2018).
Conclusion and perspectives
In this review, we described the molecular mechanisms of pain caused by TNX deficiency as well as by mutation of collagens mimicking the characteristics of EDS, the function of TNX as a tumor suppressor, and the involvement of TNX in fibrosis.
Concerning pain associated with malfunction of the ECM, the contribution of TNX-deficient clEDS and COL5A1 haploinsufficiency-related classical EDS to the development of neuropathic pain has been revealed by using a murine EDS model. Patients with EDS take large amounts of medications such as acetaminophen, non-steroid anti-inflammatory drugs (NSAIDs), anticonvulsants, antidepressants, opioids, and lidocaine; however, current managements are inadequate (Demes et al., 2020; Whalen and Crone, 2022). Interestingly, mechanical allodynia in Tnxb−/− mice was inhibited by the anticonvulsant drug gabapentin and the mu-opioid agonist [D-Ala2, N-MePhe4, Gly-ol5]-enkephalin (DAMGO) but not by the NSAID indomethacin (Okuda-Ashitaka et al., 2020). In the future, more efficacious approaches in line with the mechanisms causing pain in patients with EDS are expected.
Concerning tumor progression associated with TNX expression, the increased expression of TNX in malignant mesothelioma is very interesting, despite its expression being downregulated in most tumor tissues. Yuan et al. (2009) showed some splice variants of TNX are observed in malignant mesothelioma. The splice variants of TNX might be involved in the malignancy of mesothelioma. In the future, analyses of not only splice variants of TNX itself but also proteins that interact with their splice variants are needed to reveal the specific function of TNX in malignant mesothelioma.
Finally, we showed that COL1A1 expression was induced by expression of both the 15-aa peptide in the TNX-FBG domain and integrin α11 in hepatic stellate LX-2 cells in vitro (Matsumoto et al., 2022). Further experiments are needed to determine whether expression of the 15-aa peptide from the TNX-FBG domain in liver can induce COL1A1 expression leading to hepatic fibrosis in vivo.
Author contributions
EO-A and KM designed and wrote the manuscript. All authors contributed to the article and approved the submitted version.
Funding
This work was supported by the Japan Society for the Promotion of Science (JSPS) KAKENHI Grant Number JP17K09045 and Osaka Institute of Technology Research Projects Grants to EO-A and by JSPS KAKENHI Grant Number JP19K08470 and a part of Management Expenses Grants to Shimane University to KM.
Conflict of interest
The authors declare that the research was conducted in the absence of any commercial or financial relationships that could be construed as a potential conflict of interest.
Publisher’s note
All claims expressed in this article are solely those of the authors and do not necessarily represent those of their affiliated organizations, or those of the publisher, the editors and the reviewers. Any product that may be evaluated in this article, or claim that may be made by its manufacturer, is not guaranteed or endorsed by the publisher.
References
Aktar, R., Peiris, M., Fikree, A., Cibert-Goton, V., Walmsley, M., Tough, I. R., et al. (2018). The extracellular matrix glycoprotein tenascin-X regulates peripheral sensory and motor neurones. J. Physiol. 596 (17), 4237–4251. doi:10.1113/jp276300
Aktar, R., Peiris, M., Fikree, A., Eaton, S., Kritas, S., Kentish, S. J., et al. (2019). A novel role for the extracellular matrix glycoprotein tenascin-X in gastric function. J. Physiol. 597 (6), 1503–1515. doi:10.1113/jp277195
Alcaraz, L. B., Exposito, J. Y., Chuvin, N., Pommier, R. M., Cluzel, C., Martel, S., et al. (2014). Tenascin-X promotes epithelial-to-mesenchymal transition by activating latent TGF-β. J. Cell Biol. 205 (3), 409–428. doi:10.1083/jcb.201308031
Bristow, J., Tee, M. K., Gitelman, S. E., Mellon, S. H., and Miller, W. L. (1993). Tenascin-X: A novel extracellular matrix protein encoded by the human xb gene overlapping P450c21B. J. Cell Biol. 122 (1), 265–278. doi:10.1083/jcb.122.1.265
Burch, G. H., Gong, Y., Liu, W., Dettman, R. W., Curry, C. J., Smith, L., et al. (1997). Tenascin-X deficiency is associated with Ehlers-Danlos syndrome. Nat. Genet. 17 (1), 104–108. doi:10.1038/ng0997-104
Chakravarthy, A., Khan, L., Bensler, N. P., Bose, P., and De Carvalho, D. D. (2018). TGF-β-associated extracellular matrix genes link cancer-associated fibroblasts to immune evasion and immunotherapy failure. Nat. Commun. 9 (1), 4692. doi:10.1038/s41467-018-06654-8
Chang, J., Zhong, R., Tian, J., Li, J., Zhai, K., Ke, J., et al. (2018). Exome-wide analyses identify low-frequency variant in CYP26B1 and additional coding variants associated with esophageal squamous cell carcinoma. Nat. Genet. 50 (3), 338–343. doi:10.1038/s41588-018-0045-8
Chen, W., Perritt, A. F., Morissette, R., Dreiling, J. L., Bohn, M. F., Mallappa, A., et al. (2016). Ehlers-Danlos syndrome caused by biallelic TNXB variants in patients with congenital adrenal hyperplasia. Hum. Mutat. 37 (9), 893–897. doi:10.1002/humu.23028
Chopra, P., Tinkle, B., Hamonet, C., Brock, I., Gompel, A., Bulbena, A., et al. (2017). Pain management in the Ehlers-Danlos syndromes. Am. J. Med. Genet. C Semin.Med. Genet. 175 (1), 212–219. doi:10.1002/ajmg.c.31554
Colloca, L., Ludman, T., Bouhassira, D., Baron, R., Dickenson, A. H., Yarnitsky, D., et al. (2017). Neuropathic pain. Nat. Rev. Dis. Prim. 3, 17002. doi:10.1038/nrdp.2017.2
Davidson, B. (2011). The diagnostic and molecular characteristics of malignant mesothelioma and ovarian/peritoneal serous carcinoma. Cytopathology 22 (1), 5–21. doi:10.1111/j.1365-2303.2010.00829.x
Demes, J. S., McNair, B., and Taylor, M. R. G. (2020). Use of complementary therapies for chronic pain management in patients with reported Ehlers-Danlos syndrome or hypermobility spectrum disorders. Am. J. Med. Genet. A 182 (11), 2611–2623. doi:10.1002/ajmg.a.61837
Demirdas, S., Dulfer, E., Robert, L., Kempers, M., van Beek, D., Micha, D., et al. (2017). Recognizing the tenascin-X deficient type of Ehlers-Danlos syndrome: A cross-sectional study in 17 patients. Clin. Genet. 91 (3), 411–425. doi:10.1111/cge.12853
Dewidar, B., Meyer, C., Dooley, S., and Meindl-Beinker, A. N. (2019). TGF-β in hepatic stellate cell activation and liver fibrogenesis-updated 2019. Cells 8 (11), 1419. doi:10.3390/cells8111419
Egging, D., van den Berkmortel, F., Taylor, G., Bristow, J., and Schalkwijk, J. (2007). Interactions of human tenascin-X domains with dermal extracellular matrix molecules. Arch. Dermatol. Res. 298 (8), 389–396. doi:10.1007/s00403-006-0706-9
Erickson, H. P. (1993). Tenascin-C, tenascin-R and tenascin-X: A family of talented proteins in search of functions. Curr. Opin. Cell Biol. 5 (5), 869–876. doi:10.1016/0955-0674(93)90037-q
Geffrotin, C., Garrido, J. J., Tremet, L., and Vaiman, M. (1995). Distinct tissue distribution in pigs of tenascin-X and tenascin-C transcripts. Eur. J. Biochem. 231 (1), 83–92. doi:10.1111/j.1432-1033.1995.tb20673.x
Green, C., Ghali, N., Akilapa, R., Angwin, C., Baker, D., Bartlett, M., et al. (2020). Classical-like Ehlres-Danlos syndrome: A clinical description of 20 newly identified individuals with evidence of tissue fragility. Genet. Med. 22 (10), 1576–1582. doi:10.1038/s41436-020-0850-1
Hasegawa, K., Yoshida, T., Matsumoto, K., Katsuta, K., Waga, S., and Sakakura, T. (1997). Differential expression of tenascin-C and tenascin-X in human astrocytomas. Acta Neuropathol. 93 (5), 431–437. doi:10.1007/s004010050636
Ikuta, T., Sogawa, N., Ariga, H., Ikemura, T., and Matsumoto, K. (1998). Structural analysis of mouse tenascin-X: Evolutionary aspects of reduplication of FNIII repeats in the tenascin gene family. Gene 217 (1-2), 1–13. doi:10.1016/s0378-1119(98)00355-2
Jensen, T. S., Baron, R., Haanpää, M., Kalso, E., Loeser, J. D., Rice, A. S. C., et al. (2011). A new definition of neuropathic pain. Pain 152 (10), 2204–2205. doi:10.1016/j.pain.2011.06.017
Kramer, M., Pierredon, S., Ribaux, P., Tille, J. C., Petignat, P., and Cohen, M. (2015). Secretome identifies tenascin-X as a potent marker of ovarian cancer. Biomed. Res. Int. 2015, 208017. doi:10.1155/2015/208017
Liang, G., Wang, S., Shao, J., Jin, Y., Xu, L., Yan, Y., et al. (2022). Tenascin-X mediates flow-induced suppression of EndMT and atherosclerosis. Circ. Res. 130, 1647–1659. doi:10.1161/circresaha.121.320694
Lindor, N. M., and Bristow, J. (2005). Tenascin-X deficiency in autosomal recessive Ehlers-Danlos syndrome. Am. J. Med. Genet. A 135 (1), 75–80. doi:10.1002/ajmg.a.30671
Liot, S., Aubert, A., Hervieu, V., Kholti, N. E., Schalkwijk, J., Verrier, B., et al. (2020). Loss of tenascin-X expression during tumor progression: A new pan-cancer marker. Matrix Biol. Plus 6-7, 100021. doi:10.1016/j.mbplus.2020.100021
Malfait, F., Castori, M., Francomano, C. A., Giunta, C., Kosho, T., and Byers, P. H. (2020). The Ehlers-Danlos syndromes. Nat. Rev. Dis. Prim. 6 (1), 64. doi:10.1038/s41572-020-0194-9
Malfait, F., Colman, M., Vroman, R., De Wandele, I., Rombaut, L., Miller, R. E., et al. (2021). Pain in the Ehlers-Danlos syndromes: Mechanisms, models, and challenges. Am. J. Med. Genet. C Semin. Med. Genet. 187 (4), 429–445. doi:10.1002/ajmg.c.31950
Malfait, F., Francomano, C., Byers, P., Belmont, J., Berglund, B., Black, J., et al. (2017). The 2017 international classification of the Ehlers-Danlos syndromes. Am. J. Med. Genet. C Semin. Med. Genet. 175 (1), 8–26. doi:10.1002/ajmg.c.31552
Mao, J. R., and Bristow, J. (2001). The Ehlers-Danlos syndrome: On beyond collagens. J. Clin. Invest. 107 (9), 1063–1069. doi:10.1172/jci12881
Mao, J. R., Taylor, G., Dean, W. B., Wagner, D. R., Afzal, V., Lotz, J. C., et al. (2002). Tenascin-X deficiency mimics Ehlers-Danlos syndrome in mice through alteration of collagen deposition. Nat. Genet. 30 (4), 421–425. doi:10.1038/ng850
Margaron, Y., Bostan, L., Exposito, J. Y., Malbouyres, M., Trunfio-Sfarghiu, A. M., Berthier, Y., et al. (2010). Tenascin-X increases the stiffness of collagen gels without affecting fibrillogenesis. Biophys. Chem. 147 (1-2), 87–91. doi:10.1016/j.bpc.2009.12.011
Matsumoto, K., and Aoki, H. (2020). The roles of tenascins in cardiovascular, inflammatory, and heritable connective tissue diseases. Front. Immunol. 11, 609752. doi:10.3389/fimmu.2020.609752
Matsumoto, K., Arai, M., Ishihara, N., Ando, A., Inoko, H., and Ikemura, T. (1992a). Cluster of fibronectin type III repeats found in the human major histocompatibility complex class III region shows the highest homology with the repeats in an extracellular matrix protein, tenascin. Genomics 12 (3), 485–491. doi:10.1016/0888-7543(92)90438-x
Matsumoto, K., Ishihara, N., Ando, A., Inoko, H., and Ikemura, T. (1992b). Extracellular matrix protein tenascin-like gene found in human MHC class III region. Immunogenetics 36 (6), 400–403. doi:10.1007/bf00218048
Matsumoto, K., Kawakami, K., Yamada, K., and Takeshita, H. (2022). COL1A1 expression induced by overexpression of both a 15-amino acid peptide from the fibrinogen domain of tenascin-X and integrin α11 in LX-2 cells. Mol. Med. Rep. 26 (5), 330. doi:10.3892/mmr.2022.12846
Matsumoto, K., Minamitani, T., Orba, Y., Sato, M., Sawa, H., and Ariga, H. (2004). Induction of matrix metalloproteinase-2 by tenascin-X deficiency is mediated through the c-Jun N-terminal kinase and protein tyrosine kinase phosphorylation pathway. Exp. Cell Res. 297 (2), 404–414. doi:10.1016/j.yexcr.2004.03.041
Matsumoto, K., Saga, Y., Ikemura, T., Sakakura, T., and Chiquet-Ehrismann, R. (1994). The distribution of tenascin-X is distinct and often reciprocal to that of tenascin-C. J. Cell Biol. 125 (2), 483–493. doi:10.1083/jcb.125.2.483
Matsumoto, K., Sawa, H., Sato, M., Orba, Y., Nagashima, K., and Ariga, H. (2002). Distribution of extracellular matrix tenascin-X in sciatic nerves. Acta. Neuropathol. 104 (5), 448–454. doi:10.1007/s00401-002-0577-x
Matsumoto, K., Takayama, N., Ohnishi, J., Ohnishi, E., Shirayoshi, Y., Nakatsuji, N., et al. (2001). Tumour invasion and metastasis are promoted in mice deficient in tenascin-X. Genes 6 (12), 1101–1111. doi:10.1046/j.1365-2443.2001.00482.x
Micale, L., Guarnieri, V., Augello, B., Palumbo, O., Agolini, E., Sofia, V. M., et al. (2019). Novel TNXB variants in two Italian patients with classical-like Ehlers-Danlos syndrome. Genes (Basel) 10 (12), 967. doi:10.3390/genes10120967
Minamitani, T., Ariga, H., and Matsumoto, K. (2004a). Deficiency of tenascin-X causes a decrease in the level of expression of type VI collagen. Exp. Cell Res. 297 (1), 49–60. doi:10.1016/j.yexcr.2004.03.002
Minamitani, T., Ikuta, T., Saito, Y., Takebe, G., Sato, M., Sawa, H., et al. (2004b). Modulation of collagen fibrillogenesis by tenascin-X and type VI collagen. Exp. Cell Res. 298 (1), 305–315. doi:10.1016/j.yexcr.2004.04.030
Moehring, F., Halder, P., Seal, R. P., and Stucky, C. L. (2018). Uncovering the cells and circuits of touch in normal and pathological settings. Neuron 100 (2), 349–360. doi:10.1016/j.neuron.2018.10.019
Morel, Y., Bristow, J., Gitelman, S. E., and Miller, W. L. (1989). Transcript encoded on the opposite strand of the human steroid 21-hydroxylase/complement component C4 gene locus. Proc. Natl. Acad. Sci. USA 86 (17), 6582–6586. doi:10.1073/pnas.86.17.6582
Nakayama, K., Seike, M., Noro, R., Takeuchi, S., Matsuda, K., Kunugi, S., et al. (2019). Tenascin XB is a novel diagnostic marker for malignant mesothelioma. Anticancer Res. 39 (2), 627–633. doi:10.21873/anticanres.13156
Okuda-Ashitaka, E., Kakuchi, Y., Kakumoto, H., Yamanishi, S., Kamada, H., Yoshidu, T., et al. (2020). Mechanical allodynia in mice with tenascin-X deficiency associated with Ehlers-Danlos syndrome. Sci. Rep. 10 (1), 6569. doi:10.1038/s41598-020-63499-2
Sakai, H., Yokota, S., Kajitani, N., Yoneyama, T., Kawakami, K., Yasui, Y., et al. (2017). A potential contribution of tenascin-X to blood vessel formation in peripheral nerves. Neurosci. Res. 124, 1–7. doi:10.1016/j.neures.2017.06.003
Sakai, T., Furukawa, Y., Chiquet-Ehrismann, R., Nakamura, M., Kitagawa, S., Ikemura, T., et al. (1996). Tenascin-X expression in tumor cells and fibroblasts: Glucocorticoids as negative regulators in fibroblasts. J. Cell Sci. 109 (8), 2069–2077. doi:10.1242/jcs.109.8.2069
Schalkwijk, J., Zweers, M. C., Steijlen, P. M., Dean, W. B., Taylor, G., van Vlijmen, I. M., et al. (2001). A recessive form of the Ehlers-Danlos syndrome caused by tenascin-X deficiency. N. Engl. J. Med. 345 (16), 1167–1175. doi:10.1056/NEJMoa002939
Sumioka, T., Iwanishi, H., Okada, Y., Miyajima, M., Ichikawa, K., Reinach, P. S., et al. (2021). Impairment of corneal epithelial wound healing is association with increased neutrophil infiltration and reactive oxygen species activation in tenascin X-deficient mice. Lab. Invest. 101 (6), 690–700. doi:10.1038/s41374-021-00576-8
Sumioka, T., Iwanishi, H., Okada, Y., Nidegawa, Y., Miyajima, M., Matsumoto, K., et al. (2018). Loss of tenascin X gene function impairs injury-induced stromal angiogenesis in mouse corneas. J. Cell. Mol. Med. 22 (2), 948–956. doi:10.1111/jcmm.13397
Syx, D., De Wandele, I., Rombaut, L., and Malfait, F. (2017). Hypermobility, the Ehlers-Danlos syndromes and chronic pain. Clin. Exp. Rheumatol. 35 (5), 116–122.
Syx, D., Miller, R. E., Obeidat, A. M., Tran, P. B., Vroman, R., Malfait, Z., et al. (2020).Pain-related behaviors and abnormal cutaneous innervation in a murine model of classical Ehlers-Danlos syndrome. Pain. 2274–2283. doi:10.1097/j.pain.0000000000001935
Takeda, K., Shiba, H., Mizuno, N., Hasegawa, N., Mouri, Y., Hirachi, A., et al. (2005). Brain-derived neurotrophic factor enhances periodontal tissue regeneration. Tissue Eng. 11 (9-10), 1618–1629. doi:10.1089/ten.2005.11.1618
van Dijk, F. S., Ghali, N., Demirdas, S., and Baker, D. (2022). “TNXB-related classical-like Ehlers-Danlos syndrome,” in GeneReviews(®). M. P. Adam, D. B. Everman, G. M. Mirzaa, R. A. Pagon, S. E. Wallace, L. J. H. Beanet al. (Seattle (WA): University of Washington, Seattle, Copyright © 1993-2022, University of Washington, Seattle. GeneReviews is a registered trademark of the University of Washington, Seattle. All rights reserved).
Voermans, N. C., van Alfen, N., Pillen, S., Lammens, M., Schalkwijk, J., Zwarts, M. J., et al. (2009). Neuromuscular involvement in various types of Ehlers-Danlos syndrome. Ann. Neurol. 65 (6), 687–697. doi:10.1002/ana.21643
Voermans, N. C., Verrijp, K., Eshuis, L., Balemans, M. C., Egging, D., Sterrenburg, E., et al. (2011). Mild muscular features in tenascin-X knockout mice, a model of Ehlers-danlos syndrome. Connect. Tissue Res. 52 (5), 422–432. doi:10.3109/03008207.2010.551616
Whalen, K. C., and Crone, W. (2022). Multidisciplinary approach to treating chronic pain in patients with Ehlers-Danlos syndrome: Critically appraised topic. J. Pain Res. 15, 2893–2904. doi:10.2147/jpr.S377790
Yamaguchi, S., Kawakami, K., Satoh, K., Fukunaga, N., Akama, K., and Matsumoto, K. I. (2017). Suppression of hepatic dysfunction in tenascin-X-deficient mice fed a high-fat diet. Mol. Med. Rep. 16 (4), 4061–4067. doi:10.3892/mmr.2017.7052
Yan, F., Liu, S. W., Li, X. Y., Li, C. C., and Wu, Y. (2020). Silencing lncRNA LINC01305 inhibits epithelial mesenchymal transition in lung cancer cells by regulating TNXB-mediated PI3K/Akt signaling pathway. J. Biol. Regul. Homeost. Agents 34 (2), 499–508. doi:10.23812/20-73-a-33
Yang, N., Tian, J., Wang, X., Mei, S., Zou, D., Peng, X., et al. (2020). A functional variant in TNXB promoter associates with the risk of esophageal squamous-cell carcinoma. Mol. Carcinog. 59 (4), 439–446. doi:10.1002/mc.23166
Yuan, Y., Nymoen, D. A., Stavnes, H. T., Rosnes, A. K., Bjørang, O., Wu, C., et al. (2009). Tenascin-X is a novel diagnostic marker of malignant mesothelioma. Am. J. Surg. Pathol. 33 (11), 1673–1682. doi:10.1097/PAS.0b013e3181b6bde3
Keywords: tenascin-X, Ehlers-Danlos syndromes, clEDS, pain, tumor suppressor, fibrosis
Citation: Okuda-Ashitaka E and Matsumoto K-i (2023) Tenascin-X as a causal gene for classical-like Ehlers-Danlos syndrome. Front. Genet. 14:1107787. doi: 10.3389/fgene.2023.1107787
Received: 25 November 2022; Accepted: 06 March 2023;
Published: 15 March 2023.
Edited by:
Mahmood Rasool, King Abdulaziz University, Saudi ArabiaReviewed by:
Antonella Polimeni, Sapienza University of Rome, ItalyCopyright © 2023 Okuda-Ashitaka and Matsumoto. This is an open-access article distributed under the terms of the Creative Commons Attribution License (CC BY). The use, distribution or reproduction in other forums is permitted, provided the original author(s) and the copyright owner(s) are credited and that the original publication in this journal is cited, in accordance with accepted academic practice. No use, distribution or reproduction is permitted which does not comply with these terms.
*Correspondence: Emiko Okuda-Ashitaka, emiko.ashitaka@oit.ac.jp; Ken-ichi Matsumoto, matumoto@med.shimane-u.ac.jp