- 1Department of Nephrology, Xiangya Hospital, Central South University, Changsha, China
- 2Hunan Key Laboratory of Organ Fibrosis, Central South University, Changsha, China
- 3National Clinical Research Center for Geriatric Disorders, Xiangya Hospital, Central South University, Changsha, China
- 4Department of Pediatrics, Xiangya Hospital, Central South University, Changsha, China
- 5Department of Orthopedics, Xiangya Hospital, Central South University, Changsha, China
Short stature (OMIM: 165800) is a common pediatric disorder. Any abnormality in the cartilage formation of the growth plate can cause short stature. Aggrecan, encoded by ACAN, is an important component of the extracellular matrix. Mutations in ACAN have been reported to cause short stature. In the present study, we enrolled a Chinese family with short stature and advanced bone age across three generations. Whole-exome sequencing (WES) was performed on the proband to detect the candidate genes causing short stature in family. A novel heterozygous frameshift mutation (NM_013227.3:c.7230delT; NP_001356197.1: p. Phe2410Leufs*9) of the ACAN gene was confirmed to be a genetic lesion in this family. This variant, which was located in a functional site globular 3 (G3) domain of ACAN and predicted to be deleterious by informatics programs, was co-segregated with the affected family members by performing Sanger sequencing. Literatures review of growth hormone (GH) treatment outcome of all previously reported ACAN patients suggesting that the G3 domain of ACAN may be critical in the development of short stature and growth hormone treatment. These findings not only contribute to the genetic diagnosis and counseling of the family, but will also expand the mutation spectrum of ACAN.
1 Introduction
Short stature (OMIM: 165800) is a type of condition that is associated with a child whose height is 2 standard deviations (SD) or more below the mean for children of the same chronological age and sex (Baron et al., 2015; Finken et al., 2018; Grunauer and Jorge, 2018). The growth plate is the target organ of linear growth and height gain (Baron et al., 2015); it is composed of chondrocytes embedded in a collagen matrix that show high metabolic activity. Any abnormality in the cartilage formation of the growth plate can cause linear growth disturbance, which in turn, may lead to short stature.
Existing research suggests that defects in the genes encoding components of either paracrine regulatory systems or the extracellular matrix (ECM), and those encoding intracellular or endocrine proteins result in short stature (Jee et al., 2017). The gene encoding aggrecan (ACAN, OMIM: 155760) is located on chromosome 15q26, ACAN encodes the production of the proteoglycan aggrecan, which is an important component of the ECM (Stavber et al., 2020). Aggrecan is highly expressed in proliferating chondrocytes of the growth plate. The structural or functional disturbances in aggrecan production could significantly affect cartilage, resulting in changes in linear growth. Statistically, in 1%–5% of idiopathic short stature patients, the short stature can be explained by ACAN mutations (Jee et al., 2018).
Herein, we enrolled a Han Chinese family with short stature and advanced bone age (BA). Using whole-exome sequencing (WES) and Sanger sequencing, a novel heterozygous frameshift mutation (NM_013227.3:c.7230delT; NP_001356197.1: p. Phe2410Leufs*9) of the ACAN gene was confirmed to be a genetic lesion in this family.
2 Case description
The proband, a 4-year-old boy, came to the Xiangya Hospital Central South University with his parents due to growth retardation (Figure 1A). Physical examination of the proband showed a height of 94.8 cm (−2.02 SDS), and his weight was 14.6 kg (−1.29 SDS). According to his parents’ recollection, the height of the proband was 70 cm at 12 months of age (−2.40 SDS) and 85 cm at 30 months old (−2.03 SDS). Magnetic resonance imaging revealed a normal size and shape of the pituitary gland. The BA was found to be 5 years and 6 months, as revealed by the X-ray imaging of the left hand (Figure 1B).
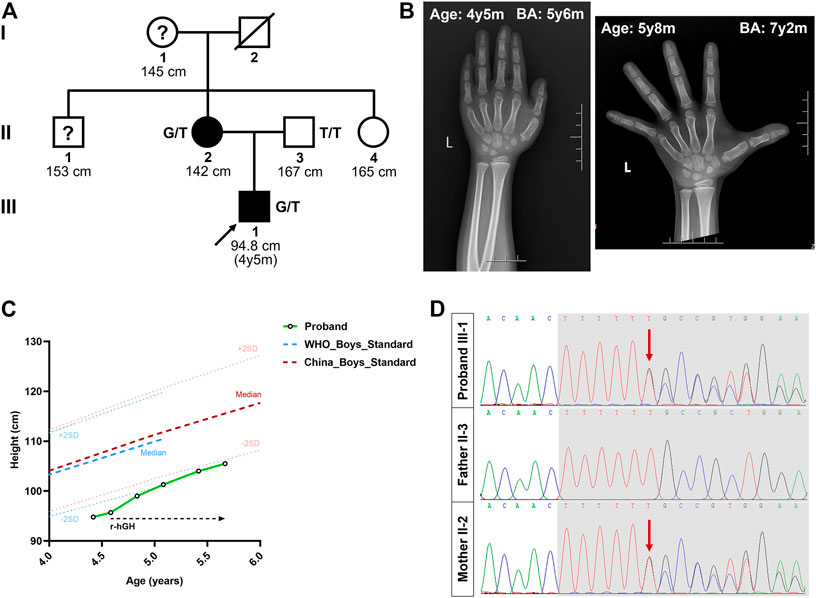
FIGURE 1. Pedigree of the Chinese family with short stature. (A) The black circles/squares represent the affected patients diagnosed with short stature diagnosis; the white circles/squares represent the unaffected subjects. The arrow indicates the proband. The question mark indicates subject with unknown genotype but known phenotype. (B) X-ray imaging of the proband’s left hand at the age of 4 years 5 months and 5 years 8 months. (C) The height growth curve of the proband during the one-year follow-up. (D) The sequencing results of the ACAN mutations. Sequence chromatograms indicate the heterozygosity for a ACAN frameshift mutation (NM_013227.3:c.7230delT; NP_001356197.1:p.Phe2410Leufs*9) in the proband and his mother. The red arrow indicates the mutation. SDS, standard deviation scores; r-hGH, Recombinant human growth hormone; y, year; m, month; BA, bone age.
We then investigated the proband’s family history. His father (II-3) was 167 cm tall (≈−1.31 SDS), and his mother (II-2) was 142 cm tall (≈−3.23 SDS) (the target height of the proband was 161 ± 5 cm). His mother’s age at menarche was approximately 12 years. Meanwhile, the heights of his grandmother (I-1), maternal uncle (II-1), and maternal aunt (II-4) were 145 cm (≈−2.78 SDS), 153 cm (≈−3.23 SDS), and 165 cm (≈0.28 SDS), respectively (Figure 1A). No other malformations, including intervertebral disc disease, osteochodritis dissecans or back pain, were observed in the affected members of the family.
At the request of his parents, recombinant human growth hormone (GH) treatment was initiated after the proband’s second visit, at a dose of 50 μg/kg/day. During this time, the height of the proband increased to 99.0 cm at 4 years 10 months (−2.17 SDS), 101.3 cm at 5 years 1 month (−1.95 SDS), 104.0 cm at 5 years and 5 months (−1.78 SDS), and 105.5 cm at 5 years and 8 months (−1.76 SDS) (Figure 1C). The growth velocity was also changed from 5.42 cm/year to 6.00 cm/year, after 13 months of GH treatment. Meanwhile, his weight increased to 18.4 kg (−0.52 SDS) and the BA was 8 years during his latest visit, at the age of 5 years 8 months (Figure 1B).
To determine the genetic cause of the short stature in these patients, we extracted the genomic DNA of the proband (III-1) and his mother (II-2). Next, WES was performed to detect the candidate genes associated with short stature and advanced BA. The filtering strategies conformed to those described in our previous study (Huang et al., 2022). Briefly, (a) Variants within intronic, intergenic, and untranslated regions (UTRs), as well as synonymous single nucleotide variants (SNVs), were excluded from later analysis; (b) Common variants were excluded with minor allele frequency ≥0.01 by the 1000 Genomes database, Genome Aggregation Database (gnomAD), version 2.1.1 and an in-house exome database of Berry Genomics; (c) Variants classified as Pathogenic/Likely Pathogenic variants, as well as variant of unknown significance (VUS) by American College of Medical Genetics (ACMG) classification (Richards et al., 2015), could be included; (d) Potential causative variants were then screened by a list of growth-associated genes (Supplementary Table S1) (Hauer et al., 2018); (e) Variants that were not existed in the affected family member (II-2) were excluded; (f) Bioinformatics analysis, including Polyphen-2, MutationTaster, SIFT, and CADD, were also carried out to predict the possible impacts of variants; (g) Accordingly, co-segregation analysis was conducted in the family (Supplementary Figure S1).
After data filtering of (a), (b), (c), (d), and (e), only a set of 7 variants in seven genes in the proband were identified (Supplementary Figure S1, Supplementary Tables S2, S3). Moreover, during further filtering of bioinformatic prediction and co-segregation analysis, a novel heterozygous frameshift mutation (NM_013227.3:c.7230delT; NP_001356197.1: p. Phe2410Leufs*9) in exon 16 of ACAN was identified in the proband (Figure 1D). No other significant variant related to short stature or advanced BA was identified. This variant of c.7230delT/p.Phe2410Leufs*9 in the ACAN gene was also predicted to be deleterious by multiple bioinformatics software (MutationTaster and CADD).
Sanger sequencing revealed that the c.7230delT/p.Phe2410Leufs*9 variant of ACAN co-segregated with the affected family members (I-1, II-1 and II-2). In addition, this novel ACAN mutation was not found in the 200 local control subjects from the control cohorts. Based on the ACMG guidelines, the variant of ACAN (c.7230delT; p. Phe2410Leufs*9) can be classified as likely pathogenic (PVS1_Moderate + PM1+PM2+PP3).
3 Discussion
Short stature is a common clinical manifestation in children. Genetic inheritance is the main determinant of stature (Grunauer and Jorge, 2018). The mutations in ACAN gene are a major cause of idiopathic short stature. Aggrecan is a chondroitin sulfate proteoglycan, that is also an important component of the ECM. Aggrecan is encoded by ACAN. Animal experiments shown that dysfunction of the chick and mouse aggrecan can lead to severe chondrodystrophies with perinatal lethality (Li et al., 1993). Moreover, the expression levels of important regulatory genes, including Col10a1, Sox9, and Ihh, were sharply reduced in ACAN knockout mice (Lauing et al., 2014). In humans, mutations in the ACAN gene are known to cause chondrodysplasia and inherited short stature syndromes associated with accelerated bone maturation (Gibson and Briggs, 2016; Kim et al., 2020; Uchida et al., 2020; Wei et al., 2021). At present, only 65 missense/non-sense and 26 small deletion/insertion variants in the ACAN gene that are associated with short stature have been recorded in the Human Gene Mutation Database (HGMD; data retrieved in November 2021).
Aggrecan contains three globular domains (G1 and, G2 at the N-terminus and G3 at the C-terminal), one keratan sulfate domain, and one chondroitin sulfate domain. It also contains two epidermal growth factor-like motifs, one C-type lectin (CLD), and one complement regulatory protein-like motif in the G3 domain (Tompson et al., 2009; Liang et al., 2020). Previous studies have shown that CLD may mediate the interaction between aggrecan and tenascin in coordination with Ca2+ (Lundell et al., 2004; Diao and Tajkhorshid, 2008). Mutations of the ACAN gene (p.Phe2410Leufs*9), which was also located in the CLD domain of G3 (Figure 2A), may lead to the dysfunction of these interactions. Noticeably, Gkourogianni et al. also described an ACAN non-sense mutation (p.Trp2401*) that located in the CLD domain and led to short stature and early-onset osteoarthritis (Gkourogianni et al., 2017). Our study may provide additional evidence for the role of CLD in cartilage development.
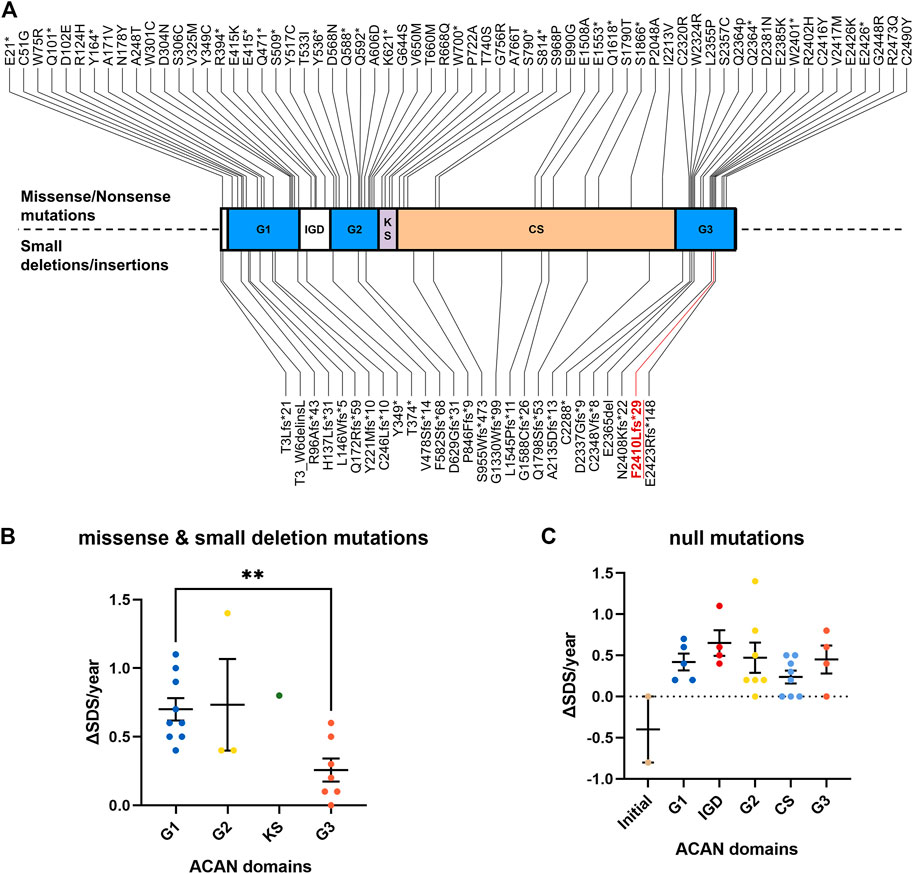
FIGURE 2. Overview of all known ACAN mutations. (A) The red font symbolizes the mutation identified in this study. (B) The increasing SDS for each year (ΔSDS/year) of patients with ACAN missense and small deletion mutations during GH treatment. (C) The increasing SDS for each year (ΔSDS/year) of patients with ACAN null mutations during GH treatment. G1, globular domain 1; IGD, interglobular domain; G2, globular domain 2; KS, keratin sulfate attachment domain; CS, chondroitin sulfate attachment domain; G3, globular domain 3; SDS, standard deviation scores. **p<0.01.
In this study, we used WES to identify a novel p. Phe2410Leufs*9 frameshift mutation in exon 16 of ACAN in a family with short stature and advanced BA. Treatment with GH was performed since his age of 4 years and 7 months. However, the effectiveness of this treatment was limited during the first year of therapy (Figure 1C). Since GH facilitates growth by promoting the release of insulin-like growth factor 1, thus inducing chondrocyte proliferation and hypertrophy (Xu et al., 2018), treatment for a longer time and/or combination therapy may need to restore the normal ECM assembly and improve the height of the patient (van der Steen et al., 2017; Lin et al., 2021).
To clarify the relationship between ACAN mutations and the outcome of GH treatment, we reviewed all previously reported ACAN patients and their prognosis data during GH treatment (Table 1). A total of 49 ACAN patients was included. Most of these ACAN patients were firstly cued with different dose of GH (from 30 to 66 μg/kg/day) at age of 5–9. The increasing SDS for each year (ΔSDS/year) were calculated after their GH treatment. The range of ΔSDS/year was from −0.8 to 1.4 and the duration of GH treatment was from 1.5 months to 97 months. As we summarized, for missense and small deletion mutations, patients with ACAN mutations in G3 domain showed significant lower ΔSDS/year compared with other ACAN domains (Figure 2B). Especially the cases in Xu et al. (Xu et al., 2018) (Gln2364Pro) and Gkourogianni et al. (Gkourogianni et al., 2017) (Leu2355Pro), the ΔSDS/year were 0.0 or 0.3 even with a relative high dose GH (>40 μg/kg/day) and long duration time of treatment (91 or 18 months, respectively) (Table 1). When we compared the therapeutic differences between non-sense and frameshift mutations in each domain, most of these patients showed a similar GH sensitivity (Figure 2C). We also compared the ΔSDS/year data from who received GH combined with or without gonadotropin-releasing hormone analog (GnRHa). However, due to the limited cases, we found no difference. An example from Xu et al. (Xu et al., 2018) showed the ΔSDS/year only have a slight changed in the same ACAN mutation (Gln2364Pro) patients that treatment with or without GnRHa (Table 1). Which may suggest the little effect of GnRHa treatment in ACAN patients. However, much more clinical evidence is still demanded. All combined, the above information of point and null variants both suggesting G3, as the last functional domain of ACAN, may be critical in the development of short stature and GH treatment.
In summary, we report a novel mutation in ACAN (c.7230delT; p. Phe2410Leufs*9) in a Chinese family with short stature and advanced BA. Our analyses not only further confirmed the clinical diagnosis of short stature in the patients, but also expanded the mutation spectrum of ACAN and contributed to the genetic diagnosis and counseling of patients with short stature.
Data availability statement
The data that support the findings of this study are not publicly available because they contain information that could compromise the privacy of research participants; however, these data are available from the corresponding author upon reasonable request. The variation information has been submitted to ClinVar (SCV002819138).
Ethics statement
The studies involving human participants were reviewed and approved by the The Review Board of the Xiangya Hospital of Central South University. Written informed consent to participate in this study was provided by the participants’ legal guardian/next of kin. Written informed consent was obtained from the individual (s), and minor (s)’ legal guardian/next of kin, for the publication of any potentially identifiable images or data included in this article.
Author contributions
XW and RX conceived and directed the project. HH collected the data and information. HH and JJ analyzed and interpreted the data. HH wrote the manuscript with the help of all the other authors. All authors contributed to the manuscript and approved the submitted version.
Funding
This study was supported by the National Natural Science Foundation of China (81970403); China Postdoctoral Science Foundation (2020TQ0363 and 2020M682598); the Natural Science Foundation of Hunan Province (2021JJ40992); the Emergency Project of Prevention and Control for COVID-19 of Central South University (160260003), and the Youth Science Foundation of Xiangya Hospital (2021Q11).
Acknowledgments
We thank the patients and their family members for their participation in this study.
Conflict of interest
The authors declare that the research was conducted in the absence of any commercial or financial relationships that could be construed as a potential conflict of interest.
Publisher’s note
All claims expressed in this article are solely those of the authors and do not necessarily represent those of their affiliated organizations, or those of the publisher, the editors and the reviewers. Any product that may be evaluated in this article, or claim that may be made by its manufacturer, is not guaranteed or endorsed by the publisher.
Supplementary material
The Supplementary Material for this article can be found online at: https://www.frontiersin.org/articles/10.3389/fgene.2023.1101695/full#supplementary-material
References
Baron, J., Savendahl, L., De Luca, F., Dauber, A., Phillip, M., Wit, J. M., et al. (2015). Short and tall stature: A new paradigm emerges. Nat. Rev. Endocrinol. 11, 735–746. doi:10.1038/nrendo.2015.165
Diao, J., and Tajkhorshid, E. (2008). Indirect role of Ca2+ in the assembly of extracellular matrix proteins. Biophys. J. 95, 120–127. doi:10.1529/biophysj.108.129106
Finken, M., van der Steen, M., Smeets, C., Walenkamp, M., de Bruin, C., Hokken-Koelega, A., et al. (2018). Children born small for gestational age: Differential diagnosis, molecular genetic evaluation, and implications. Endocr. Rev. 39, 851–894. doi:10.1210/er.2018-00083
Gibson, B. G., and Briggs, M. D. (2016). The aggrecanopathies; an evolving phenotypic spectrum of human genetic skeletal diseases. ORPHANET J. RARE Dis. 11, 86. doi:10.1186/s13023-016-0459-2
Gkourogianni, A., Andrew, M., Tyzinski, L., Crocker, M., Douglas, J., Dunbar, N., et al. (2017). Clinical characterization of patients with autosomal dominant short stature due to aggrecan mutations. J. Clin. Endocr. Metab. 102, 460–469. doi:10.1210/jc.2016-3313
Grunauer, M., and Jorge, A. (2018). Genetic short stature. GROWTH Horm. IGF Res. 38, 29–33. doi:10.1016/j.ghir.2017.12.003
Hauer, N. N., Popp, B., Schoeller, E., Schuhmann, S., Heath, K. E., Hisado-Oliva, A., et al. (2018). Clinical relevance of systematic phenotyping and exome sequencing in patients with short stature. Genet. Med. 20, 630–638. doi:10.1038/gim.2017.159
Huang, H., Chen, Y., Jin, J., Du, R., Tang, K., Fan, L., et al. (2022). CSRP3, p.Arg122*, is responsible for hypertrophic cardiomyopathy in a Chinese family. J. GENE Med. 24, e3390. doi:10.1002/jgm.3390
Jee, Y. H., Andrade, A. C., Baron, J., and Nilsson, O. (2017). Genetics of short stature. ENDOCRIN Metab. Clin. 46, 259–281. doi:10.1016/j.ecl.2017.01.001
Jee, Y. H., Baron, J., and Nilsson, O. (2018). New developments in the genetic diagnosis of short stature. Curr. Opin. Pediatr. 30, 541–547. doi:10.1097/MOP.0000000000000653
Kim, T. Y., Jang, K. M., Keum, C. W., Oh, S. H., and Chung, W. Y. (2020). Identification of a heterozygous ACAN mutation in a 15-year-old boy with short stature who presented with advanced bone age: A case report and review of the literature. Ann. Pediatr. ENDOCRIN 25, 272–276. doi:10.6065/apem.1938198.099
Lauing, K. L., Cortes, M., Domowicz, M. S., Henry, J. G., Baria, A. T., and Schwartz, N. B. (2014). Aggrecan is required for growth plate cytoarchitecture and differentiation. Dev. Biol. 396, 224–236. doi:10.1016/j.ydbio.2014.10.005
Li, H., Schwartz, N. B., and Vertel, B. M. (1993). cDNA cloning of chick cartilage chondroitin sulfate (aggrecan) core protein and identification of a stop codon in the aggrecan gene associated with the chondrodystrophy, nanomelia. J. Biol. Chem. 268, 23504–23511. doi:10.1016/s0021-9258(19)49491-x
Liang, H., Miao, H., Pan, H., Yang, H., Gong, F., Duan, L., et al. (2020). Growth-promoting therapies may Be useful in short stature patients with nonspecific skeletal abnormalities caused by acan heterozygous mutations: Six Chinese cases and literature review. Endocr. Pract. 26, 1255–1268. doi:10.4158/EP-2019-0518
Lin, L., Li, M., Luo, J., Li, P., Zhou, S., Yang, Y., et al. (2021). A high proportion of novel ACAN mutations and their prevalence in a large cohort of Chinese short stature children. J. Clin. Endocr. Metab. 106, e2711–e2719. doi:10.1210/clinem/dgab088
Lundell, A., Olin, A. I., Morgelin, M., Al-Karadaghi, S., Aspberg, A., and Logan, D. T. (2004). Structural basis for interactions between tenascins and lectican C-type lectin domains: Evidence for a crosslinking role for tenascins. STRUCTURE 12, 1495–1506. doi:10.1016/j.str.2004.05.021
Richards, S., Aziz, N., Bale, S., Bick, D., Das, S., Gastier-Foster, J., et al. (2015). Standards and guidelines for the interpretation of sequence variants: A joint consensus recommendation of the American College of medical genetics and genomics and the association for molecular pathology. Genet. Med. 17, 405–424. doi:10.1038/gim.2015.30
Stavber, L., Hovnik, T., Kotnik, P., Lovrecic, L., Kovac, J., Tesovnik, T., et al. (2020). High frequency of pathogenic ACAN variants including an intragenic deletion in selected individuals with short stature. Eur. J. Endocrinol. 182, 243–253. doi:10.1530/EJE-19-0771
Tompson, S. W., Merriman, B., Funari, V. A., Fresquet, M., Lachman, R. S., Rimoin, D. L., et al. (2009). A recessive skeletal dysplasia, SEMD aggrecan type, results from a missense mutation affecting the C-type lectin domain of aggrecan. Am. J. Hum. Genet. 84, 72–79. doi:10.1016/j.ajhg.2008.12.001
Uchida, N., Shibata, H., Nishimura, G., and Hasegawa, T. (2020). A novel mutation in the ACAN gene in a family with autosomal dominant short stature and intervertebral disc disease. Hum. GENOME Var. 7, 44. doi:10.1038/s41439-020-00132-8
van der Steen, M., Pfundt, R., Maas, S., Bakker-van, W. W., Odink, R. J., and Hokken-Koelega, A. (2017). ACAN gene mutations in short children born SGA and response to growth hormone treatment. J. Clin. Endocr. Metab. 102, 1458–1467. doi:10.1210/jc.2016-2941
Wei, M., Ying, Y., Li, Z., Weng, Y., and Luo, X. (2021). Identification of novel ACAN mutations in two Chinese families and genotype-phenotype correlation in patients with 74 pathogenic ACAN variations. Mol. Genet. GENOM Med. 9, e1823. doi:10.1002/mgg3.1823
Keywords: short stature, novel mutation, acan, whole-exome sequencing, frameshift, case report
Citation: Huang H, Jin J, Xiang R and Wang X (2023) Case report: A novel heterozygous frameshift mutation of ACAN in a Chinese family with short stature and advanced bone age. Front. Genet. 14:1101695. doi: 10.3389/fgene.2023.1101695
Received: 18 November 2022; Accepted: 10 March 2023;
Published: 21 March 2023.
Edited by:
Fang Fang Yu, Zhengzhou University, ChinaReviewed by:
Xuyun Hu, Beijing Children’s Hospital, Capital Medical University, ChinaJose Bernardo Quintos, Rhode Island Hospital, United States
Copyright © 2023 Huang, Jin, Xiang and Wang. This is an open-access article distributed under the terms of the Creative Commons Attribution License (CC BY). The use, distribution or reproduction in other forums is permitted, provided the original author(s) and the copyright owner(s) are credited and that the original publication in this journal is cited, in accordance with accepted academic practice. No use, distribution or reproduction is permitted which does not comply with these terms.
*Correspondence: Rong Xiang, c2hpcmxlc21pbGVAY3N1LmVkdS5jbiYjeDAyMDBhOw==; Xia Wang, eGlhd2FuZ0Bjc3UuZWR1LmNu